Abstract
Tungsten is a relatively rare metal with numerous applications, most notably in machine tools, catalysts, and superalloys. In 2003, tungsten was nominated for study under the National Toxicology Program, and in 2011, it was nominated for human health assessment under the US Environmental Protection Agency's (EPA) Integrated Risk Information System. In 2005, the Agency for Toxic Substances and Disease Registry (ATSDR) issued a toxicological profile for tungsten, identifying several data gaps in the hazard assessment of tungsten. By filling the data gaps identified by the ATSDR, this review serves as an update to the toxicological profile for tungsten and tungsten substances. A PubMed literature search was conducted to identify reports published during the period 2004–2014, in order to gather relevant information related to tungsten toxicity. Additional information was also obtained directly from unpublished studies from within the tungsten industry. A systematic approach to evaluate the quality of data was conducted according to published criteria. This comprehensive review has gathered new toxicokinetic information and summarizes the details of acute and repeated-exposure studies that include reproductive, developmental, neurotoxicological, and immunotoxicological endpoints. Such new evidence involves several relevant studies that must be considered when regulators estimate and propose a tungsten reference or concentration dose.
Introduction
Tungsten, also known as wolfram, is a rare metal with the chemical symbol W, atomic number 74, and molecular weight of 183.84. The most common formal oxidation state of tungsten is + 6, but it exhibits all oxidation states from − 2 to + 6. Its important ores include wolframite and scheelite. The free element is remarkable for its robustness, especially because it has the highest melting point of all metals (3422 ± 15°C). Tungsten and tungsten alloys have numerous applications, most notably in hard metals used in cutting and drilling tools, X-ray tubes (as both the filament and target), electrodes in welding, and superalloys. Because of tungsten's hardness and high density (19.3 times that of water), it has military applications in penetrating projectiles. Tungsten substances are often used industrially as catalysts.
The processing of tungsten from the raw materials into tungsten substances and finished products is depicted in . Production starts with the dissolution of the tungsten ore concentrates wolframite and scheelite by alkaline pressure digestion, using either sodium carbonate or a concentrated sodium hydroxide solution. The sodium tungstate (ST) solution obtained is purified before it is converted into ammonium paratungstate (APT) of high purity. A common secondary source of tungsten is tungsten-bearing scrap, such as used drill bits and tools, which is oxidized and then chemically digested to also form APT, which is the main tungsten substance traded in the market and the main tungsten intermediate. APT is usually calcined to tungsten trioxide (WO3; tungsten yellow oxide) or tungsten oxides (WO3-x; tungsten blue oxide), which are not defined chemical substances but rather a mixture of oxide species including WO3, W20O58, W18O49, tungsten bronze [(NH4)nWO3 (0.06 < n < 0.33)], and an amorphous material undefined by X-ray diffraction analysis. The oxygen index (molar ratio between oxygen and tungsten) depends on calcination parameters such as temperature, heating time, composition, atmospheric pressure, mass of APT flow with time, gas flow, layer height in the furnace, and furnace slope and rotation rate (CitationLassner and Schubert 1999).
Figure 1. Flow chart depicting the processing from tungsten concentrates (raw materials) to tungsten intermediates or final products, each with different water solubility characteristics, ranging from (*) soluble (sodium tungstate, ammonium paratungstate, and ammonium metatungstate) to (†) sparingly soluble (tungsten yellow oxide, tungsten blue oxide, tungsten metal, and tungsten carbide). (‡) Tungsten chemicals include tungsten disulfide (WS2), which is synthesized from tungsten metal and sulfur.
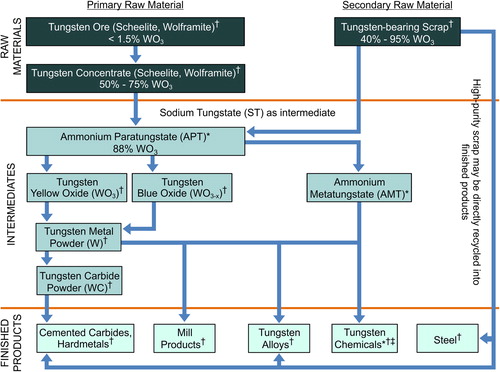
WO3 or WO3-x can be reduced at 700–1000°C to tungsten metal (W-metal) powder by hydrogen. By reaction with pure carbon powder in a process called carburization, most of the W-metal powder is converted to tungsten monocarbide, which is commonly known as tungsten carbide (WC). By mass, quantitatively, WC is the most important tungsten substance. Because of its hardness, it is the main constituent, together with cobalt, in cemented carbide (also known as hardmetal), which is a composite of tungsten carbide embedded in a matrix of metallic cobalt. By melting together W-metal and WC, a eutectic composition is formed of WC and ditungsten carbide (W2C), also known as fused tungsten carbide or casted tungsten carbide.
The occurrence of a cluster of cases of acute childhood lymphocytic leukemia in Fallon, Nevada, prompted a wide investigation that involved several local, state, and federal agencies led by the Centers of Disease Control and Prevention (CDC) (CitationCDC 2003). Groundwater is the source of drinking water in this community, and during this investigation, tungsten was detected in drinking water and in biological samples (CitationRubin et al. 2007). Principal sources of tungsten in ground water in this area of Nevada are natural and include erosion of tungsten-bearing mineral deposits (CitationSeiler et al. 2005). Although no direct link between tungsten and the incidence of leukemia was found (CitationCDC 2003, CitationRubin et al. 2007), in 2003, tungsten was selected for study under the National Toxicology Program (NTP), and in 2011, it was nominated by the US EPA for risk assessment under the Integrated Risk Information System (IRIS) program.
In 2005, the ATSDR issued its toxicological profile for tungsten (CitationKeith et al. 2005, Citation2007), identifying several data gaps in areas such as acute inhalation, oral and dermal toxicities, skin and eye irritation, as well as dermal sensitization, toxicity due to repeated subchronic and chronic oral and inhalation exposure, genotoxicity, reproductive and developmental toxicity, neurotoxicity, and immunotoxicity. In addition, for some of the endpoints in the health-effect assessment (e.g. inhalation toxicity), the ATDSR included health effects that arise predominantly from occupational exposure to dust that includes particles of tungsten and other metals (particularly cobalt), at facilities in which hardmetal is produced.
To fill the data gaps identified in the 2005 ATSDR toxicological profile for tungsten (CitationKeith et al. 2005, Citation2007), we reviewed the scientific research conducted since 2004 on water-soluble [e.g. ST, ammonium metatungstate (AMT), sodium metatungstate (SMT), and APT] and sparingly water-soluble [e.g. W-metal, WC, W2C, WO3, WO3-x, and tungsten disulfide (WS2)] tungsten substances. In addition, studies sponsored by the government and by the tungsten industry that are dated pre- and post-2005, which were not included in the ATSDR's toxicological assessment, were also reviewed, as they also help fill the data gaps identified in the ATSDR profile. Unlike the ATSDR profile, our review mainly considers relevant research on tungsten and tungsten substances, and it does not include research on alloys containing tungsten, such as hardmetal, or studies of exposure to tungsten with co-exposures to other metals.
To gather relevant information from the period of time between 2004 and 2014, a literature search was conducted. In addition, information relevant to fill gaps in the ATSDR profile was also directly obtained from the International Tungsten Industry Association (ITIA), as well as from US agencies. As recommended by the Health Risk Assessment Guidance for Metals (HERAG) (CitationICMM 2007), to assess study reliability, relevance, and adequacy for inclusion/exclusion, and as endorsed by the Organisation for Economic Co-operation and Development (OECD) in its Manual for Investigation of HPV Chemicals (CitationOECD 2014) and the European Chemicals Agency's Registration, Evaluation, and Authorisation of Chemicals (REACH) program, the unpublished and peer-reviewed published research was evaluated according to the criteria established by CitationKlimisch et al. (1997). In addition, this review is organized according to the toxicity endpoints delineated in Chapter 4 of HERAG (CitationICMM 2007).
Methodology
Literature search strategy
PubMed® (http://www.ncbi.nlm.nih.gov/pubmed) literature searches were conducted on tungsten and tungstate for the publication period of January 1, 2004 through May 19, 2014. In addition, endpoint gaps were filled with unpublished studies (dated pre- and post-2005) that were sponsored by the tungsten industry, and were conducted following OECD guidelines and using good laboratory practice (GLP). These studies were performed for hazard assessment at independent contract research laboratories, with the objective of fulfilling global regulatory requirements. Several unpublished government-sponsored studies were also reviewed.
Assessment of reliability, relevance, and adequacy
A systematic approach to the evaluation of the quality of data and their use in hazard and risk assessment was described by CitationKlimisch et al. (1997). In general, the more the details on methodology, test procedures, and analytics are documented, the easier an evaluation of their Klimisch reliability should be. The amount of information presented in the peer-reviewed publication or in the report of the study sponsored by the government or tungsten industry, provided the basis for deciding the reliability of the reported data according to the four scientific reliability categories (K1–K4), outlined in .
Table 1. Categories for ranking the reliability of studies or data from the literature or reports, based on the approach described by CitationKlimisch et al. (1997).
Regulatory status
Occupational exposure guidelines
The American Conference of Governmental Industrial Hygienists (ACGIH), the CDC's National Institute of Occupational Safety and Health (NIOSH), and the US Department of Labor's Occupational Safety and Health Administration (OSHA), have established occupational exposure limits for tungsten and tungsten substances. OSHA's current permissible exposure levels (PEL) and ACGIH's 8-hour threshold limit value (TLV)-time-weighted average (TWA) are 1 and 5 mg/m3 for water-soluble and insoluble tungsten substances (as W), respectively (CitationOSHA 2014a, CitationOSHA 2014b). NIOSH's recommended exposure level (REL) 10-h TWA values for soluble and insoluble tungsten substances (as W) are 1 and 5 mg/m3 respectively, with a 15-min short-term exposure level (STEL) of 3 and 10 mg/m3, respectively (CitationCDC 2010a, Citation2010b). OSHA PELs are intended to protect against the accumulation of insoluble tungsten substances in the lung and the acute effects of soluble substances on the central nervous system (metabolic poison) (CitationOSHA 2014a, Citation2014b).
Drinking water standards
To date, the World Health Organization (WHO) has not issued any drinking water guidelines, whereas Russia has a limit of 0.05 mg/L for drinking water (CitationKoutsospyros et al. 2006), and in the USA, the Commonwealth of Massachusetts has established action levels of 15 mg/L (CitationASTWSWMO 2008). The ATSDR toxicological profile (CitationKeith et al. 2005, Citation2007) did not derive an oral minimum risk level for tungsten or tungsten substances, stating that adequate data were not available to derive this guidance.
Toxicological studies
2005 ATSDR toxicological profile for tungsten data gap findings
Toxicological profiles were prepared in accordance with guidelines developed by the ATSDR and EPA and provide information briefly characterizing the toxic and adverse health effects of a hazardous substance. The ATSDR profile is not intended to be an exhaustive document; however, more comprehensive sources of specialty information are referenced.
The 2005 ATSDR toxicological profile for tungsten (CitationKeith et al. 2005, Citation2007) indicated that relatively few reports were located regarding health effects in animals following acute-, intermediate-, or chronic-duration exposure to tungsten or tungsten substances. Several early Russian reports were located, mainly originating from a single group of investigators. The reports predominantly assessed acute lethality or reproductive or developmental effects following oral exposure to soluble tungsten substances. In addition, for some of the assessment endpoints (e.g. inhalation toxicity), the ATDSR included health effects that resulted mainly from occupational exposure to dusts that include particles of tungsten and other metals (particularly cobalt) at facilities in which hardmetal is produced.
The ATSDR's literature search comprised tungsten toxicity studies published between the years 1924 and 2004. Hence, our literature searches were conducted on tungsten and tungstate for the publication period from January 1, 2004 through May 19, 2014, with the objectives of updating and filling the knowledge gaps identified in the 2005 ATSDR toxicological profile, and not of repeating the review of the studies already considered by ATSDR. The tungsten toxicity information included in the ASTDR's profile that pre-dated our literature search is widely available for review, and therefore, will not be discussed herein. The ATSDR had recognized that the available information was not sufficient to establish minimal risk levels, and instead, identified several data gaps in the following areas:
– | Acute inhalation, oral and dermal toxicities; skin and eye irritation; dermal sensitization | ||||
– | Repeated subchronic and chronic oral and inhalation exposure toxicity; reproductive and developmental toxicity; neuro- and immuno-toxicities | ||||
– | Carcinogenicity and genotoxicity |
Several of these data gaps have subsequently been filled with recently published studies. Other gaps can be filled using the results of unpublished studies. All of these studies are discussed in the sections below.
Literature search results
Search strings, PubMed MeSH query translations, and returned results for tungsten and tungstate for the publication period from January 1, 2004 through May 19, 2014, are shown in . Several searches at the chemical and endpoint levels were conducted to gather a complete set of toxicity endpoints for sparingly water-soluble substances (represented by tungsten) and water-soluble substances (represented by tungstate). The various tungsten substances, their CAS RegistrySM numbers, and solubility of the substances in water are listed in .
Table 2. Search strings, PubMed® MeSH query translations, and returned results for tungsten and tungstate publications from January 1, 2004 to May 19, 2014.
Table 3. Tungsten substance nomenclature and solubility in water.
Although a large number of studies were found from our PubMed search, only 95 peer-reviewed studies have been included herein, as they were considered relevant and reliable, with or without restriction (Klimisch reliability category K2 or K1, respectively, as outlined in ).
A standard toxicity testing summary for water-soluble and sparingly water-soluble tungsten substances is presented in . Some of the endpoints presented in were filled with unpublished studies (dated pre- and post-2005) that were sponsored by the tungsten industry or various government agencies. See the supplementary material for a summary of the details of the unpublished, industry- and government-sponsored studies that were reviewed, with hyperlinks to online reports (Supplementary Table 1 to be found online at http://informahealthcare.com/doi/abs/10.3109/10408444.2014.1003422), and the Klimisch reliability categories assigned to all 95 peer-reviewed and unpublished studies (Supplementary Table 2 to be found online at http://informahealthcare.com/doi/abs/10.3109/10408444.2014.1003422).
Table 4. Standard toxicity testing summary for water-soluble and sparingly water-soluble tungsten substances.
Toxicokinetics
The in vivo data show that in the case of oral exposure to water-soluble tungsten substances (e.g. ST), and inhalation exposure to sparingly soluble tungsten substances (e.g. WO3-x), the substances can be absorbed, systemically distributed, and excreted (via the urine). In vitro studies suggest that sparingly water-soluble tungsten substances such as W-metal, WC, and W2C are largely eliminated via the feces, following oral exposure. In vitro studies using artificial sweat fluid suggest that dermal exposure (or intact skin absorption) to water-soluble (ST, SMT, and APT) and sparingly soluble tungsten substances does not contribute appreciably to the amount present in humans.
It is not known whether the tungsten ion (tungstate; WO42−) is metabolized as such in the body; however, a recent in vitro study has shown that WO42− binds human albumin and other unknown protein (MW > 300 kDa) (CitationRodríguez-Fariñas et al. 2008). This finding was confirmed in rats, where approximately 80% of serum tungsten was bound to proteins, and most of the protein-bound tungsten was due to a complex with albumin, which is not highly stable. No binding to transferrin was detected. An unknown protein with a molecular weight > 100 kDa was also found to bind a small amount of tungsten (approximately 2%). The interaction between reduced glutathione (GSH) and WO42− was also evaluated; however, the formation of complexes was not observed (CitationGómez-Gómez et al. 2011).
After systemic distribution, tungsten (as WO42−) may be deposited in bone, by displacing phosphate (CitationWeber et al. 2008). CitationAng et al. (2013) investigated the uptake and speciation of tungsten in bones from adult and newborn mice exposed to WO42− at levels up to 200 mg/kg/d for 120 d via drinking water. The results suggested that exposure to tungsten results in increased bone mineralization and a resultant increase in bone strength. Whether this is due to the chemical speciation of tungsten or the alteration of cellular processes, needs to be further elucidated.
Our search identified four in vitro dissolution studies that estimated the bioavailability of tungsten substances in artificial human fluids. In addition, six new relevant in vivo studies on toxicokinetics of WO3-x (a sparingly water-soluble tungsten substance) and ST (a water-soluble substance), published after 2005, were identified.
In vitro
For metal substances, it is important to understand that simple exposure of an organism to the metal or a compound does not necessarily impart the biological properties of the metal ion. It is the bioavailability, defined as the degree of the metal ion (or a redox form of this ion) that enters systemic circulation, thereby accessing target sites, that determines the potential adverse effects. However, the distinction between bioavailability and solubility needs to be mentioned, as solubility is defined as the equilibrium distribution of a tungsten substance between water and the solute phase at a given temperature and pressure.
Several in vitro methods have been developed to determine metal dissolution in artificial fluids, as a surrogate model for the estimation of bioavailability. Four studies were investigated to elucidate the bioavailability of tungsten associated with ingestion, dermal (CitationStefaniak et al. 2010a, Citation2010b, CitationIITRI 2010b), or inhalation exposure (CitationStefaniak 2010, CitationIITRI 2010b). These data may be useful for understanding the inhalation, dermal, or oral dosimetry of tungsten substances.
