Abstract
Oral delivery of 5-fluorouracil (5-FU) is difficult due to its serious adverse effects and extremely low bioavailability. Therefore, the aim of present investigation was to develop and evaluate low HLB surfactant nanoemulsion of 5-FU for topical chemoprevention of skin cancer. Low HLB surfactant nanoemulsions were prepared by oil phase titration method. Thermodynamically stable nanoemulsions were characterized in terms of droplet size distribution, zeta potential, viscosity and refractive index. Selected formulations and control were subjected to in vitro skin permeation studies through rat skin using Franz diffusion cells. Optimized formulation F9 was subjected to stability and in vitro cytotoxic studies on melanoma cell lines. Enhancement ratio was found to be 22.33 in formulation F9 compared with control and other formulations. The values of steady state flux and permeability coefficient for formulation F9 were found to be 206.40 ± 14.56 µg cm−2 h−1 and 2.064 × 10−2 ± 0.050 × 10−2 cm h−1, respectively. Optimized formulation F9 was found to be physical stable. In vitro cytotoxicity studies on SK-MEL-5 cancer cells indicated that 5-FU in optimized nanoemulsion is much more efficacious than free 5-FU. From these results, it can be concluded that the developed nanoemulsion might be a promising vehicle for chemoprevention of skin cancer.
Introduction
Low HLB surfactant nanoemulsions are thermodynamically/thermokinetically stable dispersions of water, oil, surfactant and cosurfactant with droplet of size in the magnitude of nanometer range (Hwangl et al., Citation2009; Shakeel & Ramadan, Citation2010). In these nanoemulsions, an aqueous phase is dispersed into an external oil phase with the help of low HLB surfactants. In the recent years, nanoemulsions have been investigated as safe and pharmaceutically acceptable nanovehicles for dermal/topical (Shakeel et al., Citation2012), transdermal (Shakeel et al., Citation2009), oral (Shafiq et al., Citation2007), intranasal (Jain et al., Citation2011), ophthalmic (Ammar et al., Citation2009) and parenteral drug delivery (Ahmed et al., Citation2008) of several lipophilic drugs. Nevertheless, there is scarcity of scientific literature available for drug delivery of hydrophilic drugs using nanoemulsions. 5-Fluorouracil (5-FU) is a well known hydrophilic anticancer drug which has been used for the treatment of various kinds of cancers like breast cancer, colon cancer, lungs cancer and skin cancer (Diasio & Johnson, Citation2000; Bargman & Hochman, Citation2003; Rahman et al., Citation2006; Yassin et al., Citation2010). Moreover, 5-FU has also been used in the treatment of psoriasis and actinic keratosis (Robins & Gupta, Citation2002). It shows serious adverse effects and poor in vivo absorption with erratic and extremely low bioavailability when administered orally. Transdermal route is an alternative route to avoid adverse effects associated with its oral administration and to enhance its systemic bioavailability (Shakeel et al., Citation2009, Citation2012; Shakeel & Ramadan, Citation2010). Currently, topical and injectable formulations of 5-FU are available in the market. Oral formulations of 5-FU are not available in the market due to serious adverse effects and extremely low bioavailability. The dermal/transdermal permeation of 5-FU through highly lipophilic human stratum corneum is too low because of its hydrophilic characteristics. Therefore, many attempts namely microemulsions (Gupta et al., Citation2005; Elmeshad & Tadros, Citation2011), transferosomes (Alvi et al., Citation2011), ethosomes (Zhang et al., Citation2012), niosomes (Cosco et al., Citation2009; Alvi et al., Citation2011), liposomes (Alvi et al., Citation2011; Thomas et al., Citation2011), nanoparticles (Zhu et al., Citation2009; Chauhan et al., Citation2012), iontophoresis (Fang et al., Citation2004; Singh & Jayaswal, Citation2008), phonophoresis (Meidan et al., Citation1999), electroporation (Fang et al., Citation2004) and use of chemical enhancers (Singh et al., Citation2005; Khan et al., Citation2011) have been made to increase transdermal/topical delivery of 5-FU. In the recent literatures, oil based nanocarriers like nanoemulsions and microemulsions showed significant advantages over other unstable dispersion like emulsions and suspensions in terms of enhanced solubilization, enhanced permeation, enhanced bioavailability and thermodynamic stability for transdermal/topical delivery of many lipophilic drugs both in vitro as well as in vivo (Spernath et al., Citation2007; Elmaghraby, Citation2008; Hwangl et al., Citation2009; Shakeel et al., Citation2009, Citation2012; Shakeel & Ramadan, Citation2010; Raza et al., Citation2011). Oil based nanocarriers have not been investigated extensively for transdermal/topical delivery of hydrophilic drugs (Gupta et al., Citation2005; Shakeel & Ramadan, Citation2010; Elmeshad & Tadros, Citation2011). Therefore, the aim of present study was to develop and evaluate w/o nanoemulsions of hydrophilic drug 5-FU for topical chemoprevention of skin cancer using nontoxic, low HLB surfactants. Low HLB surfactants were used for preparation of these nanoemulsions because they favor the formation of w/o nanoemulsions whereas higher HLB surfactants favor the formation of o/w nanoemulsions.
