Abstract
An isolation procedure utilizing ammonium sulfate fractionation and affinity chromatography was used to purify an elastase inhibitor present in large amounts in marama beans (Tylosema esculentum). The protein appeared to be heterogeneous due to carbohydrate differences, demonstrating two bands on SDS gels with molecular weights of 17.8 kDa and 20 kDa. Partial sequence, derived from mass spectrometry, indicated that the protein is a Kunitz-type inhibitor distinct from other known plant serine protease inhibitors. The marama bean inhibitor is specific for elastase, with very low Ki for both pancreatic and neutrophil elastase. The quantity of elastase inhibitor present in marama beans is many times greater than in soybean or any other bean or nut source reported to date. This raises the question of why a bean found in an arid corner of the Kalahari Desert would be so rich in a very potent elastase inhibitor.
Introduction
Protease inhibitors in plants play an important role in regulation of growth and development, response to stress, and defense against insects and pathogens. (To avoid confusion, the term “protease”—which includes both endopeptidases and exopeptidases—has been used throughout this report.) As early as 1947 it was observed that the larvae of certain insects could not develop normally when grown on soybean products. Subsequently, soybean trypsin inhibitor was shown to be toxic to the larvae of the flour beetleCitation1. Since then, there have been many examples of protease inhibitors in different plant tissues, including leaves, flowers, seeds, and roots, exhibiting a protective effect against specific insect species, both in vitroCitation1–6 and in vivoCitation7,Citation8. These inhibitors are thought to be involved primarily in defense against herbivores, which rely on proteases to digest the proteins they consume, and for protection against wounds inflicted by insectsCitation9,Citation10. Protease inhibitors moderate the growth and survival of many insect herbivores when included in artificial diets, and reduce insect feeding rate and performance when expressed in transgenic plantsCitation11,Citation12. In seeds, where protease inhibitors are often found in high concentrations, they keep the plants’ own proteases in check to prevent degradation of storage proteinsCitation10. Serine proteases have been identified in the digestive tracts of insects from many families, particularly those of lepidopteraCitation13, and many plant inhibitors have been found to be active against these gut proteasesCitation2,Citation3. These protease inhibitors also exhibit a very broad spectrum of activity against other pathogens including: pathogenicnematodes such as Globodera tabaccum, G. pallida, and Meloidogyne incognitaCitation14; inhibition of spore germination and mycelium growth of Alternaria alternataCitation15; and growth of many pathogenic fungi including Trichoderma reeseiCitation16. Wound-inducible protease inhibitor proteins are present in plant leaves and soft tissue products such as tomatoes. When ingested by polyphagous insects, these inhibitors can interact with proteases of the insect midgut and lead to an arrest of growth and development, and deathCitation9,Citation17. Tissue engineering studies have shown that enhanced resistance to insects and pathogens results from overexpressing protease inhibitorsCitation9,Citation18,Citation19.
The marama bean, Tylosema esculentum, is a perennial tuberous legume that is native to the arid regions of southern AfricaCitation20,Citation21. The bean is an important supplement to the diet of tribes in the Kalahari Desert region where the bean is roasted, and then the hard, 2-mm-thick shell is removed prior to consumption, providing a valuable source of protein. The nutritional value of the marama bean is potentially quite high, being very similar to soybeanCitation22–24. There has been one study using young rats that were fed either raw or cooked marama bean meal contributing 10% of the protein in the dietCitation24. For both groups fed marama bean meal, food consumption was poor and the animals lost weight. A possible reason for the poor nutritive value of the marama bean in rats was thought to be the unusually high trypsin inhibitor level, which has been reported to be 2–4 times higher than found in soybeansCitation25. Subsequent studies indicated that there was also an elastase inhibitor in marama beansCitation26, and apparently the trypsin and elastase inhibitors were separate proteinsCitation27. The marama bean elastase inhibitor is a very potent inhibitor with low Ki for pancreatic elastase from mouse, rat, and pig as well as human neutrophil elastaseCitation28.
In the present study we further characterized the protease inhibitor present in marama beans and found unusually high levels of a specific elastase inhibitor that has sequence homology to the Kunitz family of protease inhibitors.
Materials and methods
Two sources of marama beans (Tylosema esculentum) were used: recently acquired samples were a generous gift from Dr. Chris Cullis at Case Western Reserve University; and 30-year-old beans from a previous studyCitation26. Other materials were obtained as follows: N-succinyl-(Ala)3-p-nitroanilide (SAPNA) and porcine pancreatic elastase, Elastin Products, Owensville, MO; N-benzoyl-l-arginine-ethyl-ester (BAEE), Nutritional Biochemical Corporation, Cleveland, OH; trypsin, Sigma Chemical Co., St. Louis, MO; Affigel-10, Bio-Rad Laboratories, Hercules, CA.
