Abstract
Context: As a glucocorticoid drug, dexamethasone has good therapeutic effects for ulcerative colitis. pH-sensitive hydrogels could make conventional changes of volume in response with different pH values. Meanwhile, they could load drugs depending on its internal three-dimensional network structure.
Objective: Appropriate methods were used to improve the drug-loading capacity of hydrogel and exploring the colon-targeting character of dexamethasone hydrogel.
Materials and methods: Different solvents (ethanol and 1,2-propanediol) were employed to dissolve dexamethasone as well as hydrogel monomer materials (poly(ethylene glycol) methyl ether (MPEG)–poly(lactide acid)–acryloyl chloride macromonomer, itaconic acid (IA) and MPEG–methacrylate), then mixing them together to prepare hydrogel through the heat-initiated free radical polymerization method. Differential scanning calorimetry and X-ray diffraction methods were used to verify whether dexamethasone was loaded into hydrogels. In vitro drug release behavior and in vivo pharmacokinetic study were also investigated in detail.
Results: Dexamethasone was successfully loaded into hydrogel, and its loading capacity was improved (5 mg/g). Both the in vitro release study and the in vivo pharmacokinetic study showed the good colon-targeting character of the pH-sensitive P(LE–IA–MEG) hydrogel (Tmax = 1.0 h, Cmax = 2.16 µg/ml of dexamethasone; Tmax = 3.9 h, Cmax = 0.43 µg/ml of dexamethasone hydrogel).
Discussion: Dexamethasone could be targeted to the colon site by P(LE–IA–MEG) hydrogel, thereby improving its therapeutic effect and reduce its side effects.
Conclusion: P(LE–IA–MEG) hydrogel might have great potential application in colon-targeted drug delivery systems.
Introduction
Dexamethasone is a multi-functional drug, which has a great potential application in human disease therapy, especially inflammatory therapy. For example, it could achieve good results in the treatment of ulcerative colitis (Fedorak et al., Citation1995; Buritova et al., Citation1996; Van Meeteren et al., Citation2000; Keely et al., Citation2009; Webber et al., Citation2012). However, due to the hydrophobicity, its in vivo release behavior and function were limited. Moreover, the systemic side effects of dexamethasone, such as adrenocortical hyperactivity syndrome, induced and aggravated infection, osteogenic disorder and digestive reaction, commonly associated with chronic administration are also perennial problems (Friend, Citation2005). Therefore, increasing the concentration of dexamethasone to the target site and decreasing its systemic side effects become the principal purpose.
pH-sensitive hydrogels are three-dimensionally cross-linked hydrophilic polymer networks as well as high polymer drug carriers (Hee Kyung Ju et al., Citation2001; Taşdelen et al., Citation2004; Bajpai & Singh, Citation2006; Xu et al., Citation2006; Singh et al., Citation2007). Due to the presence of chemical or physical cross-linking, they can make conventional change of volume in response with different pH values, namely swelling or deswelling in solvents of different pH values (Gupta et al., Citation2002; Kim & Peppas, Citation2003; Ranjha et al., Citation2008; Nguyen et al., Citation2009; Wang et al., Citation2010a). Therefore, they have more potential applications in drug delivery systems, especially oral drug delivery systems owning to the particularity of the physiological structure of the digestive tract (the pH in the upper gastrointestinal tract is relatively low, while in the lower gastrointestinal tract is higher) (Peppas et al., Citation2004; Sande, Citation2005; Guo & Gao, Citation2007; Wong, Citation2010; Chaturvedi et al., Citation2012; Yang et al., Citation2012).
As drug carriers, it is very important for them to improve the drug-loading capacity and achieve the quantification of drug-loading. For hydrogels, the drug-loading process is usually operated by immersing them into the drug solution (usually aqueous solution) based on their excellent swelling behavior in solution (Cavalieri et al., Citation2008; Yin et al., Citation2008; Cursaru et al., 2013). However, there are certain disadvantages in this method: initially, since hydrophobic drugs were only dissolved in organic solvents (such as dimethyl sulfoxide (DMSO) or DMF), an organic solvent removed process should be operated in order to reduce the toxicity induced by organic solvents and ensure the good biocompatibility of drug-loaded hydrogels. But this process might lead to the great loss of drug. Moreover, some macromolecular drugs cannot enter the cavities of hydrogels, thus making the drug-loading fail. Therefore, the optimization of drug-loading method of hydrogels will directly affect its further application.
Poly(ethylene glycol) methyl ether (MPEG) is a kind of widely used polymer, which reveals excellent compatibility while mixing it with other organic components (Langer & Peppas, Citation2003). Poly(lactide acid) (PLA) based on d, l-lactide can slowly degrade in vivo and in vitro in the absence of enzymes due to its hydrophobicity (Li et al., Citation2005; Sasatsu et al., Citation2005; Habraken et al., Citation2007; Kalarickal et al., Citation2007). Several studies have shown that hydrogels prepared by PLA segment combining with MPEG backbone had good amphiphilicity, thus making them dissolve well in both polar and non-polar solvents. Meanwhile, their biodegradation could also be enhanced (Hu et al., Citation2004). As a kind of unsaturated dicarboxylic acids, the structure of itaconic acid (IA) contains two carboxyl groups, which could significantly improve the pH-responsive ability of the obtained hydrogels synthesized by it (Milašinović et al., 2010). In our previous work, as the main constituent of pH-sensitive hydrogel, methoxyl poly(ethylene glycol)–PLA–acryloyl chloride (AC) amphiphilic macromonomer was successfully synthesized (Wang et al., Citation2012a). As a result, the prepared pH-sensitive hydrogel synthesized by MPEG–PLA–AC macromonomer, IA and MPEG–methacrylate (MA) might have great potential application in oral drug delivery system, especially colon targeted therapy.