Calcination of APT at a relatively low growth temperature (∼850°C) may result in the formation of WO3-x whiskers. CitationStefaniak (2010) determined that whiskers (6–9 μm in length) containing tungsten oxide powders (WO2.81, WO2.66, and WO2.51) dissolved more slowly than W-metal and WO3 powders in artificial airway epithelial lining fluid (SUF), and more slowly than W-metal in macrophage phagolysosomal simulant fluid (PSF). Overall, these results suggest that whisker-containing, less-oxidized tungsten oxide deposited in the alveolar region of the lung would have similar biopersistence to non-fiber-shaped, more-oxidized tungsten oxides (WO3 and WO3-x), but it would be more biopersistent than W-metal.
The dissolution of WC and W-metal in three types of gastric juice was assessed by CitationStefaniak et al. (2010b). Their results suggest that oral exposure to WC and W-metal do not contribute appreciably to total body burden, given the short residence time of the material in the stomach, and its relatively long dissolution half-life that ranges from 60 to 380 d. Likewise, CitationStefaniak et al. (2010a) measured the dissolution kinetics of W-metal and WC using artificial sweat. The dissolution of WC powder was independent of solvent pH (range tested 5.3–6.5); however, W-metal dissolution increased as the pH of the artificial sweat was increased from 5.3 to 5.9. Overall, the dissolution of W and WC was not influenced by solvent composition.
The findings of CitationStefaniak et al. (2010a, Citation2010b) were confirmed by an unpublished study (CitationIITRI 2010b) conducted under GLP guidelines. This industry-sponsored study used several artificial human fluids, such as gastric (pH = 2), alveolar (pH = 7.4), interstitial (pH = 7.4), lysosomal (pH = 4.5), and sweat (pH = 1.5), to test W-metal, WO3, WO2.91, WC, and W2C. Overall, the results showed that WO2.91 has a greater bioavailability in artificial lung fluids compared with the other tungsten substances tested. None of the tungsten substances were bioavailable in artificial gastric fluid and sweat (CitationIITRI 2010b).
Based on in vitro dissolution studies, a comparison of bioavailability after different exposure routes to tungsten substances can be estimated. Overall, the dissolution studies described in this section suggest that the sparingly water-soluble substances, WC, W2C, WO3, WO2.91, and W-metal, are not bioavailable via the oral or dermal routes compared with water-soluble substances (such as ST), and they potentially do not contribute appreciably to total body burden. Whereas, in lung fluids (e.g. alveolar, lysosomal, interstitial, SUF, or PSF), it seems that WO3 and WO2.91 are more systemically available by inhalation than are W-metal, WC, and W2C. For less bioavailable particles, toxicity may also depend on the lung's clearance, retention, and translocation to different compartments (CitationOberdorster et al. 1994).
In vivo
The ATSDR's toxicological profile (CitationKeith et al. 2005, Citation2007) did not identify data on the quantitative absorption or distribution of tungsten by the inhalation, oral, or dermal routes in humans, and our search did not identify any recent studies that could fill this gap. Instead, our search found seven new toxicokinetic studies conducted after 2005, using rats and mice (CitationMcDonald et al. 2007, CitationWeber et al. 2008, CitationRadcliffe et al. 2009, Citation2010, CitationGuandalini et al. 2011, CitationRajendran et al. 2012, CitationKelly et al. 2013). One study was on WO3-x (a sparingly water-soluble tungsten substance), and six studies were on ST (a water-soluble substance).
Inhalation exposure. Three rat inhalation toxicokinetic studies were published after 2005: two on ST (CitationRadcliffe et al. 2009, Citation2010), and one on WO3-x (CitationRajendran et al. 2012). The study designs and results are briefly described below.
CitationRadcliffe et al. (2009) exposed male rats, nose-only, to a 90-min single exposure of 0.256 mg 188W/L (as Na2188WO4; with a mass median equivalent aerodynamic diameter (MMAD) of 1.48–1.51 μm), equivalent to approximately 12 mg/kg/d (with one nostril occluded, to prevent deposition). After 0, 1, 3, 7, and 21 d post-exposure, the left and right sides of the nose, brain, olfactory pathway, and striatum, were sampled. Respiratory and olfactory epithelial samples from the side with the occluded nostril had significantly lower end-of-exposure 188W levels, confirming the occlusion procedure. The 188W levels of the olfactory bulb, olfactory tract/tubercle, striatum, cerebellum, and rest of brain, paralleled approximately 2–3% of measured blood 188W levels. Brain 188W concentrations were highest immediately following exposure, and returned to near-background concentrations within 3 d. The authors suggested that as 188W in the olfactory bulb remained low throughout the experiment (approximately 1–3% of the amount of tungsten seen in the olfactory epithelium), olfactory transport plays a minimal role in delivering tungsten to the brain in rats, without indicating the primary transport.
CitationRadcliffe et al. (2010) subjected male rats to a single exposure to tungsten for 90 min at 0.256 mg/L (as Na2188WO4; with a MMAD of 1.5 μm), which is equivalent to approximately 12 mg/kg/d. After 0, 1, 3, 7, and 21 d post-exposure, 188W tissue concentrations were assessed. The thyroid and urine had the highest 188W levels post-exposure, and urine was the primary route of 188W excretion. The pharmacokinetics of tungsten in most tissues followed a two-compartment pharmacokinetic model, with initial half-lives (t½) of approximately 4–6 h, and a longer terminal phase, with a t½ of approximately 6–67 d. The kidney, adrenal glands, spleen, femur, lymph nodes, and brain continued to accumulate small amounts of tungsten, as reflected by the tissue/blood activity ratios that increased throughout the 21-day period. At day-21, all tissues, except the thyroid, urine, lung, femur, and spleen, had only trace levels of 188W.
CitationRajendran et al. (2012) exposed rats to WO2.91 at 0.08, 0.325, and 0.65 mg/L of air, with MMAD ranging from 2.63 to 2.87 μm (estimated to be 15, 62, and 124 mg/kg/d, respectively) for 6 h/d for 28 consecutive days, followed by a 14-day recovery period. Inhaled WO2.91 was absorbed systemically, and blood levels of tungsten increased as inhaled concentration increased. Among the tissues analyzed for tungsten levels, the lung, femur, and kidney showed increased levels, with lung tissue having at least an order of magnitude greater than the tissues of the kidney or femur. By exposure day-14, tungsten concentration in tissues had reached steady-state.
The most recent inhalation studies on water-soluble and sparingly soluble tungsten forms confirm that both forms are readily absorbed and rapidly distributed to various organs (e.g. thyroid, spleen, lung, femur, liver), and the primary route of elimination is via urinary excretion (CitationRadcliffe et al. 2010, CitationRajendran et al. 2012). An important new finding from the ST studies is that olfactory transport plays a minimal role in delivering tungsten to the brain in rats (CitationRadcliffe et al. 2009).
Oral exposure. Reports of four relevant oral toxicokinetic studies were peer-reviewed and published after 2005; all of them were on ST. A summary of these studies is presented below.
CitationMcDonald et al. (2007) subjected rats and mice to a single exposure of ST at 1, 10, or 100 mg/kg by oral gavage, or by intravenous administration of 1 mg/kg. Plasma and tissue (liver, kidney, uterus, femur, and intestine) were collected at 1, 2, 4, or 24 h after exposure. The results showed tungsten in plasma and all tissues after both gavage and intravenous administration. Plasma concentrations peaked at 4 h in rats and 1 h in mice, with amounts decreasing significantly by 24 h.
CitationWeber et al. (2008) repeatedly exposed rats and mice to ST by gavage (10 mg/d) or drinking water (560 mg/L; estimated to be 85 mg/kg/d for rats and 148 mg/kg/d for mice) for 14 consecutive days. Plasma and tissue (intestine, liver, kidney, femur, uterus, and fetus) were collected at 1, 2, 4, or 24 h after exposure. Tungsten was detectable in plasma and all tissues and fetuses of both rats and mice. Accumulation occurred in the intestine, kidney, and femur, with urine being the primary route of elimination.
CitationGuandalini et al. (2011) exposed mice to ST at 0, 62.5, 125, and 200 mg/kg/d for 28 d, and after 1 d, the tissues of the kidney, liver, colon, bone, brain, and spleen were collected. The results showed increasing tungsten levels in all organs as exposure dose increased, with the highest concentration found in the bones and the lowest concentration found in brain tissue. Gender differences were seen only in the spleen (higher tungsten levels were found in female mice).
CitationKelly et al. (2013) exposed mice to tungsten as ST in their drinking water, at 15, 200, or 1000 mg/L (oral doses estimated to be in the order of 4, 50, and 250 mg/kg/d), for up to 16 weeks. Tungsten concentration in bone was analyzed by inductively coupled plasma mass spectrometry. Exposure resulted in a rapid deposition in the bone following 1 week, and tungsten continued to accumulate thereafter, although at a decreased rate.
These latest oral studies on water-soluble forms of tungsten have shown that it is readily absorbed, rapidly distributed to various organs (e.g. intestine, kidney, and femur), and excreted via the urine. These findings confirm most of what had been published on tungsten tissue distribution and excretion, and they also show that the brain is relatively protected when tungsten exposure occurs via oral administration.
Overall, this new toxicokinetic information on inhaled or ingested water-soluble and sparingly soluble tungsten substances in rats and mice could be used to improve the existing Legget's Physiologically-Based Pharmacokinetic model, included in the ATSDR's toxicological profile (CitationKeith et al. 2005, Citation2007) regarding the biokinetics of tungsten. However, data for rats were assigned low weightage in the selection of most parameter values in the CitationLeggett (1997) model, because of known qualitative differences in membrane transport between rats and humans in the handling of molybdate (MoO42−; which is more easily reduced in biological systems), a chemical and physiological analog of WO42−. These species differences recognized for molybdenum may apply to tungsten as well, as suggested by the faster excretion of tungsten by rats than by other species studied, and the apparent species differences in the distribution of retained tungsten. However, some aspects of the toxicokinetics of WO42− or MoO42−, including long-term bone retention due to displacement of WO42− or MoO42− for phosphate in bone, are expected to be independent of species (CitationKeith et al. 2005, Citation2007).
Mechanistic toxicity
In vitro
The ATSDR toxicological profile did not locate information on well-designed mechanistic studies that might provide valuable information to elucidate toxicity and reduce tungsten body burden (CitationKeith et al. 2005, Citation2007). Our search found several mechanistic in vitro and in vivo studies that attempted to elucidate how water-soluble forms of tungsten can treat diabetes, obesity, and hypertension, that deserve some consideration.
Mechanistic in vitro studies have shown that WO42− at the cellular level may disrupt the cell cycle (CitationLiu et al. 2012) by affecting Ca2+ homeostasis, cell signaling, bioenergetics (CitationJohnson et al. 2010, CitationColovic et al. 2011, CitationFraqueza et al. 2012a, Citation2012b, CitationFernández-Mariño et al. 2014), and/or glucose uptake. Studies on different cell lines (hepatocytes, myoblasts, adipocytes, and human embryonic kidney cells) have demonstrated that WO42− activates glucose uptake by an increase in the content and translocation of glucose transport. Other studies suggest that WO42− reduces blood pressure by vasodilatory action on Ca2+-potassium-dependent (BK) channels of arteries with β1 subunit (CitationFernández-Mariño et al. 2012). Based on the potential in vitro ability to affect glucose transport in cells and the arterial vasodilatory effects, WO42− has been proposed as an anti-diabetic and anti-hypertensive agent. Also, based on a study using hypothalamic N29/4 cells, WO42− has been shown to be a suitable candidate to manage obesity, as a leptin-mimetic compound, by activating (in an independent way) several kinases involved in the leptin signaling system (CitationAmigó-Correig et al. 2011).
One of the main mechanisms of action of metal toxicity is the production of reactive oxygen species (ROS). With this in mind, CitationStefaniak et al. (2010c) measured the generation of hydroxyl radicals (·OH) by different particle distributions of WC and W-metal powders in artificial lung and sweat fluids. In both artificial fluids, WC and W-metal powders promoted ROS production. The concentration of ROS increased as particle size decreased. However, the in vitro production of ROS by WC or W-metal in artificial sweat was not consistent with the observed low dermal acute toxicity, the lack of skin irritation in rabbits, and the lack of dermal sensitization (see Sections Dermal exposure, Skin and eye irritation caused by sparingly water-soluble substances, and Skin sensitization). For some metals, ROS production may play an important role in dermal sensitization, as demonstrated by CitationWang et al. (2010), who showed decreasing chromium hypersensitivity in guinea pigs with the administration of N-acetylcysteine. However, for other metals such as nickel, ROS production may not be part of the sensitization mechanism, as nickel did not reduce the ratio of the reduced:oxidized forms of cellular glutathione (i.e. GSH:GSSG) (CitationMizuashi et al. 2005), nor did it oxidize the fluorescent dye dichlorodihydrofluorescein diacetate (CitationMehrotra et al. 2005) when tested in vitro.
CitationLombaert et al. (2013) measured the oxidative response in human peripheral blood mononuclear cells after exposure to WC for 15 min. Their results (confirmed by reverse transcription polymerase chain reaction and Western blotting) showed that the oxidative-stress-response HMOX1 (the human gene that encodes for the enzyme heme oxygenase 1) was not expressed in samples treated with WC.
From these in vitro mechanistic studies, one may conclude the following: (1) WO42− activates glucose uptake and the leptin signaling system; (2) WO42− affects Ca2+ homeostasis, cell signaling, and bioenergetics; and (3) WC and W-metal induce the formation of ROS (without expressing HMOX1).
In vivo
Several in vivo mechanistic studies on orally administered ST have determined that tungsten can replace molybdenum as the catalytic metal in the active center of a diverse group of enzymes that, in general, catalyze oxygen atom transfer reactions (CitationBrondino et al. 2006). The most prominent molybdenum enzymes are nitrate reductase, sulfite oxidase (SO), xanthine dehydrogenase (XDH), aldehyde oxidase (AO), and the mitochondrial amidoxime reductase (CitationMendel and Kruse 2012).
Rats exposed via drinking water or gavage to several concentrations of ST ranging from 28 to 100 mg/kg/d for 14 d to 6 weeks, showed a reduction in enzymatic activities of molybdenum-dependent enzymes such as xanthine oxidase (XO), XDH, and SO, in the kidney, intestine, liver, plasma, and erythrocytes (CitationWeber et al. 2008, CitationHerken et al. 2009, CitationOzturk et al. 2010, CitationAydemir et al. 2012, CitationTutuncu et al. 2012). Mice liver AO activity was reduced by 45% when treated via drinking water for 14 d with ST at approximately 170 mg/kg/d (CitationSwenson and Casida 2013).
According to some researchers, if WO42− were to potentially replace MoO42− at the binding site of the molybdenum-cofactor enzyme family, rendering the enzymes non- or partially functional, it is biologically plausible that it could cause adverse effects (CitationTyrrell et al. 2013). This molybdenum-enzyme inactivation appears to be due to a decrease in the redox potential, caused by tungsten (CitationLiao 2013).
Several studies have used the tungsten inactivation of XO activity as proof of concept to confirm the toxicity mode of action mediated by ROS generation in the following events: renal apoptosis during pneumoperitoneum in rats (CitationKhoury and Weinbroum 2012); mononuclear phagocyte-mediated acute rat lung injury (CitationWright et al. 2004); acute renal allograft rejection in rats (CitationSun et al. 2004); post-ischemic coronary endothelial injury mediated by endothelin-1-induced superoxide generation in guinea pig hearts (CitationDuda et al. 2007); prevention of atherosclerosis in knockout mice (CitationSchröder et al. 2006); liver necrosis and fulminant hepatic failure protection in rats (CitationPawa and Ali 2004, CitationUskokovic-Markovic et al. 2007); para-phenylenediamine-induced skin lesions in rats (CitationLee et al. 2008), methotrexate-induced small intestinal toxicity in rats (CitationMiyazono et al. 2004); and joint swelling in rats (CitationKlocke et al. 2005). Several other studies inactivated SO with tungsten to elucidate sulfite toxicity in rats (CitationKucukatay et al. 2006, Citation2007, CitationNacitarhan et al. 2008, CitationNiknahad and O’Brien 2008, CitationHerken et al. 2009, CitationKocamaz et al. 2012).
Contrary to the in vitro studies reviewed above, Ca2+ homeostasis was not affected in healthy rats exposed to WO42− via gavage at 100 mg/kg/d for 6 weeks, as contractibility and intracellular calcium were not altered, nor were potassium and L-type Ca2+ currents of isolated ventricular myocytes (CitationAydemir et al. 2012).
The in vivo generation of ROS by water-soluble tungsten substances was not confirmed by lipid peroxidation assessed using the thiobarbituric acid reactive substances (TBARS) assay in heart tissue from rats exposed to WO42− via gavage at 100 mg/kg/d for 6 weeks (CitationAydemir et al. 2012). However, when male rats were exposed to ST orally at 119 or 238 mg/kg/d or intraperitoneally at 20 or 41 mg/kg/d for 2 weeks, an increase in ROS and erythrocyte antioxidant enzyme activities (catalase and glutathione peroxidase) were observed. In addition, erythrocyte glutathione-S-transferase activity showed significant inhibition, while tissue ROS and TBARS levels increased, accompanied by a decrease in GSH and in the GSH/GSSG ratio. This study suggests oxidative stress as a mechanism involved in the toxic manifestations of WO42− (CitationSachdeva et al. 2013).