Inhibitor extraction
The inhibitor was extracted from marama beans using a modified Wagner and Riehm techniqueCitation29. The shell was removed from one bean, and the nut (1.7 g) was homogenized in 24 mL of 80% ethanol. The homogenate was extracted for 1 h at room temperature and the slurry centrifuged at 20,000 × g for 15 min. The supernatant containing the seed oil was discarded and the meal was extracted in 10 mL of 0.05M HCl for 1 h at room temperature and then centrifuged at 20,000 × g for 10 min at 4°C. The extraction was repeated three times to ensure the complete removal of acid soluble proteins. The four extracts were combined and brought to 80% ammonium sulfate saturation and stirred at 4°C for 1 h. The suspension was then centrifuged for 20 min at 9000 × g at 4°C. The supernatant was discarded and the precipitate was then dissolved in 5 mL of water, dialyzed for 24 h with repeated changes of water, and freeze-dried. For comparison, protease inhibitors were similarly extracted from raw soybeans, Brazil nuts, and Macadamia nuts using the same protocol.
Affinity chromatography
Porcine pancreatic elastase was linked to Affigel-10 according to the manufacturer’s instructions. Briefly, 5 mL of Affigel-10 was washed with water and reacted with 30 mg of porcine pancreatic elastase, and the gel equilibrated with 67 mM potassium phosphate buffer, pH 7.6. The freeze-dried extract from one marama bean (77 mg) was dissolved in 13 mL of the 67 mM phosphate buffer, centrifuged at 9000 × g for 10 min, filtered through a 0.2-μm syringe filter, and applied to the elastase-affinity column. The column was washed with 100 mL of the 67 mM phosphate buffer followed by 10 mL of the same buffer containing 0.5 M sodium chloride. The column was then re-equilibrated with water, and proteins bound to the column were eluted with 0.1 M sodium citrate buffer, pH 2.1. Fractions (2 mL) were collected, the absorbance at 280 nm was measured, and the fractions assayed for both anti-trypsin and anti-elastase activity as described below. Soybean trypsin inhibitor (5 mg) was also fractionated using the elastase affinity column. The inhibitor was dissolved in 67 mM potassium phosphate buffer, pH 7.6, and treated as described above for the marama bean extract.
Anti-elastase activity
Each fraction (7 μL) was added to a 96-well microwell plate, followed by addition of 8 μL of porcine pancreatic elastase (200 μg/mL in 0.001M HCl). The volume in each well was brought to 100 μL with 0.5 M Tris-HCl buffer, pH 8.8. The mixture was pre-incubated for 10 min at room temperature and then 8 μL of the substrate (5 mM SAPNA dissolved in 1-methyl-2-pyrrolidinone) was added. After a 15-min incubation at room temperature, absorbance at 410 nm was measured. To compare the quantity of inhibitor in extracts from different sources, we defined a unit of inhibitor as the amount that inhibits 50% of the activity of 1 μg of porcine pancreatic elastase in the standard microwell assay described above.
Anti-trypsin activity
Each fraction (7 μL) was added to a 96-well microwell plate, and then 1 μL of trypsin (1 mg/mL in 0.001 M HCl) was added to each well. The volume in each well was brought to 100 μL with 67 mM potassium phosphate buffer, pH 7.6. The mixture was pre-incubated for 10 min and then 4.6 μL of BAEE (10 mM in phosphate buffer) was added. Absorbance was measured at 253 nm in 30-s intervals for 5 min to assess the rate of proteolysis.
Gel electrophoresis
The isolated marama bean elastase inhibitor (10 μg) was dissolved in loading bufferCitation30, reduced with β-mercaptoethanol, and applied to an 8–12% gradient SDS (sodium dodecyl sulfate) gel. A molecular weight standard mixture (SeeBlue Plus2; Invitrogen Corp.) was run with each gel. Proteins were visualized with Coomassie blue. The bands at apparent molecular weights of 17.8 kDa and 20 kDa were excised and eluted with water for mass spectrometry analysis.
