Abstract
The anti-hyperglycaemic effects of the leaves of Acer pycnanthum K. Koch, and the purification and identification of the active compounds were investigated. Extracts of the leaves showed a potent inhibitory effect on the α-glucosidase in both in vivo and in vitro experiments. The fractionation of the crude extract gave two active compounds, ginnalin B (6-O-galloyl-1,5-anhydro-D-glucitol) and ginnalin C (2-O-galloyl-1,5-anhydro-D-glucitol), by spectroscopic analysis. This is the first report that A. pycnanthum and its constituents may be useful for the prevention or treatment of diabetes mellitus.
Introduction
As a possible approach to the treatment of diabetes, decreasing postprandial hyperglycaemia has been proposed [Citation1]. Different types of anti-hyperglycaemic drugs are conceivable [Citation2]. By inhibiting carbohydrate hydrolysis enzymes such as α-glucosidase and α-amylase in the digestive tract, we can delay carbohydrate digestion. We could also reduce postprandial hyperglycaemia by impairing glucose production and diminishing glucose absorption from the small intestine. Such an inhibitory action has been shown to be one of the most effective approaches for preventing diabetes in recent clinical studies [Citation3]. Thus, it is believed that an anti-hyperglycaemic effect caused by the inhibition of α-glucosidase may be important for diabetes care. Worldwide, many kinds of herbs are used for diabetic care [Citation4,Citation5] and in medicinal therapy for diabetes. To identify new compounds that may be useful for preventing or treating diabetes, we screened many kinds of natural products that exert an anti-hyperglycaemic effect and identified foods that may be useful for diabetic care, as described previously [Citation6–8]. As part of these ongoing experiments, we found that an extract from the leaves of Acer pycnanthum had an anti-hyperglycaemic effect.
A. pycnanthum belongs to Aceraceae and is called “Hana-noki” in Japan. The trees grow wild in the Aichi, Gifu, Nagano, and Shiga prefectures in Japan [Citation9] and are planted in gardens and by the roadside. The genus Acer includes more than 200 species that are distributed mainly in the temperate zone of the northern hemisphere [Citation10]. In the autumn, the leaves change colour from green to yellow or red.
Acer nikoense Maxim is used in Japan as a folk medicine for the eyes and some of its constituents have been reported together with their actions [Citation11,Citation12]. A. pycnanthum is also used as a folk medicine for eye care [Citation13]. Other pharmacological effects of Acer genus have also been reported [Citation14,Citation15]. However, the phytochemical constituents of A. pycnanthum and their biological activities have not yet been reported.
In this study, we found that the extract of the leaves of A. pycnanthum had an anti-hyperglycaemic effect and we have elucidated the mechanism based on an inhibitory effect toward α-glucosidase. We also identified ginnalin B (6-O-galloyl-1,5-anhydro-d-glucitol) and ginnalin C (2-O-galloyl-1,5-anhydro-d-glucitol) in the extract.
Materials and Methods
Preparation of sample extract
The methanol extract of the leaves of A. pycnanthum (APM) was obtained as described below. The leaves were collected in July and extracted with a 10-fold volume of methanol at room temperature for 2 days. After filtration, the extract was evaporated by a rotary evaporator. The recovery rate for the APM was 15% of the weight of the fresh leaves.
Animals
Male ddY mice (6–8 weeks of age) were purchased from SLC, Shizuoka, Japan. The room was maintained at 24 ± 1°C and 50 ± 10% humidity under a 12 h light/dark cycle (lights on from 8:00 AM to 8:00 PM), and the animals had free access to water and food. Animal studies were conducted according to the 2006 guidelines entitled Notification No 88 of the Ministry of the Environment in Japan and Guidelines for Animal Experimentation of Tokyo University of Marine Science and Technology with the approval of the Animal Care and Use Committee of Tokyo University of Marine Science and Technology
Assay for inhibitory activity against α-glucosidase
Intestinal α-glucosidase inhibitory activity was determined as described in the literature with some modification [Citation16]. Fasted ddY male mice were sacrificed and the small intestines were immediately removed. The contents of the small intestines which included brush border enzymes were squeezed out and used to prepare samples at 25 mg/mL. Ice-cold phosphate buffer (pH 6.9) containing 0.2% bovine serum albumin was used as a solvent. The samples were centrifuged at 4500 × g for 5 min at 4°C. The supernatant was used as a crude enzyme solution in the experiment.
