Abstract
Context: Sequencing of cDNA clones from plant tissue to generate expressed sequence tags (ESTs) is an effective tool for gene discovery. Together with powerful bioinformatics tools, EST sequences allow the prediction of functions of putative bioactive compounds that can later be confirmed.
Objective: To isolate a detoxification enzyme from an EST library from the oil palm (Elaeis guineensis Jacq. Arecaceae).
Methods: In total, 750 clones from an oil palm cDNA library were randomly sequenced and analyzed. A clone homologous to cytochrome P450 monooxygenases (P450) was selected from the list of highly expressed genes. The full-length cDNA of P450 from E. guineensis (EgP450) was generated and transformed into a bacterial host to produce recombinant protein. A 3D model of EgP450 was generated and used in a molecular docking analysis to screen for target herbicide substrates. Finally, the detoxification activity of EgP450 was confirmed by an herbicide tolerance test with rice seedlings.
Results and discussion: The full-length EgP450 has an open reading frame (ORF) of 1515 bp that encodes a protein of 505 amino acids. Docking analysis showed that EgP450 bound to phenylurea-like herbicides such as isoproturon, chlortoluron and fluometuron. The herbicide tolerance test demonstrated that the presence of EgP450 protected the rice seedlings from the killing action of the phytotoxic agent isoproturon.
Conclusions: The gene EgP450 was detected in the roots and stems of oil palm tissues, and its recombinant product was shown to protect rice seedlings from exogenous herbicides of the phenylurea family.
Introduction
The oil palm (Elaeis guineensis Jacq.) is a monocotyledonous plant of the palm family (Arecaceae). It is a species of particular economic importance, as it is the second largest source of vegetable oil in the world after soybean. Palm oil is obtained from the mesocarp of fresh oil palm fruit, and palm kernel oil is extracted from the seed. Approximately 20% of palm oil usage is devoted to non-food items. On the basis of recent research, it has been claimed that palm oil is a beneficial health food. It is said to be packed with nutrients and bioactive compounds such as antioxidants and antitumor agents. These characteristics have motivated many efforts to discover new compounds in the oil palm that will definitely be of use for improving human health.
Expressed sequence tags (ESTs) are short DNA sequences (usually 200–500 nucleotides long) that are generated by sequencing either one or both ends of a library of cloned transcripts. They are fragments of DNA that represent genes expressed in a sample of cells or tissues from a particular organism (CitationBoguski et al., 1993). EST sequencing is an effective method for gene discovery, gene cloning and the measurement of gene expression (CitationRudd, 2003). When combined with bioinformatics tools, EST information enables the selection of a target molecule in a particular biological pathway and its subsequent expression in a recombinant host. This is a powerful method not only for discovering new bioactive products but also for expanding to large-scale production.
Much progress in science, agriculture and industry has caused serious problems on the quality of the environment especially from contamination by chemicals that can affect human health. In the past decade there have been a growing numbers of systems developed that use plants for phytoremediation to remove or metabolize pollutants from the environment (CitationJames & Strand, 2009). The metabolic processes of higher plants that can modify xenobiotic compounds normally include three stages: conversion, conjugation and compartmentalization (CitationJames & Strand, 2009; CitationMezzari et al., 2005). In the conversion step, cytochrome P450 is a key enzyme in the metabolism of toxic compounds.
P450 enzymes (also called mixed function oxidases, cytochrome P450 monooxygenases: CYP) are one of the most important enzyme systems for detoxification. They are a complex family of heme-containing enzymes and are found in most organisms. To date, various types of P450 enzymes have been reported in animals, microorganisms, and plants; they have been classified into more than 36 families (CitationNelson et al., 1993). The name of each cytochrome P450 enzyme denotes the following: order, family (clan), subfamily, and isoform. For example, the name “CYP71A1” indicates that the enzyme belongs to clan 71, subfamily A and isoform 1 (CitationKim et al., 2004). P450 enzymes bind to molecular oxygen and receive electrons from NADPH to introduce an oxygen atom to their substrates.