Materials and methods
Materials
5-FU (mass fraction purity of 0.992) was kindly donated as a gift sample from Alfa Aesar, A Johnson Metthey Company (Ward Hill, MA). Propylene glycol monolaurate (Lauroglycol-90) (mass fraction purity of 0.996) and highly purified diethylene glycol monoethyl ether (Transcutol-HP) (mass fraction purity of 0.9998) were kind gift samples from Gattefosse (Cedex, France). HPLC grade methanol and isopropyl alcohol (IPA) (mass fraction purity of 0.99) were purchased from BDH Laboratory (Liverpool, UK). SK-MEL-5 melanoma human skin cancer cell lines were purchased from American type cell culture collection (ATCC, Manassas, VA). Ultra-pure water was obtained from ELGA water purification unit (Wycombe, Bucks, UK). All other reagents used in the present study were of analytical reagent (AR) grade.
Screening of components for nanoemulsions preparation
Based on pharmaceutical acceptability, nontoxicity, nonirritating potential and safe category of components, Lauroglycol-90 was selected as the oil phase for nanoemulsions development. Surfactants were selected based on their HLB values. Transcutol-HP and IPA were selected as surfactant and cosurfactant, respectively. Deionized water was selected as an aqueous phase because water is frequently used aqueous phase for oil based nanocarriers like nanoemulsions and microemulsions.
Construction of pseudo-ternary phase diagrams and preparation of nanoemulsions
Different low HLB surfactant based w/o nanoemulsions of 5-FU were prepared by spontaneous emulsification method. Stock solutions of Tanscutol-HP (surfactant) and IPA (cosurfactant) were prepared in different weight ratios from 1:0 to 3:1. Deionized water was mixed with a particular Smix ratio and oil was added in drop wise manner till clear and transparent formulation obtained. Pseudo-ternary phase diagrams were constructed to identify clear and transparent zones (nanoemulsions zones) in the phase diagrams () (Shafiq et al., Citation2007; Shakeel et al., Citation2009; Jain et al., Citation2011). Different formulations were selected from each phase diagrams based on solubilization of drug in an aqueous phase and minimum quantity of surfactant and cosurfactant. About 1% w/w (10 mg/g of 5-FU) was dissolved in deionized water and specific amount of surfactant and cosurfactant was added with mild agitation. Required amount of oil phase was added in these systems till clear and transparent formulations obtained. Composition of these formulations is given in .
Figure 1. Pseudo-ternary phase diagrams showing w/o nanoemulsions zones for aqueous phase (deionized water), oil (Lauroglycol-90), surfactant (Transcutol-HP) and cosurfactant (isopropyl alcohol) at different Smix ratios [a (1:0), b (1:1), c (2:1) and d (3:1)].
![Figure 1. Pseudo-ternary phase diagrams showing w/o nanoemulsions zones for aqueous phase (deionized water), oil (Lauroglycol-90), surfactant (Transcutol-HP) and cosurfactant (isopropyl alcohol) at different Smix ratios [a (1:0), b (1:1), c (2:1) and d (3:1)].](/cms/asset/ddc71424-6664-4a01-b140-8d74c620c51e/idrd_a_868557_f0001_c.jpg)
Table 1. Composition of low HLB surfactant based nanoemulsions that passed thermodynamic stability tests.