The activity of α-glucosidase was assayed with 27.7 mM maltose or 55.3 mM sucrose (final concentrations) with or without APM at different concentrations. Acarbose was used as a positive control. After the samples and substrate were pre-incubated for 5 min at 36.5°C, the enzyme solution was then added. The samples were then incubated at the same temperature for 20 min for maltose, or 40 min for sucrose. The reaction was terminated by heating with boiling water for 5 min, and the quantity of glucose produced was measured with a commercial GOD-POD kit (Glucose C II-test, WAKO Pure Chem, Osaka, Japan). The reaction rate (%) was calculated and the IC50 value of each sample was determined.
Carbohydrate-loading tests in mice
The mice were fasted for 18 h and divided into groups according to body weight (n = 6). Each sample was dissolved in water and administered orally. In the control group, mice were given sucrose (2,000 mg/kg body weight) or glucose (1,000 mg/kg body weight). In the APM-treated group, mice were given a mixture of 100 mg/kg body weight of APM or, as a positive control, 3 mg/kg of acarbose, which is known to be a major inhibitor of α-glucosidase and each carbohydrate. Blood from the tail vein was collected sequentially in test tubes and centrifuged at 4,500 × g for 2 min. The serum was extracted and the serum glucose level was measured. The serum glucose level was measured before the administration, and at 30, 60, 90, and 120 min after administration. The serum glucose level was measured by GOD-POD kit (Glucose C II-test, WAKO Pure Chem).
Statistical analysis
Data are presented as the mean ± S.E. The significance of differences was calculated using Student’s t-test, and the results were considered statistically significant when p < 0.05.
Spectroscopic analysis
To determine the chemical structures of the purified compounds, NMR spectra were recorded with a 400 MHz NMR (AV-400, Bruker Biospin, Yokohama, Japan) and MS spectral data were obtained on an ESIMS spectrometer (microOTOF-QII, Bruker).
Results
Effect of APM on α-glucosidase activity
To confirm the effects of APM on α-glucosidase activity, in vitro experiments were performed with sucrose or maltose as a substrate. The IC50 (µg/mL) values of APM and acarbose against sucrase activity were 38.5 ± 5 and 0.5 ± 0.1, respectively. Against maltase these were 180.5 ± 5.1 and 0.8 ± 0.2, respectively.
Effect of APM on the serum glucose level in carbohydrate-loaded mice
shows the anti-hyperglycaemic effects of APM in sucrose (A)- and glucose (B)-loaded mice. The serum glucose level in the APM group was significantly lower than that of the control group. In the sucrose-loading test, the serum glucose levels at 30, 60 and 90 min after administration in the APM group were 121.9 ± 2.4, 129.1 ± 4.6, and 140.9 ± 4.1 mg/dl, while in the control group these values were 165.6 ± 15.2, 177.1 ± 9.8, and 178.5 ± 12.2 mg/dl, respectively. The administration of 100 mg/kg APM had a suppressive effect on hyperglycaemia that was similar to that found with the administration of 3 mg/ kg acarbose. On the other hand, there was no significant difference in the glucose-loading test.
Figure 1. Anti-hyperglycaemic effects of APM in mice. Fasted mice were given 2,000 mg/kg of sucrose (A), 1,000 mg/kg of glucose (B), with or without 100 mg/kg of APM (methanol extract of Acer pycnanthum: open squares), 3 mg/kg of acarbose (closed triangles) and vehicle (control: closed squares), Data are presented as the mean ± S.E. (n = 6). ***; p <0.005, *; p <0.05 versus the control.
