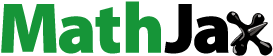
Abstract
In order to develop transdermal drug delivery system that facilitates the skin permeation of Pioglitazone (PZ) encapsulated in carbopol-based transgel system (proniosomes/niosome). The developed formulations were optimized using quality by design (QbD) approach and particle size, percentage entrapment and transdermal flux were determined. It was found to be more efficient delivery carriers with high encapsulation and enhanced flux value demonstrated that the permeation of PZ through skin was significantly increased with developed formulation. The transdermal enhancement from proniosome was 3.16 times higher than that of PZ from control formulation (ethanol buffer formulation, 3:7), which was further confirmed by confocal laser scanning microscopy. In vivo pharmacokinetic study of carbopol transgel showed a significant increase in bioavailability (2.26 times) compared with tablet formulation. It also showed better antidiabetic activity in comparison to marketed tablet, so our results suggest that carbopol-based transgel are an efficient carrier for delivery of pioglitazone through skin.
Introduction
The transdermal delivery system offers several distinct advantages over other delivery systems, including avoidance of gastrointestinal side effects of drugs, enzymatic activity and drug–food interactions and bypass first-pass effect. It allows rapid termination of drug effects when necessary (El-Kattan et al., Citation2000; Naik et al., Citation2000). Carbopols are synthetic polymers of acrylic acid cross-linked with various chemicals such as divinyl-glycol, allyl-sucrose and polyalkenyl polyether (Chawla & Saraf, Citation2012). It is soluble in polar solvents and upon salvation, they swells, and in presence of a neutralizing agent forming a microgel system (Garcia-Gonzalez et al., Citation1994; Weber et al., Citation2012). These properties make them potential candidates for the use as transdermal gel formulations (Jana et al., Citation2014).
Proniosomes are solid formulation prepared using non-ionic surfactants which can be converted into niosomes upon hydration (Fang et al., Citation2001; Varshosaz et al., Citation2005). It can encapsulate both lipophilic and hydrophilic drugs and protects them against in vivo acidic and enzymatic effects (Mokhtar et al., Citation2008). Niosomes are able to prolong the circulation of encapsulated drug altering its organ distribution and metabolic stability (Rogerson et al., Citation1988). Niosomes have been reported to enhance the residence time of drugs in the stratum corneum and epidermis, while reducing the systemic absorption of the drug and improve penetration of the trapped substances across the skin (Balakrishnan et al., Citation2009). Moreover, it has been reported in several studies, that compared to conventional dosage forms, lipid vesicular formulations exhibited an enhanced drug bioavailability (Manconi et al., Citation2006; Mura et al., Citation2007; Shumilov & Touitou, Citation2010; Imam et al., Citation2013; Vitorino et al., Citation2013). Particular efforts have been aimed at using niosomes as effective dermal and transdermal drug delivery systems (Aboelwafa et al., Citation2010; El-Laithy et al., Citation2011; Muzzalupo et al., Citation2011; Junyaprasert et al., Citation2012; Imam et al., Citation2013). Pioglitazone (PZ) is a thiazolidinedione derivative with structural formula 5-[4-[2-(5-Ethyl-2-pyridinyl) ethoxy] benzyl] thiazolidine-2,4-dione (Reginato & Lazar, Citation1999; Waugh et al., Citation2006). It is highly bound to plasma proteins to serum albumin and metabolized by CYP3A4 and CYP2C8/9. Due to poor aqueous solubility, it shows delayed onset of action because of sub-therapeutic plasma drug levels; may also lead to therapeutic failure (Elbary et al., Citation2008). It acts as agonist of the peroxisome proliferator-activated receptor subtype gamma in type II diabetes (Reginato & Lazar, Citation1999; Waugh et al., Citation2006). PZ is a water insoluble drug and having short biological half-life of 3–5 h and is eliminated rapidly (Tripathi, Citation2003). The aim of this study is to investigate the feasibility of carbopol-based transgel as drug carrier systems for pioglitazone. The optimization of proniosomal formulation was explored using three-factor three-level using Box–Behnken factorial design. The influence of different formulation variables such as tween80, phospholipidG90 and cholesterol were investigated on the size, entrapment efficiency and transdermal flux. The potential of optimized transgel systems of PZ was investigated by in vitro release, skin irritation, in vivo pharmacokinetic and antidiabetic studies.