Thermodynamic stability tests
Developed nanoemulsions were subjected to different thermodynamic stability tests in order to remove metastable and unstable formulations (Shafiq et al., Citation2007; Shakeel et al., Citation2009, Citation2012; Shakeel & Ramadan, Citation2010; Jain et al., Citation2011). These formulations were centrifuged at 5000 rpm for 30 min and observed for any phase separation, creaming, cracking or coalescence. Those formulations which were found to be stable at centrifugation were subjected to heating and cooling cycles. Six cycles between refrigerator temperature (4 °C) and 45 °C with storage at each temperature for 48 h were performed. Those formulations which were stable at heating and cooling cycles were selected for freeze thaw cycles. Three cycles between −21 °C and 20 °C with storage at each temperature for 24 h were performed. Only thermodynamically stable formulations were selected for further characterization and evaluation.
Characterization of nanoemulsions
Developed nanoemulsions were characterized in terms of droplet size distribution, poydispersity index (PI), zeta potential (ZP), viscosity and refractive index (RI).
Droplet size distribution and PI of selected formulations were measured using Brookhaven 90 Plus Particle Size Analyzer (Brookhaven Instrument Corporation, Holtsville, NY). ZP was measured using Brookhaven 90 Plus Zeta Potential Analyzer (Brookhaven Instrument Corporation, Holtsville, NY). Light scattering was monitored at a scattering angle of 90° at 20 ± 1 °C. About 3 ml of each formulation was taken in an acrylic square cell for droplet size and PI measurement. For ZP measurement, samples were taken in glass cells.
The viscosity of undiluted formulations was determined using Brookfield viscometer (Brookfield Engineering Laboratories, Inc, Middleboro, MA) using spindle #CPE40 at 25 ± 0.5 °C.
Refractive index of selected formulations was determined using an Abbes type refractometer (Precision Standard Testing Equipment Corporation, Berlin, Germany).
In vitro skin permeation studies
In vitro skin permeation studies on developed nanoemulsions (F1–F9) and saturated aqueous solution of 5-FU were conducted on a Franz diffusion cells with an effective diffusional area of 1.76 cm2 and ∼12 ml of receiver chamber capacity using rat abdominal skin as a permeation membrane. The automated Logan transdermal diffusion cell sampling system (SFDC6, Logan Instrument Corporation, Avalon, NJ) was used to study in vitro skin permeation studies. Institutional guidelines of King Saud University, Riyadh, Saudi Arabia were followed on these studies with ethical approval. The full-thickness skin was excised from the abdominal region of rats and hairs were removed by an electric clipper. The skin was prepared and stored as per procedure described in literature (Shakeel et al., Citation2009; Shakeel & Ramadan, Citation2010). On the day of transdermal experiment, the skin was mounted between the donor and receiver compartment of the Franz diffusion cell as reported in literature (Elmaghraby, Citation2008; Shakeel et al., Citation2009; Shakeel & Ramadan, Citation2010; Raza et al., Citation2011). Initially, the donor compartment was kept empty and receiver compartment was filled with 12 ml of phosphate buffer saline (PBS). The receiver fluid was stirred with a magnetic rotor at a speed of 100 rpm and the assembled apparatus was placed in the Logan transdermal permeation apparatus and the temperature was maintained at 32 ± 0.5 °C. The whole PBS was replaced every 30 min in order to get stabilization of the skin. It was observed that the receiver fluid showed negligible response (area) after 4.5 h and beyond, indicating complete stabilization of the skin. After stabilization, 1 g of each nanoemulsion formulation (containing 10 mg g−1 of 5-FU) or 1 g of saturated aqueous solution (10 mg g−1) was placed into each donor compartment and sealed with paraffin film to provide occlusive conditions. Saturated aqueous solution of 5-FU was used as control formulation in order to get enhancement factor (Ef). Samples were withdrawn at regular intervals of time (0.5, 1, 2, 4, 6, 8 and 24 h), filtered through a 0.45 -µm membrane filter, suitably diluted with PBS and analyzed for 5-FU content by validated high performance liquid chromatography (HPLC) method at the wavelength of 254 nm using combination of methanol and water (50:50) as a mobile phase (Haq et al., Citation2013).