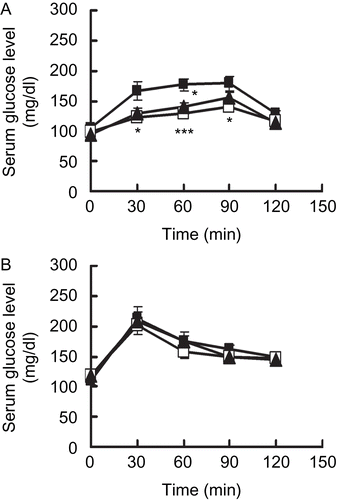
Purification of the active ingredients from APM
APM was fractionated as described below to give two active compounds. APM (3 g) was partitioned between 90% methanol and hexane. The 90% methanol layer was chromatographed on an octodecyl silane (ODS) column (Wakogel, WAKO Pure Chem) with a water-methanol gradient and an ethyl acetate elution to give three fractions. The active fraction, Fraction 1 (205 mg) eluted with 40% methanol was subjected to HPLC, using an ODS column (Develosil HG-5 20 × 250 mm; Nomura Chemical, Aichi, Japan) with 30% methanol at a flow rate of 4 mL/min, detected at UV 215 nm to give five fractions. The active fraction, Fraction 1-2 was further purified by the same HPLC system except using a more polar mobile phase 10% methanol and then divided into two fractions to give Fraction 1-2-1 (1, 2.4 mg, tR: 34 min) and Fraction 1-2-2 (2, 1.2 mg, tR: 37 min) as the active compounds.
Identification of the structures of 1 and 2
Ginnalin B, 6-O-galloyl-1,5-anhydro-d-glucitol (1) was isolated as a reddish brown crystal. The molecular formula of 1 was determined to be C13H16O9 [(M+H)+, m/z: 317.0868, δ -0.1 mmu] by HR-ESIMS (positive mode). Spectroscopic data for 1; 1H NMR (CDCl3, 400 MHz) δ 6.8 (2H, s, galloyl-H), 4.51 (1H, dd, J = 11.9, 1.1 Hz, H-6a), 4.3 (1H, dd, J = 11.9, 5.3 Hz, H-6b), 3.92 (1H, dd, J = 10.9, 5.2 Hz, H-1a), 3.43 (1H, m, H-5), 3.38 (1H, m, H-4), 3.35 (1H, m, H-2), 3.32 (1H, t, J = 10.8 Hz, H-3), 3.2 (1H, t, J = 10.9 Hz, H-1b); 13C-NMR (CDCl3, 100 MHz) δ 168.9 (C-7′), 147 (C-3′ and C-5′), 140.5 (C-4′), 121.8 (C-1′), 110.7 (C-2′ and C-6′).
Ginnalin C, 2-O-galloyl-1,5-anhydro-d-glucitol (2) was isolated as a reddish brown crystal. The molecular formula of 2 was determined to be C13H16O9 [(M+H)+, m/z: 317.0869, δ -0.2 mmu] by HR-ESIMS (positive mode). Spectroscopic data for 2; 1H NMR (CDCl3, 400 MHz) δ 6.8 (2H, s, galloyl-H), 4.85 (1H, dd, J = 10.4, 5.4 Hz, H-2), 4.08 (1H, dd, J = 10.8, 5.4 Hz, H-1a), 3.85 (1H, dd, J = 11.6, 1.4 Hz, H-6a), 3.65 (1H, dd, J = 11.6, 6.2 Hz, H-6b), 3.63 (1H, dd, J = 6.2, 5.4 Hz, H-3), 3.5 (1H, m, H-4), 3.28 (1H, m, H-1b), 3.22 (1H, m, H-5); 13C-NMR (CDCl3, 100 MHz) δ 168.3 (C-7′), 146.9 (C-3′ and C-5′), 140.4 (C-4′), 121.6 (C-1′), 110.6 (C-2′ and C-6′).
