Abstract
Context: Bacopa monniera L. (Scrophulariaceae) is used as a traditional medicine in India for various ailments such as epilepsy, mental disorders, and also as a cardio-tonic. However, its nephroprotective role is still unknown.
Objective: The present study assesses the modulatory impact of the alcoholic (ethanol) extract of Bacopa monniera (AEBM) on renal oxido-lipidemic stress in hypercholesterolemic rats.
Materials and methods: B. monniera (1 kg) was extracted with 90% ethanol, filtered, and dried (52 g). Group-I rats as control, Group-II rats fed with a hypercholesterolemic diet (HCD) for 45 d [4% cholesterol and 1% cholic acid], Group-III rats fed with HCD for 45 d + AEBM (40 mg/kg, body weight) for last 30 d, and Group-IV AEBM alone rats. Blood and kidney were removed to analyze lipid, antioxidant status, and histological analysis.
Result: The levels of total cholesterol (TC), triacylglycerol (TG), phospholipids (PLs), renal functional parameters (urea, creatinine, and uric acid), and lipid peroxidation (LPO) products were significantly attenuated (p < 0.01) in AEBM-treated hypercholesterolemic rats. Activities of both enzymic (superoxide dismutase (SOD), catalase (CAT), glutathione peroxidase (GPx), glutathione-S-transferase (GST), and glutathione reductase (GR)) and non-enzymic antioxidant (GSH, Vit-C, and Vit-E) were significantly increased (p < 0.01), on supplementation with AEBM. Administration with AEBM the mRNA levels of eNOS and iNOS genes was significantly up-regulated and down-regulated (p < 0.01). Histomorphological observations also evidenced that AEBM effectively protects the kidney from hypercholesterolemia-mediated oxido-lipidemic damage.
Discussion and conclusion: From this study, we hypothesized that AEBM can act as renoprotective agent by attenuating the renal oxido-lipidemic stress via regulating NOS level and thereby protects the nephron in hypercholesterolemic rats.
Introduction
Hypercholesterolemia and hyperlipidemia have been demonstrated to be the major risk factors for the progression of various renal diseases (Arora et al., Citation2010). The relationship between hypercholesterolemia and renal damage has been well documented for the past few decades (Chade et al., Citation2007; Montilla et al., Citation2006). Several studies have shown that the abnormal lipid metabolism (elevated lipid level) acts as an integral factor rather than primary initiator in modulating progressive renal damage (Deji et al., Citation2009; Feldstein et al., Citation1999). It is increasingly recognized that reactive oxygen species (ROS) and proinflammatory cytokines are involved in the development of renal damage induced by hypercholesterolemia (Ying et al., Citation2005). ROS produce tissue injury by initiating lipid, protein, and DNA oxidative modifications, owing to elevated oxidative stress (imbalance between oxidant and antioxidant) which could be involved in hypercholesterolemia-induced renal vasculopathies (Parthasarathy et al., Citation2000).
Several studies have shown that hypercholesterolemia usually results in oxidative stress and since Bacopa monniera L. (Scrophulariaceae) saponins, flavonoids, alkaloids, and phytosterol are potent antioxidants, they could be explored as possible supplements for treating hypercholesterolemia-induced oxidative stress in renal tissue, hence B. monniera was chosen for the present study. B. monniera (locally called Brahmi) has been used in Ayurvedic medicine to treat asthma, hoarseness, mental disorders, improve mental performance, nervine tonic, cardiotonic, and diuretic (Chopra et al., Citation1956). It also used for inflammation, pain, pyrexia, epilepsy, and as a sedative (Russo & Borrelli, Citation2005). The plant has been shown to possess a potent free radical scavenging and antioxidant properties (Tripathi et al., Citation1996). Besides, it also exhibits cardio-protective (Mohanty et al., Citation2010), vasodilatory (Channa et al., Citation2003), anti-inflammatory (Channa et al., Citation2006), hepatoprotective (Sumathi & Nongbri, Citation2008), antiulcer (Sairam et al., Citation2001), and anti-addictive (Sumathi & Niranjali Devaraj, Citation2009) properties.
The novelty of the present study is to explore the renoprotective potential of alcoholic extract of B. monniera (AEBM) by analyzing renal lipid status [TC, triacylglycerol (TG), and PLs], antioxidant profile (both enzymic and non-enzymic antioxidants), oxidative marker-lipid peroxidation (LPO), renal markers (urea, creatinine, and uric acid), nitric oxide synthase levels as well as histomorphology on oxido-lipidemic-induced hypercholesterolemic rats.