In this study, a new kind of oral delivery system based on pH-sensitive P(LE-IA-MEG) hydrogel through heat-initiated free radical polymerization method was successfully prepared. Compared with our previous work, the preparation of hydrogels was optimized by using non-toxic solvent instead of DMSO. The drug-loading process was also improved by using these non-toxic solvents to dissolve drug and hydrogel materials, respectively, to prepare drug-loaded hydrogel. X-ray diffraction (XRD) and differential scanning calorimetry (DSC) methods were used to confirm the drug-loading process. Finally, the in vitro dexamethasone release behavior from P(LE-IA-MEG) and the pharmacokinetic evaluation were studied in detail, respectively.
Materials and methods
Chemicals and reagents
MPEG (Mn = 2000), N,N'-Methylene-bis-acrylamide (BIS), IA, MPEG–MA (MEG, Mn = 300), tin (II) 2-etheylhexanoate Sn(OCt)2, AC (97%), ammonium persulfate (98%) (APS) were all of analytic grade and purchased from Aldrich Company (USA). d, l-Lactide was synthesized in our laboratory. Ethanol and 1,2-propanediol, purchased from Tianjin Fuyu Fine Chemicals and Tianjin Tianli Chemicals (Tianjin, China), respectively, were used without any purification. Dexamethasone was purchased from Whhan Dahua Pharmaceutical Co., Ltd (Wuhan, China). All other reagents were also of analytic grade and used as received.
Male Sprague Dawley rats weighing 200 ± 10 g were used in pharmacokinetic evaluation. The animals were purchased from Laboratory Animal Center of Xi'an Jiaotong University, SCXK (Shaanxi, China) 2007-001. Animals were housed at a controlled temperature of 20–22 °C, relative humidity of 50–60% and 12 h light–dark cycles. Meanwhile, they were allowed to drink water and free access to food. All the animals should be in quarantine for a week before treatment.
Synthesis of MPEG-PLA-AC copolymer
First, MPEG-PLA block polymer was synthesized by ring-opening polymerization (ROP) of D,L-lactide acid initiated by MPEG2000 using tin (II) 2-etheylhexanoate as catalyst. After polymerization, the resultant MPEG-PLA macromonomer was initially precipitated from cold petroleum ether and dried in vacuum at 25 °C, then reacted with AC at 40 °C with a considerable amount of triethylamine, allowing reflux for 4 h. Dichloromethane and cold petroleum ether were used to purify and precipitate the products, respectively. After dried in vacuum at 25 °C, the MPEG-PLA-AC macromonomer was successfully prepared. In the following text, MPEG-PLA-AC macromonomer will be abbreviated as PLE-AC for simplification (Wang et al., Citation2012b).
Preparation of P(LE-IA-MEG) blank hydrogels
The blank P(LE-IA-MEG) hydrogel was synthesized by heat-initiated free radical polymerization with APS as heat-initiator and BIS as cross-linker according to . In our previous work, DMSO was used as the solvent of all materials due to its excellent solubility of organic compounds. However, since DMSO is easily miscible with water in any proportion, it is hard to be removed and has considerable residual. Moreover, DMSO has some blood vessel, liver and kidney toxicities. All these defects limit its further applications.
Scheme 1. Synthesis scheme of pH-sensitive P(LE-IA-MEG) hydrogel. (A) synthesis of MPEG-PLA copolymer; (B) synthesis of PLE-AC copolymer; and (C) synthesis of P(LE-IA-MEG) hydrogel.
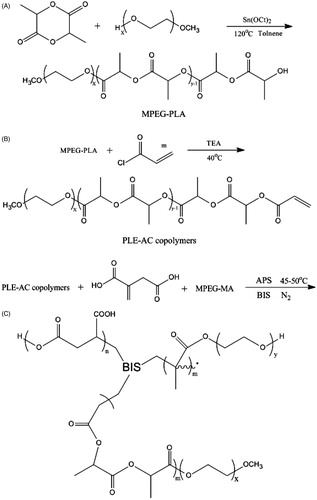
In this study, non-toxic solvents were selected instead of DMSO to prepare hydrogel. Predetermined amounts of the PLE-AC macromonomer, IA, MPEG-MA, BIS and APS (6% w/w of the total monomers) were dissolved in these solvents, then the mixture was poured into a weighting bottle and incubated at 45–50 °C with nitrogen for about 1 h. The just obtained P(LE-IA-MEG) hydrogel was first dried at room temperature for one day, and then dried at 45 °C in vacuum for three days, and they should be kept in airtight bags until use. Deionized water, ethanol and 1,2-propanediol were chosen as non-toxic solvents for hydrogel preparation according to the above method, respectively. Among them ethanol and 1,2-propanediol were also used in the following drug-loading experiments.