In higher plants, P450 enzymes have been shown to metabolize exogenous chemicals such as herbicides (CitationSchuler, 1996; CitationBolwell et al., 1994) and their role in herbicide conversion usually consists of hydroxylation or dealkylation. Two cDNAs encoding CYP81B2 and CYP71A11 that were cloned from tobacco cells, CYP81B2 showed m-methyl hydroxylation activity, and CYP71A11 exhibited both N-demethylation and m-methyl hydroxylation of chlortoluron. CYP76B1 from Helianthus tuberosus catalyzed the oxidation of several phenylurea herbicides (CitationYamada et al., 2000; CitationRobineau et al., 1998; CitationWerck-Reichhart et al., 2000). A trans-cinnamic acid hydroxylase cDNA (CYP73A1) isolated from the Jerusalem artichoke catalyzed the ring-methyl hydroxylation of chlortoluron (CitationPierrel et al., 1994; CitationSiminszky et al., 1999). The CYP71A10 enzyme from the soybean was shown to specifically catalyze the metabolism of the phenylurea herbicides fluometuron, linuron, chlortoluron, and diuron (CitationSheldon et al., 2000). The CYP76B1 enzyme from the Jerusalem artichoke catalyzed the dealkylation of several xenobiotics, including phenylurea herbicides (CitationBatard et al., 1998; CitationRobineau et al., 1998; CitationDidierjean et al., 2002).
Although an unmodified plant will sometimes take-up and metabolize pollutants into nontoxic products, it is a slow and inefficient process and it can be greatly enhanced by the genetic modification of the plant in question. Transgenic plants can be made to overexpress one or more of the enzymes in the existing metabolic pathways. It is also possible to introduce new metabolic pathways into plants. For examples, introduction of the human CYP1A1, CYP2B6, CYP2C9 and CYP2C19 cDNAs into rice and potato resulted in elevated herbicide metabolism and enhanced the resistance to a wide range of herbicides including atrazine, acetochlor, metolachlor, chlorsulfuron, imazosulfuron, chlortoluron, methabenzthiazuron, norflurazon, mefenacet and pyributicarb (CitationKawahigashi et al., 2005a). When the pig CYP2B22 and CYP2C49 cDNAs were overexpressed in rice, the resistance against 12 and 13 herbicides, respectively, was observed to have increased (CitationKawahigashi et al., 2005b). Simultaneously the expression of the human CYP2E1 and glutathione S-transferase in transgenic alfalfa increased the capabilities of alfalfa to become resistant to a mixed contamination by heavy metal-organic compounds (CitationZhang & Liu, 2011). Transgenic poplar (Populus tremula × Populus alba) plants were developed to metabolize and remove pollutants from the air at a fast rate and at a low cost through the overexpression of cytochrome P450 2E1 (CitationDoty et al., 2007). Producing transgenic plants and associated genetically modified bacteria is another promising technology that could produce broader and more efficient applications for the treatment of polychlorinated biphenyls (PCBs) by phytoremediation (CitationAken et al., 2010).
Now with the diverse types of toxic substances distributed worldwide, there are number of demands for using specific P450 enzymes to destroy specific chemicals. CitationSyed (2010) reported the first comprehensive identification and functional characterization of P450 monooxygenases capable of oxidizing different ring-size PAHs by using a genome-to-function strategy.
To accelerate the identification of bioactive compounds in E. guineensis, we constructed a cDNA library from mesocarp tissue. An analysis of the EST we identified one clone that had a high sequence identity to P450 enzymes. This paper describes the cloning and characterization of this novel E. guineensis P450 enzyme. The enzyme may be useful in the area of phytoremediation.