Experimental
Materials
Pioglitazone and Phospholipon 90G were received as a gratis sample from Ranbaxy Research Laboratories Ltd. (Gurgaon, India) and Phospholipid GmbH (Nattermannallee, Germany). Carbopol 940 was purchased from S. D. Fine Chemicals Ltd., Mumbai, India. Cholesterol and tween80 were purchased from Spectrochem Pvt. Ltd (Mumbai, India). All other chemicals used were of analytical grade. Double-distilled water was used for all experiments.
Preparation of transdermal proniosomes
PZ proniosomes were prepared by method reported using three-factor three-level Box–Behnken design (Balakrishnan et al., Citation2009). The concentration range of independent variables under study with their low, medium and high levels, which were selected based on the results from preliminary experimentation. The design showed a total of 15 formulations as per experimental design with their effect on the size, entrapment efficiency and transdermal flux are presented in . Proniosome-derived niosomes dispersions were obtained by hydrating film with phosphate buffer saline pH 7.4. Similarly, rhodamine red (RR, 0.03% w/v) loaded formulation was prepared for confocal laser microscopy. A fixed dose of the PZ (40 mg) was used in all formulations. Ethanol:buffer formulation (3:7) of same dose has been used as control formulation in the study.
Table 1. Observed and predicted response in the Box–Behnken design for pioglitazone proniosomal formulation.
Experimental design
The application of design of experiments such as Box–Behnken design, central-composite design, factorial design, D-optimal design and mixture design used for systematic optimization of drug delivery systems (Hwang & Kowalski Citation2005; Singh et al., Citation2005). BBD is a most widely acceptable approach for optimization of formulation to obtain robust formulations. It gives fewer experimental runs over others due to ease of design execution and interpretation (Chaudhary et al., Citation2011; Beg et al., Citation2012; Ahad et al., Citation2013). Response surface analyses were carried out to identify the effect of different independent variables on the observed responses. A three-factor three-level Box–Behnken design was used to explore the quadratic response surfaces and for constructing a second-order polynomial models using DESIGN EXPERT Software, Version 8.0.7.1 (Stat-Ease, Minneapolis, MN) and fitted into a multiple linear regression model. The nonlinear polynomial quadratic model is defined as:
where Y is the measured response associated with each factor level combination; β0 is constant; β1, β2, β3 are linear coefficients, β12, β13, β23 are interaction coefficients between the three factors, β11, β22, β33 are quadratic coefficients computed from the observed experimental values of Y from experimental runs and A, B and C are the coded levels [low (−), medium (0) and high (+)] of independent variables. The terms AB and A2 (i = 1, 2 or 3) represent the interaction and quadratic terms, respectively. The Tween80 (A), phospholipid (B) and cholesterol (C) were selected as independent variables, whereas dependent variables were size (Y1), % EE (Y2) and flux (Y3), with constraints applied in the formulation. The responses were statistically evaluated using the ANOVA procedure. Further, the optimum formulation was selected by a numerical optimization procedure using desirability function.
Liquid chromatography
PZ content in the samples was quantitated by reversed phase RP-HPLC compact LC system (Agilent Technologies 1120) using Hypersil ODS C-18 column (250 × 4.6 mm, 5 μm particle size). The detection of PZ was performed at 270 nm. The mobile phase used in this study was acetonitrile: ammonium acetate buffer (pH 5) in the ratio of 60:40 v/v. The mobile phase was pumped from the solvent reservoir to the column at a flow rate of 1.0 mL/min and the volume of analyte sample injected was 20 μL.
Quality evaluation of proniosome
Size and size distribution
The size and size distribution were determined by dynamic light scattering method, using a computerized Malvern system (Zetasizer, HAS 3000; Malvern, United Kingdom). The formulation was taken in the glass tube was diluted with appropriate medium to determine the vesicle size. The values of mean particle size and PDI of formulated niosomes were measured.
Vesicle morphology
The morphology of the prepared proniosome formulations was determined by transmission electron microscopy (TEM Morgani 268D, Eindhoven, The Netherlands). The drop of the dispersion was diluted using deionized water, and then dispersion was applied to a copper grid and kept for 1 min. The excess of dispersion was removed by absorbing with a piece of filter paper, and then a drop of 1% phosphotungstic acid was added. The sample was visualized by using soft imaging viewer software (Olympus Soft Imaging Solutions GmbH, Münster, Germany) for image analysis.