In a follow-up study, rats orally exposed to WO42− at 10 mg/kg/d for 3 months presented inhibited activity of blood δ-aminolevulinic acid dehydratase, decreased levels of reduced GSH in liver and blood, and increased GSSG and TBARS levels in tissues. Antioxidant supplementation post-exposure, as α-lipoic acid or n-acetylcysteine, resulted in increased GSH levels, and was beneficial to the recovery of altered superoxide dismutase and catalase activity. In addition, it significantly reduced blood and tissue ROS and TBARS levels. These results confirm that WO42− induces oxidative stress in rats (CitationSachdeva and Flora 2014).
An in vivo mechanistic study on rats exposed via intraperitoneal injection to ST at 10 mg/kg showed that WO42− crosses the blood-brain barrier and independently increases the activity of several kinases involved in the leptin signaling system, suggesting that WO42− could be used to treat obesity (CitationAmigó-Correig et al. 2011). In addition, oral administration of WO42− (40–270 mg/kg/d) for 30 d significantly decreased body weight gain and adiposity without modifying caloric intake, intestinal fat absorption, or growth rate in obese rats. These effects were mediated by an increase in whole-body energy dissipation, and by changes in the expression of the genes involved in the oxidation of fatty acids and mitochondrial uncoupling in adipose tissue (CitationClaret et al. 2005).
Acute toxicity
The ATSDR identified a lack of information concerning adverse effects and targets of toxicity following acute-duration oral, dermal, or inhalation exposure to tungsten or tungsten substances (CitationKeith et al. 2005, Citation2007). Our literature search did not find any relevant studies. However, the tungsten industry sponsored several acute toxicity studies using different exposure routes. These K1 studies were conducted under GLP and followed OECD Testing Guidelines (TG). The respective acute toxicity values are presented in .
Table 5. Acute oral, dermal, and inhalation studies on tungsten substances.
Oral exposure
As expected, bioavailability dictated the acute oral toxicity of water-soluble tungsten substances such as ST, AMT, APT and SMT, as they are more toxic than sparingly water-soluble substances (e.g. W-metal, WC, WO3, or WO3-x). The low bioavailability found in artificial gastric fluids (Section In vitro under Toxicokinetics) was consistent with the low acute oral toxicity found in sparingly water-soluble tungsten substances.
Gross necropsy observations in animals that died after oral exposure to SMT at 2800–5000 mg/kg body weight and to AMT at 1373–2000 mg/kg body weight, revealed congestive changes such as dark red small intestine, dark red fluid in the stomach, red foci in the stomach, dilated urinary bladder, enlarged cervical lymph node, enlarged and red mesenteric, mandibular and bronchial lymph nodes, pale liver and kidney, and clear fluid in the pleural cavity (CitationRCC NOTOX BV 1991c, CitationHuntingdon Life Sciences Ltd., 1999h, CitationIITRI 2010a).
From the oral LD50 values (adjusted by the percent tungsten composition) shown in , it cannot be concluded that polytungstates are more toxic than monotungstates, as ST is slightly less toxic than AMT, but more toxic than APT and SMT. The overall rat acute toxicity of tungsten and its substances administered orally is low, based on an LD50 value ≥ 1000 mg/kg.
Dermal exposure
Water-soluble and sparingly soluble tungsten substances are not systemically bioavailable via the dermal route, as supported by an LD50 value > 2000 mg/kg body weight in rats (). Local skin effects ranged from none to slight dermal irritation (erythema) that resolved by day-4 post-exposure. No macroscopic abnormalities were observed at study termination. This low acute toxicity of sparingly water-soluble tungsten substances agrees with the low bioavailability seen in the results of the artificial sweat fluid study (Section In vitro under Toxicokinetics).
Inhalation exposure
Based on the rats’ LC50 values > 5 mg/L, tungsten substances are not acutely toxic if inhaled (). Some acute inhalation study results showed that the mean body weight gain of male test rats were lower than that of the controls following exposure, and macroscopic pathology at day-14 showed slight lung congestion (CitationHuntingdon Life Sciences Ltd., 1999f, Citation1999r). Bioavailability studies of sparingly water-soluble tungsten substances in artificial lung fluids predicted that WO3 and WO2.91 could be more bioavailable than W-metal and WC; however this did not translate to lower LC50 values.
Irritation and sensitization
Limited information was cited by the ATSDR concerning previous local (point of contact) toxicity observations, referencing only rabbit studies using 5% tungsten hexachloride (a water-soluble tungsten substance) that resulted in contact dermatitis and ocular irritation, as hydrolyses in an aqueous solution yielding hydrogen chloride (CitationKeith et al. 2005, Citation2007). Our literature search did not find any relevant studies. However, the tungsten industry sponsored several reliable (K1) rabbit skin and eye irritation studies conducted under GLP and following OECD guidelines. The following is a discussion on local irritation toxicity based on degree of tungsten solubility in water (soluble and sparingly soluble).
Skin and eye irritation caused by water-soluble substances
Based on rabbit skin irritation studies conducted according to OECD TG 404 (CitationOECD 2012a), SMT, APT, and ST were found to be non-irritant, as they caused no-irritation or only slight erythema that was reversible within 24 h (CitationRCC NOTOX BV 1991e, CitationHuntingdon Life Sciences Ltd., 1999e, Citation1999j).
OECD TG 405 (CitationOECD 2012b) studies on APT and ST showed minimal irritation that was fully reversible within 21 d, in the conjunctiva, cornea, or iris of rabbits (CitationHuntingdon Life Sciences Ltd., 1999d, Citation1999i). However, SMT is an eye irritant, as it causes adverse effects in the cornea, iris, and the conjunctiva of rabbits, which in some animals, did not reverse 21 d after instillation (CitationRCC NOTOX BV 1991a).
Skin and eye irritation caused by sparingly water-soluble substances
Rabbit skin irritation studies conducted according to OECD 404 (CitationOECD 2012a) deemed WC, W-metal, and WO3 to be non-irritating (CitationHuntingdon Life Sciences Ltd., 1999p, Citation2000b, CitationARC Seibersdorf Research GmbH 2002a).
Also, sparingly water-soluble tungsten substances were found to be non-irritating, based on rabbit eye irritation studies of WC, W-metal, WO3, and WO3-x, conducted according to OECD TG 405 (CitationOECD 2012b), causing only transient very slight to well-defined conjunctival irritation, or eliciting no irritation at all (CitationHuntingdon Life Sciences Ltd., 1999o, Citation2000a, CitationARC Seibersdorf Research GmbH 2002b, Citation2003a).
Having discussed the results of non-irritation in standard in vivo eye and skin irritation tests, one needs to recognize that, in general, metal powders may cause mechanical eye or skin irritation.
Skin sensitization
Metal hypersensitivity is a common immune disorder, as metal ions such as Ni2+, Co2+, Cu2+, and Cr3+ are haptens with a high immunogenic potential (CitationBüdinger and Hertl 2000). Human immune systems mount allergic attacks on metal ions through skin contact, lung inhalation, and metal-containing artificial body implants.
To assess the immunogenic potential of WO42−, the tungsten industry sponsored several studies of tungsten substances in guinea pigs. The studies followed OECD TG 406 (CitationOECD 1992). Water-soluble (CitationRCC NOTOX BV 1991d, CitationHuntingdon Life Sciences Ltd., 1998, Citation1999k) and sparingly soluble (CitationHuntingdon Life Sciences Ltd., 1999q, Citation1999u) tungsten substances elicited no positive sensitization reactions.
Repeated-dose toxicity
The ATSDR noted that the available repeated-exposure animal studies did not include critical dose-response information and other details (including methods used in statistical analysis of data), which precluded their usefulness for the purpose of risk assessment (CitationKeith et al. 2005, Citation2007).
In oral repeated-exposure studies, ST has become the more studied tungsten substance because of its water solubility, and hence likely higher bioavailability, compared with other tungsten substances. Some of the ST repeated-exposure studies identified are pre-clinical investigations on the treatment of diabetes or obesity with WO42−. To distinctly elucidate the potential adverse effects of tungsten in these pre-clinical diabetes studies, the healthy WO42− -treated group deserves more consideration than the diabetic WO42− - treated group.
To standardize the exposure dose as mg/kg/d in repeated-exposure studies that reported only the concentration given in the drinking water (in mg/L), the CitationUS EPA (1988) default value recommendations of body weight and water consumption per day, in conjunction with specific study details, were employed.
A common adverse effect observed in oral repeated- exposure studies in male and female rats (CitationBallester et al. 2007, CitationMcCain et al. 2010) or in male mice (CitationKelly et al. 2013) that were exposed to WO42− was a decrease in body weight. It was suggested that this could be mediated by the activation of the hypothalamic leptin pathway (CitationAmigó-Correig et al. 2011, 2012).
Reductions in body weight have been observed when dosing ST via drinking water at 2 mg/L (equivalent to 160 mg/kg/d) for a period of 12 weeks, or to 10–250 mg/kg/d via gavage for a period of 13 weeks. This body weight reduction was also seen in mice exposed to ST at approximately 130 mg/kg/d for 2 weeks (CitationAmigó-Correig et al. 2012).
Statistically significant body weight reduction findings that had been reported in preclinical diabetic studies were also seen by CitationMcCain et al. (2010), who exposed male and female rats to ST for 13 weeks. Significant treatment-group differences in mean body weight compared with untreated animals were observed in males (but not in females) between days 70 and 90, from the 10, 75, and 200 mg/kg/d exposure groups. In females, glucose levels in the 75 mg/kg/d exposure group were significantly higher than those of controls. No other significant dose-related adverse alterations were observed for hematological and clinical chemistry parameters for any treatment group.
A 13-week study using rats and mice was conducted by NTP, in which the animals were exposed to ST at estimated doses of 0–200 mg/kg/d and 0–500 mg/kg/d, respectively. Preliminary results showed significant body weight reductions in male and female rats in all exposed groups after the 13-week treatment, with no mortality. However, decreases in the body weight of male and female mice were only significant at the 500 mg/kg/d dose (CitationNTP 2014a).
Several preclinical studies have tested WO42− as an anti-diabetic or anti-obesity agent. Healthy male and/or female rats (as a healthy control group) were orally exposed for 2–12 weeks, to concentrations ranging from 100 to 190 mg/kg/d, or male mice were orally exposed for 2 weeks to 130 mg/kg/d. The exposure did not alter the biochemical parameters of the parotid salivary gland (CitationLeite and Nicolau 2009), systemic blood pressure, or levels of serum/blood glucose and insulin, triglycerides, high density lipoprotein (HDL) cholesterol, alanine aminotransferase (ALT) activity, and/or glycogen synthase total activity (CitationBallester et al. 2007, CitationPeredo et al. 2010, CitationNocito et al. 2012, CitationAmigó-Correig et al. 2012, CitationAydemir et al. 2012). In mice, WO42− treatment modified several groups of proteins in the hypothalamic nuclei, such as cell morphology, axonal growth, tissue remodeling, proteins related to energy metabolism, and synaptogenesis (CitationAmigó-Correig et al. 2012).
When treating mice with WO42− via drinking water at 5, 50 or 250 mg/kg/d, CitationKelly et al. (2013) did not observe liver toxicity as assessed by the levels of peripheral blood aspartate aminotransferase (AST) and ALT enzymes, but significantly lower body weight was reported in mice given the highest dose. WO42− did not alter erythrocyte and platelet counts or hemoglobin and hematocrit levels. However, peripheral blood white blood cell counts decreased in a dose-dependent manner following 1 week of exposure, but the decrease was only significant at 12 weeks of exposure at the highest dose tested.
In the most-recent diabetes-related study, CitationOliveira et al. (2014) treated wild type or insulin-receptor substrate deficient mice for 3 weeks with an ad libitum solution of 2000 mg/L of ST (equivalent to approximately 500 mg/kg/d) in distilled water. WO42− treatment of wild-type healthy mice had no effect on body weight, pancreas (assessed by β-cells and α-cells per mass or per pancreatic area, and β-cell survival), glucose homeostasis (evaluated by blood glucose levels and intraperitoneal glucose tolerance test), peripheral insulin sensitivity, or hepatic glucose metabolism (measured by hepatic glucogen and total or intrinsic glycogen synthase activities; active-phosphorylated glycogen phosphorylase and total glycogen phosphorylase activities). However, it seems that WO42− slightly increased the hepatic phosphoenolpyruvate carboxykinase levels compared with the untreated control group.
Histopathological examination of the kidneys in both sexes of rats by CitationMcCain et al. (2010) revealed WO42− treatment-related effects at 125 and 200 mg/kg/d dose groups. Kidney changes ranged from mild to severe regeneration of cortical tubules showing basophilic tubular profile and thickened basement membrane, diagnosed as progressive nephropathy. Based on these adverse kidney effects, the lowest observable adverse effect level (LOAEL) in male and female rats was 125 mg/kg/d. The no observable adverse effect level (NOAEL) in male and female rats was 75 mg/kg/d.
CitationSachdeva et al. (2013) also noted indicators of nephrotoxicity when exposing rats to ST orally at 119 mg or 238 mg/kg/d or intraperitoneally at 20 mg and 41 mg/kg/d for 2 weeks. An increase in the activities of plasma transaminases, creatinine, and urea levels were observed, suggesting hepatic and renal injury. These indicators of adverse effects were more prominent in rats undergoing intraperitoneal administration than those that were subjected to oral administration.
As most metals are excreted through renal clearance and by gastrointestinal excretion, the findings of CitationMcCain et al. (2010) were not unexpected. Within these nephrotoxic effects, WO42− (in addition to MoO42−, selenate, thiosulfate, succinate, and citrate) may also specifically affect sulfate homeostasis by inhibiting the Na+-sulfate co-transporter NaS1 (SLC13A1), which mediates the sulfate (re)absorption across the renal proximal tubule and small intestinal epithelia (CitationMarkovich 2014).
It is noteworthy that none of the preclinical studies that tested WO42− as an anti-diabetic/obesity agent (CitationBallester et al. 2007, CitationPeredo et al. 2010, CitationNocito et al. 2012, CitationAydemir et al. 2012, CitationAmigó-Correig et al. 2012) showed any adverse histopathological findings in the kidneys, despite having exposed the animals for a period of 12 weeks (CitationBallester et al. 2007) to similar concentrations (i.e. 100–190 mg/kg/d or higher, in a study by CitationOliveira et al. (2014), who exposed mice to 500 mg/kg/d for 3 weeks) as those in the study by CitationMcCain et al. (2010).
Overall, repeated oral exposure of rats to WO42− may result in body weight reductions and nephrotoxicity, these effects being seen with doses between 125–250 mg/kg/d. Within this dose range, it was suggested by CitationSachdeva et al. (2013) that oxidative stress is the mechanism involved in the toxic manifestations of WO42−. At WO42− doses in the range of 100–190 mg/kg/d, neither systemic blood pressure nor levels of serum/blood glucose and insulin, triglycerides, HDL, cholesterol, AST, and ALT enzyme levels or activity, and/or glycogen synthase total activity, were affected. Furthermore, no adverse effects on pancreas, glucose homeostasis, peripheral insulin sensitivity, or hepatic glucose metabolism were seen in mice exposed to WO42− at an estimated dose of 500 mg/kg/d for 3 weeks. Surprisingly however, this high dose did not cause any body weight reduction or nephrotoxic effects, perhaps because the exposure duration was shorter (10 weeks) compared with the NTP's 13-week studies (CitationNTP 2014a).
The ATSDR identified a gap in hazard assessment with regard to repeated-dose toxicity via the inhalation route, and described, instead, several intratracheal studies instilling W-metal and WC (CitationKeith et al. 2005, Citation2007). In addition, the NTP has planned intratracheal studies on W-metal, WO3, and WO3-x (CitationNTP 2014b, Citation2014c, Citation2014d). However, instillation is not a physiological route for human exposure. Intratracheal instillation involves invasive delivery that bypasses the upper respiratory tract. Instillation is usually done at a dose and/or dose rate substantially greater than that which would occur during inhalation. Several investigators have shown discrepancies between inhalation and instillation routes of exposure, including instilled vehicle effects on particle distribution and physicochemical nature of the test material, as well as differences in clearance and pattern of injury. Of additional concern is the potential confounding effect of anesthesia, which could influence the initial effect of the instilled material on the lung surface, as well as the retention and clearance of test material. These concerns have tempered the adoption of intratracheal instillation as an acceptable alternative to inhalation (CitationOsier and Oberdörster 1997, CitationDriscoll 2000).
Our literature search identified one study using the inhalation route. Female and male rats were exposed to WO2.91 aerosol at a target air concentration of 0.08, 0.325, and 0.65 mg/L of air for 6 h/d (equivalent to 15, 62 and 124 mg/kg/d) for 28 d. Increased lung weight was noted for both terminal and recovering animals, which was attributed to deposition of WO2.91 in the lungs, inducing a macrophage influx (e.g. pigmented macrophages, alveolar, and foamy macrophages). No toxicologically significant effects were reported, and therefore the NOAEL was > 0.65 mg/L of air (CitationRajendran et al. 2012).
The results of more recent repeated-exposure studies by the oral and inhalation routes may serve as a basis for calculating oral and inhalation reference doses for tungsten and tungsten substances. As dermal bioavailability is limited, as discussed in previous sections, it does not appear that repeated-exposure studies associated with dermal exposure to tungsten or tungsten substances are necessary.
