Abstract
The standard treatment of poisoning by organophosphorus compounds (OP) includes the reversible muscarine receptor antagonist atropine and oximes for the reactivation of OP-inhibited acetylcholinesterase (AChE). There is an ongoing discussion on the benefit of oxime therapy in OP pesticide poisoning, and experimental data indicate a limited efficacy of oximes against various nerve agents. Oxime effectiveness can be quantified in vitro by determination of the reactivity (kr) and affinity constants (1/KD). These constants can be used to calculate reactivation velocities and oxime concentrations necessary for the reactivation of a desired fraction of inhibited AChE. Model calculations indicate that a kr > 0.1 min−1 and KD < 100 µM are minimal requirements for oxime effectiveness when reactivation is performed in the absence of free OP. In addition, the findings demonstrate that selective increase of either reactivity or affinity of an oxime would be insufficient. Hereby, it has to be taken into account that an increase of affinity to OP-inhibited AChE is generally accompanied by an increased affinity to native AChE and subsequent reduction in oxime tolerance. Hence, future developments of more effective oximes should consider kinetic demands by attempting to achieve a certain level of reactivity and affinity, preferentially towards OP-inhibited AChE.
Introduction
The clinical treatment of poisoning by organophosphorus compound (OP)-based acetylcholinesterase (AChE) inhibitors, i.e. pesticides and nerve agents, did not experience fundamental change since the first use of the oxime pralidoxime (2-PAM, ) in 1958Citation1. Basically, the specific treatment of OP-poisoning is a combination of an anticholinergic drug, mostly atropine, and an oxime which is intended to reactivate OP-inhibited AChECitation2. The antidotal therapy is supplemented by benzodiazepines, catecholamines, antibiotics, and further drugs depending on the clinical signs and symptomsCitation3–6.
Apart from pralidoxime, only few oximes have been used in human OP-poisoning in the past 50 yearsCitation7. Obidoxime () was first used in the early 1960sCitation8 followed by trimedoxime (TMB-4, )Citation9 while HI-6 () was tested only in few cases of OP pesticide poisoningCitation10.
Apart from pralidoxime, only few oximes have been used in human OP-poisoning in the past 50 yearsCitation7. Obidoxime () was first used in the early 1960sCitation8 followed by trimedoxime (TMB-4, )Citation9 while HI-6 () was tested only in few cases of OP pesticide poisoningCitation10.
Apart from pralidoxime, only few oximes have been used in human OP-poisoning in the past 50 yearsCitation7. Obidoxime () was first used in the early 1960sCitation8 followed by trimedoxime (TMB-4, )Citation9 while HI-6 () was tested only in few cases of OP pesticide poisoningCitation10.
Although only very few oximes are used clinically a huge number of oximes were synthesized in the past decades mainly in Germany, USA, Croatia, Israel, Czech Republic, and KoreaCitation7,Citation11. The main purpose of these oxime development programs was to find oximes which are more effective than the clinically used compounds in nerve agent poisoning. The ultimate goal of this development was the search for highly effective oximes which can be used in autoinjectors with limited volume for self and buddy aid. Experimental oximes synthesized so far are in most cases derivatives of the mono-pyridinium oxime pralidoxime or the bis-pyridinium oxime obidoximeCitation7 and only few are bearing additional imidazolium or quinuclidinium groupsCitation12.
There is convincing evidence that the main mechanism of action of oximes is the reactivation of OP-inhibited AChE by removal of the phosphoryl- or phosphonyl-moiety from the active site serineCitation7,Citation13–15. By restoring the function of the pivotal enzyme AChE OP-induced disruption of the cholinergic signalling chain may be counteracted and life-saving in severe cases of OP-poisoning. The ability of oximes to reactivate OP-inhibited AChE may be quantified in vitro by determination of the different reactivation rate constants (). According to the scheme the ability of oximes to reactivate OP-inhibited AChE is determined by the affinity (1/KD) and the reactivity (kr). The efficiency of an oxime may be quantified by the second order rate constant kr2 determined by the ratio of kr and KDCitation16. Based on these constants the reactivating ability of oximes may be estimated prior to in vivo evaluation of the oxime efficacy in animals or humans.
Figure 2. Reaction scheme for the reactivation of organophosphate-inhibited acetylcholinesterase (AChE) by oximes. The respective concentrations are: [EP] the phosphylated AChE, [OX] the reactivator, [EPOX] the Michaelis-type phosphyl-AChE-oxime-complex, [E] the active enzyme and [POX] the phosphylated oxime. KD is equal to the ratio [EP] × [OX]/[EPOX] and describes the dissociation constant which is inversely proportional to the affinity of the oxime to [EP], and kr is the rate constant for the displacement of the phosphyl residue from [EPOX], indicating the reactivity of the oxime.