Drug loaded into hydrogel
In our previous study, drug was loaded into hydrogels by immersing the blank hydrogel into the drug solution. However, low contents of drug and uneven distribution of drug in the hydrogel limited its further application. In this study, dexamethasone was selected as the model drug, and it was added into the hydrogel during the heat-initiated free radical polymerization process. Ethanol and 1,2-propanediol were chosen as solvents for dexamethasone as well as the material of hydrogel. One gram hydrogel was prepared according to the following method: the predetermined amounts of PLE-AC monomer, IA, MPEG-MA, BIS and APS were added into an EP tube, and the prepared dexamethasone solution mentioned above was added. Afterwards, in order to ensure that the ultimate obtained hydrogel contain the same amount of dexamethasone (5 mg/g), three different composition of dexamethasone solutions were selected to prepare hydrogels: 2 ml solution a, b and c were added into each EP tube, respectively (the composition of each solution is listed in ). After shaken to form a homogeneous mixed solution, these mixtures were incubated for 1 h at 45–50 °C. The just obtained drug-loaded hydrogel was initially dried at room temperature for one day, then dried at 45 °C in vacuum for three days. The dried drug-loaded hydrogel was cut into less than 4-mm square pieces and stored at 4 °C before use.
Table 1. Dexamethasone solution prepared by ethanol, 1,2-propanediol and ethanol–water mixed solution, respectively.
XRD spectrometry
XRD spectrometry of blank hydrogel, dexamethasone hydrogel and dexamethasone was obtained by using X-ray diffractometer (DX-2000, Dandong Fangyuan Instrument Company, Liaoning, China) using CuKα radiation.
Differential scanning calorimetry
The difference of thermal properties between blank hydrogel and drug-loaded hydrogel was characterized by using a differential scanning calorimeter (DSC822e, METTLER TOLEDO, Columbus, OH). Specimens of blank hydrogel, dexamethasone hydrogel and dexamethasone powder were first heated from 30 °C to 350 °C under nitrogen atmosphere at a heating rate of 10 °C/min, then cooled to 30 °C at the same rate.
In vitro drug-release studies
To simulate the process of gastrointestinal transit, the in vitro release study was carried out according to the following method: initially, 0.1 g drug-loaded hydrogels, which were prepared in different solutions (solution a, b and c), were immersed in artificial gastric juice (pH = 1.2), and the operation was carried out under the condition of 37 ± 0.2 °C and 100 rpm for 2 h. At appropriate 1 h time interval, all the release media was withdrawn, and an equal volume of medium was added to maintain the volume constant. Afterwards, the drug-loaded hydrogels were transferred into pH 6.8 artificial small intestinal juice, and samples were collected again at 1 h time interval till 6 h. Finally, the drug-loaded hydrogels were transferred into pH 7.4 artificial colon juice until 10 h. After centrifuged at 12 000 rpm for 5 min, the supernatants of the removed release media were collected and stored at − 20 °C before analysis. HPLC method was chosen to determine the concentration of dexamethasone. The cumulative release of dexamethasone was calculated according to the following equation (Wang et al., Citation2010b):
where Q was cumulative release weight, and Cn was the dexamethasone concentration at time t. Vt was the volume of medium (Vt = 5 ml), and Vs was the volume of solution taken from each supernatant (Vs = 1 ml).
Pharmacokinetic analysis
Owning to its excellent pH-sensitivity, the good targeting and sustained-release characteristics of hydrogel might make drug concentrated in the targeting organ and thus improve its bioavailability. In this study, rats were orally administered with dexamethasone or dexamethasone hydrogel. The pharmacokinetic characteristics of dexamethasone in different forms were evaluated through the variation of rats' drug concentration.
Drug administration and sampling
For the pharmacokinetic study, rats were randomly divided into two groups (six animals each): control group and experimental group were i.g. administrated with dexamethasone and dexamethasone hydrogel at a single dose of 5 µmol/kg, respectively. Blood samples were collected through jugular veins at 0.25, 0.5, 1, 1.5, 2, 3, 4, 6, 9 and 12 h post-dosing. Each blood sample was instantly centrifuged at approximately 4000 rpm for 10 min followed by 100 µl of supernatant plasma collected and stored at − 20 °C until analysis.
Sample processing
Initially, 400 µl of methanol was added into the obtained rat plasma sample. Then the mixture was vigorously stirred for 5 min followed by centrifugation at 12 000 rpm for 5 min to precipitate proteins; 400 µl of upper organic phase was transferred to another tube and evaporated to dryness at 40 °C with nitrogen. The resulting extract was dissolved in 150 µl of methanol and mixed by vortex. Finally, 100 µl of supernatant was taken for HPLC analysis after centrifugation at 12 000 rpm for 5 min.
Methodological study of HPLC
The linearity of the HPLC method for the determination of dexamethasone in rat blood was evaluated by a calibration curve, which was obtained by plotting the peak area of each analyte versus dexamethasone concentration. The final concentrations of dexamethasone were 0.05, 0.1, 0.5, 1, 5, 10 and 20 µg/ml. The chromatographic column was C18 BDS Hypersil, and the composition of mobile phase was acetonitrile/water (35/65). Operation was conducted under the condition of column temperature 30 °C and flow rate 1 ml/min. The detection wavelength was 240 nm using a UV detector, and the sample injection volume was 100 µl.