Entrapment efficiency
The entrapment efficiency of developed formulation was determined by reconstitution with 10 mL of phosphate buffer. The resulting suspension was sonicated in bath sonicator for 30 min. The untrapped drug was separated by centrifuging at 14 000 rpm for 30 min (REMI Cooling Centrifuge, Mumbai, India). The supernatant was taken and diluted with appropriate medium and PZ concentration in the resulting solution was assayed by UV-spectrophotometer at 270 nm. The percentage of drug entrapment was calculated by the following equation (El-Laithy et al., Citation2011).
where Ct concentration of total PZ and Cf concentration of free PZ.
Ex vivo skin permeation studies
The ex vivo permeation of PZ from all formulations across the rat skin was studied using a Franz diffusion cell (Logan, UT) with an effective permeation area of 1 cm2 and a receptor volume of 10 mL capacity. The permeation study was performed with receiver vehicle maintained at temperature of 32 ± 1 °C and stirring speed of 600 rpm. The rats used for the permeation study were sacrificed with prolonged ether anesthesia. The hair from abdominal skin was removed and skin was excised. The dermis side was wiped with isopropyl alcohol to remove residual adhering fat. The skin was washed with phosphate buffer saline and skin was placed in-between donor and receptor compartment (Ahad et al., Citation2011). Aliquots of niosomes equivalent to 10 mg of PZ were placed in the donor compartment and after specific time intervals 2 mL samples were withdrawn from the receptor compartment via the sampling port and replaced with fresh samples. The collected samples were filtered through membrane filter and diluted with medium for analysis by HPLC method as stated above. Similar experiments were performed for control formulation of pioglitazone. To determine the extent of permeation enhancement, enhancement ratio (ER) was calculated as follows:
Confocal laser scanning microscopy
Confocal laser scanning microscopy (CLSM) was used to scan fluorescence signal of pioglitazone optimized proniosomal formulation (POPF) and control formulation at different skin depths (Dayan & Touitou, Citation2000; Touitou et al., Citation2000). Both the formulations (containing 0.03% RR) were treated with skin. The treated skin was cut into sections of 6–10 μm and washed with distilled water. The sections were positioned on the microscopic slide with the SC side face to the cover glass. The probe penetration was carried out with Fluorescence Correlation Spectroscope-Olympus Fluo View FV1000 (Olympus, Melville, NY) with an argon laser beam at z-axis.
In vitro drug release
The release of PZ from niosomal gel formulation (POPF-gel) was determined using formulation containing equivalent of 10 mg PZ was placed in dialysis bag and attached to the shafts. The shaft was then lowered to the vessels so that formulation was fixed at a lower level. The study was performed with 500 mL of release medium at pH 6.8 with a speed of 100 rpm. The experimental conditions were set following the FIP/AAPS Guidelines for dissolution/in vitro release testing of transdermal formulations (Siewert et al., Citation2003). Five milliliter samples were withdrawn at predetermined time intervals for 24 h and replaced with fresh medium to maintain sink condition. The in vitro release study of control formulation was carried out in a similar manner. The collected samples were filtered and analyzed using HPLC as stated above. The obtained release data were subjected to various kinetic mechanism treatments (Higuchi, Citation1963).
In vivo study
Study design
The in vivo study was carried out to compare the pharmacokinetics profile of pioglitazone-optimized proniosomal formulation transdermal therapeutic system (POPF-TTS) and oral suspension of marketing tablet (10 mg/kg; Pioz, USV, Himachal Pradesh, India). The POPF-TTS of optimized formulation was fabricated as reservoir using double adhesive tape (Scothpak, 3M, Virginia, USA). The study protocol was approved by the University Animal Ethics Committee (Jamia Hamdard), and Committee for the Purpose of Control and Supervision of Experiments on Animals (CPSCEA), Government of India. The blood samples were withdrawn and collected into heparinized tubes at the following time points (0, 2, 4, 6, 8, 12, 24 and 48 h) after the administration. Plasma samples were separated by centrifugation (Remi Centrifuge, Mumbai, India) at 5000 rpm for 20 min and stored at −20 °C until drug analysis was carried out by HPLC method (Souri et al., Citation2008).
Pharmacokinetic analysis
Pharmacokinetic characteristics from plasma data following PZ administration at different time intervals were analyzed to calculate various parameters. The maximum plasma concentration (Cmax) and time to reach maximum concentration (Tmax) were directly determined from the plasma concentration versus time curve. The area under curve (AUC0–48 and AUC0–∞) were calculated with the help of GraphPad Software (GraphPad Software Inc., San Diego, CA), whereas the elimination half-life (t1/2) was calculated using the PK relationship. The relative bioavailability (%F) for transdermal drug delivery was calculated by comparing transdermal and oral AUCs. The PK data between two different formulations were compared for statistical significance by one-way ANOVA followed by Turkey–Kramer multiple comparisons test using Graph Pad Instat Software (GraphPad Software Inc., San Diego, CA).