Permeation data analysis
The cumulative amount of 5-FU permeated through rat abdominal skin (µg cm−2) was plotted against time (h) for each nanoemulsion formulation and control. The drug permeation rate through rat skin at steady state (Jss) was determined by dividing the slope of the linear portion of the graph plotted between cumulative drug permeated and time by the area of the diffusion cell. The permeability coefficient (Kp) and enhancement ratio (Er) were calculated using Equations (Equation1)(1) and Equation(2
(2) ), respectively (Shakeel et al., Citation2009; Shakeel & Ramadan, Citation2010):
(1)
(2)
where, C0 is the initial concentration of 5-FU in donor compartment.
Stability studies on F9
Based on physicochemical characterization and transdermal permeation data, formulation F9 was selected for physical stability studies. Physical stability studies on F9 were performed by keeping the sample of F9 at refrigerator temperature (4 °C) in triplicates. Various physicochemical parameters like droplet size, PI, ZP, viscosity and RI were determined at 0, 1, 2 and 3 months. All these parameters were analyzed as per procedure described under characterization of nanoemulsion section.
In vitro cytotoxicity studies on SK-MEL-5 malignant melanoma cell lines
In vitro cytotoxicity studies on melanoma cell lines were performed to compare the cytotoxic effects of optimized nanoemulsion (F9) with its aqueous solution and control. Optimized nanoemulsion formulation (F9) without 5-FU was used as control. An WST-1 [2-(4-iodophenyl)-3-(4-nitrophenyl)-5-(2, 4-disulfophenyl)-2H-tetrazolium] assay was used to investigate these effects. SK-MEL melanoma cells were cultured in RPMI-1640 medium and supplemented with 10% fetal bovine serum (FBS), 1% ABM (GIBCO), 100 IU ml−1 penicillin, 100 mg ml−1 streptomycin and 2 mmoll−1 of l-glutamine at 37 °C in a 5% CO2 atmosphere.
Cells were seeded into 96-well plates at 0.4 × 104 cells per well and incubated for 24 h in a incubator at 37 °C in a 5% CO2 atmosphere. The medium was replaced with fresh one containing the desired concentrations of aqueous solution, control and F9 in the same volumes. After 72 h, 10 μl of the WST-1 reagent was added to each well and the plates were re-incubated for 4 h at 37 °C. The amount of formazan was quantified using ELISA reader at 450 nm.
Statistical analysis
All results were expressed as mean ± SD (n = 3). Results of in vitro skin permeation, characterization and cytotoxicity were statistically evaluated by one-way analysis of variance (ANOVA) using Dunnett’s test to evaluate the significant differences. The statistical software SPSS Version 11 was used for this purpose.
Results and discussion
Screening of components
For screening of components, solubility of hydrophobic drugs in oil phase is very important as reported in previous literatures (Shafiq et al., Citation2007; Ahmed et al., Citation2008; Ammar et al., Citation2009; Shakeel et al., Citation2009; Jain et al., Citation2011). Our drug (5-FU) is hydrophilic and it could be solubilized in an internal aqueous phase because it is easily soluble in an aqueous media (Singh et al., Citation2005). Therefore, solubility studies of 5-FU were not performed in oil phase. Lauroglycol-90 was randomly selected as the oil phase for the development of optimal nanoemulsion formulations of 5-FU. Due to hydrophilic nature of 5-FU, the aim was to develop w/o nanoemulsions. Therefore, surfactants were selected based on their HLB values. Low HLB value surfactants favor the formation of w/o nanoemulsions whereas higher HLB value surfactants favor the formation of o/w nanoemulsions (Shafiq et al., Citation2007; Shakeel et al., Citation2009, Citation2012; Shakeel & Ramadan, Citation2010). Therefore, surfactants Transcutol-HP and IPA were selected in the present study as both have low HLB values. Transcutol-HP was chosen as surfactant whereas IPA was chosen as cosurfactant. Deionized water was selected as an aqueous phase because water is frequently used aqueous media for nanoemulsions (Shakeel et al., Citation2012).