In this study, the absolute recoveries of dexamethasone from rat blood were determined at different standard concentrations (0.5, 5 and 20 µg/ml) by spiking the corresponding fresh blank plasma. The percentages of recovery were calculated by comparing the peak area of extracted samples with samples in which the same amount of compounds were diluted with mobile phase and injected directly. The recoveries at three standard concentration levels of dexamethasone in blood were examined at least five times. For the evaluation of precision, these extracted samples of three different concentrations (0.5, 5 and 20 µg/ml) were processed and injected five times within a day (intra-day) and within five days (inter-day) for calculating RSD, respectively.
Statistical analysis
All the statistical data were expressed as the means with 95% confidence intervals and represented as mean ± SD. In vitro release curves were compared and analyzed using the analysis of variance test. Plasma concentration curves were performed with Student's t test. For all the tests, p values less than 0.05 were considered to be statistically significant. All statistical tests were two-sided.
Results
Preparation of P(LE-IA-MEG) hydrogels and drug-loading
In this study, XRD and DSC were used to explore whether dexamethasone was successfully loaded into hydrogel. was the X-ray powder diffraction pattern of blank hydrogel, dexamethasone and dexamethasone hydrogel. As presented in , there were two reflection peaks in the XRD spectra of blank hydrogel. One was at 16.7146 and another relatively weak one was at 19.1353. In dexamethasone XRD spectra, there were a series of main reflection peaks appeared between 13.4767 and 21.5138. Compared with the former two XRD spectra, spectra of drug-loaded hydrogel was more similar as blank hydrogel. Its reflection peaks appeared at 16.6936 and 18.9879.
Figure 1. X-ray powder diffraction pattern of (a) blank hydrogel; (b) dexamethasone; and (c) dexamethasone hydrogel.
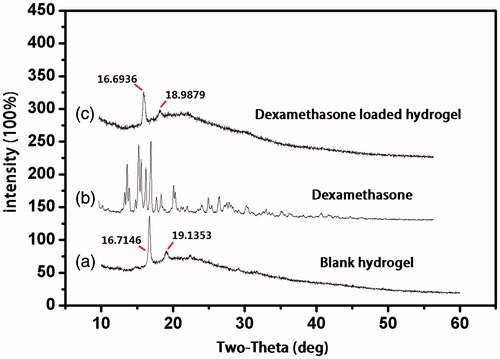
The drug-loaded condition of hydrogel could also be investigated through the thermal property. shows the DSC measurements of the thermotropic behavior of blank hydrogel, dexamethasone and dexamethasone hydrogel. As shown in , blank hydrogel served as the control, and its exothermic peak maxima appeared at 192.52 °C. Compared to the control, thermogram with a certain extent decreasing of dexamethasone hydrogel was 175.43 °C and the exothermic peak maxima of dexamethasone appeared at 253.89 °C. It was suggested that after loaded into hydrogel, the exothermic peak of dexamethasone was disappeared. Simultaneously, the position of hydrogel exothermic peak was also moved. Both results of XRD and DSC suggested that dexamethasone had been wrapped in the cavities of the hydrogel.
In vitro drug-release studies
The release of dexamethasone from P(LE-IA-MEG) hydrogel at different pH (pH 1.2, 6.8 and 7.4) was performed, and its cumulative release profile is displayed in . From , we could find that the pH value had great effect on the release behavior of dexamethasone from these hydrogels.
Figure 3. In vitro release behavior of dexamethasone from dexamethasone P(LE-IA-MEG) hydrogel samples prepared by 1,2-propanediol, ethanol-water mixed solution and ethanol, respectively. At pH 1.2 (from 0 to 2 h), pH 6.8 (from 3 to 6 h) and pH 7.4 (from 7 to 10 h).
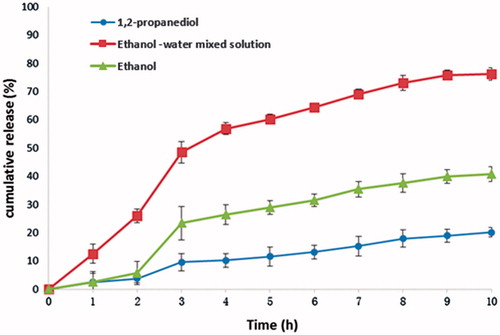
Obviously, the release rates of the drug-loaded hydrogels prepared by three solvents respectively were all strongly influenced by pH value. And when pH changed, the release rates changed significantly. For example, when pH changed from 1.2 to 6.8 (from the second to the third hour), the release rates increased dramatically. Among them, the release rate of the hydrogel prepared by solution a, b and c increased from 5.8% to 23.4%, from 3.8% to 9.6% and from 25.9% to 48.5%, respectively. However, when pH changed from 6.8 to 7.4 (from the sixth to the seventh hour), the three release rates slightly changed (release rates of drug-loaded hydrogel prepared by solution a, b and c increased from 31.4% to 35.4%, from 13.1% to 15.3% and from 64.5% to 69.1%, respectively). The ultimate cumulative release rates in 10 h were 40.7%, 20.1% and 77.2%, respectively. was the swelling condition of three kinds of drug-loaded hydrogels at 2 h (pH 1.2), 4 h (pH 6.8) and 10 h (pH 7.4), respectively. From , it could be concluded that with the increasing pH value, the drug-loaded hydrogel swelled significantly. Furthermore, the swelling degree of hydrogel was proportional to the release rate of dexamethasone, indicating that the expansion of the hydrogel cavities caused by swelling made dexamethasone release faster.