Pharmcodynamic study
The preclinical assessment of antidiabetic activity of the developed POPF-TTS and tablet were performed on rats. Diabetes was induced by injecting with STZ injection (Arora et al., Citation2009). The animals were divided into four groups (Groups I–IV) of six animals each and initial blood sugars (BS) of rats were measured. Group I was taken as normal control, Group II served as diabetic control and received no further treatment, Group III received POPF-TTS with dose of 10 mg/kg, whereas Group IV received an oral suspension of PZ tablet of same dose (Pioz, USV, Himachal Pradesh, India; Elbary et al., Citation2008). The POPF-TTS was applied to the previously shaven abdominal area of rat skin. The rat was then placed in the restrainer and blood from the tail was taken on strip and measured on the electronic machine glucometer.
Skin irritation study
Irritancy test was carried out to determine possible reaction of the POPF-TTS on the Wistar rat skin. Test formulation and formalin solution (0.8% v/v) as standard irritant (Ubaidulla et al., Citation2007) were applied to the abdominal region of rats shaved approximately 24 h before the study. Care was taken to avoid abrading the skin. Animals were examined for signs of erythema and oedema responses. An adjacent area of untreated skin of each animal was used as a control. Skin irritation was observed and scoring was done according to scoring scale (Draize et al., Citation1944).
Results and discussion
Optimization
In this study, proniosome formulation was prepared by the film deposition technique using three factors, three levels Box–Behnken design employed to optimize the formulation. Total 15 runs with three central points were generated. The predicted and experimental values for the responses of vesicle size, entrapment efficiency and flux are shown in . The value of all responses was fitted to first order, second order and quadratic models, and was observed; the best-fitted model was quadratic and the comparative values of R2, SD and % CV are given in . The quadratic model resulted several response surface graphs for each response. The response surface graphs for vesicle size, entrapment efficiency and flux are shown in ). These plots show the effect of independent variables (interaction effects) on the dependent variables (responses), i.e. effect of two factors on the response at one time.
Figure 1. (a) Response surface analysis: a response surface graph representing the effect of independent variables on size, (b) response surface graph representing the effect of independent variables on % encapsulation efficiency and (c) response surface graph representing the effect of independent variables on flux.

Table 2. Summary of results of regression analysis for responses Y1, Y2 and Y3..
depicts response surface plot, characterizing increase in size with increasing concentration of Tween80 and phospholipid. The diameter of vesicles is dependent on the length of the alkyl chain of surfactants. Surfactants with long alkyl chains generally give larger vesicles.
depicts a dome-shaped response surface plot, characterizing initial increase in %EE with increasing concentration of both Tween80 and cholesterol, followed by a gradual decrease. Increasing cholesterol content from lower level to intermediate level leads to significant increase in %EE (p < 0.05), whereas further increase showed significant decrease (p < 0.05). High concentration of cholesterol may compete with drug for the space within the bilayers, hence excluding drug and can disrupt the regular linear structure of vesicular membranes (Mokhtar et al., Citation2008). Hence, it can be revealed that medium levels of cholesterol and Tween 80, show maximum %EE.
© portrays an interaction effect and the relationship between dependent variables on the flux as response variable. The 3D plot was observed, where with increasing the amount of cholesterol and phospholipid shows slight increase in flux, after that gradual decrease in flux value was observed. Higher concentration of cholesterol beyond certain concentration can disrupt regular linear structure of vesicular membranes (EL-Samaligy et al., Citation2006). All response surfaces were best fitted with quadratic polynomial models, and able to predict the interaction effects too. Then, the model was observed for ANOVA (), which revealed that the model terms for main effects and interaction effects were statistically significant. Finally, optimized formulation was selected by numerical optimization method having the desirability value as 1. The composition of the optimized formulation by point prediction was found to be Tween 80 (150 mg), phospholipid (45 mg), cholesterol (25 mg) with size (426.85 nm), %EE (91.46) and transdermal flux (50.84 µg/cm2/h). Based on the factorial design approach, optimized proniosomal formulation was selected for further in vivo studies by converting it into gel. Briefly, Carbopol® 934 (1% w/w) was added into water and kept overnight for complete humectation of polymer chains. The optimized formulation (POPF) equivalent to 40 mg of PZ was added to hydrated carbopol solution with constant stirring (Chaudhary et al., Citation2013; Ahad et al., Citation2014). Other ingredients, such as 15% w/v polyethylene glycol-400 (PEG-400) and triethanolamine (0.5% w/v), were added to obtain homogeneous dispersion of gel. This PZ optimized niosomal formulation-gel (POPF-gel) was incorporated into reservoir-type TTS for in vivo antidiabetic studies.