The similarities of the spectral data for 1 and 2 revealed that the two compounds were isomers of each other. NMR data showed that both compounds possessed a galloyl moiety and a 1-deoxyglucose moiety. The heteronuclear multiple bond coherence (HMBC) correlations to the carbonyl carbon of the galloyl moiety differed between 1 and 2. As a result, 1 was determined to be 6-O-galloyl-1,5-anhydro-d-glucitol (ginnalin B) based on δH 4.49 and 4.24 (H-6a and 6b) and 2 was determined to be 2-O-galloyl-1,5-anhydro-d-glucitol (ginnalin C) based on δH 4.65 (H-2). Other spectral data correspond to those in a previous report [Citation17]. These structures are shown in .
Effects of ginnalins B and C on sucrase activity
Ginnalins B (1) and C (2) exerted an sucrase-inhibitory effect. The IC50 values of 1 and 2 were calculated to be 79.8 ± 5.6 and 42.1 ± 2.9 µg/mL, respectively.
Kinetics analysis of sucrase inhibition by ginnalins B and C
In order to examine the type of inhibition of ginnalins, the sucrase activities were measured with increasing concentrations of sucrose (6.91–110.67 mM) with or without 1 or 2. The amount of protein was determined by the Bradford protein assay kit (Pierce Biotech, IL, USA). One unit of enzyme activity was taken as the amount of enzyme which liberates one micromole of glucose from sucrose under the experimental conditions. The mode of inhibition by 1 and 2 were assessed by a Lineweaver-Burk plot analysis. The results revealed that 1 and 2 inhibited sucrase activity in a mixed competitive manner ().
Effects of ginnalins B and C on serum glucose levels in sucrose-loaded mice
Both compounds (24 mg/kg) isolated from APM were used in an in vivo experiment in sucrose-loaded mice (2,000 mg/ kg). As shown in , the serum glucose levels of in the treated groups were significantly lower than that in the control group at 30 min. In the ginnalin B and C group, each value was 223.7 ± 10.1, 196.4 ± 8.8 respectively, while in the control group it was 274.2 ± 10.9 for the equivalent time.
Figure 4. Anti-hyperglycaemic effects of ginnalins B and C in sucrose-loaded mice. Fasted mice were given 2,000 mg/kg of sucrose with 24 mg/ kg of ginnalin B (open squares) or 24 mg/kg of ginnalin C (closed triangles) and vehicle (control: closed squares). Data are presented as the mean ± S.E. (n = 8). ***; p <0.005 versus the control.
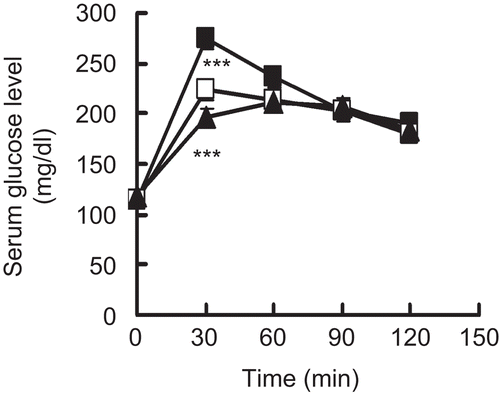
Discussion
This report is the first to show that Acer pycnanthum contains ginnalins B (1) and C (2), which exert anti-hyperglycaemic effects through a mechanism that involves the inhibition of α-glucosidase.
As revealed in , APM showed an anti-hyperglycaemic effect in a sucrose-loading test with the oral administration of APM (100 mg/kg) to the same degree as with the oral administration of acarbose (3 mg/kg), which is a major α-glucosidase inhibitor. However, APM did not have a similar effect in a glucose-loading test. Thus, the anti-hyperglycaemic effect of APM is due to an inhibitory effect on α-glucosidase, which is a disaccharide hydrolysis enzyme, and an in vitro test showed such an effect. In fact, both in vivo and in vitro, APM showed the same degree of anti-hyperglycaemic effect and α-glucosidase-inhibitory effect as an extract of mulberry (Morus alba) leaf, which is a medicinal herb that is used to treat diabetes (data not shown) [Citation18].