![Figure 2. Reaction scheme for the reactivation of organophosphate-inhibited acetylcholinesterase (AChE) by oximes. The respective concentrations are: [EP] the phosphylated AChE, [OX] the reactivator, [EPOX] the Michaelis-type phosphyl-AChE-oxime-complex, [E] the active enzyme and [POX] the phosphylated oxime. KD is equal to the ratio [EP] × [OX]/[EPOX] and describes the dissociation constant which is inversely proportional to the affinity of the oxime to [EP], and kr is the rate constant for the displacement of the phosphyl residue from [EPOX], indicating the reactivity of the oxime.](/cms/asset/80fe925d-3936-4409-b68f-09d2803f96e5/ienz_a_504673_f0002_b.gif)
In the past decade we determined the reactivation rate constants for a variety of oximes with a large number of structurally different OP using AChE from different speciesCitation16–23. Hereby it became apparent that the ability of oximes to reactivate OP-inhibited AChE is strongly dependent on the structure of the oxime, the OP-AChE conjugate and the AChE source. Due to, in part substantial, differences between human and animal AChE further considerations will focus only on human AChE. Moreover, only reactivation data from identical experimental set-up were considered for comparison, i.e. reaction conditions at pH 7.4, 37°C, in the absence of substrate and after removal of free OP.
The determination of the affinity and reactivity of oximes revealed enormous differences. The spectrum ranged from complete inability of oximes to reactivate inhibited AChE (e.g. HI-6 and tabun), hardly measurable kr and KD values to utmost high reactivity and affinity. shows the extremes determined so far. Accordingly, the difference between the determined dissociation and reactivity rate constants is almost 2000 and 3000 fold, respectively.
Table 1. Highest and lowest reactivation rate constants of oximes with OP-inhibited human AChE.
Table 2. Reactivation rate constants of selected oximes and OP.
Animal experiments and clinical data demonstrate that the therapeutic efficacy of oximes is also determined by additional factorsCitation3,Citation4,Citation24–29. Post-inhibitory reactions of the OP-inhibited AChE, i.e. spontaneous reactivation and aging (), may enhance or impair the oxime efficacy. Further factors include pre-treatment, OP body load and competing acetylcholine concentration, time interval between poisoning and onset of treatment, oxime dosing and duration of treatment. In view of multiple factors influencing the efficacy of oximes in OP-poisoning there is an ongoing debate on the benefit of oximes and on the necessity to develop more effective oximes as antidotes against pesticide and nerve agent poisoningCitation2–4,Citation11,Citation28–31.
These controversies prompted us to use theoretical models, based on kinetic data obtained under identical experimental conditions, to characterize the requirements of an oxime as an effective antidote in OP-poisoning.
Which level OF AChE activity is necessary?
Impairment of neuromuscular transmission at respiratory muscles is one of the life threatening effects of OP which cannot be counteracted by anticholinergicsCitation32. Hence, the reactivation of AChE at neuromuscular synapses is a key parameter for the assessment of oxime efficacy in vivo. Unfortunately, synaptic AChE is hardly accessible to direct monitoring and the evaluation of oxime effects has to be based on surrogate parameters. Repetitive measurement of erythrocyte AChE activity can be used to follow changes in AChE activity as a result of oxime treatment provided that erythrocyte AChE reflects synaptic AChE.
Recently, in vitro studies using a dynamic model with real-time determination of membrane-bound AChE activity demonstrated almost identical kinetics of human erythrocyte and intercostal muscle AChE following inhibition by OP and reactivation by oximesCitation33,Citation34. A thorough analysis of data from OP pesticide poisoned patients revealed that erythrocyte AChE levels higher than 40% of normal were accompanied by normal neuromuscular transmissionCitation26,Citation35.
Consequently, an effective oxime does probably not require a complete reactivation of inhibited AChE and a 40% reactivation will be considered as lower edge for further considerations. Now, it was tempting to determine whether presently available oximes could meet this goal. Initial calculations were performed using determined reactivation rate constantsCitation16 and excluding excess inhibitor and premature aging. shows the marked differences between the selected OP. None of the selected oximes would be able to reactivate tabun-inhibited AChE at clinically used oxime concentrations within a reasonable time, primarily due to low reactivity (). With cyclosarin-inhibited AChE HI-6 (high reactivity and affinity) and MMB-4 (high reactivity) should result in a rapid and complete restoration of enzyme activity within a few minutes (), obidoxime and 2-PAM would fail due to low affinity. With paraoxon- and methamidophos the oximes should be able to reactivate the enzyme although HI-6 would result in a rather slow increase of paraoxon-inhibited AChE activity ().
Figure 3. Calculated acetylcholinesterase (AChE) activities of tabun- (A), cyclosarin- (B), paraoxon-ethyl- (C), and methamidophos-inhibited AChE (D) after reactivation by obidoxime (OBI, 10 µM), 2-PAM (100 µM), HI-6 (50 µM), and MMB-4 (100 µM). Based on experimental reactivation rate constantsCitation16, the AChE activities were calculated using the equation AChEt% = 100 × (1-exp−kobs × t).