Genotoxicity
The ATSDR toxicological profile indicated that the genotoxic potential of tungsten and tungsten substances has not been extensively assessed, and it cited several positive non-standard genotoxicity methods (e.g. bacterial bioluminescence, lambda prophage, gene conversion and reverse mutation in yeast, and increased recombinant frequency), and a positive result for WO42− in the HPRT forward mutation method (CitationKeith et al. 2005, Citation2007).
The tungsten industry sponsored several K1 mutagenicity studies conducted under GLP, following OECD testing guidelines (). Bacterial mutagenicity tests in Salmonella typhimurium and Escherichia coli using TG 471 (CitationOECD 1997a) on water-soluble (CitationCCR 1989b, CitationCovance Laboratories, Inc., 2004e, CitationNTP 2014a) and sparingly soluble (CitationHuntingdon Life Sciences Ltd., 2001a, CitationARC Seibersdorf Research GmbH 2002e, Citation2003c, CitationCovance Laboratories, Inc., 2004f, CitationNTP 2014b) tungsten substances were negative. However, according to the International Council of Mining & Metals, bacterial genotoxicity assays, in general, lack sensitivity due to either the probable mechanism of action or lack of metal uptake (CitationICMM 2007).
Table 6. Genotoxicity studies on tungsten substances.
Tests that detect forward gene mutations, such as OECD TG 476 using mouse lymphoma (CitationOECD 1997d), chromosomal aberrations (TG 473; CitationOECD 1997b), or cytogenetic damage using the micronucleus test (TG 474; CitationOECD 1997c) in mammalian cells, would be preferable for metal substances (CitationICMM 2007). Mouse lymphoma assays were negative for two tungsten substances (ST and W-metal) which bracket the water solubility extremes (CitationCovance Laboratories, Inc., 2004c, 2004d). Chromosomal aberrations were negative in water-soluble (ST) and sparingly soluble (WO3, WC, and W-metal) tungsten substances (CitationHuntingdon Life Sciences Ltd., 2001b, CitationCovance Laboratories, Inc., 2003, 2004a, Citation2008, CitationIITRI 2010c). In vivo rodent micronucleus studies on two tungsten substances with the highest water solubility (SMT and ST) were also negative in bone marrow cells (CitationCCR 1989a, CitationCovance Laboratories, Inc., 2004b, CitationNTP 2014a).
CitationAnard et al. (1997) and CitationVan Goethem et al. (1997) first reported the Comet assay, to detect DNA damage (strand breaks) in human cells exposed to sparingly water-soluble tungsten substances. Human peripheral lymphocytes in vitro exposed to pure WC for 15 min did not have any more DNA breaks than those of controls at doses up to 250 μg/mL (CitationAnard et al. 1997, CitationVan Goethem et al. 1997). These negative Comet assay results were confirmed by CitationDe Boeck et al. (2003) using similar exposure conditions.
In a recent Comet assay study, primary murine bone marrow cell cultures were treated with vehicle control or varying concentrations of ST (5–500 μg/mL) for 6 h. In all cells tested, WO42− induced a significant increase in the Comet tail moment, indicating an increase in DNA breakage, and confirmed with Western blotting experiments using antibodies against γH2AX, a phosphorylated histone variant that is induced upon DNA damage (CitationGuilbert et al. 2011).
As noted, the more recent Comet studies exposed cells for a longer period of time (6 h) than older studies (15 min), and this may be the reason for the conflicting results, as an exposure period of 3–6 h is recommended. In addition, neither of the Comet assays exposed cells in the presence and absence of the metabolic activation system (S9-mix) (CitationBurlinson 2012).
To determine whether in vivo exposure to tungsten might induce DNA damage within the bone marrow compartment, CitationGuilbert et al. (2011) exposed mice to ST in the drinking water (15 or 200 mg/mL, estimated to be 4 and 50 mg/kg/d, respectively) for 8 weeks. While WO42− did not significantly alter the total bone marrow cellularity at these concentrations, it was found that WO42− exposure resulted in an increase in DNA damage, as assessed by the Comet assay.
Overall, standard genotoxicity testing yielded negative results on forward mutations as well as on assays of chromosomal aberrations, and also in the in vivo micronucleus tests, which were all done in mammalian cell systems. These negative results support a lack of genotoxic potential of water-soluble and sparingly soluble tungsten substances. However, there are equivocal in vitro Comet assay results that were conducted on different cells (human peripheral lymphocytes versus mouse bone marrow cells), and equivocal in vivo micronucleus (CitationCCR 1989a, CitationCovance Laboratories, Inc., 2004b, CitationNTP 2014a) and Comet assay (CitationGuilbert et al. 2011) studies in bone marrow cells of mice that were orally exposed to water-soluble tungsten substances. Therefore, due to the equivocal in vivo results, the genotoxicity of soluble substances in bone marrow cells merits further investigation before the genotoxic potential of soluble tungsten forms can be established. CitationNTP (2014a) has recognized the need for a confirmatory study, and has completed Comet assays (cell type has not been identified) in rats and mice, but the results are pending.
Carcinogenicity
Information regarding the carcinogenicity of ingested tungsten in humans is restricted to a single report from CDC, in which no statistically significant association (odds ratio 0.78, p-value 0.57) was found between exposure to tungsten in the drinking water and cases of leukemia observed in a population of Churchill County, Nevada (CitationCDC 2003, CitationRubin et al. 2007).
ATSDR information (CitationKeith et al. 2005, Citation2007) concerning a carcinogenicity study of oral exposure to tungsten includes a study design in which 5 ppm of tungsten as ST (approximately 0.5 mg/kg/d) were given to rats in drinking water for a lifetime. In the study, control rats and mice exhibited similar growth patterns and incidences of gross tumors (CitationSchroeder and Mitchener 1975). However, the ATSDR considered that the study assessed growth, gross tumor incidence, and longevity, without evaluating either dose-response data or comprehensive histopathological examinations, which precluded the results from being used in risk assessment.
An ongoing 2-year carcinogenicity study of ST using rats and mice is currently being conducted by the CitationNTP (2014a). Based on a 13-week range-finding study (CitationNTP 2014a) (which exposed rats and mice via drinking water to 0–2000 mg/L; the preliminary results of this study are discussed in Section Repeated-dose toxicity), the drinking water doses that were selected for rats ranged from 0 to 1000 mg/L (estimated exposure doses of 25, 50, and 100 mg/kg/d), and the same for mice ranged from 0 to 2000 mg/L (estimated exposure doses of 100, 250, and 500 mg/kg/d). The results of this chronic carcinogenicity study are not expected until late 2015.
To date, no carcinogenicity studies on individual tungsten substances are available. Two studies on implanted tungsten-pellets in rodents could assist in the assessment for this endpoint. In the first study, CitationKalinich et al. (2005) reported on tumor development in rats implanted intramuscularly with pellets comprising 91.1% tungsten, 6% nickel, and 2.9% cobalt (W/Ni/Co) or with 100% nickel (positive control) pellets, but not with 100% tantalum (negative control) pellets. However, as described below, a follow-up study in mice conducted by the same investigator concludes that not all the tungsten-based alloys are carcinogenic, and that the adverse effects are only specifically seen with the (W/Ni/Co) alloy.
In the follow-up 2-year study, CitationKalinich (2011) implanted pellets in mice focusing on two tungsten alloys: W/Ni/Co, with the same composition as the alloy used the first study, and an alloy composed of 91% tungsten, 7% nickel, and 2% iron (W/Ni/Fe). The rest of the study design included several treatment groups consisting of negative and positive controls (tantalum and nickel, respectively), 11 alloy combinations of tungsten (W), nickel (Ni), cobalt (Co), iron (Fe), and/or tantalum (Ta), and a toxicity reference metal (lead). The pellets were cylindrical, and measured 1 mm in diameter and 2 mm in length. The protocol for the follow-up mouse studies included serial collection of tissues, at 1, 3, 6, and 12 months post-implantation, aimed at identifying early changes relevant to the development of carcinogenic endpoints. In addition, serum and urine samples were assessed for metal content.
As in the previous study using rats, the Wi/Ni/Co alloy induced tumor formation at the implant site. However, no other tungsten alloy exhibited tumor formation or indications of pellet-induced neoplasia. Tungsten levels were detected in serum and urine of animals implanted with Wi/Ni/Co, W/Ta, W/Ni/Ta, W/Co/Ta and W/Fe/Ta pellets (CitationKalinich 2011). Although these studies did not focus on individual water-soluble tungsten substances per se, serum and urine analysis for tungsten, conducted as part of the implant study, showed that the tungsten in the implanted pellets became bioavailable in vivo without causing muscle tumor development.
In a separate study, CitationSchuster et al. (2012) confirmed that the tumorigenic potential of the W/Ni/Co pellets is related to the mobilization of nickel and cobalt bioavailability, by conducting electron microscopy of pellets which had been embedded for 6 months in rats and were extracted from them. Progressive galvanic corrosion of the matrix phase of the W/Ni/Co pellet was observed, and was accompanied by high urinary concentrations of tungsten, nickel, and cobalt. The galvanic corrosion takes place because of the difference in electrode potential between the matrix phase (anode) and the tungsten phase (cathode).
Overall, evidence from these embedded pellet studies would indicate that bioavailable tungsten, in and of itself, may not be carcinogenic.
Reproductive and developmental/neurotoxicity
The ATSDR review did not identify any reproductive or developmental reports related to exposure to tungsten substances in humans, stating that “only limited animal studies were identified, and deficiencies in study details regarding exposure and quantitative results render the results of these studies of limited value for purposes of quantitative risk assessment” (CitationKeith et al. 2005, Citation2007).
Four published reproductive/developmental studies of ST were found. In the first study, male rats were orally exposed for 3 months to ST with a solution of 2 mg/mL (estimated dose of 190 mg/kg/d) in 0.9% sodium chloride. All rats survived at the end of the study; however, they showed a much lower body weight gain than the untreated controls. The ST-exposed rats did not show hypoglycemia or any alteration in Leydig cell function, as shown by the absence of change in serum testosterone levels (CitationBallester et al. 2005).
In a related study, female rats were exposed to WO42− at an estimated dose of 160 mg/kg/d for 12 weeks. Markers for reproductive function (progesterone, follicle-stimulating hormone, and luteinizing hormone) and the uterine expression of the progesterone and estrogen receptors were not affected by WO42− (CitationBallester et al. 2007).
A one-generation study that exposed male and female rats to 5 or 125 mg/kg/d of ST for 70 d (pre- and post-natal exposure) confirmed no effect on reproductive functions (mating success) or physical development of offspring. Daily administration of ST produced no evidence of toxicity; however, in the dams, the average gestation duration was longer for the group dosed with 125 mg/kg/d than for the control and 5 mg/kg/d groups. There were no differences in the average number of pups born (CitationMcInturf et al. 2008, Citation2011).
Overall, the most-recent reproductive toxicity evidence confirms that WO42− does not affect the reproductive functions or physical development of offspring.
Neurobehavioral assessment of pups born and nursed from WO42−-exposed rats found elevation of distress vocalizations in the 125 mg/kg/d group, and the righting reflex showed unexpected sex-dependent differences (but not dose-dependent), where male pups demonstrated faster righting than females (CitationMcInturf et al. 2008, Citation2011). When looking closer at these results, it can be noted that the statistical difference observed in the righting reflex between male and female pups was due to a decrease in male righting reflex with increasing dose (but not statistically significant), combined with a numerical increase in the righting reflex among females (CitationJackson et al. 2013).
The pups’ locomotor activity was affected in both groups of dams that were treated with either 5 or 125 mg/kg/d, without affecting maternal retrieval, and no apparent effects of treatment on F1 acoustic startle response or water maze navigation (CitationMcInturf et al. 2008, Citation2011) were seen.
CitationMcInturf et al. (2008, Citation2011) only used two neurobehavioral tests, and these measured only very early reflexive behavioral responses. In addition, no histopathological effects were noted that indicated effects in the brain. Based on the results, one could conclude that ST may produce subtle neurobehavioral effects in offspring related to motor activity and emotionality; however, the collection of results is insufficient to delineate a clear dose-response in either the pups or dams and deserves further investigation.
Immunotoxicity
No information was located by the ATSDR concerning tungsten-induced immunotoxicity following inhalation, oral, or dermal exposure to tungsten or tungsten substances (CitationKeith et al. 2005, Citation2007).
After 2005, two in vitro and three in vivo peer-reviewed studies attempted to elucidate the immunotoxic potential of ST. In addition, NTP issued a report in 2012 on a series of immunotoxicity studies conducted as part of the tungsten testing program (CitationNTP 2012). The following is a discussion of these recent immunotoxicity studies.
An in vitro study of isolated human peripheral blood lymphocytes showed an increase in early apoptosis and reduction in the number of cells entering into the cell cycle, when the cells were treated with 0.01, 0.1, 1.0, and 10 mM ST (equivalent to 0.006, 0.063, 0.626, and 6.26 mM as tungsten). The selected concentrations were substantially higher than tungsten levels (16.2 to 39.7 nM) found in tap water from Churchill County, Nevada (CitationCDC 2003, CitationRubin et al. 2007). Apoptosis and cell cycle effects were not as pronounced at lower treatment levels (CitationOsterburg et al. 2010).
Bone marrow-derived pre-B cell lines (BU-11 cells) and BMS2 stromal cells were treated in vitro for 48 h with ST concentrations varying from 50, 100, 250, and 500 μg/ml (equivalent to 31.3, 62.6, 156.4, and 312.9 μg of tungsten/mL, respectively). These in vitro concentrations were significantly higher than tungsten levels (0.003 to 0.007 μg/mL) from tap water in Churchill County, Nevada (CitationCDC 2003, CitationRubin et al. 2007). BU 11-cells exhibited a dose- and time-dependent apoptosis. As compared with BU-11 cells, BMS2 cells required higher concentrations of tungsten in order to inhibit cell growth. This suggests that the developing B-cells are more sensitive to the toxic effects of tungsten than the cells in the surrounding bone marrow microenvironment; however WO42− induced different cell cycle changes in the stromal cells as compared with the BU-11 cells. These in vitro data suggest that the developing hematopoietic compartment may be sensitive to WO42−-induced changes (CitationGuilbert et al. 2011).
To determine if WO42− can cause an unnatural immune response, mice were exposed in utero by parental inhalation or ingestion of an estimated dose of 1 and 4 mg/kg/d, respectively; and inoculated with respiratory syncytial virus (within 2 weeks of weaning). Compared with controls, WO42−-treated animals presented significant spleen enlargement (splenomegaly); however, the nature of the hematological/immunological disease was inconclusive (CitationFastje et al. 2012).
CitationOsterburg et al. (2014) tested if exposure to WO42− can result in immune suppression. For this, parental male and female mice were orally exposed to 2, 62.5, 125, and 200 mg/kg/d for 28 d in a one-generation model, and intraperitoneally injected with Staphylococcal enterotoxin. Exposure to WO42− at the highest dose resulted in a decrease of activated cytotoxic T-cells and helper T-cells. In delayed-type hypersensitivity Type IV experiments, exposure to 200 mg/kg/d of WO42− prior to primary and secondary antigen challenge significantly reduced footpad swelling. According to the authors, these results suggest that WO42− could cause an immune suppression that may reduce host defense against pathogens.
CitationKelly et al. (2013) exposed mice for 16 weeks to WO42− via drinking water. Mice receiving 15, 200, or 1000 mg/L (oral doses estimated to be in the order of 50 and 250 mg/kg/d) had a significantly greater percentage of cells in the late pro-B/large pre-B developmental stages. An increase in DNA damage in both whole marrow and isolated B cells at the lower tungsten concentration (15 mg/L), but not at the highest concentration (1000 mg/L), was also noted.
The CitationNTP (2012) conducted a 28-day study to establish the potential effects on the immune system of female mice receiving ST via drinking water, at concentrations of 125, 250, 500, 1000, and 2000 mg/L (estimated doses ranged from 30 to 500 mg/kg/d). Over the 28-day exposure period, WO42− did not alter body weight, body weight gain, or the weights of the major organs of the immune system, the thymus and spleen. Total splenocyte number and both absolute values and percent values of spleen cell phenotypes were unaffected by WO42− exposure (CitationNTP 2012). No effects were observed on T-dependent antibody responses, as evaluated using the antibody-forming cell response, the sheep red blood cell enzyme-linked immunosorbent assay (ELISA), and the keyhole limpet hemocyanin ELISA, suggesting that humoral (antibody) immunity is not adversely affected by WO42− exposure (CitationNTP 2012).
The mixed leukocyte responses and the cytotoxic T-lymphocyte responses presented some significant differences, but they were not dose-responsive. Furthermore, no effects were observed on the in vivo delayed-type hypersensitivity response to Candida albicans or in the anti-CD3-mediated proliferation assay, suggesting that WO42− does not affect cell-mediated immunity (CitationNTP 2012).
Lastly, innate immunity was not affected by WO42− exposure, as indicated by a lack of effect on both natural killer-cell activity and the functional activity of the mononuclear phagocytic system. With the exception of effects on bone marrow differentials at the 2000 mg/L dose level, WO42− did not adversely alter innate, humoral, or cell-mediated immunities in mice (CitationNTP 2012).