We purified two active ingredients from APM and a spectroscopic analysis of their structures revealed that these compounds were 1 and 2, as shown in . And both compounds also showed sucrase-inhibitory effect and had an anti-hyperglycaemic effect in a sucrose-loading test as shown in . Compared with the IC50 values of APM and 1 or 2, each compound showed less sucrase-inhibitory action. The recovery rate of 1 and 2 purified from APM were 0.08 and 0.04%, respectively. Therefore 1 or 2 may not be the core of the active compound and the other compounds may also contribute to the inhibitory effect of APM on α-glucosidase.
Conpounds 1 and 2 were previously isolated from the leaves of Acer ginnala Maxim by Song et al. [Citation17] and used as antibacterial compounds, in vitro, toward Flexner’s dysentery bacillus, Sonne dysentery bacillus and Aurococcus [Citation19]. A. ginnala has also been used as a folk medicine in Korea as a stomachic and for the treatment of diarrhea [Citation20].
With regard to 1 and 2, it has been reported that some galloyl derivatives have shown α-glucosidase-inhibitory activity, such as epigallocatechingallate from tea (Camellia sinensis) [Citation21] and ellagitannins from clove (Syzgium aromaticum) [Citation22]. Gallotannins show high affinity for sugars [Citation23], and gallotannin and sugars may form hydrogen bonds through the galloyl part of these compounds in the small intestine, and this may prevent their absorption. In addition, the galloyl part may inhibit enzymes through a high affinity for proteins [Citation24]. And these tannins inhibit α-glucosidase in an uncompetitive manner [Citation21,Citation25]. It is also conceivable that the 1-deoxyglucose part of 1 or 2 may imitate glucose and mimic a disaccharide. This may explain the inhibitory effects of these two compounds [Citation26,Citation27] in a competitive manner. Therefore 1 and 2 may inhibit sucrase in a mixed competitive manner ().
Some reports have shown that plants in the Acer genus contain galloyl derivatives, such as gallotannin and epigallocatechin gallate [Citation28,Citation29]. There have been some reports on the constituents of the genus. These have included flavonoid structures, such as quercetin [Citation30], vitexin [Citation31], afzelin, and quercitrin [Citation32]. These constituents have an α-glucosidase-inhibitory effect [Citation33,Citation34] and some interesting bioactivities, such as anti-oxidative, anti-tumour, and anti-inflammatory effects. In one report on the constituents of Aceraceae, the contents of anthocyanins in the leaves were increased in spring and autumn. In particular, for A. pycnanthum, cyanidin 3-glucoside, 3-rutinoside, 3-galloylglucoside, 3-galloylrutinoside, and 3,5-diglucoside have been identified [Citation35]. Cyandin 3-sambubioside [Citation36] and cyanidin 3-galactoside [Citation37] have been reported to have inhibitory effect of α-glucosidase. Therefore, these compounds may also contribute to the inhibitory effect of APM on α-glucosidase.
Conclusion
Acer pycnanthum has a potent anti-hyperglycaemic effect and an α-glucosidase inhibitory effect. The active compounds were purified and identified as ginnalins B and C. It is possible that A. pycnanthum and its constituents may be useful for the prevention and treatment of diabetes mellitus.
Acknowledgements
We would like to thank K. Oyama (Chemical Instrumentation Facility, Nagoya University) for measuring the HR-ESIMS of purified compounds.
Declaration of interest
This study was supported in part by a Grant-in-Aid for Scientific Research for Creative Scientific Research (No. 21510223) from the Ministry of Education, Culture, Sports, Science and Technology, Japan.