Preparation and thermodynamic stability tests
Different nanoemulsion formulations were precisely prepared from pseudo-ternary phase diagrams () based on the evidence based performance of different thermodynamic stability tests. About 10 mg of 5-FU (1% w/w) was solubilized in deionized water and then required amount of surfactant and cosurfactant (Smix) was added in different ratios (1:1, 2:1 and 3:1) with drop-wise addition of oil phase till a clear and transparent solution was obtained. The prepared nanoemulsions were tightly sealed in glass vials and subjected to different thermodynamic stability tests namely centrifugation, heating and cooling cycles and freeze thaw cycles. The formulations which were found to be stable at all stress conditions of thermodynamic stability tests were selected for further characterization and evaluation ().
Characterization of nanoemulsions
Thermodynamically stable formulations were further characterized in terms of droplet size distribution, PI, ZP, viscosity and RI for further optimization processes. Results of these parameters are given in . The mean droplet size of selected nanoemulsions (F1–F9) ranged from 68.2 to 124.3 nm. Largest droplet size was observed with formulation F1, which could be due to the presence of higher concentrations of oil (50% w/w) and lower concentration of surfactants (40% w/w of Smix) as shown in . Droplet size analysis of nanoemulsion formulations (F1–F9) showed that the size increased with increase in the concentration of oil. The droplet size of formulation F9 was observed to be lowest (68.2 nm) which could be due to lower concentration of oil, optimum concentration of surfactants and higher Smix ratio. Droplet size of formulations F1–F9 was found to be reduced by increasing the concentration of surfactants and Smix ratio. The PI is related with the uniformity of droplets within the formulation. Lower the value of PI, result in more uniformity of droplets within the formulation. The PI value was found to be <0.4 in case of all formulations which indicated uniformity of droplets within the formulations. Formulation F9 had least PI value (0.219) suggesting highest uniformity in droplet size ().
Table 2. Characterization of developed nanoemulsions in terms of droplet size distribution, zeta potential, refractive index and viscosity (n = 3).
Zeta potential values of nanoemulsions F1–F9 were observed in the range of −26.10 to −24.82 mV. It was reported that ZP values in the range of ±25–30 mV characterizes a stable formulation (Craig et al., Citation1995; Freitas & Muller, Citation1998). There were no significant changes observed in ZP values among all nanoemulsion formulations [F1–F9] (p ≥ 0.05). Therefore, developed nanoemulsions were considered physically as well as thermodynamically stable. The negative values of ZP for all formulations were probably due to the presence of fatty acids and esters in the Lauroglycol-90. The RI of nanoemulsion formulations (F1–F9) was observed in the range of 1.390–1.394 (). These values of RI were very close to the RI of Lauroglycol-90 (RI 1.391) which was use used an external oil phase for preparation of nanoemulsions. These values of RI characterized the w/o nature of drug-loaded nanoemulsions. The viscosity of developed nanoemulsions (F1–F9) was observed in the range of 50.15–106.43 cps (). The viscosity of the formulation F9 was found to be lowest (50.15 cps) as compared to other formulations which could be due to the presence of lower concentration of Lauroglycol-90.
In vitro skin permeation studies
In vitro skin permeation studies were performed to compare the drug permeation rate of developed nanoemulsions (F1–F9) with control. In vitro skin permeation rate was observed highest in formulation F9 and lowest for control (). The skin permeation profile of F9 was found to be highly significant as compared to control and other formulations (p < 0.05). The skin permeation profile of all nanoemulsions (F1–F9) was found to be extremely significant as compared to control (p < 0.05). The skin permeation rate was found to be increased with decreased in droplet size of formulation. The skin permeation rate of control was observed too low that could be due to hydrophilic nature of 5-FU. Hydrophilic drugs are not permeated easily through the skin because of lipophilic nature of stratum corneum (Shakeel et al., Citation2009). The lowest drug permeation profile was obtained with formulation F1 among all nanoemulsions. The lowest and highest permeation profile of F1 and F9 was probably due to the highest and lowest droplet size, respectively.