Mass spectrometry
Proteins in the gel bands were digested in situ with trypsin (Promega modified) in 40 mM NH4HCO3 at 37°C for 4 h. The digests were analyzed by MALDI-TOF-MS (matrix-assisted laser desorption/ionization-time of flight-mass spectrometry) and HPLC-ESI-MS/MS (high perfomance liquid chromatography-electrospray ionization-tandem MS). MALDI-TOF mass spectra were obtained on an Applied Biosystems Voyager-DE STR with positive ion detection in reflectron mode and α-cyano-4-hydroxycinnamic acid as matrix. HPLC-ESI tandem mass spectra were acquired on a Thermo Fisher LTQ linear ion trap mass spectrometer fitted with a New Objective PicoView 550 nanospray interface. On-line HPLC separation of the digests was accomplished with an Eksigent NanoLC micro HPLC: column, PicoFrit™ (New Objective; 75 μm i.d.) packed to 10 cm with C18 adsorbent (Vydac; 218MSB5, 5 μm, 300 å); mobile phase A, 0.5% acetic acid (HAc)/0.005% trifluoroacetic acid (TFA); mobile phase B, 90% acetonitrile/0.5% HAc/0.005% TFA; gradient 2–42% B in 30 min; flow rate, 0.4 μL/min. MS conditions were: ESI voltage, 2.9 kV; isolation window for MS/MS, 3; relative collision energy, 35%; scan strategy, survey scan followed by acquisition of data dependent collision-induced dissociation (CID) spectra of the seven most intense ions in the survey scan above a set threshold. The peptide mass maps and uninterpreted CID spectra were searched against the NCBInr database by means of Mascot (Matrix Science). Manual de novo sequencing of the tandem mass spectra was then conducted because no high-confidence identifications were found by the Mascot searches.
Results
Both sources of marama beans gave identical results during purification through the affinity step and appearance on SDS gels. Storage for 30 years at room temperature had no apparent ill effects on the structure or function of the elastase inhibitor. However, the data shown are from the fresher marama beans supplied by Dr. Cullis.
Marama beans are similar to soybeans in that they contain over 30% protein by weight (). This is in accordance with the observations of other investigatorsCitation22–24. Macadamia and Brazil nuts were comparable in texture to the marama bean yet contained much lower levels of protein. The quantity of protein in the ammonium sulfate fraction (containing the inhibitor) and the total units of elastase inhibitor were much greater in the marama bean than the Brazil and Macadamia nuts and soybeans used for comparison (). Even though the elastase inhibitor was present in relatively high concentrations, the trypsin inhibitor still represented 90% of the protein present in the ammonium sulfate fraction.
Table 1. Protein content and elastase inhibitor content of Macadamia nut, Brazil nut, soybean, and marama bean.
The protein and activity profiles of the trypsin and elastase inhibitors eluting from the affinity column in fractions 1–50 in the equilibration buffer are shown in . The majority of the protein eluted as a broad peak coinciding with the anti-trypsin activity. A small peak with elastase inhibitory activity eluted immediately following the anti-trypsin fractions. Subsequent elution with pH 2.2-citrate buffer (fractions 51–77) yielded a single, broad peak with anti-elastase activity and no detectable anti-trypsin activity. Fractions 60–70 were pooled, dialyzed against water, and freeze-dried, yielding approximately 10 mg of elastase-specific inhibitor.
Figure 1. Elution profile of elastase and trypsin inhibitory activity from marama bean extracts using an elastase affinity column. The column wash included fractions 1–50 and the citrate elution fractions 51–77. Protein quantity measured at 280 nm is illustrated by the dotted line, trypsin inhibitory activity by squares, and elastase inhibition by open circles. Each fraction was 2 mL. Inhibitory activity was expressed as a percent of the control with no added inhibitor.
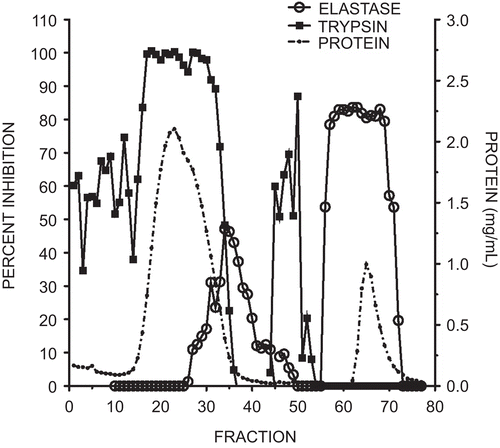
Soybean trypsin inhibitor applied to the elastase affinity column yielded a different separation pattern (). A large peak of protein with low anti-trypsin and anti-elastase activity eluted early followed by a broad peak containing high levels of anti-trypsin activity but no detectable elastase inhibition. Elution with pH 2.2-citrate buffer resulted in a low-intensity, broad peak that exhibited low levels of both trypsin and elastase inhibitory activity.