Materials and methods
Plant materials and total RNA isolation
Mesocarp tissue was excised from oil palm fruits, 12 weeks after anthesis (WAA). Total RNA was extracted from 50 mg of 12 WAA mesocarp tissue using Trizol reagent (GIBCOBRL) according to the manufacturer’s instructions. The total RNA obtained was used as a template for cDNA synthesis.
cDNA library construction
A cDNA library was constructed using the ZAP-cDNA synthesis kit and the ZAP-cDNA Gigapack® III Gold packaging extract (Stratagene, CA, USA). The mRNA was reverse transcribed, and double-stranded cDNA was synthesized following the manufacturer’s instructions. Synthesized cDNA was directionally cloned into the ZAP Express vector using the EcoRI and XhoI sites. The cDNA library was amplified. Fragments of about 500–1500 bp were selected by subjecting the library to electrophoresis on an agarose gel, and the cDNA inserts from the amplified phage library were rescued as a pBK-CMV phagemid in XLOLR Escherichia coli according to the manufacturer’s instructions. Plasmids from selected colonies were sequenced ( Macrogen Inc., Republic of Korea) on the ABI Prism 377 Automated DNA sequencer (Applied Biosystems Inc., CA, USA).
Sequence processing and analysis
EST sequences were loaded into the Vector NTI Suite (version 8.0, Informax), and alignments were created using ClustalX 1.81. A BLASTX search of the NCBI non-redundant protein library was performed to identify entries similar to the edited EST sequences (CitationAltschul et al., 1990). Finally, the sequences, alignments and BLAST results were automatically integrated in a relational database, making them searchable via a local web browser-based interface. Translated and untranslated oil palm ESTs were manually sorted into 12 functional groups and an “unclassified” group on the basis of comparisons to similar GenBank entries.
Isolation of cytochrome P450 monooxygenase cDNA clones
A 936 bp partial sequence EgP450 was selected from the EST library. The C-terminus of this DNA fragment shows homology to a cytochrome P450 monooxygenase. A full-length cDNA was generated by 3′ RACE (Rapid Amplification of cDNA Ends). Five micrograms of total RNA from the mesocarp was ligated with the GeneRacer™ RNA Oligo according to the manufacturer’s instructions. Amplification of cDNA was carried out with the GeneRacer™ 3′ primer and EgP450-GSP reverse primer (5′-GTT CTT GAC GAC GTG ATC AAG-3′). A second amplification was performed with the nested forward primer provided in the kit and a reverse nested primer designed to target the EgP450-Nested sequence (5′-AAT TTG CTA GTG CAA GCT CCA C-3′). The PCR products were separated by electrophoresis, purified and cloned into pGEM-T Easy for sequence analysis.
Modeling analysis
Modeling of the homology of the 2D/3D structures was performed using the SWISS-MODEL (CitationArnold et al., 2006; CitationZhang, 2009). The best predicted structure was validated based on the best scoring model given by a Ramachandran plot analysis, which was performed using the ProFunc and PROCHECK software packages (http://www.ebi.ac.uk/thornton-srv/databases/profunc) (CitationLaskowski et al., 2005).
Molecular docking simulations and interaction analysis
The screening for molecular interactions of EgP450 with various chemicals and herbicides was performed using the AutoDOCK simulation tool (CitationHuey et al., 2007; CitationMorris et al., 1996) and the SLITHER server (CitationLee et al., 2009).
Semi-quantitative RT-PCR analysis
Samples of 3-month-old oil palm leaves, stems, crown galls, and roots were used for semi-quantitative RT-PCR analysis. Total RNA was treated with RQ1 RNase-free DNase (Promega) according to the manufacturer’s instructions. Reverse transcription was performed using 2 µg of the total RNA as template in a 25 µL reaction with reverse transcriptase (Promega) and a random primer. To amplify a 350 bp fragment from EgP450, EgP450 sense and antisense primers were used. Then, 18S rDNA primers were used to amplify a PCR product of 530 bp as an internal control for gene expression. The conditions for the PCR reaction were as follows: 1 cycle at 94°C for 5 min, then 35 cycles of 94°C for 1 min, 48°C for 1 min and 72°C for 1 min. The program was terminated by a 10 min incubation step at 72°C.