References
- Brend S, Diethelm T. Atherogenesis and atherothrombosis – focus on diabetes mellitus. Best Pract Res Clin Endocrinol Metabol 2009;23:291–303.
- Foreyt PJ. Need for lifestyle intervention: How to begin. Am J Cardiol 2005;96:11–14.
- Kawamori R, Tajima N, Iwamoto Y, Kashiwagi A, Shimamoto K, Kaku K. Voglibose for prevention of type 2 diabetes mellitus: a randomised, double-blind trial in Japanese individuals with impaired glucose tolerance. Lancet 2009;373:1607–1614.
- Li WL, Zheng HC, Buruku J, De ND. Natural medicines used in the traditional Chinese medical system for therapy of diabetes mellitus. J Ethnopharmacol 2004;92:1–21.
- Zareba G, Serradell N, Castaner R, Davis SL, Prous J, Mealy N. Phytotherapies for diabetes. Drug Future 2005;30:1253–1282.
- Karato M, Yamaguchi K, Takei S, Kino T, Yazawa K. Inhibitory effect of Pasuchaca (Geranium dielsiaum) extract on α-glucosidase in mice. Biosci Biotechnol Biochem 2006;70:1482–1484.
- Honma A, Koyama K, Yazawa K. Anti-hyperglycemic effect of Kamaboko in mice. 5th World Fisheries Congress 2008;20–24 Oct. Yokohama.
- Shirosaki M, Koyama T, Yazawa K, Anti-hyperglycemic activity of Kiwifruit leaf (Actinidia deliciosa) in mice. Biosci Biotechnol Biochem 2008;72:1099–1102.
- Barnes VB, Saeki I, Kitazawa A. Occurrence and landscape ecology of a rare disjunct maple species, Acer pycnanthum, and comparison with Acer rubrum. Environ Rev 2004;12:163–196.
- Ogata K. A dendrological study on the Japanese Aceraceae, with special reference to the geographical distribution. Bull Tokyo Univ Forests 1967;60:1–100.
- Morikawa T, Tao J, Ueda K, Matsuda H, Yoshikawa M. Medicinal foodstuffs XXXI. Structures of new aromatic constituents and inhibitors of degranulation in RBL-2H3 cell from a Japanese folk medicine, the stem bark of Acer nikoense. Chem Pharm Bull 2003;51:62–67.
- Akazawa H, Akihisa T, Taguchi Y, Banno N, Yoneiwa R, Yasukawa K. Melanogenesis inhibitory effect and free radical scavenging activities of diarylheptanoids and other phenolic compounds from the bark of Acer nikoense. Chem Pharm Bull 2003;29:1970–1972.
- Shibata K. “Shigen Syokubutsu Jiten” (Encyclopedia of Plant Resources). Tokyo: Hokuryukan, 1989. pp. 1556.
- Kupchan SM, Takasugi M, Smith RM, Steyn PS. Acerotin and Acerocin, novel triterpene ester aglycones from the tumour-inhibitory saponins of Acer negundo. Chem Commun 1970:969–970.
- Kim JH., Woo RE, Shin GC, Park HK. A new flavonol glucoside callate ester from Acer okamotoanum and its inhibitory activity against Human Immunodeficiency Virus-1 (HIV-1) integrase. J Nat Prod 1998;61:145–148.
- Dahlqvist A. Method for assay of intestinal disaccharidases. Anal Biochem 1964;71:18–25.
- Song C, Zhang N, Xu R, Song, HX. Studies on antibacterial constituents of the leaves of Acer ginnala Maxim. II. Isolation and identification of ginnalin B and ginnalin C and six other compounds. Acta Chim Sinica 1982;40:1142–1147.
- Miyahara C, Miyazawa M, Sato S, Sakai A, Mizusaki S. Inhibitory effect of mulberry leaf extract on postprandial hyperglycemia in normal rats. J Nutr Sci Vitaminol 2004;50:161–164.