Permeation data analysis
For determination of permeability parameters like Jss, Kp and Er, the graph was plotted between cumulative 5-FU permeated (µg cm−2) and time (h) as shown in . All permeability parameters of nanoemulsions (F1–F9) were found to be highly significant as compared to aqueous solution of 5-FU (control) [p < 0.05] as shown in . These parameters were found to be highest in formulation F9 and lowest in F1 (). Er was found to be 22.33 in formulation F9 compared with other formulations. The values of Jss and Kp for formulation F9 were found to be 206.40 ± 14.56 µg cm−2 h−1 and 2.064 × 10−2 ± 0.050 × 10−2 cm h−1, respectively (). The enhanced permeability parameters of F9 could be due to the lowest droplet size, lowest viscosity and optimum concentrations of Lauroglycol-90, Transcutol-HP and IPA.
Table 3. Permeability parameters of different w/o nanoemulsions (F1–F9) and control.
Stability studies on F9
Based on lowest droplet size, lowest viscosity and highest skin permeation rate, formulation F9 was selected for stability studies. Optimized formulation F9 was physicochemically characterized in terms of droplet size, PI, viscosity, RI and ZP for the period of 3 months at refrigerator temperature (4 °C). It was observed that droplet size, PI, viscosity, RI and ZP were not changed significantly after storage at refrigerator temperature (). The droplet size and PI were found to be 69.9 nm and 0.226 after 3 months of storage. However, viscosity, RI and ZP were found to be 51.21 cps, 1.340 and −27.08 mV after 3 months of storage (). Overall, these results indicated that optimized formulation F9 is sufficiently stable at refrigerator temperature as there were no significant changes in physicochemical parameters observed.
Table 4. Stability studies on F9 in terms of physicochemical parameters at refrigerator temperature.
In vitro cytotoxic studies on SK-MEL-5 malignant melanoma cell lines
With the background to develop a suitable nanoemulsion formulation for chemoprevention of skin cancer, we evaluated in vitro therapeutic efficacy of optimized formulation F9 on SK-MEL-5 melanoma cancer cell lines. In vitro cytotoxicity (%) was evaluated by exposing different molar concentrations of 5-FU from F9 and aqueous solution in melanoma cell lines. Cell survival (%) was determined at various molar concentrations (3.75, 7.5, 15, 30, 60 and 120 µM) of 5-FU in an aqueous solution (free 5-FU) and F9 (). Melanoma cell lines were found to be highly resistant to free 5-FU (aqueous solution) as no significant cell inhibition was obtained by free 5-FU (). The highest % cytotoxicity was found to be 5.40 ± 0.32 at concentration of 120 µM (). However, the same concentration of 5-FU (120 µM) in optimized nanoemulsion F9 showed 60.60 ± 4.12% cytotoxicity which was much more significant than free 5-FU (p < 0.05). 5-FU in the form of nanoemulsion was found to be much more potent and efficacious than free 5-FU on melanoma cell lines which indicated the ability of nanoemulsion for chemoprevention of skin cancer. However, the incubation of SK-MEL-5 cells with control (blank nanoemulsion) did not inhibit cells growth at greater extent as shown in . Negligible inhibition in cell growth by blank nanoemulsion (control) indicated that developed nanoemulsion was nontoxic and cytotoxic effects of optimized nanoemulsion F9 were due to the presence of 5-FU in nanoemulsion. The concentration of F9 leading to 50% cell death (IC50) was also determined from concentration dependent cell viability curve (). The IC50 value of free 5-FU was not detectable at investigated concentrations because cell growth inhibition was found to be negligible by free 5-FU. However, the IC50 value of 5-FU in F9 was found to be 398 µM (). Based on IC50 value, the 5-FU in nanoemulsion was found to be much more efficacious than free 5-FU which could reduce the dose of 5-FU as well as adverse effects of 5-FU associated with its oral administration. Overall, these results indicated that developed nanoemulsion could be successfully applied topically for chemoprevention of skin cancer.
Figure 3. Cell viability (mean % cell viability ± SD, n = 3) of 5-fluorouracil (5-FU) in aqueous solution (free 5-FU), control and optimized nanoemulsion (F9) on SK-MEL-5 melanoma cell lines.