Response analysis
The response surface analysis was carried out to understand the effect of selected independent variables on observed responses. Linear correlation plot between actual and predicted response variables are shown in , and their R2 was found near to unity, which gives high predictive ability of RSM. The mathematical relationships were established and coefficients of the second-order polynomial equation were found to be quadratic in nature with interaction terms. The coefficients of polynomials fitted well to the data, with the values of R2 ranging between 0.9965 and 0.9996. This equation shows the individual and combination effects of all independent variables. A positive value in the regression equation for a response represents an effect that favors the optimization (synergistic effect), whereas a negative value indicates an inverse relationship (antagonistic effect) between the factor and response. The polynomial equation for average particle size is given as:
Figure 2. Regression line of actual versus predicted value: (a) particle size, (b) entrapment efficiency and (c) flux.

The model F-value and values of “Prob > F” were found 1267.24 and less than 0.05 indicate model terms are significant. In this case A, B, C, AB, BC, AC, A2, B2, C2 are significant model terms. The “Pred. R2” 0.9934 in reasonable agreement with the “Adj. R2” of 0.9982. This model can be used to navigate the design space. The polynomial equation of response Y1 shows the combined effect of cholesterol, Tween 80, and phospholipid on size of vesicle. The effect of Tween 80 and phospholipid concentrations were found more pronounced than cholesterol on size. An increase in vesicle size by increasing the concentration of Tween 80 and phospholipid.
The polynomial equation for average entrapment efficiency is given as
The Model F-value for EE was found to be 247.76, with “Prob > F” less than 0.05 which implies the model terms are significant. The “Pred R2” of 0.9691 is in reasonable agreement with the “Adj R2” of 0.9937. This model can be used to navigate the design space. This equation shows the individual and combination effects of all independent variables with A, B, C, AC, A2, B2 and C2 are found to be significant. Among independent factor, it was observed that independent factors showed positive effect on the EE due to lipophilic nature of drug. The polynomial equation for flux is given as:
The Model F-value of 1298.94 implies the model is significant due to “Prob > F” less than 0.05. In this case, the “Predicted R2” of 0.9958 is in reasonable agreement with “Adjusted R2” of 0.9985. This equation shows individual and combination effects of all independent variables with A, B, C, AB, AC, BC, A2, B2 and C2 are significant model terms. Among this independent factor, it was observed that cholesterol has significantly higher negative effect on flux and is evident from the very high negative value for its coefficient, means decreased flux on increased cholesterol, due to leaching of the drug from vesicle.
Vesicle size and size distribution
The mean vesicle size of all formulations was determined by dynamic light scattering as shown in . The vesicle size ranges found to be PZ formulations 391.76 ± 9.13–578.27 ± 6.29 nm (). The average vesicle size of optimized formulation from point prediction was found to be about 426.85 ± 3.56 nm. Small values of The PDI value for all the formulation was found between <0.1 and >0.3 indicate a homogeneous population.
Vesicle morphology
Transmission electron micrographs revealed the formation of well-identified spherical niosomal vesicles with sharp boundaries after hydration of proniosomes. The surface characteristics of proniosomes-derived niosomes of containing Tween 80, phospholipid and cholesterol, respectively, appeared as spherical vesicles under transmission electron microscope (). The absence of typical crystalline structures of PZ in developed formulation indicates transformation of drug to amorphous or molecular state.
Confocal laser microscopy
The extent of penetration of developed formulation and control formulation across skin was measured by CLSM after application of 0.03% RR to each formulation clearly explained transdermal potential of vesicular carrier (). The results revealed that developed formulation was evenly distributed throughout the SC, viable epidermis, and dermis with high fluorescence intensity. The formulations were delivered to a maximum possible depth of skin (up to 38.96 µm) of penetration as compared to control formulation that were confined to few micrometers (up to 13.94 µm) depth only. This prominently efficient delivery of RR by proniosomes suggests their enhanced penetration and consequent fusion with membrane lipids in depths of skin supporting the hypothesis of many researchers.