Materials and methods
Collection of plant material and extraction procedure
Bacopa monniera was collected from Chennai, India, on 30 March 2010. The plant was authenticated by Dr. A. Sasikala, Botanist, Captain Srinivasa Murthi Drug Research Institute for Ayurveda, Arumbakkam, Chennai, India. A voucher specimen (BOTM-7) was deposited in the herbarium of the same research Institute. The shade-dried and coarsely powdered whole plant material (1 kg) was extracted with 90% ethanol in the cold (48 h). The extract was filtered and distilled on a water bath to get a dark green syrupy mass. It was finally dried in vacuo (52 g). The alcoholic extract of B. monniera (AEBM) was given orally as a suspension based on appropriate concentration in experimental design.
Experimental animals
Twenty-four male albino rats of the Wistar strain weighing 200 ± 10 g were used for the current study. The animals were procured from the Central Animal House Block, Dr. ALM PG IBMS, University of Madras, Taramani Campus, Chennai. The animals were housed in large spacious cages. Food and water were given ad libitum. Rats were allowed to adapt to their environment condition for at least 10 d before the initiation of experiment. Animal experiments were conducted according to the guidelines of the Institutional Animal Ethics Committee (IAEC No: 01/09/12). Animals were randomly divided into four groups of six rats each.
Group I served as control rats fed with normal diet, Group II rats fed with hypercholesterolemic diet (HCD) for 45 d [rat chow supplemented with 4% cholesterol (w/w) and 1% cholic acid (w/w)], Group III rats fed with HCD for 45 d (Sudhahar et al., Citation2008) in addition to receiving AEBM (40 mg/kg, body weight/day orally) for the last 30 d, and Group IV rats were fed with normal diet for 45 d along with AEBM (40 mg/kg, body weight/day orally) (Kamesh & Sumathi, Citation2012a) for last 30 d.
On the 46th day, all the animals were sacrificed by cervical decapitation under sodium pentobarbitone (60 mg/kg) anesthesia. The renal tissue with renal artery were excised immediately, washed with ice-cold saline and then dried with filter paper. The slice of renal tissue was fixed with 10% formalin and stained with hematoxylin and eosin stain for histopathological studies. A 10% homogenate of renal tissue and artery were prepared by using 0.1 M Tris HCl buffer pH 7.4. Blood was collected in a tube without anticoagulant for the separation of serum. The above said samples were used for biochemical analysis.
Biochemical analysis
Renal total lipids (TL) were extracted in chloroform:methanol (2:1) ratio and purified by Folch’s wash procedure (Folch et al., Citation1957) and aliquot were used for the estimation of renal total cholesterol (TC) (Parekh & Jung, Citation1970), triglyceride (Rice, Citation1970), and phospholipids (PLs) (Rouser et al., Citation1970 with slight modification) according to the above-mentioned method. The renal antioxidant enzymes, namely, superoxide dismutase (SOD) (Marklund & Marklund, Citation1974), catalase (CAT) (Sinha et al., Citation1972), glutathione peroxidase (GPx) (Rotruck et al., Citation1973), glutathione reductase (GR) (Stall et al., Citation1969), glutathione-S-transferase (GST) (Habig et al., Citation1974), glutathione content (Moron et al., Citation1979), vitamin C (Omaye et al., Citation1979), and vitamin E (Baker & Frank, Citation1951) were estimated according to the reported methods. The LPO in the renal tissue was measured by method of Devasagayam and Tarachand (Citation1987). Serum creatinine, urea, and uric acid were determined using commercial kits (Roche Diagnostics Corporation, Basel, Switzerland) according to manufactures instructions. Protein was estimated by the method of Lowry et al. (Citation1951) method.
Reverse transcriptase-polymerase chain reaction (RT-PCR) analysis
Total RNA was isolated from the renal artery using Trizol Reagent (Invitrogen, Carlsbad, CA) according to the manufacturer’s instructions. The quality and integrity of RNA were confirmed by spectrophotometric analysis (OD260/280). RT-PCR for endothelial NOS (eNOS) and inducible NOS (iNOS), mRNA expression was done according to manufacturer’s guidelines (Qiagen One Step RT-PCR mix). Briefly, the reaction mixture contained 10 µl of 5× Qiagen one step RT-PCR buffer containing final concentration of 2.5 mM MgCl2, 2 µl of dNTP mix, 5 µl of each sense and antisense primers of eNOS and iNOS, 5µl of sense and antisense primers of housekeeping β-actin (each of 0.6 µM final concentration), 1.0 µg of template RNA, 2 µl of Qiagen one step RT-PCR enzyme mix and made up to 50 µl with RNase free water. β-Actin was used as an internal control.