The latest NTP immunotoxicity evidence conflicts with the evidence obtained by CitationOsterburg et al. (2014) and CitationFastje et al. (2012) with regard to potential effects of WO42− on innate, humoral, or cell-mediated immunities. It is difficult to pinpoint the cause(s) of the discrepancy, as WO42− doses and exposure time were similar in both studies, and it seems that the only difference was the mice strain used in the studies: CitationOsterburg et al. (2014) and CitationFastje et al. (2012) used C57BL/6 mice, whereas the NTP studies were conducted using B6C3F1/N mice. A report from the International Program on Chemical Safety indicates that a lack of consistency of chemical-induced immunotoxicity in specific assays across species or strains does not necessarily represent conflicting data, and may represent species or strain differences (CitationWHO 2012). The adverse effects on bone marrow differentials observed by CitationKelly et al. (2013) were also observed in NTP's immunotoxicity studies. Overall, the immunotoxicity potential of WO42− needs to be investigated further.
Nano-tungsten toxicity
The ATSDR toxicological profile did not cover the toxicity of tungsten nanoparticles (CitationKeith et al. 2005, Citation2007). However, because nano-tungsten (with a mean particle size < 100 nm) applications are being explored in various fields (e.g. catalysts, coatings, pigments, lubricants, plastics, nano-wires/fibers, textiles, films, semi-conductors, or electrodes), it was deemed appropriate to discuss the toxicity of nano-sized tungsten.
Tungsten nanoparticles have a high surface area (which can lower the sintering temperatures), low vapor pressure, unusual quantum confinement, and grain boundary effects. However, the high surface area of tungsten nanoparticles could produce a different toxic effect than that of larger (e.g. micron-sized/bulk) tungsten particles.
Several in in vitro studies have been published in the last 9 years on the toxicity of W-metal, WC, WS2, or WO3 nanoparticles. The cytotoxicity/cell viability of nano-tungsten substances has been evaluated by assessing fluorescence indicators of cell metabolic activity and membrane integrity (CitationBastian et al. 2009, CitationKühnel et al. 2012), mitochondrial function (MTT/MTS) (CitationHussain et al. 2005, CitationLanone et al. 2009, CitationMachado et al. 2011, CitationPardo et al. 2014), mitochondrial membrane potential (CitationHussain et al. 2005), and neutral red uptake (CitationLanone et al. 2009, CitationKühnel et al. 2012), or by measuring membrane leakage of lactate dehydrogenase (CitationHussain et al. 2005).
Several reports of attempts to elucidate the mode of action of nano-tungsten substances have been published, such as the recording of human keratinocyte transcription profiles by microarray analysis (CitationBusch et al. 2010), the assessment of cellular antioxidant activity by measuring the quenching of the dye dichlorodihydrofluorescein diacetate, by quantifying GSH (CitationHussain et al. 2005), or antioxidant response activation (measured by the antioxidant-response element using a luciferase reporter), or by the induction Nrf2-mediated Phase II detoxification genes (CitationPardo et al. 2014).
The genotoxic effects of nano-WO3 were assessed in bacteria using the reverse mutation assay in S. typhimurium (CitationHasegawa et al. 2012), or by looking at the direct interaction of WO3 nanoplates with naked DNA plasmid, and then transferred into E. coli cells for mutation observation (CitationThongkumkoon et al. 2014). In mammalian systems, the genotoxic potential of WO3 was evaluated by the transformation assay in BALB/c cells (CitationHasegawa et al. 2012). Nano-WC genotoxicity was assessed as a potential cause of ROS generation by micronuclei formation in HepG2 cells (CitationKühnel et al. 2012).
It is recognized that metal nanoparticles (e.g. silver, copper, and aluminum) can easily cross the blood-brain barrier, affecting neuronal function and possibly leading to neurotoxicity and neuropathology (CitationSharma et al. 2009). With this in mind, in vitro studies were conducted on rat neuronal and glial cells (CitationBastian et al. 2009) and on the hippocampal neurons of rats (CitationShan et al. 2012, Citation2013), to assess the neurotoxic potential of nano-sized WC.
A summary of the results of these studies for each nano-tungsten substance tested is presented in . Overall, from the available evidence, nano-sized W-metal, WC, and WS2 were shown to be chemically inert with regard to cell viability, but not for oxidative stress in the case of WC and WS2. However, equivocal WO3 mutagenic results warrant further investigation, and the neurotoxicity effects by WC in in vivo models need to be confirmed.
Table 7. Studies on nano-tungsten substances.
Human studies
Clinical studies
A 6-week study was identified as a proof-of-concept trial on the efficacy of ST in human obesity (CitationHanzu et al. 2010). A prospective, randomized, placebo-controlled, and double-blind clinical trial was conducted with 30 obese non-diabetic subjects, who were randomized to receive 100 mg of ST twice per day, or placebo. The primary study endpoint was the absolute change in body weight relative to the time of randomization.
Treatment with ST was not associated with a significant weight loss compared with placebo (p = 0.854). Likewise, treatment with ST was not associated with significant changes in fat mass, resting energy expenditure, or caloric consumption. The data do not support ST as a pharmacological agent in the treatment of human obesity (CitationHanzu et al. 2010).
Tungsten levels in humans
Most background environmental exposures to tungsten are from the soluble forms such as WO42− that may occur in drinking water. Several studies have reported tungsten levels in blood, red blood cells, and serum or urine, in a variety of human subpopulations.
A 2-year program for human biomonitoring measured tungsten and 18 other metals in the blood and serum of subjects living in two different regions of Italy. The serum tungsten level showed an arithmetic mean of 0.15 ± 0.004 μg/L, in the inhabitants of the region of Umbria (n = 290), and was slightly higher than that of inhabitants of the region of Calabria (0.12 ± 0.003 μg/L; n = 218) (CitationBocca et al. 2010).
Urinary tungsten in 21 athletes was reported to be 82 ± 53 μg/g-creatinine whereas levels in 26 sedentary subjects were below the limit of detection (CitationLlerena et al. 2012). Another investigation examined the possible relationship between metals in children and severity of autism. In a comparison between 54 case subjects and 44 controls (age and gender matched), the authors concluded that the autism group had higher urinary levels of lead, thallium, tin, and tungsten than the neurotypical control group. The mean urinary tungsten levels in the autism and control groups were 0.334 ± 0.23 and 0.232 ± 0.40 μg/g-creatinine, respectively (CitationAdams et al. 2013).
Canada's 2009–2011 cycle human biomonitoring program reports urinary tungsten 95th percentile geometric mean from participants aged 3 to 79 years: 0.63 μg/L (0.69 μg/g of creatinine) with 95% confidence interval (CI) of 0.50–0.76 μg/L (0.56–0.81 μg/g of creatinine), based on a representative sample of 6290 individuals (CitationMinistry of Health Canada 2013). Urinary levels in females (n = 3262; 0.82 μg/g of creatinine; 95th CI: 0.57–1.1 μg/g of creatinine) were higher than in males (n = 3028; 0.58 μg/g of creatinine; 95th CI: 0.49–0.66 μg/g of creatinine).
Data from the National Health and Nutrition Examination Survey (NHANES) program (2009–2010), revealed the geometric mean urinary concentration in the US population to be 0.081 μg/L (0.086 μg/g of creatinine) with 95% CI 0.075–0.087 μg/L (0.080–0.093 μg/g of creatinine), based on a representative sample of 2847 individuals (CitationCDC 2013). Over the 12 years (1999–2010) that the survey has been conducted, the geometric mean urinary concentrations ranged from 0.071 to 0.99 μg/L (0.070–0.103 μg/g of creatinine). Males, and the group comprising those less than 20 years of age, consistently show higher mean tungsten urinary concentrations than females. The groups < 20 years old, in general, show higher tungsten levels than the group ≥ 20 years or older. Over the 12-year survey, urine geometric mean range concentrations of the Mexican-Americans (0.079–0.106 μg/g of creatinine) tended to be higher than those of Non-Hispanic blacks (0.066–0.088 μg/g of creatinine) and Non-Hispanic whites (0.069–0.104 μg/g of creatinine) (CitationCDC 2013).
The latest analysis of the NHANES data (CitationJain 2013) showed that the levels of urinary tungsten (together with cobalt and molybdenum) have increased over the 2003–2010 period. Smokers’ adjusted geometric mean urinary concentration (0.10 μg/L; 95% CI 0.091–0.111) displayed significantly higher levels (together with lead, antimony, and uranium) than that of non-smokers (0.08 μg/L; 95% CI 0.076–0.084). Tobacco plants can uptake heavy metals such as zinc, copper, nickel, cadmium, iron, and manganese from the soil in which they grow, and tend to accumulate the metals in their leaves. When cigarettes are smoked, metals contained in the tobacco leaves can be transferred to main- and side-stream smoke and inhaled by smokers or non-smokers via environmental tobacco smoke (CitationGolia et al. 2009). Pregnancy (0.099 μg/L; 95% CI 0.087–0.111) was found to be associated with higher levels of tungsten (jointly with barium, cesium, cobalt, molybdenum, lead, and mercury) compared with nongravid females (0.082 μg/L; 95% CI 0.078–0.086).
Several researchers have conducted statistical analyses on the NHANES data in an attempt to identify possible associations between measured parameters and health status. For example, urinary tungsten (n = 712; adjusted odds ratio of 2.30, 95% CI 1.72–3.07, p < 0.001) concentrations were associated with an increased incidence of vision disorder (CitationShiue 2013). Urinary cadmium and tungsten (adjusted odds ratio of 2.25 95% CI: 0.97–5.24; geometric mean 0.07 μg/L; n = 751) were reported to be associated with peripheral arterial disease (CitationNavas-Acien et al. 2005), and a significant association was observed between cardiovascular disease (CVD) and cerebrovascular disease and urinary tungsten (adjusted odds ratio of 1.78), antimony, cadmium, and cobalt (CitationAgarwal et al. 2011). In an examination of the relationship between heavy metals and various medical conditions, tungsten was found to be related to asthma (odds ratio of 1.72; 95% CI: 1.15–2.59), but not to CVDs (CitationMendy et al. 2012). In a study of associations of metals with total and central obesity indices based on NHANES data (n = 3816), no significant associations between tungsten with waist circumference and body mass index were found (CitationPadilla et al. 2010). CitationYorita Christensen (2013) reported an association between urinary levels of tungsten with increased levels of thyroid-stimulating hormone.
One evaluation of the NHANES data focused specifically on tungsten. A cohort of 8614 adults with 193 reported stroke diagnoses and 428 reported diagnoses of CVD was assessed using crude and adjusted logistic regression models (CitationTyrrell et al. 2013). The authors concluded that elevated weighted urinary tungsten concentrations were associated (odds ratio of 1.66; 95% CI 1.17–2.34) with an increased prevalence of stroke, independent of typical risk factors even in people below 50 years of age (odds ratio of 2.17; 95% CI 1.33–3.53); but logistic regression models suggest that tungsten is not involved in the etiology of CVD.
Reported reference dose and concentration estimations
CitationSchell and Pardus (2009) derived an oral reference dose (RfD) using the benchmark dose (BMDL10) approach, defined as the dose at the 95% lower confidence limit of a 10% response. The US EPA's Benchmark Dose Software (BMDS, Version 1.4.1) was used to model the ST data from both the 90-day oral toxicity study of CitationMcCain et al. (2010) and the rat reproductive study of CitationMcInturf et al. (2008).
The lowest (most precautionary) BMDL10 from the renal toxicity endpoint was 102 mg/kg/d; the lowest BMDL10 from the development endpoint was 93 mg/kg/d. Using the lowest of the BMDLs to estimate the tungsten RfD in the calculations, and a factor of 1000 to account for inherent uncertainty, the oral RfD for ST is 0.093 mg/kg/d, which is equivalent to approximately 0.06 mg of tungsten/kg/d or 0.08 mg WO42−/kg/d (CitationSchell and Pardus 2009).
Following the EPA's standard equations, CitationSchell and Pardus (2009) developed a protective drinking water risk-based concentration for tungsten of 2.28 mg/L.
Using the studies done by CitationMcCain et al. (2010) and CitationRajendran et al. (2012), CitationJackson et al. (2013) calculated the values of derived no effect levels (DNELs) for occupational long-term inhalation, according to the REACH program's guidance on characterization of dose-response for human health. The inhalation DNELlong-term for ST, from which values for all other soluble tungsten substance DNELs were derived, is 3 mg/m3 (1.7 mg tungsten/m3), and the inhalation DNELlong-term for WO2.91 is 7.3 mg/m3 (5.8 mg tungsten/m3). Although derived using different methodologies and supported by different studies, the occupational inhalation DNELlong-term values for water-soluble and sparingly soluble tungsten substances are similar to the current NIOSH-recommended exposure level and the ACGIH 8-h TLV TWA of 1 mg tungsten/m3 for soluble tungsten substances and 5 mg tungsten/m3 as metal and insoluble tungsten substances (CitationCDC 2010a, Citation2010b, CitationOSHA 2014a, Citation2014b).
Conclusions
This review gathered new toxicokinetic information, as well information on acute and repeated-exposure studies with a variety of endpoints, including reproductive toxicity, developmental toxicity, neurotoxicity, and immunotoxicity. The recent available evidence shows that tungsten and tungsten substances are not bioavailable via the dermal route. WO42− and WO3-x can be systemically distributed and excreted via urine after oral and inhalation exposure, respectively; but sparingly water-soluble tungsten forms have a limited in vitro bioavailability, suggesting excretion via feces after oral exposure.
Tungsten and tungsten substances are not acute toxicants, eye and skin irritants, or dermal sensitizers. The low toxicity seen in in vivo studies is consistent with the low bioavailability shown in in vitro dissolution studies. W-metal, WC, W2C, WO3, and WO2.91 are not bioavailable via the oral or dermal route, compared with soluble substances (ST, AMT, SMT, and APT), and potentially do not contribute appreciably to total body burden (e.g. mechanism of tungsten storage). As dermal bioavailability is limited, it does not appear that repeated-exposure studies associated with dermal exposure to tungsten or tungsten substances are necessary.
Standard testing supports a lack of genotoxicity of tungsten and tungsten substances; however, the in vivo Comet assay from one study reported positive results within the bone marrow compartment that contradicts the negative results from other in vivo studies of micronucleus in bone marrow cells. The contradiction may possibly arise because the studies assessed genotoxicity on different bone marrow cells, but merit further investigation as toxicokinetic studies via the inhalation or oral routes indicate that after systemic distribution, tungsten is deposited in bone by displacing phosphate. The CitationNTP (2014a) has recognized the need for a confirmatory study and has completed Comet assays (cell type has not been identified) in rats and mice, but the results are pending.
After exposure, soluble tungsten forms are readily absorbed, rapidly distributed to different organs (e.g. thyroid, spleen, lung, femur, liver), and excreted via the urine as the primary route of elimination, causing nephrotoxicity at estimated exposure doses greater than 125 mg/kg/d. As most metals are excreted through renal clearance and gastrointestinal excretion, these findings were not unexpected. In vivo (CitationSachdeva et al. 2013) and in vitro (CitationStefaniak et al. 2010c) studies suggest that the toxic mode of action of water-soluble and sparingly soluble tungsten substances is by producing ROS, as demonstrated by an inhibition of erythrocyte glutathione S-transferase activity, and increases in catalase and glutathione peroxidase activities. These enzymatic changes are accompanied by lipid peroxidation, or by an increase in the generation of hydroxyl radicals, together with a decrease of the GSH/GSSG ratio. Possibly, this oxidative stress response is produced without expressing the HMOX1 gene (CitationLombaert et al. 2013).
Alternatively to a ROS mechanism of action, toxicity could also occur if WO42− replaces MoO4 − 2 at the binding site of the molybdenum-cofactor enzyme family, rendering them non- or partially functional.
The healthy, treated group in diabetic and weight reduction rodent study designs deserves consideration when elucidating the potential effects of water-soluble tungsten forms. In addition to the nephrotoxic effects described above, repeated exposure to WO42− resulted in decreased body weight (possibly mediated by the activation of the hypothalamic leptin pathway), but altered neither the systemic blood pressure nor the levels of serum/blood glucose and insulin, triglycerides, HDL cholesterol, ALT activity, and/or glycogen synthase total activity. Conversely, no adverse effects on pancreas, glucose homeostasis, peripheral insulin sensitivity, or hepatic glucose metabolism were observed.
There is no relevant information on the carcinogenic potential of tungsten and tungsten substances, and unfortunately, the results of the 2-year NTP study on ST with rodents are not expected until late 2015 (CitationNTP 2014a). As no carcinogenic studies are available on individual tungsten substances, chronic studies on tungsten pellets (alloyed with other metals) implanted in rodents were considered to evaluate this endpoint, and the evidence suggests that water-soluble and sparingly soluble tungsten substances may not be carcinogenic.
The most recent reproductive toxicity evidence confirms that WO42− does not have any apparent effect on the reproductive function or the physical development of offspring; however the collection of neurobehavioral results on subtle neurobehavioral effects in offspring related to motor activity and emotionality are insufficient to delineate a clear dose-response in either the pups or dams, and thus deserves further investigation.
There is some contradiction regarding the effects of WO42− on innate, humoral, or cell-mediated immunity results reported by CitationOsterburg et al. (2014), CitationFastje et al. (2012), and CitationNTP (2012). However, there are reports of bone marrow cell changes after WO42− exposure (CitationNTP 2012, CitationKelly et al. 2013) that merit follow-up, as this is in line with evidence relating to the deposition of tungsten in bone.