In vitro drug release
The release profile of PZ from niosomes was apparently biphasic release pattern. The kinetic analysis of release profiles showed diffusion controlled mechanism with rapid drug release was observed during initial hour followed by prolonged slower release (). This could be because desorption of PZ from the surface of niosomes while drug release in the slower phase was regulated by diffusion through the swollen niosomal bilayers (Pardakhty et al., Citation2007). Comparing release profile of control formulations, prolonged release rate and efficiency were observed, revealing that niosomal structure improved PZ release properties. This release profile could be important with initial fast drug release to achieve high-concentration gradient required for successful transdermal drug delivery to the blood (Csoka et al., Citation2007).
Pharmacokinetic study
The plasma concentration profiles of PZ following application of POPF-TTS and oral suspension in rats are shown in . The Cmax value was significantly lower (p < 0.05) for the transdermal formulation (41.19 ± 27.27 ng/mL) compared with the oral tablet formulation (54.52 ± 11.41 ng/mL). The Tmax values were higher for transdermal application (8 ± 0.76 h) than oral administration (2 ± 0.23 h) and the difference was highly significant statistically (p < 0.001). This difference in Tmax was because of barrier property of stratum corneum as well as sustained release of drug from the TTS. In contrast, oral formulation is conventional dosage form and hence lower Tmax values were observed. It was also observed that AUC0–48 and AUC0–∞ of transdermal formulation were 853.53 ± 61.1 and 1113.92 ± 84.27 ng/mL/h, respectively, and were extremely significant (p < 0.001) as compared to AUC0–48 (427.82 ± 57.59 ng/mL/h), AUC0–∞ (481.36 ± 69.67 ng/mL/h) of oral suspension. The elimination half-lives of pioglitazone after oral and transdermal administration were 2.41 ± 0.41 and 10.14 ± 1.63 h, respectively, and were statistically significant (p < 0.01). On transdermal administration, the half-life value of PZ was prolonged as compared to oral delivery. This could be attributed to longer duration of absorption via the transdermal system. The overall mean value of AUC0–48 by POPF was 2.03 times higher than that of oral route, and the difference was found to be statistically significant (p < 0.001) demonstrating improved bioavailability over a period of 48 h. The relatively high bioavailability from POPF-TTS could be due to the avoidance of hepatic metabolism by transdermal administration of PZ which provided high systemic drug availability compared to oral route.
Table 3. Pharmacokinetic parameters of pioglitazone in rats (mean ± SD, n = 6).
Antidiabetic activity
The results of the antidiabetic activity of transdermal formulation in comparison to tablet formulation are shown in . The developed POPF-TTS was found to decrease the BS significantly (p < 0.001) to normal level for 48 h in comparison to oral formulation. The effects of percentage reductions in BS of rats by POPF-TTS and oral formulation were 44.49 and 8.91%, respectively. Results of in vivo antidiabetic activity clearly indicate that POPF-TTS released the drug gradually over a period of time, which resulted in prolonged control of blood sugar up to 48 h. Formulation POPF-TTS was successful in decreasing the rat BS to normal values, whereas oral formulation failed to reduce the BS to normal value for prolonged period. The above results suggest that the developed transdermal formulation of pioglitazone holds promise for the management of diabetes and needs to be validated by clinical trials.
Table 4. Influence of formulations of pioglitazone on mean Blood sugar level (BS) in STZ-induced diabetic rats (mean ± SD, n = 6).
Skin irritation study
The skin irritation test of the developed formulations showed skin irritation score for erythema (0.51 ± 0.40) and edema (0.85 ± 0.57), whereas formalin solution shows higher irritation score for erythema (2.66 ± 0.78) and edema (3.33 ± 0.51). According to Draize et al. (Citation1944), compounds producing scores of 2 or less are considered negative (no skin irritation). No obvious erythema, edema or inflammation was observed on skin after application of the formulation whereas formalin solution showed significant skin irritation. Hence, the developed transdermal formulations are free of skin irritation.
Conclusion
Transdermal proniosomal formulation of pioglitazone prepared using carbopol as release-controlling polymer showed promising results which were optimized using three-factor, three-level Box–Behnken design. The quantitative responses size, EE and flux of PZ for different combinations of independent variables were obtained experimentally and the results were found to fit the design model. The quantitative effect of these factors at different levels on the responses could be predicted using polynomial equations as high linearity was observed between predicted and actual values of response variables. The in vitro release data showed that the release profiles of PZ from formulation showed apparently biphasic release process. The pharmacokinetic data showed carbopol transgel containing the same drug load as in one commercial tablet, was able to preserve a plasma concentration over the minimum effective concentration for 48 h. The antidiabetic profile showed transgel provide reduction in blood sugar level for longer period time in comparison to oral tablet and holds promise for improving patient compliance and ensuring better clinical outcomes.