Figure 2. Elution profile of elastase and trypsin inhibitory activity from soybean trypsin inhibitor using an elastase affinity column. The column wash included fractions 1–50 and the citrate elution included fractions 51–77. Protein measured at 280 nm is illustrated by the dotted line, trypsin inhibitory activity by squares, and elastase inhibition by open circles. Each fraction was 2 mL. Inhibitory activity was expressed as a percent of the control with no added inhibitor.
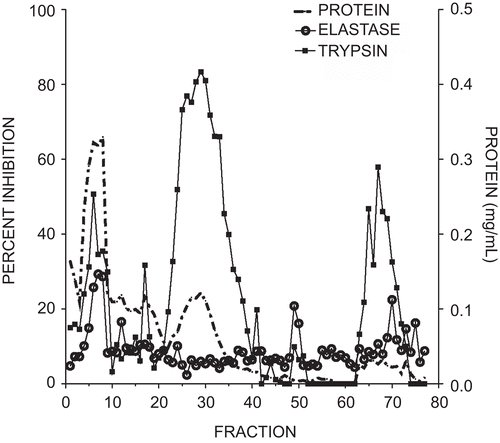
An image of the SDS PAGE (polyacrylamide gel electrophoresis) separation of proteins in the pooled fractions of marama bean extract that eluted from the elastase affinity column with pH 2.2-citrate buffer is shown in . Two major bands can be seen, with apparent molecular weights of 17.8 kDa and 20 kDa. The bands were excised and the protein eluted from the gel with water and assayed for anti-trypsin and anti-elastase activity. The proteins that eluted from both of the major protein bands excised from the marama bean extract expressed elastase inhibitory activity, while no trypsin inhibition was detected in either band. In a previous reportCitation28 from our laboratory, the Ki data for the elastase inhibitor from marama beans were derived from an impure ammonium sulfate fraction that we now know contains approximately 80% trypsin inhibitor and 20% elastase inhibitor. This indicates that the Ki values for the marama bean elastase inhibitor are actually four-fold lower than our published values. Re-calculation of the marama bean elastase inhibitor Ki using the corrected value for the protein content indicates that the Ki values are comparable to those obtained for other specific elastase inhibitors and correspond to uniformly strong inhibition of all sources of elastase we investigated.
Figure 3. SDS PAGE of purified elastase inhibitor compared with soybean trypsin inhibitor. Lane 1, standard proteins with molecular mass shown; lane 2, two intensely-staining bands of apparent 17.8 kDa and 20 kDa in a reduced sample of the marama bean extract purified through the affinity column; lane 3, separation of reduced soybean trypsin inhibitor indicating major bands at 23.1 kDa and 24.0 kDa.
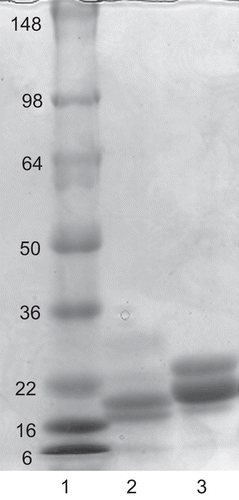
The results obtained by MALDI-TOF-MS and HPLC-ESI-MS/MS analyses indicated that the same protein was present in the two major bands of the marama bean elastase inhibitor. However, no high-confidence assignments were obtained by database searching of the tandem mass spectra, indicating a lack of sufficient identity with protein sequences in the NCBInr database. As such, de novo analysis was undertaken of the tandem mass spectra, resulting in the series of amino acid sequences shown in . Several peptides appeared to be glycosylated, as fragments corresponding to the loss of sugar units were observed. Other peptides that were not glycosylated yielded more complete sequences. The tandem mass spectrum for peptide AVVXNPHNTFGXPVXFR is shown in . BLAST (Basic Local Alignment Search Tool) searches (NCBInr) indicated that several peptides had homology to Kunitz-like serine protease inhibitors, as shown in .
Figure 4. Tandem mass spectrum of a 1893.1-Da tryptic peptide from the marama bean elastase inhibitor; assigned b-series (N-termal) and y-series (C-teminal) are indicated above the corresponding peaks and on the peptide sequence.