Primers were designed by primer3 software (Whitehead Institute for Biomedical Research, Cambridge, MA) with the following sequences: forward primer of eNOS was 5′-CACACTGCTAGAGGTGCTGGA-3′; the reverse primer was 5′-TGCTGAGCTGACAGAGTAGTA-3′. The forward primer of iNOS was 5′-GCAGGTTGAGGATTACTTCTTCCA-3′; the reverse primer was 5′-GCCCTTTTTTGCTCCATAGGAAA-3′. The forward primer of β-actin was 5′-AGCCATGTACGTAGCCATCC-3′; the reverse primer of β-actin was 5′-CTCTCAGCTGTGGTGGTGAA-3′ (MWG Biotech, Ebersberg, Germany). Amplification conditions were used in this study consisted of an initial denaturation at 94 °C for 5 min followed by denaturation at 94 °C for 2 min. Annealing at 58 °C 30 s and extension at 72 °C for 2 min for 30 cycles. The cycles were followed incubation at 72 °C for 7 min. To compare with the amount of steady state mRNA, 5 μl of each PCR product was resolved onto 2% agarose gel using TBE buffer after electrophoresis, the gel were viewed under UV light and digital images were captured on biorad gel documentation system. The expression of each target gene was standardized with internal control gene expression (β-actin) and represented as a ratio.
Histopathological studies
A portion of renal tissue was quickly removed from experimental rats and were fixed in 10% formalin, then dehydrated in the descending grades of isopropanol and xylene. The renal tissue was then embedded in molten paraffin wax and sectioned at 5 μm thickness and was stained with hematoxylin and eosin (H&E). The sections were then viewed under a light microscope (Nikon microscope ECLIPSE E400, model 115, Tokyo, Japan) for histopathological changes.
Statistical analysis
The values were expressed as mean ± SD (n = 6) for six animals in each group. Differences between each group were assessed by a one-way analysis of variance (ANOVA) using SPSS 17 version (SPSS Inc., Chicago, IL) and least significant difference (LSD) was determined using post hoc test at the level of p < 0.05.
Results
Effect of AEBM on renal lipid status
The levels of renal lipid status, TC, triglyceride (TG), PLs are shown in . Significant elevation (p < 0.01) in the levels of renal lipid profile such as TC, TG, and PLs in HCD fed rats, as compared with control rats. Treatment with AEBM substantially decreased (p < 0.01) the levels of renal TC, TG, and PLs when equivalence to the HCD group.
Effect of AEBM on the activities of renal enzymic and non-enzymic antioxidant
The activities of renal tissue antioxidant enzymes, namely, SOD, CAT, GPx, GST, and GR enzymes, and non-enzymic antioxidant, namely, glutathione contents (GSH), vitamin C, and vitamin E were significantly reduced (p < 0.01) in HCD-induced rats, when matched to normal rats ( and ). Oral administration with AEBM brought back the activities of both enzymic and non-enzymic antioxidants to near normal.
Table 1. Effect of AEBM on the activities of renal enzymic antioxidant of HCD-induced hypercholesterolemia in control and experimental rats.
Table 2. Effect of AEBM on the activities of renal non-enzymic antioxidant of HCD-induced hypercholesterolemia in control and experimental rats.
Effect of AEBM on the levels of renal LPO
The levels of renal LPO of HCD-induced control and experimental rats are illustrated in . A significant increase (p < 0.01) in LPO levels of renal tissues were observed in HCD fed groups as compared with the control group. Treatment with AEBM caused a substantial decline (p < 0.05) in the levels of LPO products, when compared with untreated hypercholesterolemic rats.
Figure 2. Effect of AEBM on the levels in renal LPO of HCD-induced hypercholesterolemia in control and experimental rats. Values were expressed as mean ± S.D. for six rats in each group. Statistical significance (p value): *p < 0.05, **p < 0.01, (a) compared with the control group, (b) compared with the HCD group.
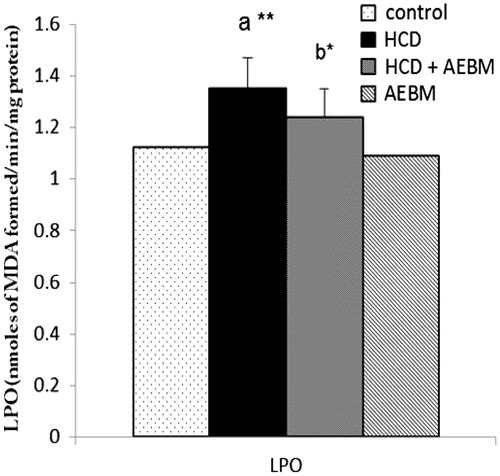
Effect of AEBM on the levels of urea, creatinine, and uric acid (renal functional markers) in serum
summarizes the levels of renal markers in serum of HCD-induced control and experimental rats. There was a concomitant elevation (p < 0.01) in the levels of renal markers such as urea, creatinine, and uric acid were observed in HCD-induced rats in comparison with control rats. AEBM treatment significantly (p < 0.01) restored the activity of urea, uric acid, and creatinine to near normal levels. AEBM alone-treated group did not show any significant change in renal lipid profile, antioxidant status, and renal functional parameters as compared with normal rats.
Table 3. Effect of AEBM on the levels of urea, creatinine, and uric acid (renal markers) in serum of HCD-induced hypercholesterolemia in control and experimental rats.