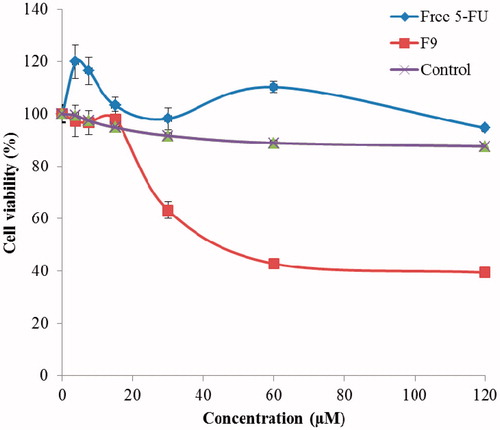
Table 5. Cytotoxicity (%) and IC50 of free 5-FU, optimized nanoemulsion (F9) and control on melanoma cancer cell lines (n = 3).
Conclusion
Based on highest drug permeation, lowest droplet size, lowest polydispersity index, lowest viscosity, lowest concentration of oil phase, optimum surfactant and cosurfactant concentration, nanoemulsion formulation F9 has been optimized as an optimized formulation, containing aqueous phase (including 10 mg of 5-FU; 20% w/w); Transcutol-HP (37.5% w/w), isopropyl alcohol (12.5% w/w) and Lauroglycol-90 (30% w/w). In vitro cytotoxicity studies on melanoma cancer cells indicated that 5-FU in optimized nanoemulsion is much more efficacious than free 5-FU. From these results, it can be concluded that the developed nanoemulsion might be a promising vehicle for chemoprevention of skin cancer. Further preclinical and clinical studies are required to confirm these results.
Acknowledgements
The authors are highly thankful to Gattefosse (France) for donating the gift samples of Lauroglycol-90 and Transcutol-HP.
Declaration of interest
The authors declare no conflict of interest. The authors alone are responsible for content and writing of the article. The research work was kindly funded by National Plan for Science and Technology (Grant No. 11 NAN 1286-02), Riyadh, Saudi Arabia.
References
- Ahmed M, Ramadan W, Rambhu D, Shakeel F. (2008). Potential of nanoemulsions for intravenous delivery of rifampicin. Pharmazie 63:806–11
- Alvi IA, Madan J, Kaushik D, et al. (2011). Comparative study of transferosomes, liposomes, and niosomes for topical delivery of 5-fluorouracil to skin cancer cells: preparation, characterization, in vitro release, and cytotoxicity analysis. Anticancer Drugs 22:774–82
- Ammar HO, Salama HA, Ghorab M, Mahmoud AA. (2009). Nanoemulsion as a potential ophthalmic drug delivery system for dorzolamide hydrochloride. AAPS Pharm Sci Tech 10:808–19
- Bargman H, Hochman J. (2003). Topical treatment of Bowen’s disease with 5-fluorouracil. J Cutan Med Surg 7:101–5
- Chauhan A, Zubair S, Sherwani A, Owais M. (2012). Aloe vera induced bimimetic assembalage of nucleobase into nanosized particles. Plos One 7:E32049
- Cosco D, Paolino D, Muzzalupo R, et al. (2009). Novel PEG-coated nisomes based on bola-surfactant as drug carriers for 5-fluorouracil. Biomed Microd 11:1115–25
- Craig DQM, Barker SA, Banning D. (1995). An investigation into the mechanisms of self-emulsification using particle size analysis and low frequency dielectric spectroscopy. Int J Pharm 114:103–10
- Diasio RB, Johnson MR. (2000). The role of pharmacogenetics and pharmacogenomics in cancer chemotherapy with 5-fluorouracil. Pharmacol 61:199–203
- Elmaghraby GM. (2008). Transdermal delivery of hydrocortisone from eucalyptus oil microemulsion: effects of cosurfactants. Int J Pharm 355:285–92
- Elmeshad AN, Tadros MI. (2011). Transdermal delivery of an anticancer drug via w/o emulsions based on alkyl polyglycosides and lecithin: design, characterization and in vivo evaluation of the possible irritation potential in rats. AAPS Pharm Sci Tech 12:1–9
- Fang JY, Hung CF, Fang YP, Chan TF. (2004). Transdermal iontophoresis of 5-fluorouracil combined with electroporation and laser treatment. Int J Pharm 270:241–9
- Freitas C, Muller RH. (1998). Effect of light and temperature on zeta potential and physical stability in solid lipid nanoparticle (SLN™) dispersions. Int J Pharm 168:221–9