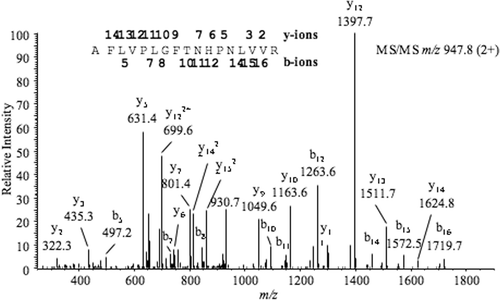
Figure 5. Sequence alignment of gi145579433 (Bbki, a Kunitz-type Kallikrein inhibitor, chain A) with peptides from the marama bean elastase inhibitor. Initial BLAST search indicated homology with gi145579433. A search of the peptides against Pfam family PF00197 using the Sequence Alignment Modeling system (SAMCitation31,Citation32) indicated that the peptides shown are significant matches.

Table 2. De novo sequences obtained from HPLC-ESI-MS/MS analysis of the elastase-specific inhibitor in marama bean.
Discussion
We have isolated a protease inhibitor from marama bean that is specific for elastase and distinct from the marama bean trypsin inhibitor. The elastase inhibitor represents around 14 % of the total acid-extractable protein from the marama bean. It was detected as two separate, low- molecular-weight proteins with apparently identical amino acid sequences. The difference in migration of these proteins on SDS PAGE is most likely due to differences in attached carbohydrates.
BLAST searches of the sequences determined by de novo analysis of the tandem MS data indicated homology with Kunitz-like serine protease inhibitors. The Kunitz-type soybean trypsin inhibitor (STI) family consists mainly of protease inhibitors from Leguminosae seeds and contains from 170 to 200 amino acid residues and one or two intra-chain disulfide bonds. The highest level of conservation has been found in the N-terminal regions of these proteins. The Kunitz-type inhibitors exhibit activity against trypsin and subtilisin, thiol proteases, aspartic proteases, and elastase.
Soybean protease inhibitors have understandably received more attention than other plant inhibitors due to their widespread availability and contribution to the human diet. There has, however, been renewed interest in the marama bean due to its potential as a food source in arid regions. It is quite interesting that the marama bean contains significantly higher levels of elastase inhibitor than other known beans or nuts. The marama bean is native to only a small area of the world in or around the Kalahari Desert. If these protease inhibitors serve as a protective mechanism against insects or other predators, it seems likely that there would be a predator that is unique to this area that contains high levels of elastase.
In summary, we have isolated and partially characterized a Kunitz-type serine protease inhibitor from marama beans. The inhibitor is present in two molecular weight forms with apparent differences in glycosylation. The inhibitor is present in unusually large quantities and is specific for inhibition of pancreatic and neutrophil elastase with no activity toward trypsin or chymotrypsin.
Acknowledgments
We are grateful to Dr. Stephen Hardies, UTHSCSA Department of Biochemistry, for his assistance with SAM analyses.
Declaration of interest: This study was supported by a grant from the Flight Attendants Medical Research Institute. The authors report no conflicts of interest.
References
- Lipke H, Fraenkel GS, Liener IE. Effects of soybean inhibitors on growth of Tribolium confusum. J Sci Food Agri 1954;2:410–15.
- Pannetier C, Giband M, Couzi P, Letan V, Mazier M, Tourneur J, et al. Introduction of new traits into cotton through genetic engineering: insect resistance as example. Euphytica 1997;96:163–6.
- Koiwa K, Shade RE, Zhu-Salzman K, Subramanian L, Murdock LL, Nielsen SS, et al. Phage display selection can differentiate insecticidal activity of soybean cystatins. Plant J 1998;14:371–9.
- Duan X, Li X, Xue Q, Abo-ei-Saad M, Xu D, Wu R. Transgenic rice plants harboring an introduced potato proteinase inhibitor II gene are insect resistant. Nature Biotech 1996;14:494–8.
- Gatehouse AMR, Boulter D. Assessment of the antimetabolic effects of trypsin inhibitors from cowpea (Vigna unguiculata) and other legumes on development of the bruchid beetle Callosobruchus maculatus. J Sci Food Agric 1983;34:345–50.
- Fan Wu. Plant proteinase inhibitors against phytophagous insects. Bot Bull Acad Sin 2005;46:273–92.
- Urwin PE, Lilley CJ, Mcpherson MJ, Atkinson HJ. Resistance to both cyst and rootknot nematodes conferred by transgenic Arabidopsis expressing a modified plant cystatin. Plant J 1997;12:455–61.