Figure 4. Schematic illustration of the swelling condition of dexamethasone hydrogel in dissolution medium of different pH values (a) pH 1.2 artificial gastric juice at 2 h, (b) pH 6.8 artificial small intestinal juice at 4 h, (c) pH 7.4 artificial colonic juice at 10 h; the dexamethasone hydrogels were prepared in ethanol, 1,2-propanediol and the ethanol–water mixed solution from left to right).
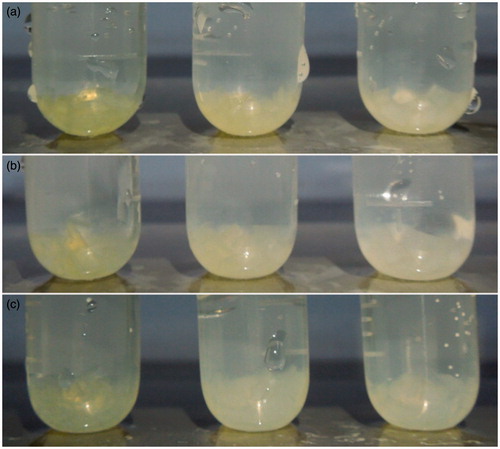
Among the three release curves, the drug-loaded hydrogel prepared by solution c (20% ethanol and 80% water) got the highest release rate. However, it released too fast (25.9% within 2 h) at the beginning of the whole period. The release rate of the drug-loaded hydrogel prepared by solution b was considerably lower than that of solution c. The probable reason might be that 1,2-propanediol has a relative high boiling point so that during the drying process it was hard to evaporate. Thus 1,2-propanediol might remain in the hydrogel that causing dexamethasone dissolved in 1,2-propanediol and hardly released. Compared with the former two solvents, the drug-loaded hydrogel prepared by solution a got the best pH sensitivity; consequently, it could achieve relatively low release rate at low pH (pH 1.2). However, its ultimately cumulative release rate was still relatively low. Based on the careful comparison of release profiles between the two hydrogels prepared form solution a and c, the mixed solvent of water and ethanol could be taken into consideration to prepare drug-loaded hydrogel in order to improve release rate as well as maintain higher pH sensitivity.
was the cumulative release profile of four kinds of hydrogel samples prepared in ethanol–water mixed solution in different ratios. The four samples were displayed in ; and the hydrogel drug-loading and releasing methods were the same as given in sections “Drug loaded into hydrogel” and “In vitro drug release studies”. shows, as the proportion of ethanol was increased, the release rates gradually decreased. This might be due to the residual ethanol in the hydrogel, which made hydrophobic drug difficult to diffuse into the dissolution medium. Among them, sample 2 not only released less at low pH value (pH 1.2) but also ultimately reached considerable release rate. Therefore, sample 2 was chosen for the following pharmacokinetic evaluation.
Figure 5. In vitro release behavior of dexamethasone from dexamethasone P(LE-IA-MEG) hydrogel samples prepared in four kinds of ethanol–water mixed solution in different ratios, respectively. At pH 1.2 (from 0 to 2 h), pH 6.8 (from 3 to 6 h) and pH 7.4 (from 7 to 10 h).
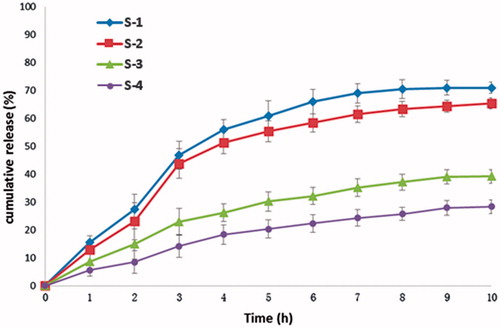
Table 2. Four kinds of ethanol–deionized water mixed solution of dexamethasone.
Pharmacokinetic study
A linear relationship within the range of 0.05–20 µg/ml was found between peak areas of dexamethasone in blood and theoretical concentrations. And the regression equation of calibration curves was A = 206390c + 3412.6, r = 0.9996 (n = 6). The lowest detectable concentration of dexamethasone in blood was 0.05 µg/ml. For recovery detection, the absolute recoveries of dexamethasone in blood ranged from 85.3% to 90.8% (), and no obvious difference occurred among the three concentration (0.5, 5, and 20 µg/ml). For precision test, the intra- and inter-day RSD of blood assay were less than 15% in three different concentrations (). These results indicated that the reproducibility and recovery were acceptable over the studied concentration range.
Table 3. Recovery and precision for dexamethasone determination in rats' plasma by HPLC.
The plasma concentration-time profiles of dexamethasone and dexamethasone hydrogel after oral administration are shown in . The corresponding pharmacokinetic parameters are summarized in . The single oral dose was 5 µmol dexamethasone per kg. After oral administration of dexamethasone, the peak blood concentrations (Cmax = 1.0 µg/ml) of dexamethasone appeared at 2.16 h post-dose. The average residence time MRT0–12 was 3.27 h and AUC0–12 was 8.29 µg h/ml. Nevertheless, when orally taking dexamethasone hydrogel, the variation of blood concentrations of rats was stable and no sharp changes occurred. The peak blood concentrations (Cmax = 0.43 µg/ml) of dexamethasone appeared at 4 h post-dose. The MRT0–12 was 5.49 h and AUC0–12 was 3.70 µg h/ml. The Cmax of the oral dexamethasone hydrogel group was far lower than that of the oral dexamethasone group. The AUC0–12 of the experimental group was far lower than that of the control group, and the former was 0.44 times of the latter. Compared with the group which was directly administrated with dexamethasone, the elimination speed of the dexamethasone hydrogel group was relatively slow (its MRT0–12 and MRT0–∞ were both greater than that of the dexamethasone group), indicating that through the variation of pH value of the digestive tract, dexamethasone could be targeted to the colon site by hydrogel and stay longer, thereby enhancing its efficacy.