Acknowledgements
The authors are also grateful to University Grants Commission, New Delhi, for providing Junior Research Fellowship to Mr. Prem Sunder. Authors are also thankful to AIIMS and JNU, New Delhi, for providing TEM, and CLSM facilities.
Declaration of interest
Authors declare no conflict(s) interest.
References
- Aboelwafa A, El-Setouhy DA, Elmeshad AN. (2010). Comparative study on the effects of some polyoxyethylene alkyl ether and sorbitan fatty acid ester surfactants on the performance of transdermal carvedilol proniosomal gel using experimental design. AAPS PharmSciTech 11:1591–602
- Ahad A, Aqil M, Kohli K, et al. (2011). Interactions between novel terpenes and main components of rat and human skin: mechanistic view for transdermal delivery of propranolol hydrochloride. Curr Drug Del 8:213–24
- Ahad A, Aqil M, Ali A. (2014). Investigation of antihypertensive activity of carbopol valsartan transdermal gel containing 1,8-Cineole. Int J Biol Macromol 64:144–9
- Ahad A, Aqil M, Kohli K, et al. (2013). Enhanced transdermal delivery of an anti-hypertensive agent via nanoethosomes: statistical optimization, characterization and pharmacokinetic assessment. Int J Pharm 443:26–38
- Arora S, Ojha SK, Vohora D. (2009). Characterisation of streptozotocin induced diabetes mellitus in Swiss Albino mice. Glob J Pharm 3:81–4
- Balakrishnan P, Shanmugam S, Lee WS, et al. (2009). Formulation and in vitro assessment of minoxidil niosomes for enhanced skin delivery. Int J Pharm 377:1–8
- Beg S, Swain S, Singh HP, et al. (2012). Development, optimization, and characterization of solid self-nanoemulsifying drug delivery systems of valsartan using porous carriers. AAPS PharmSciTech 13:1416–27
- Chaudhary H, Kohli K, Amin S, et al. (2011). Optimization and formulation design of gels of diclofenac and curcumin for transdermal drug delivery by Box-Behnken statistical design. J Pharm Sci 100:580–93
- Chaudhary H, Rohilla A, Rathee P, Kumar V. (2013). Optimization and formulation design of carbopol loaded Piroxicam gel using novel penetration enhancers. Int J Biol Macromol 55:246–53
- Chawla V, Saraf SA. (2012). Rheological studies on solid lipid nanoparticles based carbopol gels of aceclofenac. Colloids Surf B: Biointerfaces 92:293–8
- Csoka G, Marton S, Zelko R, et al. (2007). Application of sucrose fatty acid esters in transdermal therapeutic systems. Eur J Pharm Sci 65:233–7
- Dayan N, Touitou E. (2000). Carriers for skin delivery of trihexyphenidyl HCl: ethosomes vs. liposomes. Biomaterials 21:1879–85
- Draize JH, Woodward G, Calvery HO. (1944). Methods for the study of irritation and toxicity of substances applied topically to the skin and mucous membranes. J Pharm Exp Ther 82:377–9
- Elbary AA, Kassem MA, Samra MMA, Khalil RM. (2008). Formulation and hypoglycemic activity of pioglitazone-cyclodextrin inclusion complexes. Drug Discov Ther 2:94–107
- El-Kattan A, Asbill CS, Haidar S. (2000). Transdermal testing: practical aspects and methods. PharmSci Tech Today 3:426–30
- El-Laithy HM, Shoukry O, Mahran LG. (2011). Novel sugar esters proniosomes for transdermal delivery of vinpocetine: preclinical and clinical studies. Eur J Pharm Sci 77:43–55
- EL-Samaligy MS, Afifi NN, Mahmoud EA. (2006). Increasing bioavailability of silymarin using a buccal liposomal delivery system: preparation and experimental design investigation. Int J Pharm 308:140–8
- Fang JY, Yu SY, Wu PC, et al. (2001). In vitro skin permeation of estradiol from various proniosome formulations. Int J Pharm 215:91–9
- Garcia-Gonzalez N, Kellaway IW, Blanco FH, et al. (1994). Influence of glycerol concentration and carbopol molecular weight on swelling and drug release characteristics of metochlopramide hydrogels. Int J Pharm 104:107–13
- Higuchi T. (1963). Mechanism of sustained-action medication: theoretical analysis of rate of release of solid drugs dispersed in solid matrices. J Pharm Sci 52:1145–9