Effect of AEBM on the mRNA expression of NOS in renal artery
and show the mRNA expression of eNOS and iNOS in renal artery of HCD-induced control and experimental rats. The mRNA expression levels of eNOS and iNOS gene were significantly decreased (p < 0.05) and increased (p < 0.01), respectively, in the renal artery of HCD-induced rats (lane 3) as compared with normal rats (lane 2). Oral treatment with AEBM substantially up-regulated and down-regulated (p < 0.01) the mRNA expression levels of eNOS and iNOS, respectively, in renal artery (lane 4) when compared with HCD rats (lane 3). AEBM alone-treated rats do not show any changes in mRNA expression levels of eNOS and iNOS.
Figure 3. Effect of AEBM on mRNA expression levels of eNOS in renal artery of control and experimental rats. Lane 1 represents the DNA marker; lane 2 represents the control group; lane 3 represents the HCD group; lane 4 represents the HCD + AEBM treatment group; lane 5 represents the AEBM alone group. Densitometric analysis of the bands is expressed as net intensity ratio corrected for the corresponding β-actin contents. Values were expressed as mean ± S.D. for six rats in each group. Statistical significance (p value): *p < 0.05, **p < 0.01, (a) compared with the control group, (b) compared with the HCD group.
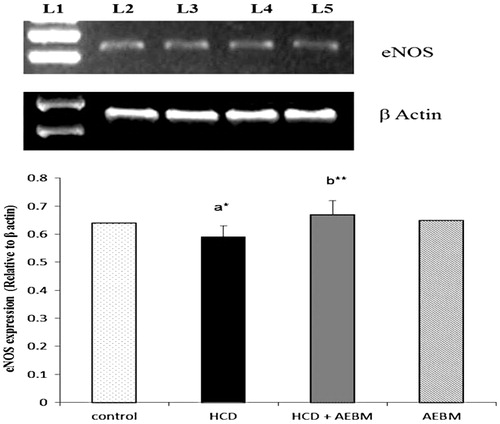
Figure 4. Effect of AEBM on mRNA expression levels of iNOS in renal artery of control and experimental rats. Lane 1 represents the DNA marker; lane 2 represents the control group; lane 3 represents the HCD group; lane 4 represents the HCD + AEBM treatment group; lane 5 represents the AEBM alone group. Densitometric analysis of the bands is expressed as net intensity ratio corrected for the corresponding β-actin contents. Values were expressed as mean ± S.D. for six rats in each group. Statistical significance (p value): **p < 0.01, (a) compared with the control group, (b) compared with the HCD group.
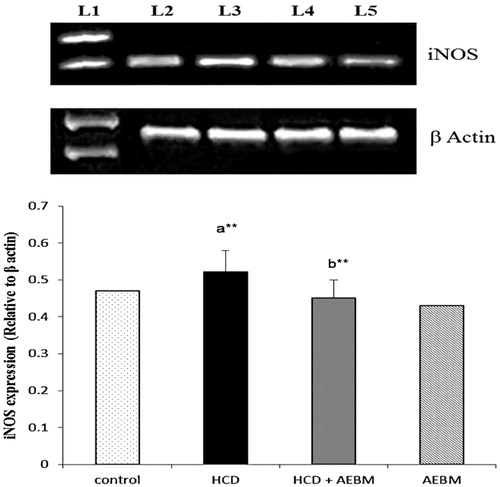
Effect of AEBM on histopathological changes in renal tissue
portraits the histopathological changes in renal tissue of HCD-induced control and experimental rats. Transection of renal tissue (control) rendered normal architecture of glomeruli and tubules of nephron (H&E, 100×) (). Transection of HCD-induced rats depicts swelling of renal tubules and tubular epithelial denudation with casts in the widened lumens (glomerular hypertrophy) (). Transection of AEBM + HCD rats portrait mild tubular epithelial damage when compared with HCD rats (), when compared with HCD rats. AEBM alone-treated group () showed no changes in the morphology/structure of renal tissue (H&E, 100×).
Figure 5. Effect of AEBM on histopathological changes in renal tissue of HCD-induced hypercholesterolemia in control and experimental rats. Transection of renal tissue (control) showed normal architecture of glomeruli and tubules of nephron (H&E, 100×) (). Transection of HCD-induced rats depict swelling of renal tubules and tubular epithelial denudation with casts in the widened lumens (glomerular hypertrophy) of renal tissue (). Transection of AEBM + HCD rats showed mild tubular epithelial damage when compared with HCD rats (), when compared with HCD rats. AEBM alone ()-treated rats showed normal renal architecture similar to that of control rats (H&E, 100×) (scale bar: 50 µm).