- Gupta RR, Jain SK, Varshney M. (2005). AOT water-in-oil microemulsions as a penetration enhancer in transdermal drug delivery of fluorouracil. Coll Surf B 41:25–32
- Haq N, Shakeel F, Fars FK, et al. (2013). Development and validation of an isocratic, sensitive and facile RP-HPLC method for rapid analysis of 5-fluorouracil and stability studies under various stress conditions. Asian J Chem 25:7177–82
- Hwangl TL, Fang CL, Chen CH, Fang JY. (2009). Permeation enhancer containing water-in-oil nanoemulsions as carriers for intravesical cisplatin delivery. Pharm Res 26:2314–23
- Jain N, Akhtar S, Jain GK, et al. (2011). Antiepileptic intranasal amiloride loaded mucoadhesive nanoemulsion: development safety assessment. J Biomed Nanotechnol 7:142–53
- Khan GM, Hussain A, Hanif RM. (2011). Preparation and evaluation of 5,9 dimethyl-2-cyclopropyl-2-decanol as a penetration enhancer for drugs through rat skin. Pak J Pharm Sci 24:451–7
- Meidan VM, Walmsley AD, Docker MF, Irwin WJ. (1999). Ultrasound-enhanced diffusion into coupling gel during phonophoresis of 5-fluorouracil. Int J Pharm 185:205–13
- Rahman Z, Kohli K, Khar RK, et al. (2006). Characterization of 5-fluorouracil microspheres for colonic delivery. AAPS Pharm Sci Tech 7:E47
- Raza K, Negi P, Takyar S, et al. (2011). Novel dithranol phospholipid microemulsion for topical application: development, characterization and percutaneous absorption studies. J Microencapsul 28:190–9
- Robins P, Gupta AK. (2002). The use of topical fluorouracil to treat actinic keratosis. Cutis 70:4–7
- Shafiq S, Shakeel F, Talegaonkar S, et al. (2007). Development and bioavailability assessment of ramipril nanoemulsion formulation. Eur J Pharm Biopharm 66:227–43
- Shakeel F, Ramadan W, Ahmed MA. (2009). Investigation of true nanoemulsions for transdermal potential of indomethacin: characterization, rheological characteristics and ex vivo skin permeation studies. J Drug Target 17:435–41
- Shakeel F, Ramadan W. (2010). Transdermal delivery of anticancer drug caffeine from water-in-oil nanoemulsions. Coll Surf B 75:356–62
- Shakeel F, Shafiq S, Haq N, et al. (2012). Nanoemulsions as potential vehicles for transdermal and dermal delivery of hydrophobic compounds: an overview. Expert Opin Drug Deliv 9:953–74
- Singh BN, Jayaswal SB. (2008). Iontophoretic delivery of 5-fluorouracil through excised human stratum corneum. Drug Discov Ther 2:128–35
- Singh BN, Singh RB, Singh J. (2005). Effects of ionization and penetration enhancers on the transdermal delivery of 5-fluorouracil through excised human stratum corneum. Int J Pharm 298:98–107
- Spernath A, Aserin A, Ziserman L, et al. (2007). Phosphatidylcholine embedded microemulsions: physical properties and improved Caco-2 cell permeability. J Controlled Release 119:279–90
- Thomas AM, Kapanen AI, Hare JI, et al. (2011). Development of a liposomal nanoparticle formulation of 5-fluorouracil for parenteral administration: formulation design, pharmacokinetics and efficacy. J Controlled Release 150:212–9
- Yassin AEB, Anwer MK, Mowafy HA, et al. (2010). Optimization of 5-fluorouracil solid-lipid nanoparticles: a preliminary study to treat colon cancer. Int J Med Sci 7:398–408
- Zhang Z, Wo Y, Zhang Y, et al. (2012). In vitro study of ethosome penetration in human skin and hypertrophic scar tissue. Nanomed 8:1026–33
- Zhu L, Ma J, Jia N, et al. (2009). Chitosan coated magnetic nanoparticles as carriers of 5-fluorouracil: preparation, characterization and cytotoxicity studies. Coll Surf B 68:1–6