Figure 6. Plasma concentration curves of dexamethasone (i.g. administration of 5 µmol/kg) and dexamethasone hydrogel (i.g. administration of hydrogel containing 5μmol/kg dexamethasone) in rats (data are mean ± SD, n = 6).
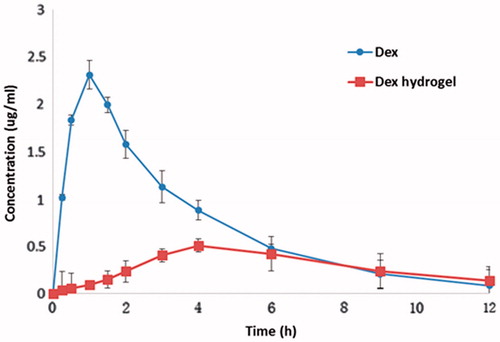
Table 4. Pharmacokinetic parameter of free dexamethasone and dexamethasone hydrogel in rats' blood after i.g. administration of dexamethasone (5 µmol/kg) and dexamethasone hydrogel containing 5 µmol/kg dexamethasone (data are mean ± SD, n = 6).
Discussion
In recent years, pH-sensitive hydrogels have been paid more attention in the field of drug delivery systems due to their special properties. Among them, anionic hydrogels, which do not swell in acidic medium but swell significantly in alkaline medium were suitable for oral drug delivery systems. In this article, through ROP, MPEG and PLA segments were combined to form MPEG-PLA block polymer. To improve the unsatisfied pH-sensitive characteristics, the P(LE-IA-MEG) hydrogel was successfully synthesized via introducing IA with two carboxyl groups, which might greatly enhance its pH-sensitivity (Teijon et al., Citation2009; Milasinovic et al., Citation2012; Milosavljević et al., 2011).
Moreover, in order to reduce the system toxicity of hydrogel, the solvent DMSO, which was once used to dissolve the materials of hydrogel, was replaced by non-toxic solvents. Meanwhile, the obtained hydrogel was no longer immersed in water for a long time to remove DMSO, and it also did not need to dry for too long, avoiding the acceleration of drug exudation to ensure the precise amount of drug. In addition, this new preparation method could also avoid the toxic solvent (DMSO) and enhance the biocompatibility of pH-sensitive P(LE-IA-MEG) hydrogel.
As a hydrophobic drug, dexamethasone gets considerably low solubility in water, thus making it very difficult to be absorbed and limiting its function. Therefore, choosing suitable solvents to dissolve dexamethasone and loading it into an appropriate carrier could improve its concentration, thereby enhancing its efficacy. In this study, ethanol and 1,2-propanediol were chosen to dissolve dexamethasone. Furthermore, these two organic solvents and water were employed to dissolve the composition materials of hydrogel, respectively. Then the drug solutions and material solutions were mixed together. After shaken to form a homogeneous solution and heated, the drug-loaded hydrogel was obtained.
The result of XRD showed that after loaded into hydrogel, the reflection peak of dexamethasone was disappeared. And the positions of two main reflection peaks were almost the same as blank hydrogel, indicating that dexamethasone had been wrapped into the hydrogel instead of attaching to the hydrogel surface or leaking from the cavity of hydrogel. As only dexamethasone was wrapped into the cavities of hydrogel, its XRD peaks could be shielded. Therefore, only hydrogel reflection peaks could be observed but dexamethasone reflection peaks could not.
From the result of DSC, we could also infer that once attached to the surface of hydrogel or leaked out from hydrogel, exothermic peak of dexamethasone could be detected. Contrarily, if dexamethasone could not be totally loaded into the hydrogel, there might be two exothermic peaks representing hydrogel and dexamethasone, respectively. Furthermore, their position must be the same as when they were tested individually. However, dexamethasone exothermic peak did not appear. At the same time, hydrogel exothermic peak also varied, indicating that dexamethasone had been successfully loaded into hydrogel, and dexamethasone had a certain influence on hydrogel.
Both the results of in vitro release study and in vivo pharmacokinetic study indicated the excellent pH-sensitive and good colon-targeting character of P(LE-IA-MEG) hydrogel. From the in vitro release study, we could infer that the composition of the solvent was extremely important for drug releasing. The suitable solvent not only dissolves the drug and hydrogel monomer materials but also facilitates the preparation of hydrogels. In this study, dexamethasone was a hydrophobic drug and 1,2-propanediol was a less polar organic solvent, which had a higher boiling point and was difficult to dry. This property made dexamethasone easy to dissolve in it and hardly to release, thus making the hydrogel prepared by 1,2-propanediol get lower release rate. Although alcohol was also organic solvent, its boiling point was relatively low and was easy to dry, thus making the drug-loaded hydrogel prepared by alcohol get higher release rate than hydrogel prepared by 1,2-propanediol. Furthermore, changing the ratio of ethanol and water could significantly increase the release rate, indicating that with the increase of water proportion, fewer residual ethanol remained in the dried hydrogel, following fewer drug dissolved in ethanol and making release rate increase.