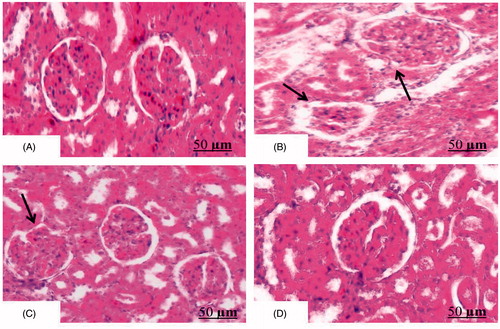
AEBM alone (Group IV)-treated rats showed normal renal architecture which is similar to that of control rats.
Discussion
The aim of the present study was to assess the modulatory impact of alcoholic extract of B. monniera (AEBM) on renal lipid levels, antioxidant profile, renal markers, mRNA expression of NOS levels, and renal morphology in high-cholesterol-induced rats. It has been convincingly demonstrated that rats with chronic hyperlipidemia and hypercholesterolemia develop glomerulosclerosis in kidneys without immune complex disease (Grone et al., Citation1994; Saini et al., Citation2004; Scheuer et al., Citation2000). In our present study, we found that there was a significant elevation in the levels of renal lipid profile such as TC, TG, and PLs in HCD fed rats, as compared with normal rats owing to increased absorption of lipids (plasma) which reflect in elevated renal lipid levels. These results are well supported by many previous studies (Al-Rejaie et al., Citation2012; Sudhahar et al., Citation2008). Oral administration of AEBM significantly decreases the levels of TC, TG, and PLs when compared with HCD-induced rats which may be due to hypocholesterolemic effect (Kamesh & Sumathi, Citation2012b).
It was postulated that cholesterol/lipid-induced renal injury might occur due to increased oxidative stress (imbalance between oxidant and antioxidant enzyme activities) in renal tissue (Salem & Salem, Citation2011). In the animal model, feeding of high-cholesterol diet produces severe hypercholesterolemia and glomerular lesion by increased oxidative stress in kidney (Akpolat et al., Citation2011; Ishiyama et al., Citation1999). Several reports have shown that hypercholesterolemia deteriorate the antioxidant defense systems of various organs especially renal tissue (Deepa & Varlakshmi, Citation2003; Green et al., Citation2012). During HCD condition, the activities of renal antioxidant enzymes, namely, SOD, CAT, GPx, GST, and GR enzymes and non-enzymic antioxidant, namely, glutathione contents, vitamin C, and vitamin E, were substantially suppressed. These results were parallel to the previous report that during HCD condition there is a concomitant increase in the levels of free radicals (ROS) which leads to oxidative stress and finally ends up in diminished activities of these antioxidant enzymes (Salem & Salem, Citation2011; Sudhahar et al., Citation2008).
On treatment with AEBM, the activities of these antioxidant enzymes in renal tissue were reverted back to near normal. Earlier it has been reported that AEBM has antioxidant activity owing to the presence of its saponins, flavonoids, and phytosterol (Tripathi et al., Citation1996) and hence antioxidants attenuate the hypercholesterolemic-induced oxidative stress in animal models, mainly due to their free radical scavenging capabilities. Martinet and coworkers (Citation2001) have demonstrated that high-cholesterol diet (HCD) induces ROS overproduction which could in turn initiate LPO. During the HCD condition, there is a pronounced increase in ROS generation which in turn converts the normal LDL to OxLDL which is an important contributory factor for glomerulosclerosis (Deepa & Varlakshmi, Citation2005). In our current study, we found that a concomitant elevation in LPO levels was observed in HCD-fed groups compared to the normal group, because of increased ROS generation. These results are compatible with several previous studies (Chenni et al., Citation2007; Green et al., Citation2012). AEBM treatment restored the level of LPO and equivalence to normal rats due to its antioxidant capacity.
Numerous investigations have suggested the important role of abnormal lipid metabolism (high-cholesterol level) as an integral factor in progressive renal damage (Feldstein et al., Citation1999; Ying et al., Citation2005). Scheuer and his colleague (Citation2000) reported that oxidative stress is a pathogenetic factor in lipid-induced nephropathy. Nephrons are the basic unit of renal tissue and used for filtering blood especially for excretion of urea, uric acid, and creatinine (Yadav & Srivastava, Citation2013). During the HCD condition a greater amount of ROS is generated which in turn damage nephrons (depletion in nephron count) which leads to a decreased filtration rate and thereby increasing the levels of renal functional parameters (markers) such as urea, uric acid, and creatinine in serum and hence the level of marker enzymes was notably elevated in HCD-fed rats. Similar observations were reported earlier in hypercholesterolemic rats (Akpolat et al., Citation2011; Montilla et al., Citation2006). Oral supplementation with AEBM significantly reduced the levels of urea, uric acid, and creatinine because of its antioxidant activity thus protecting nephron from ROS-induced damage.