Preparation of recombinant EgP450
The full-length EgP450 coding sequence was subcloned into pGEX 4T-1 and transformed into E. coli BL21. The addition of 0.1 mM IPTG was used to induce the expression of the recombinant protein, and the protein was harvested as described by CitationFavacho et al. (2006).
Herbicide tolerance test
A modified version of the herbicide tolerance test described by CitationSiminszky (1999) was used, and isoproturon was chosen as the herbicide in the test. Concentrations of isoproturon ranging from 0.25 to 3.0 µM were verified to be the correct concentrations before starting the experiment.
Rice seeds (Oryza sativa L. Poaceae) were germinated by incubation in a hydroponic solution for 4 days. Seedlings that were 5 cm in height were then divided into 3 groups: Group 1, in which the seedlings were incubated in the hydroponic solution containing 2.5 µM isoproturon; Group 2, in which the seedlings were incubated in a hydroponic solution containing 2.5 µM isoproturon + 1.45 mg/mL of recombinant EgP450; and Group 3 in which the seedlings were incubated in a hydroponic solution without any other supplement. The test chambers were incubated at 27°C with a 16 h light and 8 h dark cycle. The growth of the seedlings was monitored for 2 weeks.
Results and discussion
Sequencing and clustering of oil palm ESTs
From the EST library, 750 clones were chosen at random for sequencing. The sequences were annotated according to their closest BLAST hits with known functions, and they were classified into general biochemical and metabolic functional categories. The results showed that 357 ESTs, corresponding to 48% of the total ESTs, had significant sequence similarity to genes with known functions, whereas 227 ESTs (30%) were similar to genes with unknown function and 166 ESTs (22%) showed no similarity to known genes. Of the ESTs matching genes with known functions, the most common functional categories were involved with gene expression, regulation and protein synthesis (21.9%); metabolism (18.2%); defense and cell rescue (17.1%); miscellaneous functions (12.3%); signal transduction (7.3%); ribosomal and rRNA (5.3%); cell division cycle (5.0%); transport (4.0%), mitochondrial protein (3.6%); biosynthesis (2.5%); abiotic stimuli and development (2.0%); and secretion (0.8%). These results illustrate that the gene expression profiles in E. guineensis were comparable to those of other plants in terms of the types of the functional categories represented.
provides a list of genes that were highly expressed in the oil palm that are homologous to genes involved in biosynthesis and metabolism, protein synthesis and stress/defense, and detoxification. The oil palm ESTs related to detoxification included metallothionein-like proteins and a cytochrome P450. These proteins have been reported to be involved in the defense response and the detoxification of exogenous molecules such as pesticides and pollutants (CitationWerck-Reichhart et al., 2000). In this work, we pursued functional studies of the P450 clone.
Table 1. Identical genes may have roles in biosynthesis and metabolism, protien synthesis and stress/defense and detoxification in cDNA library.
Characterization of EgP450
The 936 bp clone FC25 was identified by a BLASTX search. It closely matched the C-terminus of other plant P450 genes, and it was thus named EgP450. The full-length EgP450 had an open reading frame (ORF) of 1515 bp encoding a protein of 505 amino acids, and its sequence was deposited in GenBank under accession no. GU117705. EgP450 has a predicted molecular weight of 54 kDa, with a calculated pI of 6.63. Sequence analysis of EgP450 led to the identification of several conserved regions found in other P450 proteins (CitationWerck-Reichhart et al., 2002), such as the heme-binding sequence motif FGsGRRICPG at amino acid residues 435–444 and an oxygen-binding pocket involved in oxygen activation and the transfer of protons to the active site (A/G)Gx(D/E)T(T/S). The E-R-R triad is generally thought to be involved in the locking of the heme pocket into position and for the stabilization of the conserved core structure (CitationHasemann et al., 1995). Most other regions were highly variable, including the aromatic region (F/Y/W)(G)xxPxx(F/Y/W)xPx(R/H)(F/Y/W), which is involved in substrate recognition ().