The result of in vivo pharmacokinetic study suggested that dexamethasone was quickly hydrolyzed and absorbed in the acidic stomach environment, so that it could reach the peak concentration within a short time. To the contrary, before reaching the peak concentration, the plasma concentration of rats orally administered with dexamethasone hydrogel rose slowly, indicating that in the lower pH environment of stomach, the carboxylic acid groups of the dexamethasone hydrogel are in the form of COOH and large amounts of hydrogen bonds formed by IA chain corresponding with PEG chain. As a result, the hydrogel swelled slowly and released a small amount of drug. When hydrogel reached the higher pH colon environment, the hydrogen bond broke and carboxylic acid groups become ionized. Meanwhile, electrostatic repulsion caused the network to expand (). Therefore, the hydrogel swelling speed accelerated rapidly, and the drug release also increased rapidly. Therefore, dexamethasone wrapped by hydrogel could greatly reduce the degree of dexamethasone into blood. Consequently, more drug could be released at the target site to play a good therapeutic effect (the in vivo transportation of dexamethasone hydrogel was shown in ).
Conclusions
In this work, the MPEG-PLA-AC copolymers were successfully synthesized by solvent polymerization, and the P(LE-IA-MEG) hydrogel via heat-initiated free radical polymerization method was successfully prepared. Simultaneously, the preparation method was also improved by using non-toxic solvents instead of DMSO. The drug-loading method was also optimized via combining drug solution and hydrogel solution together to prepare drug-loaded hydrogel. DSC and XRD methods were employed to characterize the condition of drug-loading. Through the in vitro drug release experiment, the optimal ratio of solvent was explored. The pharmacokinetic study was also conducted in detail. All the results showed that dexamethasone loaded in hydrogel could release more at the target site to improve the bioavailability of dexamethasone. These data indicate that P(LE-IAMEG) hydrogel was based on MPEG-PLA-AC copolymers and might have great potential application in drug delivery system.
Acknowledgements
Ethical approval: the study protocol for the care and use of laboratory animals have been observed and approved by the Xi’an Jiaotong University Medical and Biological Research Ethics Committee.
This work was financially supported by Fundamental Research Funds for the Central Universities (xjj2013054 and xj08142016) and National Natural Science Foundation of China (No. 30973578 and No. 30973673).
Declaration of interest
The authors report no conflicts of interest.
References
- Bajpai SK, Singh S. (2006). Analysis of swelling behavior of poly(methacrylamide-co-methacrylic acid) hydrogels and effect of synthesis conditions on water uptake. React Funct Polym 66:431–40
- Buritova J, Honor P, Chapman V, Besson J-M. (1996). Enhanced effects of co-administered dexamethasone and diclofenac on inflammatory pain processing and associated spinal c-Fos expression in the rat. Pain 64:559–68
- Cavalieri F, Chiessi E, Villa R, et al. (2008). Novel PVA-based hydrogel microparticles for doxorubicin delivery. Biomacromolecules 9:1967–73
- Chaturvedi K, Ganguly K, Nadagouda MN, Aminabhavi TM. (2012). Polymeric hydrogels for oral insulin delivery. J Control Release 165:129–38
- Cursaru B, Teodorescu M, Boscornea C, et al. (2013). Drug absorption and release properties of crosslinked hydrogels based on diepoxy-terminated poly(ethylene glycol)s and aliphatic polyamines – a study on the effect of the gel molecular structure. Mater Sci Eng C 33:1307–14
- Fedorak RN, Haeberlin B, Empey LR, et al. (1995). Colonic delivery of dexamethasone from a prodrug accelerates healing of colitis in rats without adrenal suppression. Gastroenterology 108:1688–99
- Friend DR. (2005). New oral delivery systems for treatment of inflammatory bowel disease. Adv Drug Deliv Rev 57:247–65
- Guo BL, & Gao QY. (2007). Preparation and properties of a pH/temperature-responsive carboxymethyl chitosan/poly(N-isopropylacrylamide)semi-IPN hydrogel for oral delivery of drugs. Carbohydr Res 342:2416–22
- Gupta P, Vermani K, & Garg S. (2002). Hydrogels: from controlled release to pH-responsive drug delivery. Drug Discov Today 7:569–79
- Habraken W, Wolke J, & Jansen J. (2007). Ceramic composites as matrices and scaffolds for drug delivery in tissue engineering. Adv Drug Deliv Rev 59:234–48
- Hee Kyung JU, So Yeon Kim, & Lee, YM. (2001). pH/temperature-responsive behaviors of semi-IPN and comb-type graft hydrogels composed of alginate and poly(N-isopropylacrylamide). Polymer 42:6851–7, 7