Hypercholesterolemia has been found to decrease nitric oxide (NO) availability in the renal circulation and renal artery (Stulak et al., Citation2001). NO is reported to play an important role in the body and be involved in the control of renal function (Plotnikov et al., Citation2009). Especially in health status, endothelial NOS (eNOS) is a protective NO-generating enzyme and contributes to the maintenance of the normal renal structure (Sandovici et al., Citation2004). NO derived from eNOS usually plays a protective effect. However, NO from iNOS might be implicated in renal injury (Tain et al., Citation2008). Over-expression of iNOS and excessive NO are proposed as one of the causes of vascular dysfunction in kidney by activating inflammatory cytokines (Bhatia et al., Citation2003). Renal nitric oxide (NO) levels are significantly decreased and peroxynitrite levels are significantly enhanced in cholesterol-fed animal models (Amin et al., Citation2011; Feldstein et al., Citation1999). In agreement with the above statement, we found marked down-regulation and up-regulation of mRNA expression in the levels of both eNOS and iNOS gene, respectively, in renal artery of hypercholesterolemic-induced rats, it might be because of enhanced production of superoxide (O2−), which then reacts with NO to form peroxynitrite (ONOO−) and thereby decreases NO levels as well as increases oxidation of LDL (oxLDL). oxLDL is suggested to be a potential regulator of NO generation. It has the capacity of regulating eNOS and iNOS activities via inhibiting Akt-mediated eNOS serine 1177 phosphorylation, preventing eNOS–Hsp90 interaction, up-regulating caveolin (Cav-1) and activating endothelial arginase II (Arg II) (Li & Forstermann, Citation2013). The overexpression of iNOS may result in a reduced expression of eNOS (Wessells et al., Citation2006). Consequently, the iNOS up-regulation most likely contributes to eNOS reduction in the present model as well (both are inversely proportional to each other).
However, on treatment with AEBM, substantial up-regulation and down-regulation in mRNA expression levels of eNOS (maintain normal NO level) and iNOS (decrease inflammatory cytokines level) were noted in renal artery when compared with HCD-induced rats, owing to its antioxidant property (SOD) and thereby suppression of the production of superoxide (O2−) radical as well as attenuating the level of oxLDL production in the renal artery of AEBM-treated hypercholesterolemia rats. Thus, the renal protective effect of AEBM was associated with the activity of regulating the NO signaling pathway due to its antioxidant capacity in hypercholesterolemic rats.
Hypercholesterolemia and the resulting atherosclerosis have been implicated in the pathophysiology of glomerulosclerosis (Ishiyama et al., Citation1999; Ricardo et al., Citation1997). Histological examination of the control renal tissue depicted normal glomeruli and tubules of nephron (H&E, 100×) (). In the case of HCD-induced rats, portraits mild swelling of renal tubules and tubular epithelial denudation with casts in the widened lumens (glomerular hypertrophy). In the previous section, we also reported that increase in renal markers was due to increased nephron damage by free radical produced during HCD condition which might be the reason for this significant morphological alteration in renal tissue. Our results are well correlated with previous studies (Ishiyama et al., Citation1999; Sudhahar et al., Citation2008; Ying et al., Citation2005). On treatment with AEBM (), renal tissue showed mild tubular epithelial damage when compared with HCD rats due to its free radical scavenging activity. AEBM alone-treated rats () showed normal renal architecture similar to that of control rats.
Conclusion
From the above results, we conclude that consumption of AEBM significantly regulates renal lipid levels, oxidative stress, renal markers, mRNA expression of NOS, and morphology of renal tissue mainly due to its hypocholesterolemic and antioxidant properties and we hypothesized that AEBM can effectively alleviate the renal oxido-lipidemic injury associated with hypercholesterolemia. Further investigations are recommended to ascertain the mode of mechanism behind the renoprotective effect of B. monniera during the hypercholesterolemic condition.
Declaration of interest
The authors declare that there is no declaration of interest to disclose. The financial support extended by UGC in the form of JRF for meritorious students under UGC-SAP Programme is gratefully acknowledged.