Figure 1. Multiple sequence alignment of the EgP450 protein with similar sequences to the P450 from A. officinalis (GenBank accession no. BAD06417), M. armeniacum (GenBank accession no. BAD16679), E. guineensis (GenBank accession no. GU117705), T. aestivum (GenBank accession no. CAO64222), P. americana (GenBank accession no. AAA32913) and E. oleifera (ES414710). Conserved regions that may be important for detoxification activity, including the active site itself, are highlighted in brown boxes.
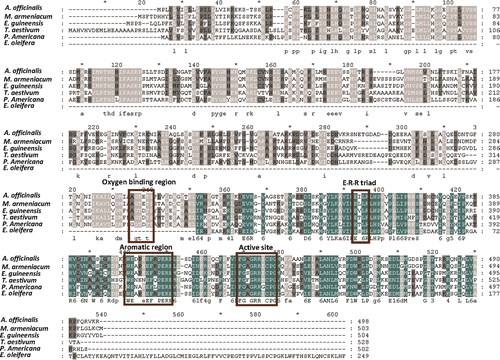
BLASTX alignments against P450 genes from other plant species revealed 57% identity to Asparagus officinalis and Muscari armeniacum, 54% to Persea americana and 52% to Triticum aestivum (). The EgP450 protein contains the same characteristic functional domains as the CYP71A1 enzyme from P. americana, and indicates that EgP450 belongs to the CYP71A superfamily (CitationNelson et al., 1993).
Tissue distribution of EgP450
The expression of EgP450 was investigated in various tissues (leaves, stems, crown galls, and roots) by semi-quantitative RT-PCR. As shown in , the transcripts of this gene are expressed abundantly in root and stem tissues, and indicates that the enzyme is active in these organs.
Figure 2. Detection of EgP450 expression by semi-quantitative RT-PCR, using total RNA from various tissues as samples and the expression of the 18s rRNA gene as an internal control. Lane L: leaf; lane S: stem; lane Cr: crown gall; lane R: root.
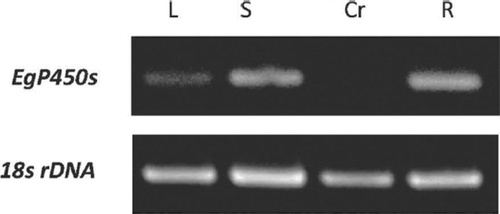
Docking simulations and interaction analysis
In 1999, Siminszky cloned the CYP71A10 gene from the soybean and overexpressed it in tobacco. CYP71A10 increased the tolerance of transgenic tobacco to chlortoluron by converting the herbicides to non-phytotoxic products. We sought to predict the three-dimensional structure of EgP450 and simulate its potential interactions with herbicides. The predicted 3D structure is shown in . The docking results () clearly show that EgP450 binds to the phenylurea group of herbicides, which includes isoproturon, chlortoluron, and fluometuron. The core binding site predictions for EgP450 comprise four regions: residues 303–308 (oxygen-binding site 1), residues 360–363 (E-R-R triad site 2), residues 407–418 (aromatic site 3), and residues 360–363 and 435–444 (active site 4).
Figure 3. The 3D structure of the putative EgP450 protein. The image was generated by using SWISS-MODEL and the I-TASSER server.