With regard to nanoparticles, in vitro studies with nano-sized W-metal and WC have shown them to be chemically inert with regard to cell viability and oxidative stress. However, WO3 equivocal mutagenic results warrant further investigation, and the neurotoxic effects of WC in in vivo models need to be confirmed.
Several studies have statistically analyzed the urinary levels of tungsten from specific cohorts or the NHANES survey. Studies have reported potential relationships of tungsten (together with other metals such as antimony, cadmium, and cobalt) with various medical conditions such as autism, asthma, vision disorder, peripheral arterial disease, CVD, cerebrovascular disease, and stroke. Future studies are warranted to clearly understand the biological mechanism along the pathway before drawing firm conclusions on the causation of a specific medical condition due to tungsten.
In 2009, when new evidence of oral toxicity of WO42− emerged, CitationSchell and Pardus (2009) attempted to estimate a tungsten reference dose equivalent to approximately 0.06 mg of tungsten/kg/d or 0.08 mg WO42−/kg/d. However, a significant number of studies have been subsequently published on water-soluble and sparingly soluble tungsten substances that filled major toxicity endpoint gaps identified by the ATSDR in the 2005 toxicological profile for tungsten (CitationKeith et al. 2005, Citation2007). Such new evidence includes several recent repeated-exposure studies that need to be considered when regulators estimate and propose a tungsten reference or concentration dose.
Supplementary material available online
itxc_a_1003422_sm3055.pdf
Download PDF (354.8 KB)Acknowledgements
We are grateful to Dr. Philip Mitchell, Emeritus Reader in Chemistry, University of Reading, UK, for his objective review of the manuscript. Our sincere appreciation is due to Dr. Burghard Zeiler, Secretary-General of International Tungsten Industry Association, for drafting the tungsten flow chart, and we acknowledge the editorial assistance of Eileen Y. Ivasauskas of Accuwrit Inc.
Declaration of interest
Details of the authors’ affiliation are as shown on the title page. Mr. Carmen Venezia, Manager, Safety and Environment for Global Tungsten and Powders Corp., for more than twenty years. Dr. Ranulfo Lemus was an ITIA consultant for six years, being appointed as ITIA's Health, Safety and Environment Director in February 2013. The authors have sole responsibility for the writing and content of the paper.
References
- Adams JB, Audhya T, McDonough-Means S, Rubin RA, Quig D, Geis E, et al. (2013). Toxicological status of children with autism vs. neurotypical children and the association with autism severity. Biol Trace Elem Res, 151, 171–80.
- Agarwal S, Zaman T, Tuzcu EM, Kapadia SR. (2011). Heavy metals and cardiovascular disease: results from the National Health and Nutrition Examination Survey (NHANES) 1999–2006. Angiology, 62, 422–29.
- American Elements. (2014). Tungsten blue oxide (TBO). [Online] Available at: http://www.americanelements.com/blue-tungsten-oxide.html. Accessed on 10 November 2014.
- Amigó-Correig M, Barceló-Batllori S, Piquer S, Soty M, Pujadas G, Gasa R, et al. (2011). Sodium tungstate regulates food intake and body weight through activation of the hypothalamic leptin pathway. Diabetes Obes Metab, 13, 235–42.
- Amigó-Correig M, Barceló-Batllori S, Soria G, Krezymon A, Benani A, Pénicaud L, et al. (2012). Anti-obesity sodium tungstate treatment triggers axonal and glial plasticity in hypothalamic feeding centers. PLoS One, 7, e39087.
- Anard D, Kirsch-Volders M, Elhajouji A, Belpaeme K, Lison D. (1997). In vitro genotoxic effects of hard metal particles assessed by alkaline single cell gel and elution assays. Carcinogenesis, 18, 177–84.
- Ang CY, Johnson DR, Seiter JM, Allison PG, Osterburg AR, Babcock GF, Kennedy AJ. (2013). Effects of tungsten chemical species on biochemical pathways and mineralization in bone. [Online] Available at:http://www.toxicology.org/AI/PUB/Tox/2013Tox.pdf (Abstract 1519). Accessed on 23 May 2014.
- ARC Seibersdorf Research GmbH. (2002a). Tungsten oxide (WO3): acute dermal irritation/corrosion study with rabbits. Report No. ARC—UL-0440. [Unpublished Report] Seibersdorf, Austria. Sponsor: HC Starck GmbH. Report date: 20 August 2002.
- ARC Seibersdorf Research GmbH. (2002b). Tungsten oxide (WO3): acute eye irritation/corrosion study with rabbits. Report No. ARC—UL-0441. [Unpublished Report] Seibersdorf, Austria. Sponsor: HC Starck GmbH. Report date: 20 August 2002.
- ARC Seibersdorf Research GmbH. (2002c). Tungsten oxide (WO3): acute inhalation toxicity study in rats. Report No. ARC—UL-0565. [Unpublished Report] Seibersdorf, Austria. Sponsor: HC Starck GmbH. Report date: 28 November 2002.
- ARC Seibersdorf Research GmbH. (2002d). Tungsten oxide (WO3): acute oral toxicity study with rats (acute toxic class method). Report No. ARC—UL-0439. [Unpublished Report] Seibersdorf, Austria. Sponsor: HC Starck GmbH. Report date: 20 August 2002.
- ARC Seibersdorf Research GmbH. (2002e). Tungsten oxide (WO3): Salmonella typhimurium reverse mutation test. Report No. ARC—UL-0518. [Unpublished Report] Seibersdorf, Austria. Sponsor: HC Starck GmbH. Report date: 18 October 2002.
- ARC Seibersdorf Research GmbH. (2003a). Tungsten oxide blue: acute eye irritation/corrosion study with rabbits. Report No. ARC—UL-0805. [Unpublished Report] Seibersdorf, Austria. Sponsor: HC Starck GmbH. Report date: 28 July 2003.
- ARC Seibersdorf Research GmbH. (2003b). Tungsten oxide blue: acute oral toxicity study with rats (acute toxic class method). Report No. ARC—UL-0804. [Unpublished Report] Seibersdorf, Austria. Sponsor: HC Starck GmbH. Report date: 28 July 2003.
- ARC Seibersdorf Research GmbH. (2003c). Tungsten oxide blue: Salmonella typhimurium reverse mutation test. Report No. ARC—UL-0807. [Unpublished Report] Seibersdorf, Austria. Sponsor: HC Starck GmbH. Report date: 29 July 2003.
- ASTWSWMO. (2008). Tungsten issues paper. [Online] Available at: http://www.astswmo.org/Files/Policies_and_Publications/Federal_Facilities/TUNGSTEN_FINAL_120208.pdf. Accessed on 23 May 2014.
- Aydemir M, Ozturk N, Dogan S, Aslan M, Olgar Y, Ozdemir S. (2012). Sodium tungstate administration ameliorated diabetes-induced electrical and contractile remodeling of rat heart without normalization of hyperglycemia. Biol Trace Elem Res, 148, 216–23.
- Ballester J, Muñoz MC, Domínguez J, Palomo MJ, Rivera M, Rigau T, et al. (2007). Tungstate administration improves the sexual and reproductive function in female rats with streptozotocin-induced diabetes. Hum Reprod, 22, 2128–35.
- Ballester J, Domínguez J, Muñoz MC, Sensat M, Rigau T, Guinovart JJ, Rodríguez-Gil JE. (2005). Tungstate treatment improves Leydig cell function in streptozotocin-diabetic rats. J Androl, 26, 706–15.
- Bastian S, Busch W, Kühnel D, Springer A, Meissner T, Holke R, et al. (2009). Toxicity of tungsten carbide and cobalt-doped tungsten carbide nanoparticles in mammalian cells in vitro. Environ Health Perspect, 117, 530–6.
- Bocca B, Mattei D, Pino A, Alimonti A. (2010). Italian network for human biomonitoring of metals: preliminary results from two Regions. Ann Ist Super Sanità, 46, 259–65.
- Brondino CD, Romão MJ, Moura I, Moura JJG. (2006). Molybdenum and tungsten enzymes: the xanthine oxidase family. Curr Opin Chem Biol, 10, 109–14.
- Budavari S. (1996). The Merck Index - An Encyclopedia of Chemicals, Drugs, and Biologicals. Whitehouse Station, New Jersey: Merck and Co., Inc., p. 1673.
- Büdinger L, Hertl M. (2000). Immunologic mechanisms in hypersensitivity reactions to metal ions: an overview. Allergy, 55, 108–15.
- Bureau of Reclamation. (2011). Investigation of molybdenum disulfide and tungsten disulfide as additives to coatings for foul release systems. [Online] Available at: www.usbr.gov/research/projects/download_product.cfm?id = 312. Accessed on 10 November 2014.
- Burlinson B. (2012). The in vitro and in vivo comet assays. Methods Mol Biol, 817, 143–63.
- Busch W, Kühnel D, Schirmer K, Scholz S. (2010). Tungsten carbide cobalt nanoparticles exert hypoxia-like effects on the gene expression level in human keratinocytes. BMC Genomics, 11, 65.
- CCR. (1989a). Micronucleus assay in bone marrow cells of the mouse with sodium metatungstate. Report No. 145124. [Unpublished Report] Cytotest Cell Research GmbH & Co. Darmstadt, F.R.G. Sponsor: HC Starck GmbH. Report date: 16 June 1989.
- CCR. (1989b). Salmonella typhimurium reverse mutation assay with sodium metatungstate. Report No. 145113. [Unpublished Report] Cytotest Cell Research GmbH & Co. Darmstadt, F.R.G. Sponsor: HC Starck GmbH. Report date: 22 March 1989.
- CDC. (2003). Cross-sectional exposure assessment of environmental exposures in Churchill County, Nevada. [Online] Available at: http://www.cdc.gov/nceh/clusters/Fallon/study.htm. Accessed on 23 May 2014.
- CDC. (2010a). NIOSH pocket guide to chemical hazards. Tungsten. [Online] Available at: http://www.cdc.gov/niosh/npg/npgd0645.html. Accessed on 10 November 2014.
- CDC. (2010b). NIOSH pocket guide to chemical hazards. Tungsten (soluble compounds, as W). [Online] Available at: http://www.cdc.gov/niosh/npg/npgd0646.html. Accessed on 10 November 2014.
- CDC. (2013). Fourth national report on human exposure to environmental chemicals. [Online] Availableat: http://www.cdc.gov/exposurereport/. Accessed on 23 May 2014.
- Claret M, Corominola H, Canals I, Saura J, Barcelo-Batllori S, Guinovart JJ, Gomis R. (2005). Tungstate decreases weight gain and adiposity in obese rats through increased thermogenesis and lipid oxidation. Endocrinology, 146, 4362–69.
- Colovic MB, Bajuk B, Avramovic N, Holclajtner A, Bošnjakovic-Pavlovic N, Vasic V, Krstic D. (2011). Inhibition of rat synaptic membrane Na+/K+-ATPase and ecto-nucleoside triphosphate diphosphohydrolases by 12-tungstosilicic and 12-tungstophosphoric acid. Bioorg Med Chem, 19, 7063–69.
- Covance Laboratories, Inc. (2003). Chromosomal aberrations in Chinese hamster ovary (CHO) cells. Report No. 7273-116. [Unpublished Report] Vienna, VA, USA. Sponsor: U.S. Army Center for Health Promotion and Preventative Medicine. Report date: 22 December 2003.
- Covance Laboratories, Inc. (2004a). Chromosomal aberrations in Chinese hamster ovary (CHO) cells. Report No. 7273-122. [Unpublished Report] Vienna, VA, USA. Sponsor: U.S. Army Center for Health Promotion and Preventative Medicine. Report date: 04 August 2004.
- Covance Laboratories, Inc. (2004b). In vivo mouse micronucleus assay with sodium tungstate dihydrate. Report No. 7273-115. [Unpublished Report] Vienna, VA, USA. Sponsor: U.S. Army Center for Health Promotion and Preventative Medicine. Report date: 06 January 2004.
- Covance Laboratories, Inc. (2004c). L5178Y TK +/− Mouse lymphoma forward mutation assay with a confirmatory assay with sodium tungstate dihydrate. Report No. 7273-118. [Unpublished Report] Vienna, VA, USA. Sponsor: U.S. Army Center for Health Promotion and Preventative Medicine. Report date: 07 January 2004.
- Covance Laboratories, Inc. (2004d). L5178Y TK +/− mouse lymphoma forward mutation assay with a confirmatory assay with tungsten powder. Report No. 7273-119. [Unpublished Report] Vienna, VA, USA. Sponsor: U.S. Army Center for Health Promotion and Preventative Medicine. Report date: 26 July 2004.
- Covance Laboratories, Inc. (2004e). Salmonella-Escherichia coli/mammalian-microsome reverse mutation assay with a confirmatory assay with sodium tungstate dihydrate. Report No. 7273-117. [Unpublished Report] Vienna, VA, USA. Sponsor: U.S. Army Center for Health Promotion and Preventative Medicine. Report date: 02 January 2004.
- Covance Laboratories, Inc. (2004f). Salmonella-Escherichia coli/mammalian-microsome reverse mutation assay with a confirmatory assay with tungsten powder, APS 1–5 micron. Report No. 7273-120. [Unpublished Report] Vienna, VA, USA. Sponsor: U.S. Army Center for Health Promotion and Preventative Medicine. Report date: 22 July 2004.
- Covance Laboratories, Ltd. (2008). Induction of chromosome aberrations in cultured Chinese hamster lung (CHL) cells. Report No. 2811/1-D6172. [Unpublished Report] North Yorkshire, England. Sponsor: International Tungsten Industry Association. Report date: 23 January 2008.
- De Boeck M, Lombaert N, De Backer S, Finsy R, Lison D, Kirsch-Volders M. (2003). In vitro genotoxic effects of different combinations of cobalt and metallic carbide particles. Mutagenesis, 18, 177–86.
- Driscoll KE. (2000). Intratracheal instillation as an exposure technique for the evaluation of respiratory tract toxicity: uses and limitations. Toxicol Sci, 55, 24–35.
- Duda M, Konior A, Klemenska E, Beresewicz A. (2007). Preconditioning protects endothelium by preventing ET-1-induced activation of NADPH oxidase and xanthine oxidase in post-ischemic heart. J Mol Cell Cardiol, 42, 400–10.
- Fastje CD, Harper K, Terry C, Sheppard PR, Witten ML. (2012). Exposure to sodium tungstate and Respiratory Syncytial Virus results in hematological/immunological disease in C57BL/6J mice. Chem Biol Interact, 196, 89–95.
- Fernández-Mariño AI, Porras-González C, González-Rodríguez P, Selent J, Pastor M, Ureña J, et al. (2012). Tungstate activates BK channels in a β subunit- and Mg2+-dependent manner: relevance for arterial vasodilatation. Cardiovasc Res, 95, 29–38.
- Fernández-Mariño AI, Valverde MA, Fernández-Fernández JM. (2014). BK channel activation by tungstate requires the β1 subunit extracellular loop residues essential to modulate voltage sensor function and channel gating. Pflugers Arch, 466, 1365–75.
- Fraqueza G, Batista de Carvalho LAE, Marques MPM, Maia L, Ohlin CA, Casey WH, Aureliano M. (2012a). Decavanadate, decaniobate, tungstate and molybdate interactions with sarcoplasmic reticulum Ca2+-ATPase: quercetin prevents cysteine oxidation by vanadate but does not reverse ATPase inhibition. Dalton Trans, 41, 12749–58.
- Fraqueza G, Ohlin CA, Casey WH, Aureliano M. (2012b). Sarcoplasmic reticulum calcium ATPase interactions with decaniobate, decavanadate, vanadate, tungstate and molybdate. J Inorg Biochem, 107, 82–9.
- Golia EE, Dimirkou A, Mitsios IK. (2009). Heavy-metal concentration in tobacco leaves in relation to their available soil fractions. Comm Soil Sci Plant Anal, 40, 106–20.
- Gómez-Gómez MM, Rodríguez-Fariñas N, Cañas-Montalvo B, Domínguez J, Guinovart J, Cámara-Rica C. (2011). Biospeciation of tungsten in the serum of diabetic and healthy rats treated with the antidiabetic agent sodium tungstate. Talanta, 84, 1011–18.
- Guandalini GS, Zhang L, Fornero E, Centeno JA, Mokashi VP, Ortiz PA, et al. (2011). Tissue distribution of tungsten in mice following oral exposure to sodium tungstate. Chem Res Toxicol, 24, 488–93.
- Guilbert C, Kelly ADR, Petruccelli LA, Lemaire M, Mann KK. (2011). Exposure to tungsten induces DNA damage and apoptosis in developing B lymphocytes. Leukemia, 25, 1900–4.
- Hanzu F, Gomis R, Coves MJ, Viaplana J, Palomo M, Andreu A, et al. (2010). Proof-of-concept trial on the efficacy of sodium tungstate in human obesity. Diabetes Obes Metab, 12, 1013–18.
- Hasegawa G, Shimonaka M, Ishihara Y. (2012). Differential genotoxicity of chemical properties and particle size of rare metal and metal oxide nanoparticles. J Appl Toxicol, 32, 72–80.