- Hwang RC, Kowalski DL. (2005). Design of experiments for formulation development. Pharm Tech 1–5
- Imam SS, Aqil M, Akhtar M, et al. (2013). Formulation by design based proniosome for accentuated transdermal delivery of risperidone: in vitro characterization and in vivo pharmacokinetic study. Drug Delivery, Early Online: 1–12, DOI: 10.3109/10717544.2013.870260
- Jana S, Manna S, Nayak AK, et al. (2014). Carbopol gel containing chitosan-egg albumin nanoparticles for transdermal aceclofenac delivery. ColloidsSurf B: Biointerfaces 114:36–44
- Junyaprasert VB, Singhsa P, Suksiriworapong J, Chantasart D. (2012). Physicochemical properties and skin permeation of Span 60/Tween 60 niosomes of ellagic acid. Int J Pharm 423:303–11
- Manconi M, Sinico C, Valenti D, et al. (2006). Niosomes as carriers for tretinoin III A study into the in vitro cutaneous delivery of vesicle-incorporated tretinoin. Int J Pharm 311:11–19
- Mokhtar M, Omaima AS, Mohammed AH, Nagia AM. (2008). Effect of some formulation parameters on flurbiprofen encapsulation and release rates of niosomes prepared from proniosomes. Int J Pharm 361:104–11
- Mura S, Pirot F, Manconi M, et al. (2007). Liposomes and niosomes as potential carriers for dermal delivery of minoxidil. J Drug Target 15:101–8
- Muzzalupo R, Tavano L, Cassano R, et al. (2011). A new approach for the evaluation of niosomes as effective transdermal drug delivery systems. Eur J Pharm Sci 79:28–35
- Naik A, Kalia YN, Guy RH. (2000). Transdermal drug delivery: overcoming the skin’s barrier function. Pharm Sci Tech Today 3:318–26
- Pardakhty A, Varshosaz J, Rouholamini A. (2007). In vitro study of polyoxyethylene alkyl ether niosomes for delivery of insulin. Int J Pharm 328:130–41
- Reginato MJ, Lazar MA. (1999). Mechanisms by which Thiazolidinediones enhance insulin action. Trends Endocr Metab 10:9–13
- Rogerson A, Cummings J, Willmott N, Florence AT. (1988). The distribution of doxorubicin in mice administration in niosomes. J Pharm Pharmacother 40:337–42
- Shumilov M, Touitou E. (2010). Buspirone transdermal administration for menopausal syndromes, in vitro and in animal model studies. Int J Pharm 387:26–33
- Siewert M, Dressman J, Brown CK, Shah VP. (2003). FIP/AAPS guidelines to dissolution/in vitro release testing of novel/special dosage forms. AAPS PharmSciTech 4:1–10
- Singh B, Kumar R, Ahuja N. (2005). Optimizing drug delivery systems using systematic “design of experiments.” Part I: fundamental aspects. Crit Rev Ther Drug Carrier Syst 22:27–105
- Souri E, Jalalizadeh H, Saremi S. (2008). Development and validation of a simple and rapid HPLC method for determination of pioglitazone in human plasma and its application to a pharmacokinetic study. J Chromatogr Sci 46:809–12
- Touitou E, Dayan N, Bergelson L, et al. (2000). Ethosomes–novel vesicular carriers for enhanced delivery: characterization and skin penetration properties. J Control Release 65:403–18
- Tripathi KD. (2003). Essential of medical pharmacology. New Delhi: Jaypee Brother’s Medical Publishers (P) Ltd., 247–51
- Ubaidulla U, Reddy MV, Ruckmani K, et al. (2007). Transdermal therapeutic system of carvedilol: effect of hydrophilic and hydrophobic matrix on in vitro and in vivo characteristics. AAPS PharmSciTech 8:E1–8
- Varshosaz J, Pardakhty A, Baharanchi SM. (2005). Sorbitan monopalmitate based proniosomes for transdermal delivery of chlorpheniramine maleate. Drug Deliv 12:75–82
- Vitorino C, Almeida J, Gonçalves LM, et al. (2013). Co-encapsulating nanostructured lipid carriers for transdermal application: from experimental design to the molecular detail. J Control Release 167:301–14
- Waugh J, Keating GM, Plosker GL, et al. (2006). Pioglitazone – a review of its use in type 2 diabetes mellitus. Drugs 66:85–109
- Weber E, Moyers-Gonzalez M, Burghelea TI. (2012). Thermorheological properties of a Carbopol gel under shear. J Non-Newt Fluid Mech 183–4:14–24