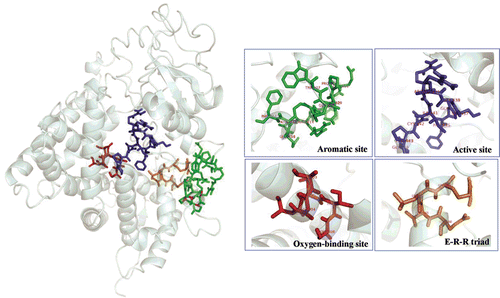
Figure 4. A 3D representation of the predicted structure of EgP450 and its binding partners (herbicides). The core binding site predictions of EgP450 comprise four regions: residues 303–308 (red), oxygen-binding site 1; residues 360–363 (orange), E-R-R triad site 2; residues 407–418 (green), aromatic site 3; and residues 360–363 and 435–444 (blue), active site 4.
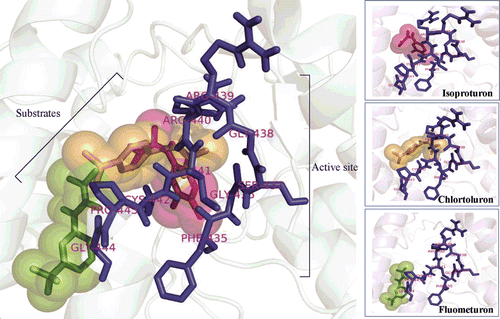
EgP450 has herbicide detoxification activity
The reports by others that CYP71A is involved in herbicide detoxification, together with the molecular docking work described above, indicate that EgP450 would have an ability to detoxify phenylurea. We conducted an experiment to determine the activity of EgP450 in an herbicide tolerance test. In the first week of the experiment, seedlings in all test groups grew at the same rate. In the second week, the phytotoxicity of isoproturon was observed in seedling group 1, in which death was observed on day 12. For the seedlings in group 3 (hydroponic solution only) and group 2 (hydroponic solution + isoproturon+ EgP450), growth continued at the same rate (). This result clearly demonstrates that EgP450 provides protection against isoproturon.
Figure 5. Growth of rice (O. sativa) seedling at 12 days after germination. Seedlings that were 5 cm in height were used in all test groups, and 3 replicate experiments were performed. The seedlings were incubated in the following solutions 1: hydroponic solution containing 2.5 µM isoproturon. 2: hydroponic solution containing 2.5 µM isoproturon + 1.45 mg/mL of recombinant EgP450 and 3: hydroponic solution alone.
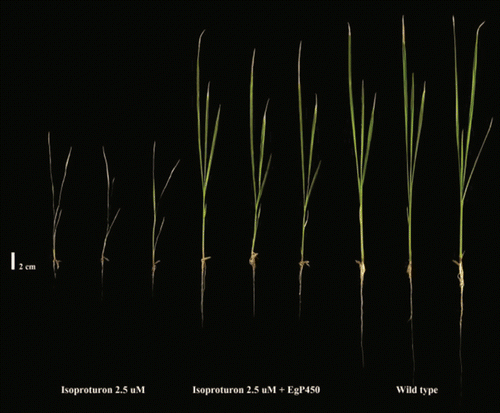
Conclusions
The use of an EST-screening approach enabled the identification of genes in E. guineensis. Of the 750 ESTs sequenced, 48% were had significant sequence similarities to known genes in the databases searched. The ESTs analyzed in this study represented a variety of biological pathways.
The EgP450 gene was cloned from this EST library. It is 54% similar to the CYP71A1 enzyme from P. americana and has the same essential functional sites. A molecular docking analysis revealed that EgP450 specifically bound to herbicides such as isoproturon, chlortoluron and fluometuron. The presence of this recombinant EgP450 in the growth medium of rice seedlings protected them from the killing action of the herbicide isoproturon.
Declaration of interest
This work was supported by the National Research University Project of Thailand’s Office of the Higher Education Commission (SCI540561S) for financial support to Sureeporn Nualkaew, the Program Strategic Scholarships for Frontier Research Network for the Ph.D. Program Thai Doctoral degree from the Office of the Higher Education Commission, Thailand for support to Alisa Nakkaew, the Graduated School, the Center for Genomics and Bioinformatics Research, the Faculty of Science, and Prince of Songkla University.