- Herken EN, Kocamaz E, Erel O, Celik H, Kucukatay V. (2009). Effect of sulfite treatment on total antioxidant capacity, total oxidant status, lipid hydroperoxide, and total free sulfydryl groups contents in normal and sulfite oxidase-deficient rat plasma. Cell Biol Toxicol, 25, 355–62.
- Huntingdon Life Sciences, Ltd. (1998). Ammonium paratungstate skin sensitization to the Guinea-pig. Report No. ITU 030/983093/SS. [Unpublished Report] Cambridgeshire, England. Sponsor: International Tungsten Industry Association. Report date: 19 May 1998.
- Huntingdon Life Sciences, Ltd. (1999a). Ammonium paratungstate acute (four-hour) inhalation study in rats. Report No. ITU 031/983504. [Unpublished Report] Cambridgeshire, England. Sponsor: International Tungsten Industry Association. Report date: 28 May 1999.
- Huntingdon Life Sciences, Ltd. (1999b). Ammonium paratungstate acute dermal toxicity to the rat. Report No. ITU 027/982708/AC. [Unpublished Report] Cambridgeshire, England. Sponsor: International Tungsten Industry Association. Report date: 24 June 1999.
- Huntingdon Life Sciences, Ltd. (1999c). Ammonium paratungstate acute oral toxicity. Report No. ITU 026/982707/AC. [Unpublished Report] Cambridgeshire, England. Sponsor: International Tungsten Industry Association. Report date: 24 June 1999.
- Huntingdon Life Sciences, Ltd. (1999d). Ammonium paratungstate eye irritation to the rabbit. Report No. ITU 029/982807/SE. [Unpublished Report] Cambridgeshire, England. Sponsor: International Tungsten Industry Association. Report date: 08 September 1999.
- Huntingdon Life Sciences, Ltd. (1999e). Ammonium paratungstate skin irritation to the rabbit. Report No. ITU 028/982644/SE. [Unpublished Report] Cambridgeshire, England. Sponsor: International Tungsten Industry Association. Report date: 08 September 1999.
- Huntingdon Life Sciences, Ltd. (1999f). Sodium tungstate dihydrate acute (four hour) inhalation study in rats. Report No. ITU 007/983498. [Unpublished Report] Cambridgeshire, England. Sponsor: International Tungsten Industry Association. Report date: 28 May 1999.
- Huntingdon Life Sciences, Ltd. (1999g). Sodium tungstate dihydrate acute dermal toxicity to the rat. Report No. ITU 003/982923/AC. [Unpublished Report] Cambridgeshire, England. Sponsor: International Tungsten Industry Association. Report date: 24 June 1999.
- Huntingdon Life Sciences, Ltd. (1999h). Sodium tungstate dihydrate acute oral toxicity to the rat. Report No. ITU 002/983107/AC. [Unpublished Report] Cambridgeshire, England. Sponsor: International Tungsten Industry Association. Report date: 24 June 1999.
- Huntingdon Life Sciences, Ltd. (1999i). Sodium tungstate dihydrate eye irritation to the rabbit. Report No. ITU 005/982804/SE. [Unpublished Report] Cambridgeshire, England. Sponsor: International Tungsten Industry Association. Report date: 08 September 1999.
- Huntingdon Life Sciences, Ltd. (1999j). Sodium tungstate dihydrate skin irritation to the rabbit. Report No. ITU 004/982758/SE. [Unpublished Report] Cambridgeshire, England. Sponsor: International Tungsten Industry Association. Report date: 08 September 1999.
- Huntingdon Life Sciences, Ltd. (1999k). Sodium tungstate dihydrate skin sensitization to the Guinea-pig. Report No. ITU 006/983091/SS. [Unpublished Report] Cambridgeshire, England. Sponsor: International Tungsten Industry Association. Report date: 01 April 1999.
- Huntingdon Life Sciences, Ltd. (1999l). Tungsten carbide powder - pure acute (four-hour) inhalation study in rats. Report No. ITU 019/983502. [Unpublished Report] Cambridgeshire, England. Sponsor: International Tungsten Industry Association. Report date: 28 May 1999.
- Huntingdon Life Sciences, Ltd. (1999m). Tungsten carbide powder - pure acute dermal toxicity to the rat. Report No. ITU 015/982706/AC. [Unpublished Report] Cambridgeshire, England. Sponsor: International Tungsten Industry Association. Report date: 24 June 1999.
- Huntingdon Life Sciences, Ltd. (1999n). Tungsten carbide powder - pure acute oral toxicity to the rat. Report No. ITU 014/982705/AC. [Unpublished Report] Cambridgeshire, England. Sponsor: International Tungsten Industry Association. Report date: 24 June 1999.
- Huntingdon Life Sciences, Ltd. (1999o). Tungsten carbide powder - pure eye irritation to the rabbit. Report No. ITU 017/982713/SE. [Unpublished Report] Cambridgeshire, England. Sponsor: International Tungsten Industry Association. Report date: 08 September 1999.
- Huntingdon Life Sciences, Ltd. (1999p). Tungsten carbide powder - pure skin irritation to the rabbit. Report No. ITU 016/982643/SE. [Unpublished Report] Cambridgeshire, England. Sponsor: International Tungsten Industry Association. Report date: 08 September 1999.
- Huntingdon Life Sciences, Ltd. (1999q). Tungsten carbide powder - pure skin sensitization to the Guinea-pig. Report No. ITU 018/983092/SS. [Unpublished Report] Cambridgeshire, England. Sponsor: International Tungsten Industry Association. Report date: 01 April 1999.
- Huntingdon Life Sciences, Ltd. (1999r). Tungsten metal powder - acute (four-hour) inhalation study. Report No. ITU 025/983503. [Unpublished Report] Cambridgeshire, England. Sponsor: International Tungsten Industry Association. Report date: 28 May 1999.
- Huntingdon Life Sciences, Ltd. (1999s). Tungsten metal powder acute dermal toxicity to the rat. Report No. ITU 021/983005/AC. [Unpublished Report] Cambridgeshire, England. Sponsor: International Tungsten Industry Association. Report date: 24 June 1999.
- Huntingdon Life Sciences, Ltd. (1999t). Tungsten metal powder acute oral toxicity to the rat. Report No. ITU 020/983126/AC. [Unpublished Report] Cambridgeshire, England. Sponsor: International Tungsten Industry Associaiton. Report date: 24 June 1999.
- Huntingdon Life Sciences, Ltd. (1999u). Tungsten metal powder skin sensitization to the Guinea-pig. Report No. ITU 024/983200/SS. [Unpublished Report] Cambridgeshire, England. Sponsor: International Tungsten Industry Association. Report date: 07 April 1999.
- Huntingdon Life Sciences, Ltd. (2000a). Tungsten metal powder eye irritation to the rabbit. Report No. ITU 023/982949/SE. [Unpublished Report] Cambridgeshire, England. Sponsor: International Tungsten Industry Association. Report date: 04 January 2000.
- Huntingdon Life Sciences, Ltd. (2000b). Tungsten metal powder skin irritation to the rabbit. Report No. ITU 022/982851/SE. [Unpublished Report] Cambridgeshire, England. Sponsor: International Tungsten Industry Association. Report date: 04 January 2000.
- Huntingdon Life Sciences, Ltd. (2001a). Tungsten carbide DS100 bacterial mutation assay. Report No. SKC 032/004688. [Unpublished Report] Cambridgeshire, England. Sponsor: International Tungsten Industry Association. Report date: 08 February 2001.
- Huntingdon Life Sciences, Ltd. (2001b). Tungsten carbide powder DS 100. In vitro mammalian chromosome aberration test in human lymphocytes. Report No. SKC 033/012208. [Unpublished Report] Cambridgeshire, England. Sponsor: International Tungsten Industry Association. Report date: 12 April 2001.
- Hussain SM, Hess KL, Gearhart JM, Geiss KT, Schlager JJ. (2005). In vitro toxicity of nanoparticles in BRL 3A rat liver cells. Toxicol in Vitro, 19, 975–83.
- ICMM. (2007). Health risk assessment guidance for metals (HERAG). [Online] Available at: http://www.ebrc.de/industrial-chemicals-reach/projects-and-references/herag.php. Accessed on 23 May 2014.
- IITRI. (2010a). Acute oral toxicity study (Limit Test) of ammonium metatungstate in rats. Report No. 22488. [Unpublished Report] Chicago, IL. Sponsor: International Tungsten Industry Association. Report date: 20 December 2010.
- IITRI. (2010b). Bioaccesibility studies of five tungsten substances using simulated gastric, alveolar, interstitial, lysosomal and sweat fluids. Report No. 2234-001. [Unpublished Report] Chicago, IL. Sponsor: International Tungsten Industry Association. Report date: 14 September 2010.
- IITRI. (2010c). Evaluation of potential clastogenic activity of tungsten yellow oxide using the structural chromosomal aberration assay in Chinese hamster ovary (CHO) cell. Report No. 2248-001. [Unpublished Report] Chicago, IL. Sponsor: International Tungsten Industry Association. Report date: 07 July 2010.
- Jackson M, Lemus-Olalde R, Inhof C, Venezia C, Pardus M. (2013). Development of worker inhalation derived no effect levels for tungsten compounds. J Toxicol Environ Health B Crit Rev, 16, 114–26.
- Jain RB. (2013). Effect of pregnancy on the levels of urinary metals for females aged 17–39 years old: data from National Health and Nutrition Examination Survey 2003–2010. J Toxicol Environ Health A, 76, 86–97.
- Johnson DR, Ang C, Bednar AJ, Inouye LS. (2010). Tungsten effects on phosphate-dependent biochemical pathways are species and liver cell line dependent. Toxicol Sci, 116, 523–32.
- Kalinich JF. (2011). Carcinogenicity of embedded tungsten alloys in mice, final report. U.S. Army Medical Research and Materiel Command, Rockville, MD. Award No. WX81XWH-06-2-0025. [Online] Available at: http://www.dtic.mil/dtic/tr/fulltext/u2/a553208.pdf. Accessed on 23 May 2014.
- Kalinich JF, Emond CA, Dalton TK, Mog SR, Coleman GD, Kordell JE, et al. (2005). Embedded weapons-grade tungsten alloy shrapnel rapidly induces metastatic high-grade rhabdomyosarcomas in F344 rats. Environ Health Perspect, 113, 729–34.
- Keith LS, Moffett D, Rosemond ZA, Wohlers DW, Agency for Toxic Substances and Disease Registry. (2007). ATSDR evaluation of health effects of tungsten and relevance to public health. Toxicol Ind Health, 23, 347–87.
- Keith S, Moffett D, Rosemond ZA, Wohlers D. (2005). Toxicological profile for tungsten. [Online] Available at: http://stacks.cdc.gov/view/cdc/7004/. Accessed on 23 May 2014.
- Kelly ADR, Lemaire M, Young YK, Eustache JH, Guilbert C, Molina MF, Mann KK. (2013). In vivo tungsten exposure alters B-cell development and increases DNA damage in murine bone marrow. Toxicol Sci, 131, 434–46.
- Khoury W, Weinbroum AA. (2012). Oxidants as important determinants of renal apoptosis during pneumoperitoneum: a study in an isolated perfused rat kidney model. Surg Endosc, 26, 1417–24.
- Klimisch HJ, Andreae M, Tillmann U. (1997). A systematic approach for evaluating the quality of experimental toxicological and ecotoxicological data. Regul Toxicol Pharmacol, 25, 1–5.
- Klocke R, Mani AR, Moore KP, Blake DR, Mapp PI. (2005). Inactivation of xanthine oxidoreductase is associated with increased joint swelling and nitrotyrosine formation in acute antigen-induced arthritis. Clin Exp Rheumatol, 23, 345–50.
- Kocamaz E, Adiguzel E, Er B, Gundogdu G, Kucukatay V. (2012). Sulfite leads to neuron loss in the hippocampus of both normal and SOX-deficient rats. Neurochem Int, 61, 341–6.
- Koutsospyros A, Braida W, Christodoulatos C, Dermatas D, Strigul N. (2006). A review of tungsten: from environmental obscurity to scrutiny. J Hazard Mater, 136, 1–19.
- Kucukatay V, Hacioglu G, Savcioglu F, Yargicoglu P, Agar A. (2006). Visual evoked potentials in normal and sulfite oxidase deficient rats exposed to ingested sulfite. Neurotoxicology, 27, 93–100.
- Kucukatay V, Bor-Kucukatay M, Atsak P, Ağar A. (2007). Effect of ingested sulfite on hippocampus antioxidant enzyme activities in sulfite oxidase competent and deficient rats. Int J Neurosci, 117, 971–83.
- Kühnel D, Scheffler K, Wellner P, Meißner T, Potthoff A, Busch W, et al. (2012). Comparative evaluation of particle properties, formation of reactive oxygen species and genotoxic potential of tungsten carbide based nanoparticles in vitro. J Hazard Mater, 227–228, 418–26.
- Lanone S, Rogerieux F, Geys J, Dupont A, Maillot-Marechal E, Boczkowski J, et al. (2009). Comparative toxicity of 24 manufactured nanoparticles in human alveolar epithelial and macrophage cell lines. Part Fibre Toxicol, 6, 14.
- Lassner S, Schubert W-D. (1999). Industrial production. In: Tungsten: Properties, Chemistry, Technology of the Elements, Alloys, and Chemical Compounds. New York, USA: Kluwer Academic/Plenum Publishers, 179–253.
- Lee SH, Cho HG, Lee SI. (2008). The effects of tungstate on skin lesions caused by PPD in rats. Biol Pharm Bull, 31, 681–4.
- Leggett RW. (1997). A model of the distribution and retention of tungsten in the human body. Sci Total Environ, 206, 147–65.
- Leite MF, Nicolau J. (2009). Sodium tungstate on some biochemical parameters of the parotid salivary gland of streptozotocin-induced diabetic rats: a short-term study. Biol Trace Elem Res, 127, 154–63.
- Liao RZ. (2013). Why is the molybdenum-substituted tungsten-dependent formaldehyde ferredoxin oxidoreductase not active? A quantum chemical study. J Biol Inorg Chem, 18, 175–81.
- Liu TT, Liu YJ, Wang Q, XG Y, Wang K. (2012). Reactive-oxygen-species-mediated Cdc25C degradation results in differential antiproliferative activities of vanadate, tungstate, and molybdate in the PC-3 human prostate cancer cell line. J Biol Inorg Chem, 17, 311–20.
- Llerena F, Maynar M, Barrientos G, Palomo R, Robles MC, Caballero MJ. (2012). Comparison of urine toxic metals concentrations in athletes and in sedentary subjects living in the same area of Extremadura (Spain). Eur J Appl Physiol, 112, 3027–31.
- Lombaert N, Castrucci E, Decordier I, Van Hummelen P, Kirsch-Volders M, Cundari E, Lison D. (2013). Hard-metal (WC-Co) particles trigger a signaling cascade involving p38 MAPK, HIF-1alpha, HMOX1, and p53 activation in human PBMC. Arch Toxicol, 87, 259–68.
- Machado BI, Suro RM, Garza KM, Murr LE. (2011). Comparative microstructures and cytotoxicity assays for ballistic aerosols composed of micrometals and nanometals: respiratory health implications. Int J Nanomedicine, 6, 167–78.
- Markovich D. (2014). Na(+)-sulfate cotransporter SLC13A1. Pflugers Arch, 466, 131–7.
- McCain WC, Crouse L, Thompson M, Quinn M, Bazar M, Beall P, et al. (2010). Subchronic oral toxicity of sodium tungstate in Sprague-Dawley rats. [Unpublished Report] Hillsborough, NC. Sponsor: U.S. Army Center for Health Promotion and Preventive Medicine.
- McDonald JD, Weber WM, Marr R, Kracko D, Khain H, Arimoto R. (2007). Disposition and clearance of tungsten after single-dose oral and intravenous exposure in rodents. J Toxicol Environ Health A, 70, 829–36.
- McInturf SM, Bekkedal MYV, Wilfong E, Arfsten D, Chapman G, Gunasekar PG. (2011). The potential reproductive, neurobehavioral and systemic effects of soluble sodium tungstate exposure in Sprague–Dawley rats. Toxicol Appl Pharmacol, 254, 133–7.
- McInturf SM, Bekkedal MYV, Wilfong E, Arfsten D, Gunasekar PG, Chapman GD. (2008). Neurobehavioral effects of sodium tungstate exposure on rats and their progeny. Neurotoxicol Teratol, 30, 455–61.
- Mehrotra P, Mishra KP, Raman G, Banerjee G. (2005). Differential regulation of free radicals (reactive oxygen and nitrogen species) by contact allergens and irritants in human keratinocyte cell line. Toxicol Mech Methods, 15, 343–50.
- Mendel RR, Kruse T. (2012). Cell biology of molybdenum in plants and humans. Biochim Biophys Acta, 1823, 1568–79.
- Mendy A, Gasana J, Vieira ER. (2012). Urinary heavy metals and associated medical conditions in the US adult population. Int J Environ Health Res, 22, 105–18.
- Ministry of Health Canada. (2013). Second Report. On human biomonitoring of environmental chemicals in Canada. Health Measures Survey Cycle 2 (2009–2011). [Online] Available at: http://www.hc-sc.gc.ca/ewh-semt/contaminants/human-humaine/index-eng.php. Accessed on 23 May 2014.
- Miyazono Y, Gao F, Horie T. (2004). Oxidative stress contributes to methotrexate-induced small intestinal toxicity in rats. Scand J Gastroenterol, 39, 1119–27.