- Xu RS, Zhu QZ, Xie YY. Recent advances in studies on Chinese medicinal herbs with physiological activity. J Ethnopharmacol 1985;14:223–253.
- Han SH, Lo CS, Choi WY, Kim HJ, Baek HS. Antioxidant activity of crude extract and pure compounds of Acer ginnala Max. Bull Korean Chem Soc 2004;25:389–391.
- Gamberucci A, Konta L, Colucci A, Gunti J, Magyar EJ, Mandl J, Banhegyi G, Benedetti A, Csala M. Green tea flavonoids inhibit glucosidase II. Biol Pharmacol 2006;72:640–646.
- Toda M, Kawabata J, Kasai T. Inhibitory effect of ellagi- and gallotannnins on rat intestinal α-glucosidase complex. Biosci Biotechnol Biochem 2004;65:542–547.
- Qiang H, Bi S, Kai Y. Interactions of gallotannins with proteins, amino acids, phospholipids and sugars. Food Chem 2006;95:250–254.
- Shimada T, Salivary proteins as a defense against dietary tannins. J Chem Ecol 2006;32:1149–1163.
- Gao H, Huan NY, Xu YP, Kawabata J. Inhibitory effect on α-glucosidase by the fruits of Terminalia chebura Retz. Food Chem 2007;105:628–634.
- Oku T, Yamada M, Nakamura M, Sadamori M, Nakamura S, Inhibitory effect of extractives from leaves of Morus alba on human and rat small intestinal disaccharidase activity. Br J Nutr 2006;95:933–938.
- Seles JP, Hujiberts MH,Wolffenbuffel BW. Miglitol, a new alpha-glucosidase inhibitor. Expert Opin Pharmacother 1999;1:149–156.
- Ma X, Wu L, Ito Y, Tian W. Application of preparative high-speed counter-current chromatography for separation of methyl gallate from Acer truncatum Bunge. J Chromatogr A 2005;1076:212–215.
- Barbehenn RV, Jones CP, Karonen M, Salminen JP. Tannin composition affects the oxidative activities of tree leaves. J Chem Ecol 2006;32:2235–2251.
- Ma X, Wu L, Tian W, Ito, Y. Isolation of quercetine-3-O-l-rhamnoside from Acer truncatum Bunge by high-speed counter-current chromatography. J Chromatogr A 2005;1070:211–214.
- Kim JH, Lee BC, Sim GS, Lee DL, Yun YP, Pyo HB. The isolation and antioxidative effects of vitexin from Acer palmatum. Arch Pharm Res 2005;28:195–202.
- Aritomi M. Chemical constituents in Aceraceous plants. II. Flavonoid constituents in leaves of Acer capinifolium Siebold et Zuccarini, A. diabolicum Blume, and A. negundo. J Pharm Soc 1964;84:360–362.
- Miwa I, Okuda J, Horie T, Nakayama M. Inhibition of intesitinal α-glucosidase and sugar absorption by flavones. Chem Pharm Bull 1986;34:838–844.
- Shibano M, Kakutani K, Taniguchi M, Yasuda M, Baba K. Antioxxidant constituents in the dayflower (Commelina communis L.) and their α-glucosidase-inhibitory activity. J Nat Med 2008;62:349–353.
- Ji SB, Sato N, Yokoi N, Sugiura A, Honda T. Galloylcyanidine glycoside from Acer. Biochem System Ecol 1992;20:771–781.
- Iwai K, Kim MY, Onodera A, Matsue H. α-glucosidase inhibitory and antihyperglycemic effect of polyphenols in the fruit of Viburum dilatatum Thumb. J Agric Food Chem 2006;54:4588–4592.
- Sirichai A, Piyawan C, Sirintron YA. α-glucosidase inhibitory activity of cyaniding-3-galactoside and synergistic effect with acarbose. J Enzyme Inhib Med Chem 2009;24:65–69.