- Vain P, Worland B, Clarke MC, Richard G, Beavis M, Liu H, et al. Expression of an engineered cysteine proteinase inhibitor (Oryzacystatin-I delta D86) for nematode resistance in transgenic rice plants. Theor Appl Genet 1998;96:266–71.
- Ryan CA. Protease inhibitors in plants: genes for improving defenses against insects and pathogens. Annu Rev Phytopathol 1990;28:425–49.
- Koiwa H, Bressan RA, Hasegawa PM. Regulation of protease inhibitors and plant defense. Trends Plant Sci 1997;2:379–84.
- Mcmanus MT, White DWR, Mcgregor PG. Accumulation of chymotrypsin inhibitor in transgenic tobacco can affect the growth of insect pests. Transgenic Res 1994;3:50–8.
- Cipollini D, Bergelson J. Environmental and developmental regulation of trypsin inhibitor activity in Brassica napus. J Chem Ecol 2000;26:1411–22.
- Houseman JG, Downe AER, Philogene BJR. Partial characterization of proteinase activity in the larval midgut of the European corn borer Ostrinia nubilalis Hubner (Lepidoptera: Pyralidae). Can J Zool 1989;67:864–8.
- Williamson VM, Hussey RS. Nematode pathogenesis and resistance in plants. Plant Cell 1996 8:1735–45.
- Dunaevskii YE, Gladysheva IP, Pavlukova EB, Beliakova GA, Gladyschev DP, Papisova AI, et al. The anionic protease inhibitor BBWI-1 from buckwheat seeds. Kinetic properties and possible biological role. Physiol Plant 1997;100:483–8.
- Joshi B, Sainani M, Bastawade K, Gupta VS, Ranjekar PK. Cysteine protease inhibitor from pearl millet: a new class of antifungal protein. Biochem Biophys Res Commun 1998;246:382–7.
- Lawrence PK, Koundal KR. Agrobacterium tumefaciens mediated transformation of pigeonpea (Cajanus cajan (L.) Millsp) and molecular analysis of regenerated plants. Curr Sci 2001;80:1428–32.
- Urwin PE, McPherson MJ, Atkinson HJ. Enhanced transgenic plant resistance to nematodes by dual proteinase inhibitor constructs. Planta 1998:204:472–9.
- Haq SK, Atif SM, Khan RH. Protein proteinase inhibitor genes in combat against insects, pests, and pathogens: natural and engineered phytoprotection. Arch Biochem Biophys 2004;431:145–59.
- Powell AM. Marama bean (Tylosema esculentum, Fabaceae) seed crop in Texas. Econ Bot 2008;41:216–20.
- Keegan AB, Staden JV. Marama bean, Tylosema esculentum, a plant worthy of cultivation. South Afr J Sci 1989;77:387.
- Bower N, Hertel K, Oh J, Storey R. Nutritional evaluation of marama bean (Tylosema esculentum Fabaceae): analysis of the seed. Econ Bot 1988;42:533–40.
- Blesele M, Murry RE. Alternative food plants for arid regions. Final project report, marama and other plant foods of Kalahari foragers: an applied ethnobotanical study. Center for the Study of Human Adaptation, University of Texas at Austin, 1983.
- Ripperger-Suhler JA. Protein quality of the African marama bean. Master’s Thesis, University of Texas at Austin, 1983.
- Kakade ML, Hoffa DE, Liener IE. Contribution of trypsin inhibitors to the deleterious effects of unheated soybeans fed to rats. J Nutr 1973;103:1772–8.
- Elfant M, Bryant L, Starcher B. Isolation and characterization of a proteinase inhibitor from marama beans. Proc Soc Exp Biol Med 1985;180:329–33.
- Starcher B, Bryant L, Elfant M. Protease inhibitors of the marama bean. Adv Exp Med Biol 1986;199:429–38.
- Nadarajah D, Atkinjson MAL, Heubner P, Starcher B. Enzyme kinetics and characterization of mouse pancreatic elastase. Connect Tissue Res 2008;49:409–15.
- Wagner LP, Riehm JP. Purification and partial characterization of a trypsin inhibitor isolated from the navy bean. Arch Biochem Biophys 1967;121:672–7.
- Laemmli UK. Cleavage of structural proteins during the assembly of the head of bacteriophage T4”. Nature 1970;227:680–5.
- Hughey R, Krogh A. Hidden Markov models for sequence analysis: extension and analysis of the basic method. Comput Appl Biosci 1996;12:95–107.
- Karplus K, Barrett C, Hughey R. Hidden Markov models for detecting remote protein homologies. Bioinformatics 1998;14:846–56.