References
- Aken BV, Correa PA, Schnoor JL. (2010). Phytoremediation of polychlorinated biphenyls: New trends and promises. Environ Sci Technol, 44, 2767–2776.
- Altschul SF, Gish W, Miller W, Myers EW, Lipman DJ. (1990). Basic local alignment search tool. J Mol Biol, 215, 403–410.
- Arnold K, Bordoli L, Kopp J, Schwede T. (2006). The SWISS-MODEL workspace: A web-based environment for protein structure homology modelling. Bioinformatics, 22, 195–201.
- Batard Y, LeRet M, Schalk M, Robineau T, Durst F, Werck-Reichhart D. (1998). Molecular cloning and functional expression in yeast of CYP76B1, a xenobiotic-inducible 7-ethoxycoumarin O-de-ethylase from Helianthus tuberosus. Plant J, 14, 111–120.
- Boguski MS, Lowe TM, Tolstoshev CM. (1993). dbEST–database for “expressed sequence tags”. Nat Genet, 4, 332–333.
- Bolwell GP, Bozak K, Zimmerlin A. (1994). Plant cytochrome P450. Phytochemistry, 37, 1491–1506.
- Didierjean L, Gondet L, Perkins R, Lau SM, Schaller H, O’Keefe DP, Werck-Reichhart D. (2002). Engineering herbicide metabolism in tobacco and Arabidopsis with CYP76B1, a cytochrome P450 enzyme from Jerusalem artichoke. Plant Physiol, 130, 179–189.
- Doty SL, James CA, Moore AL, Vajzovic A, Singleton GL, Ma C, Khan Z, Xin G, Kang JW, Park JY, Meilan R, Strauss SH, Wilkerson J, Farin F, Strand SE. (2007). Enhanced phytoremediation of volatile environmental pollutants with transgenic trees. Proc Natl Acad Sci USA, 104, 16816–16821.
- Favacho AR, Kurtenbach E, Sardi SI, Gouvea VS. (2006). Cloning, expression, and purification of recombinant bovine rotavirus hemagglutinin, VP8*, in Escherichia coli. Protein Expr Purif, 46, 196–203.
- Hasemann CA, Kurumbail RG, Boddupalli SS, Peterson JA, Deisenhofer J. (1995). Structure and function of cytochromes P450: A comparative analysis of three crystal structures. Structure, 3, 41–62.
- Huey R, Morris GM, Olson AJ, Goodsell DS. (2007). A semiempirical free energy force field with charge-based desolvation. J Comput Chem, 28, 1145–1152.
- James CA, Strand SE. (2009). Phytoremediation of small organic contaminants using transgenic plants. Curr Opin Biotechnol, 20, 237–241.
- Kawahigashi H, Hirose S, Inui H, Ohkawa H, Ohkawa Y. (2005a). Enhanced herbicide cross-tolerance in transgenic rice plants co-expressing human CYP1A1, CYP2B6 and CYP2C19. Plant Sci, 168, 773–781.
- Kawahigashi H, Hirose S, Ozawa K, Ido Y, Kojima M, Ohkawa H, Ohkawa Y. (2005b). Analysis of substrate specificity of pig CYP2B22 and CYP2C49 towards herbicides by transgenic rice plants. Transgenic Res, 14, 907–917.
- Kim B-G, Ko JH, Hur H-G, Ahn J-H. (2004). Classification and expression analysis of cytochrome P450 genes from soybean. Agric Chem Biotechnol, 47, 173–177.
- Laskowski RA, Watson JD, Thornton JM. (2005). ProFunc: A server for predicting protein function from 3D structure. Nucleic Acids Res, 33, W89–W93.