References
- Akpolat M, Kanter M, Topcu-Tarladacalisir Y, Aydogdu N. (2011). Protective effect of flaxseed oil on renal injury in hyperlipidaemic rats, the effect of flaxseed oil on hyperlipidaemia. Phytother Res 25:796–802
- Al-Rejaie SS, Abuohashish HM, Alkhamees OA, et al. (2012). Gender difference following high cholesterol diet induced renal injury and the protective role of rutin and ascorbic acid combination in Wistar albino rats. Lipids Health Dis 11:41–50
- Amin KA, Kamel HH, Abd Eltawab MA. (2011). Protective effect of Garcinia against renal oxidative stress and biomarkers induced by high fat and sucrose diet. Lipids Health Dis 10:6–19
- Arora MK, Reddy K, Balakumar P. (2010). The low dose combination of fenofibrate and rosiglitazone halts the progression of diabetes-induced experimental nephropathy. Eur J Pharmacol 636:137–44
- Baker AF, Frank G. (1951). Estimation of vitamin E in tissues. In: Bollinger G, ed. Dunnshchicht, Chromatographic in Laboratorium Hand brich. Berlin, Germany: Springer-Verlag, 41–52
- Bhatia S, Shukla R, Venkata-Madhu S, et al. (2003). Antioxidant status, lipid peroxidation and nitric oxide end products in patients of type 2 diabetes mellitus with nephropathy. Clin Biochem 36:557–62
- Chade AR, Krier JD, Galili O, et al. (2007). Role of renal cortical neovascularization in experimental hypercholesterolemia. Hypertension 50:729–36
- Channa S, Dar A, Anjum S, et al. (2006). Anti-inflammatory activity of Bacopa monniera in rodents. J Ethnopharmacol 104:286–9
- Channa S, Dar A, Yaqoob M, et al. (2003). Broncho-vasodilatory activity of fractions of pure constituents isolated from Bacopa monniera. J Ethnopharmacol 86:27–35
- Chenni A, Yahia DA, Boukortt FO, et al. (2007). Effect of aqueous extract of Ajuga iva supplementation on plasma lipid profile and tissue antioxidant status in rats fed a high-cholesterol diet. J Ethnopharmacol 109:207–13
- Chopra RN, Nayar SL, Chopra IC. (1956). Glossary of Indian Medicinal Plants. New Delhi: CSIR, 32
- Deepa PR, Varalakshmi P. (2003). Salubrious effect of low molecular weight heparin on atherogenic diet-induced cardiac, hepatic and renal lipid peroxidation and collapse of antioxidant defences. Mol Cell Biochem 254:111–16
- Deepa PR, Varalakshmi P. (2005). Atheroprotective effect of endogenous heparin-derivative treatment on the aortic disturbances and lipoprotein oxidation in hypercholesterolemic diet fed rats. Clin Chim Acta 355:119–30
- Deji N, Kume S, Araki S, et al. (2009). Structural and functional changes in the kidneys of high-fat diet-induced obese mice. Am J Physiol Renal Physiol 296:F118–26
- Devasagayam TPA, Tarachand U. (1987). Decreased lipid peroxidation in rat kidney during gestation. Biochem Biophys Res Commun 145:134–8
- Feldstein A, Krier JD, Sarafov MH, et al. (1999). In vivo renal vascular and tubular function in experimental hypercholesterolemia. Hypertension 34:859–64
- Folch J, Lees M, Sloane-Stanley GH. (1957). A simple method for isolation and purification of total lipids from animal tissues. J Biol Chem 226:497–509
- Green CO, Wheatley AB, McGrowder DA, et al. (2012). Modulation of antioxidant enzymes activities and lipid peroxidation products in diet-induced hypercholesterolemic rats fed ortanique peel polymethoxylated flavones extract. J Appl Biomed 10:91–101
- Grone EF, Walli AK, Grone HJ, et al. (1994). The role of lipids in nephrosclerosis and glomerulosclerosis. Atherosclerosis 107:1–13
- Habig WH, Pabst MJ, Jakoby WB. (1974). Glutathione-S-transferases: The first enzymatic step in mercapturic acid formation. J Biol Chem 249:7130–9
- Ishiyama A, Atarashi K, Minami M, et al. (1999). Role of free radicals in the pathogenesis of lipid-induced glomerulosclerosis in rats. Kidney Int 55:1348–58
- Kamesh V, Sumathi T. (2012a). Effect of Bacopa monniera Linn. in attenuating hepatic oxidative stress on hypercholesterolemic induced rats. Asian J Pharm Clin Res 5:90–5
- Kamesh V, Sumathi T. (2012b). Anti-hypercholesterolemic effect of Bacopa monniera Linn. on high cholesterol diet induced hypercholesterolemia in rats. Asian Pac J Trop Med 949–55
- Li H, Forstermann U. (2013). Uncoupling of endothelial NO synthase in atherosclerosis and vascular disease. Curr Opin Pharmacol 13:161–7
- Lowry OH, Rosebrough NJ, Farr AL, Randall R. (1951). Protein measurement with Folin’s phenol reagent. J Biol Chem 193:265–75
- Marklund S, Marklund G. (1974). Involvement of the superoxide anion radical in the autoxidation of pyrogallol and a convenient assay for superoxide dismutase. Eur J Biochem 47:469–74