- Hu Y, Zhang L, Cao Y, et al. (2004). Degradation behavior of poly (ε-caprolactone)-b-poly (ethylene glycol)-b-poly (ε-caprolactone) micelles in aqueous solution. Biomacromolecules 5:1756–62
- Kalarickal NC, Rimmer S, Sarker P, Leroux J-C. (2007). Thiol-functionalized poly (ethylene glycol)-b-polyesters: synthesis and characterization. Macromolecules 40:1874–80
- Keely S, Ryan SM, Haddleton DM, et al. (2009). Dexamethasone–pDMAEMA polymeric conjugates reduce inflammatory biomarkers in human intestinal epithelial monolayers. J Control Rel 135:35–43
- Kim B, & Peppas NA. (2003). In vitro release behavior and stability of insulin in complexation hydrogels as oral drug delivery carriers. Int J Pharm 266:29–37
- Langer R, & Peppas NA. (2003). Advances in biomaterials, drug delivery, and bionanotechnology. AIChE J 49:2990–3006
- Li F, Li S, & Vert M. (2005). Synthesis and rheological properties of polylactide/poly (ethylene glycol) multiblock copolymers. Macromol Biosci 5:1125–31
- Milasinovic N, Knezevic-Jugovic Z, Milosavljevic N, et al. (2012). Controlled release of lipase from Candida rugosa loaded into hydrogels of N-isopropylacrylamide and itaconic acid. Int J Pharm 436:332–40
- Milašinović N, Kalagasidis Krušić M, Knežević-Jugović Z, et al. (2010). Hydrogels of N-isopropylacrylamide copolymers with controlled release of a model protein. Int J of Pharm 383:53–61
- Milosavljević NB, Ristić MĐ, Perić-Grujić AA, et al. (2011). Sorption of zinc by novel pH-sensitive hydrogels based on chitosan, itaconic acid and methacrylic acid. J Hazard Mater 192:846–54
- Nguyen MK, Huynh CT, & Lee DS. (2009). pH-sensitive and bioadhesive poly(β-amino ester)–poly(ethylene glycol)–poly(β-amino ester) triblock copolymer hydrogels with potential for drug delivery in oral mucosal surfaces. Polymer 50:5205–10
- Peppas NA, Wood KM, & Blanchette JO. (2004). Hydrogels for oral delivery of therapeutic proteins. Expert Opin Biol Ther 4:881–7
- Ranjha NM, Mudassir J, & Akhtar N. (2008). Methyl methacrylate-co-itaconic acid (MMA-co-IA) hydrogels for controlled drug delivery. J Solgel Sci Technol 47:23–30
- Sande SA. (2005). Pectin-based oral drug delivery to the colon. Expert Opin Drug Deliv 2:441–50
- Sasatsu M, Onishi H, & Machida Y. (2005). Preparation of a PLA–PEG block copolymer using a PLA derivative with a formyl terminal group and its application to nanoparticulate formulation. Int J Pharm 294:233–45
- Singh B, Chauhan GS, Kumar S, & Chauhan N. (2007). Synthesis, characterization and swelling responses of pH sensitive psyllium and polyacrylamide based hydrogels for the use in drug delivery (I). Carbohydr Polym 67:190–200
- Taşdelen B, Kayaman-Apohan N, G Ven O, & Baysal BM. (2004). pH-thermoreversible hydrogels. I. Synthesis and characterization of poly(N-isopropylacrylamide/maleic acid) copolymeric hydrogels. Radiat Phys Chem 69:303–10
- Teijon C, Guerrero S, Olmo R, et al. (2009). Swelling properties of copolymeric hydrogels of poly(ethylene glycol) monomethacrylate and monoesters of itaconic acid for use in drug delivery. J Biomed Mater Res B Appl Biomater 91:716–26
- Van Meeteren M, Meijssen M, & Zijlstra F. (2000). The effect of dexamethasone treatment on murine colitis. Scand J Gastroenterol 35:517–21
- Wang K, Fu Q, Chen X, et al. (2012a). Preparation and characterization of pH-sensitive hydrogel for drug delivery system. RSC Adv 2:7772–80
- Wang K, Li WF, Xing JF, et al. (2012b). Preliminary assessment of the safety evaluation of novel pH-sensitive hydrogel. Eur J Pharm Biopharm 82:332–9
- Wang K, Xu X, Liu T, et al. (2010a). Synthesis and characterization of biodegradable pH-sensitive hydrogel based on poly(ε-caprolactone), methacrylic acid, and pluronic (L35). Carbohydr Polym 79:755–61
- Wang K, Xu X, Wang Y, et al. (2010b). Synthesis and characterization of poly(methoxyl ethylene glycol-caprolactone-co-methacrylic acid-co-poly(ethylene glycol) methyl ether methacrylate) pH-sensitive hydrogel for delivery of dexamethasone. Int J Pharm 389:130–8
- Webber MJ, Matson JB, Tamboli VK, & Stupp SI. (2012). Controlled release of dexamethasone from peptide nanofiber gels to modulate inflammatory response. Biomaterials 33:6823–32
- Wong TW. (2010). Design of oral insulin delivery systems. J Drug Target 18:79–92
- Xu FJ, Kang ET, & Neoh KG. (2006). pH- and temperature-responsive hydrogels from crosslinked triblock copolymers prepared via consecutive atom transfer radical polymerizations. Biomaterials 27:2787–97
- Yang J, Chen J, Pan D, et al. (2012). pH-sensitive interpenetrating network hydrogels based on chitosan derivatives and alginate for oral drug delivery. Carbohydr polym 92:719–25
- Yin L, Ding J, Fei L, et al. (2008). Beneficial properties for insulin absorption using superporous hydrogel containing interpenetrating polymer network as oral delivery vehicles. Int J Pharm 350:220–9