- Lee PH, Kuo KL, Chu PY, Liu EM, Lin JH. (2009). SLITHER: A web server for generating contiguous conformations of substrate molecules entering into deep active sites of proteins or migrating through channels in membrane transporters. Nucleic Acids Res, 37, W559–W564.
- Mezzari MP, Walters K, Jelínkova M, Shih MC, Just CL, Schnoor JL. (2005). Gene expression and microscopic analysis of Arabidopsis exposed to chloroacetanilide herbicides and explosive compounds. A phytoremediation approach. Plant Physiol, 138, 858–869.
- Morris GM, Goodsell DS, Huey R, Olson AJ. (1996). Distributed automated docking of flexible ligands to proteins: Parallel applications of AutoDock 2.4. J Comput Aided Mol Des, 10, 293–304.
- Nelson DR, Kamataki T, Waxman DJ, Guengerich FP, Estabrook RW, Feyereisen R, Gonzalez FJ, Coon MJ, Gunsalus IC, Gotoh O. (1993). The P450 superfamily: Update on new sequences, gene mapping, accession numbers, early trivial names of enzymes, and nomenclature. DNA Cell Biol, 12, 1–51.
- Pierrel MA, Batard Y, Kazmaier M, Mignotte-Vieux C, Durst F, Werck-Reichhart D. (1994). Catalytic properties of the plant cytochrome P450 CYP73 expressed in yeast. Substrate specificity of a cinnamate hydroxylase. Eur J Biochem, 224, 835–844.
- Robineau T, Batard Y, Nedelkina S, Cabello-Hurtado F, LeRet M, Sorokine O, Didierjean L, Werck-Reichhart D. (1998). The chemically inducible plant cytochrome P450 CYP76B1 actively metabolizes phenylureas and other xenobiotics. Plant Physiol, 118, 1049–1056.
- Rudd S. (2003). Expressed sequence tags: Alternative or complement to whole genome sequences? Trends Plant Sci, 8, 321–329.
- Schuler MA. (1996). Plant cytochrome P450 monooxygenases. Critical Reviews in Plant Sci, 15, 235–284.
- Sheldon BS, Corbin FT, Dewey RE. (2000). A cytochrome P450 monooxygenase cDNA (CYP71Al0) confers resistance to linuron in transgenic Nicotiana tabacum. Weed Sci, 48, 291–295.
- Siminszky B, Corbin FT, Ward ER, Fleischmann TJ, Dewey RE. (1999). Expression of a soybean cytochrome P450 monooxygenase cDNA in yeast and tobacco enhances the metabolism of phenylurea herbicides. Proc Natl Acad Sci USA, 96, 1750–1755.
- Syed K, Doddapaneni H, Subramanian V, Lam YW, Yadav JS. (2010). Genome-to-function characterization of novel fungal P450 monooxygenases oxidizing polycyclic aromatic hydrocarbons (PAHs). Biochem Biophys Res Commun, 399, 492–497.
- Werck-Reichhart D, Bak S, Paquette S (2002) Cytochrome P450. In The Arabidopsis Book, Somerville C, Meyerowitz (eds) American Society of Plant Biologists Available at: http://www.aspb.org/publications/arabidopsis.
- Werck-Reichhart D, Hehn A, Didierjean L. (2000). Cytochromes P450 for engineering herbicide tolerance. Trends Plant Sci, 5, 116–123.
- Yamada T, Kambara Y, Imaishi H, Ohkawa H. (2000). Molecular cloning of novel cytochrome P450 species induced by chemical treatments in cultured tobacco cells. Pest Biochem Physiol, 68, 11–25.
- Zhang Y. (2009). I-TASSER: Fully automated protein structure prediction in CASP8. Proteins, 77 Suppl 9, 100–113.
- Zhang Y, Liu J. (2011). Transgenic alfalfa plants co-expressing glutathione S-transferase (GST) and human CYP2E1 show enhanced resistance to mixed contaminates of heavy metals and organic pollutants. J Hazard Mater, 189, 357–362.