- Mizuashi M, Ohtani T, Nakagawa S, Aiba S. (2005). Redox imbalance induced by contact sensitizers triggers the maturation of dendritic cells. J Invest Dermatol, 124, 579–86.
- Nacitarhan C, Kucukatay V, Sadan G, Ozturk OH, Agar A. (2008). Effects of sulphite supplementation on vascular responsiveness in sulphite oxidase-deficient rats. Clin Exp Pharmacol Physiol, 35, 268–72.
- Navas-Acien A, Silbergeld EK, Sharrett R, Calderon-Aranda E, Selvin E, Guallar E. (2005). Metals in urine and peripheral arterial disease. Environ Health Perspect, 113, 164–69.
- Niknahad H, O’Brien PJ. (2008). Mechanism of sulfite cytotoxicity in isolated rat hepatocytes. Chem Biol Interact, 174, 147–54.
- Nocito L, Zafra D, Calbó J, Domínguez J, Guinovart JJ. (2012). Tungstate reduces the expression of gluconeogenic enzymes in STZ rats. PLoS One, 7, e42305.
- NTP. (2012). NTP range-finding report: immunotoxicity of sodium tungstate dihydrate in female B6C3F1/N mice (CASRN: 10213-10-2). [Online] Available at: http://ntp.niehs.nih.gov/?objectid = 01DA0FF7-D366-1995-E67280574CD3B952. Accessed on 23 May 2014.
- NTP. (2014a). Sodium tungstate, dihydrate - M030038; CASRN: 10213-10-2. [Online] Available at: http://ntp.niehs.nih.gov/testing/status/agents/ts-m030038.html. Accessed on 10 November 2014.
- NTP. (2014b). Ttungsten trioxide - M030034; CASRN: 1314-35-8. [Online] Available at: http://ntp.niehs.nih.gov/testing/status/agents/ts-m030034.html. Accessed on 10 November 2014.
- NTP. (2014c). Tungsten - M020051; CASRN: 7440-33-7. [Online] Available at: http://ntp.niehs.nih.gov/testing/status/agents/ts-m020051.html. Accessed on 23 May 2014.
- NTP. (2014d). Tungsten suboxide fibers-12080; CASRN: TUNGSTENFIB. [Online] Available at: http://ntp.niehs.nih.gov/testing/status/agents/ts-12080.html. Accessed on 15 October 2014.
- Oberdorster G, Ferin J, Lehnert BE. (1994). Correlation between particle size, in vivo particle persistence, and lung injury. Environ Health Perspect, 102 Suppl 5, 173–79.
- OECD. (1992). OECD guideline for the testing of chemicals. Test no. 406: skin sensitisation. [Online] Available at: http://www.oecd-ilibrary.org/environment/test-no-406-skin-sensitisation_9789264070660-en. Accessed on 10 November 2014.
- OECD. (1997a). OECD guideline for the testing of chemicals. Test no. 471: bacterial reverse mutation test. [Online] Available at: http://www.oecd-ilibrary.org/environment/test-no-471-bacterial-reverse-mutation-test_9789264071247-en. Accessed on 10 November 2014.
- OECD. (1997b). OECD guideline for the testing of chemicals. Test no. 473: in vitro mammalian chromosome aberration test. [Online] Available at: http://www.oecd-ilibrary.org/environment/test-no-473-in-vitro-mammalian-chromosome-aberration-test_9789264071261-en. Accessed on 10 November 2014.
- OECD. (1997c). OECD guideline for the testing of chemicals. Test no. 474: mammalian erythrocyte micronucleus test. [Online] Available at: http://www.oecd-ilibrary.org/environment/test-no-474-mammalian-erythrocyte-micronucleus-test_9789264071285-en. Accessed on 10 November 2014.
- OECD. (1997d). OECD guideline for the testing of chemicals. Test no. 476: in vitro mammalian cell gene mutation test. [Online] Available at: http://www.oecd-ilibrary.org/environment/test-no-476-in-vitro-mammalian-cell-gene-mutation-test_9789264071322-en. Accessed on 10 November 2014.
- OECD. (2012a). OECD guideline for the testing of chemicals. Test no. 404: acute dermal irritation/corrosion. [Online] Available at: http://www.oecd-ilibrary.org/environment/test-no-404-acute-dermal-irritation-corrosion_9789264070622-en. Accessed on 10 November 2014.
- OECD. (2012b). OECD guideline for the testing of chemicals. Test no. 405: acute eye irritation/corrosion. [Online] Available at: http://www.oecd-ilibrary.org/environment/test-no-405-acute-eye-irritation-corrosion_9789264185333-en. Accessed on 10 November 2014.
- OECD. (2014). Manual for the assessment of chemicals. [Online] Available at: http://www.oecd.org/fr/env/ess/risques/manualfortheassessmentofchemicals.htm. Accessed on 04 November 2014.
- OECD SIDS. (2005). Tungsten carbide. CAS N: 12070-12-1. [Unpublished Report] Washington, DC. Available at: http://www.inchem.org/documents/sids/sids/12070121.pdf. Sponsor: HC Starck GmbH. Report date: 23 December 2005.
- Oliveira JM, Rebuffat SA, Gasa R, Burks DJ, Garcia A, Kalko SG, et al. (2014). Tungstate promotes β-cell survival in Irs2-/- mice. Am J Physiol Endocrinol Metab, 306, E36–E47.
- OSHA. (2014a). Tungsten (as W) insoluble compounds. [Online] Available at: https://www.osha.gov/dts/chemicalsampling/data/CH_274500.html. Accessed on 10 November 2014.
- OSHA. (2014b). Tungsten (as W) soluble compounds. [Online] Available at: https://www.osha.gov/dts/chemicalsampling/data/CH_274600.html. Accessed on 10 November 2014.
- Osier M, Oberdörster G. (1997). Intratracheal inhalation vs intratracheal instillation: differences in particle effects. Fundam Appl Toxicol, 40, 220–7.
- Osterburg AR, Robinson CT, Mokashi V, Stockelman M, Schwemberger SJ, Chapman G, Babcock GF. (2014). Oral tungstate (Na2WO4) exposure reduces adaptive immune responses in mice after challenge. J Immunotoxicol, 11, 148–59.
- Osterburg AR, Robinson CT, Schwemberger S, Mokashi V, Stockelman M, Babcock GF. (2010). Sodium tungstate (Na2WO4) exposure increases apoptosis in human peripheral blood lymphocytes. J Immunotoxicol, 7, 174–82.
- Ozturk OH, Oktar S, Aydin M, Kucukatay V. (2010). Effect of sulfite on antioxidant enzymes and lipid peroxidation in normal and sulfite oxidase-deficient rat erythrocytes. J Physiol Biochem, 66, 205–12.
- Padilla MA, Elobeid M, Ruden DM, Allison DB. (2010). An examination of the association of selected toxic metals with total and central obesity indices: NHANES 99-02. Int J Environ Res Public Health, 7, 3332–47.
- Pardo M, Shuster-Meiseles T, Levin-Zaidman S, Rudich A, Rudich Y. (2014). Low cytotoxicity of inorganic nanotubes and fullerene-like nanostructures in human bronchial epithelial cells: relation to inflammatory gene induction and antioxidant response. Environ Sci Technol, 48, 3457–66.
- Pawa S, Ali S. (2004). Liver necrosis and fulminant hepatic failure in rats: protection by oxyanionic form of tungsten. Biochim Biophys Acta, 1688, 210–22.
- Peredo HA, Zabalza M, Mayer MA, Carranza A, Puyó AM. (2010). Sodium tungstate and vanadyl sulfate effects on blood pressure and vascular prostanoids production in fructose-overloaded rats. Clin Exp Hypertens, 32, 453–57.
- Radcliffe PM, Leavens TL, Wagner DJ, Olabisi AO, Struve MF, Won BA, et al. (2010). Pharmacokinetics of radiolabeled tungsten (188W) in male Sprague–Dawley rats following acute sodium tungstate inhalation. Inhal Toxicol, 22, 69–76.
- Radcliffe PM, Olabisi AO, Wagner DJ, Leavens T, Wong BA, Struve MF, et al. (2009). Acute sodium tungstate inhalation is associated with minimal olfactory transport of tungsten (188W) to the rat brain. Neurotoxicology, 30, 445–50.
- Rajendran N, Shu-Chieh H, Dennis S, Miguel M, Detrisac C, Venezia C. (2012). Toxicologic evaluation of tungsten: 28-day inhalation study of tungsten blue oxide in rats. Inhal Toxicol, 24, 985–94.
- RCC NOTOX BV. (1991a). Acute eye irritation/corrosion study with sodium metatungstate in the rabbit. Report No. 042378. [Unpublished Report] Hertogenbosch, The Netherlands. Sponsor: HC Starck GmbH. Report date: 20 June 1991.
- RCC NOTOX BV. (1991b). Assessment of acute dermal toxicity with sodium metatungstate in the rat. Report No. 042389. [Unpublished Report] Hertogenbosch, The Netherlands. Sponsor: HC Starck GmbH. Report date: 07 January 1991.
- RCC NOTOX BV. (1991c). Assessment of acute oral toxicity with sodium metatungstate in the rat. Report No. 042176. [Unpublished Report] Hertogenbosch, The Netherlands. Sponsor: HC Starck GmbH. Report date: 28 February 1991.
- RCC NOTOX BV. (1991d). Assessment of contact hypersensitivity to sodium metatungstate in the albino Guinea pig (maximization-test). Report No. 042187. [Unpublished Report] Hertogenbosch, The Netherlands. Sponsor: HC Starck GmbH. Report date: 31 January 1991.
- RCC NOTOX BV. (1991e). Primary skin irritation/corrosion study with sodium metatungstate in the rabbit (4-hour semi-occlusive application). Report No. 042367. [Unpublished Report] Hertogenbosch, The Netherlands. Sponsor: HC Starck GmbH. Report date: 18 March 1991.
- RCC NOTOX BV. (1992). Determination of the water solubility of sodium metatungstate. Report No. 042301. [Unpublished Report] Hertogenbosch, The Netherlands. Sponsor: HC Starck GmbH. Report date: 08 April 1992.
- Rodríguez-Fariñas N, Gomez-Gomez MM, Camara-Rica C. (2008). Study of tungstate-protein interaction in human serum by LC-ICP-MS and MALDI-TOF. Anal Bioanal Chem, 390, 29–35.
- Rubin CS, Holmes AK, Belson MG, Jones RL, Flanders WD, Kieszak SM, et al. (2007). Investigating childhood leukemia in Churchill County, Nevada. Environ Health Perspect, 115, 151–57.
- Sachdeva S, Flora SJS. (2014). Efficacy of some antioxidants supplementation in reducing oxidative stress post sodium tungstate exposure in male wistar rats. J Trace Elem Med Biol, 28, 233–39.
- Sachdeva S, Kushwaha P, Flora SJ. (2013). Effects of sodium tungstate on oxidative stress enzymes in rats. Toxicol Mech Methods, 23, 519–27.
- Schell JD, Pardus MJ. (2009). Preliminary risk-based concentrations for tungsten in soil and drinking water. Land Contamination & Reclamation, 17, 179–88.
- Schröder K, Vecchione C, Jung O, Schreiber JG, Shiri-Sverdlov R, van Gorp PJ, et al. (2006). Xanthine oxidase inhibitor tungsten prevents the development of atherosclerosis in ApoE knockout mice fed a Western-type diet. Free Radic Biol Med, 41, 1353–60.
- Schroeder HA, Mitchener M. (1975). Life-term studies in rats: effects of aluminum, barium, beryllium, and tungsten. J Nutr, 105, 421–27.
- Schuster BE, Roszell LE, Murr LE, Ramirez DA, Demaree JD, Klotz BR, et al. (2012). In vivo corrosion, tumor outcome, and microarray gene expression for two types of muscle-implanted tungsten alloys. Toxicol Appl Pharmacol, 265, 128–38.
- Seiler RL, Stollenwerk KG, Garbarino JR. (2005). Factors controlling tungsten concentrations in ground water, Carson Desert, Nevada. Appl Geochem, 20, 423–41.
- Shan D, Xie Y, Ren G, Yang Z. (2012). Inhibitory effect of tungsten carbide nanoparticles on voltage-gated potassium currents of hippocampal CA1 neurons. Toxicol Lett, 209, 129–35.
- Shan D, Xie Y, Ren G, Yang Z. (2013). Attenuated effect of tungsten carbide nanoparticles on voltage-gated sodium current of hippocampal CA1 pyramidal neurons. Toxicol In Vitro, 27, 299–304.
- Sharma HS, Ali SF, Hussain SM, Schlager JJ, Sharma A. (2009). Influence of engineered nanoparticles from metals on the blood-brain barrier permeability, cerebral blood flow, brain edema and neurotoxicity. An experimental study in the rat and mice using biochemical and morphological approaches. J Nanosci Nanotechnol, 9, 5055–72.
- Shiue I. (2013). Urinary environmental chemical concentrations and vitamin D are associated with vision, hearing, and balance disorders in the elderly. Environ Int, 53, 41–6.
- Stefaniak AB. (2010). Persistence of tungsten oxide particle/fiber mixtures in artificial human lung fluids. Part Fibre Toxicol, 7, 38.
- Stefaniak AB, Harvey CJ, Virji MA, Day GA. (2010a). Dissolution of cemented carbide powders in artificial sweat: implications for cobalt sensitization and contact dermatitis. J Environ Monit, 12, 1815–22.
- Stefaniak AB, Abbas Virji M, Harvey CJ, Sbarra DC, Day GA, Hoover MD. (2010b). Influence of artificial gastric juice composition on bioaccessibility of cobalt- and tungsten-containing powders. Int J Hyg Environ Health, 213, 107–15.
- Stefaniak A, Harvey CJ, Bukowski VC, Leonard SS. (2010c). Comparison of free radical generation by pre- and post-sintered cemented carbide particles. J Occup Environ Hyg, 7, 23–34.
- Sun K, Kiss E, Bedke J, Stojanovic T, Li Y, Gwinner W, Gröne HJ. (2004). Role of xanthine oxidoreductase in experimental acute renal-allograft rejection. Transplantation, 77, 1683–92.
- Swenson TL, Casida JE. (2013). Aldehyde oxidase importance in vivo in xenobiotic metabolism: imidacloprid nitroreduction in mice. Toxicol Sci, 133, 22–8.
- Thongkumkoon P, Sangwijit K, Chaiwong C, Thongtem S, Singjai P, Yu LD. (2014). Direct nanomaterial-DNA contact effects on DNA and mutation induction. Toxicol Lett, 226, 90–7.
- Tutuncu B, Kuçukatay V, Arslan S, Sahin B, Semiz A, Sen A. (2012). Alteration of drug metabolizing enzymes in sulphite oxidase deficiency. J Clin Biochem Nutr, 51, 50–4.
- Tyrrell J, Galloway TS, Abo-Zaid G, Melzer D, Depledge MH, Osborne NJ. (2013). High urinary tungsten concentration is associated with stroke in the National Health and Nutrition Examination Survey 1999–2010. PLoS One, 8, e77546.
- US EPA. (1988). Recommendations for and documentation of biological values for use in risk assessment. [Online] Available at: http://cfpub.epa.gov/ncea/cfm/recordisplay.cfm?deid = 34855. Accessed on 23 May 2014.
- Uskokovic-Markovic S, Milenkovic M, Topic A, Kotur S, Stefanovic A, Antic-Stankovic J. (2007). Protective effects of tungstophosphoric acid and sodium tungstate on chemically induced liver necrosis in wistar rats. J Pharm Pharm Sci, 10, 340–49.
- Van Goethem F, Lison D, Kirsch-Volders M. (1997). Comparative evaluation of the in vitro micronucleus test and the alkaline single cell gel electrophoresis assay for the detection of DNA damaging agents: genotoxic effects of cobalt powder, tungsten carbide and cobalt-tungsten carbide. Mutat Res, 392, 31–43.
- Wang BJ, Guo YL, Chang H, Sheu HM, Pan MH, Lee YH, Wang YJ. (2010). N-acetylcysteine inhibits chromium hypersensitivity in coadjuvant chromium-sensitized albino guinea pigs by suppressing the effects of reactive oxygen species. Exp Dermatol, 19, e191–e200.
- Weast RC. (1973). Handbook of Chemistry and Physics. 54th Ed. Boca Raton, Florida: CRC Press Inc.
- Weber WM, Marr R, Kracko D, Gao Z, McDonald JD, Ui Chearnaigh K. (2008). Disposition of tungsten in rodents after repeat oral and drinking water exposures. Toxicol Environ Chem, 90, 445–55.
- WHO. (2012). Guidance for Immunotoxicity Risk Assessment for Chemicals. [Online] Available at:http://www.who.int/ipcs/methods/harmonization/areas/guidance_immunotoxicity.pdf?ua = 1. Accessed on 10 November 2014.
- Wright RM, Ginger LA, Kosila N, Elkins ND, Essary B, McManaman JL, Repine JE. (2004). Mononuclear phagocyte xanthine oxidoreductase contributes to cytokine-induced acute lung injury. Am J Respir Cell Mol Biol, 30, 479–90.
- Yorita Christensen KL. (2013). Metals in blood and urine, and thyroid function among adults in the United States 2007–2008. Int J Hyg Environ Health, 216, 624–32.