- Martinet W, Knaapen MW, De Meyer GR, et al. (2001). Oxidtive DNA damage and repair in experimental atherosclerosis are reversed by dietary lipid lowering. Circ Res 88:733–9
- Mohanty IR, Maheshwari U, Joseph D, Deshmukh Y. (2010). Bacopa monniera protects rat heart against ischaemia-reperfusion injury: Role of key apoptotic regulatory proteins and enzymes. J Pharm Pharmacol 62:1175–84
- Montilla P, Espejo I, Munoz MC, et al. (2006). Protective effect of red wine on oxidative stress and antioxidant enzyme activities in the brain and kidney induced by feeding high cholesterol in rats. Clin Nutr 25:146–53
- Moron MS, Depierre JW, Mannervik B. (1979). Levels of glutathione reductase and glutathione S-transferase activities in rat lung and liver. Biochim Biophys Acta 582:67–78
- Omaye ST, Turnbull JD, Sauberlich HE. (1979). Selected methods for the determination of ascorbic acid in animal cells, tissues and fluids. Meth Enzymol 62:3–11
- Parekh AC, Jung DH. (1970). Cholesterol determination with ferric chloride–uranium acetate and sulfuric acid–ferrous sulfate reagents. Anal Chem 42:1423–7
- Parthasarathy S, Santanam N, Ramachandran S, Meilhac O. (2000). Potential role of oxidized lipids and lipoproteins in antioxidant defense. Free Radic Res 33:197–215
- Plotnikov EY, Chupyrkina AA, Pevzner IB, et al. (2009). Myoglobin causes oxidative stress, increase of NO production and dysfunction of kidney’s mitochondria. Biochim Biophys Acta 1792:796–803
- Ricardo SD, Van Goor H, Diamond JR. (1997). Hypercholesterolemia and progressive kidney disease, the role of macrophages and macrophage-derived products. Contrib Nephrol 120:197–209
- Rice EW. (1970). Triglycerides (“neutral fat”) in serum. Stand Meth Clin Chem 6:215–22
- Rotruck JT, Pope AL, Ganther HE. (1973). Selenium: Biochemical role as a component of glutathione peroxidase. Science 179:588–90
- Rouser G, Fkeischer S, Yamamoto A. (1970). Two dimensional then layer chromatographic separation of polar lipids and determination of phospholipids by phosphorus analysis of spots. Lipids 5:494–6
- Russo A, Borrelli F. (2005). Bacopa monniera, a reputed nootropic plant, an overview. Phytomedicine 12:305–17
- Saini HK, Arneja AS, Dhalla NS. (2004). Role of cholesterol in cardiovascular dysfunction. Can J Cardiol 20:333–46
- Sairam K, Rao CV, Babu MD, Goel RK. (2001). Prophylactic and curative effects of Bacopa monniera in gastric ulcer models. Phytomedicine 8:423–30
- Salem NA, Salem EA. (2011). Renoprotective effect of grape seed extract against oxidative stress induced by gentamicin and hypercholesterolemia in rats. Ren Failure 33:824–32
- Sandovici M, Henning RH, Hut RA, et al. (2004). Differential regulation of glomerular and interstitial endothelial nitric oxide synthase expression in the kidney of hibernating ground squirrel. Nitric Oxide 11:194–200
- Scheuer H, Gwinner W, Hohbach J, et al. (2000). Oxidant stress in hyperlipidemia-induced renal damage. Am J Physiol Renal Physiol 2000 278:F63–74
- Sinha AK. (1972). Colorimetric assay of catalase. Anal Biochem 47:389–94
- Stall GEJ, Visser J, Veeger C. (1969). Purification and properties of glutathione reductase of human erythrocytes. Biochim Biophys Acta 185:39–48
- Stulak JM, Lerman A, Caccitolo JA, et al. (2001). Impaired renal vascular endothelial function in vitro in experimental hypercholesterolemia. Atherosclerosis 154:195–201
- Sudhahar V, Kumar SA, Varalakshmi P, Sujatha V. (2008). Protective effect of lupeol and lupeol linoleate in hypercholesterolemia associated renal damage. Mol Cell Biochem 317:11–20
- Sumathi T, Niranjali Devaraj S. (2009). Effect of Bacopa monniera, on liver and kidney toxicity in chronic use of opioids. Phytomedicine 16:897–903
- Sumathi T, Nongbri A. (2008). Hepatoprotective effect of bacoside-A, a major constituent of Bacopa monniera Linn. Phytomedicine 15:901–5
- Tain YL, Muller V, Szabo AJ, et al. (2008). Renal cortex neuronal nitric oxide synthase in response to rapamycin in kidney transplantation. Nitric Oxide 18:80–6
- Tripathi YB, Chaurasia S, Tripathi E, et al. (1996). Bacopa monniera Linn. as an antioxidant: Mechanism of action. Indian J Exp Biol 34:523–6
- Wessells H, Teal TH, Luttrell IP, Sullivan CJ. (2006). Effect of endothelial cell-based iNOS gene transfer on cavernosal eNOS expression and mouse erectile responses. Int J Imp Res 18:438–45
- Yadav YC, Srivastava DN. (2013). Nephroprotective and curative effects of Ficus religiosa latex extract against cisplatin-induced acute renal failure. Pharm Biol 51:1480–5
- Ying Y, Xing-kui T, Xiao-cheng L, Ju-fang S. (2005). Early renal morphological changes in high cholesterol diet rats model. J Nat Sci 10:1063–8