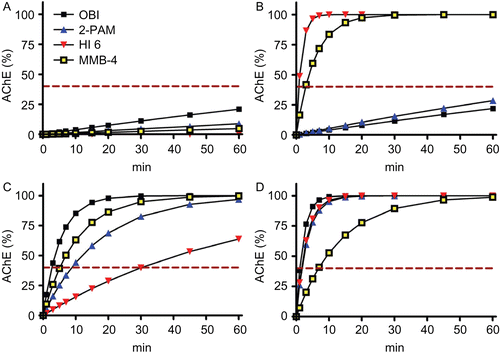
Reactivation of OP-inhibited AChE by oximes: model calculations
Initial model calculations were performed at following conditions:
Complete AChE inhibition without premature aging,
Use of fixed oxime concentrations at a clinical relevant level of established oximes, i.e. 10 and 100 µMCitation3,Citation26, and
Use of four different affinities and reactivities of theoretical oximes in the limits of experimentally determined numbers ()Citation16.
One important determinant for the efficacy of oximes is the reactivation velocity (half-time t1/2) which is determined by the reactivity (kr) and affinity (1/KD) as well as by the concentration of an oxime (Equation 1)Citation2.
shows the reactivation half-time in relation to the reactivity rate constants at different KD values. Hereby, a t1/2 of 5 min was considered as upper limit since this was shown to be a reasonable value in OP pesticide poisoned patientsCitation36. This example demonstrates that a certain level of oxime reactivity and affinity is necessary to achieve the desired reactivation half-time. At clinically relevant oxime concentrations, i.e. between 10 and 100 µM, kr of 0.1 min−1 at a KD ~ 10 µM would be the lower limit. Consequently, markedly higher kr values would be necessary at lower affinities of the oxime. shows additionally the corresponding reactivation half-times of obidoxime with tabun-inhibited AChE and of obidoxime, pralidoxime, and HI-6 with cyclosarin-inhibited AChE at an oxime concentration of 100 µM. This example demonstrates the relevance of adequate reactivities and affinities of oximes.
Figure 4. Double-logarithmic plot showing the relationship between reactivation half-time (t1/2, min) and oxime reactivity (kr in min−1). The t1/2 was calculated using equation (1) assuming five different dissociation constants (KD), i.e. 1 µM, 10 µM, 100 µM, 1000 µM, and 3000 µM for two different oxime concentrations, i.e. 10 µM (A) and 100 µM (B). The dashed line indicates a reactivation t1/2 of 5 min. In addition, reactivation half-times of selected oximes and organophosphorus compounds were included using measured reactivation rate constants (cf. ): Tabun and obidoxime (x), cyclosarin and pralidoxime (+), obidoxime (*), and HI-6 (▪).
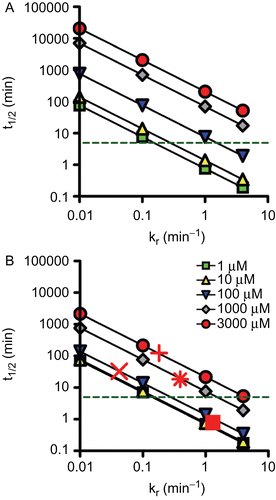
Apart from the reactivation velocity the oxime concentration required for the regeneration of a defined part of inhibited AChE is an important factor for defining properties of oximesCitation2.
Equation 2 may be used for calculating the oxime concentration necessary to obtain a certain fraction of reactivated enzyme within a given time in the absence of excess inhibitorCitation17. At clinically relevant conditions, i.e. 40% reactivation within 10 min (i.e. 2 × t1/2), the minimal requirements for an oxime would be a kr of 0.1 min−1 and a KD of 100 µM (). Lower oxime reactivity would result in an enormous rise of the required oxime concentration far above the therapeutic range while higher KD values could be compensated by a substantial increase in reactivity. At the anticipated conditions (40% reactivation within 10 min) and a kr < 0.052 min−1 the denominator would become positive resulting in negative oxime concentrations. Therefore, shows only calculations with kr values of 0.1, 1, and 4 min−1. Again, the results of the model calculations are supported by calculating the necessary oxime concentrations for obidoxime, pralidoxime, and HI-6. It becomes evident that for the adequate reactivation of cyclosarin-inhibited AChE by pralidoxime extraordinary high and toxic oxime concentrations would be required to achieve the desired reactivation. On the other hand, the high reactivity and affinity of HI-6 would result in adequate reactivation with very low oxime concentrations.
Figure 5. Double-logarithmic plot showing the relationship between oxime concentration (µM) and dissociation constant (KD in µM). Oxime concentrations necessary to obtain 40% reactivation of inhibited acetylcholinesterase within 10 min were calculated according toCitation17 for three different oxime reactivity constants (kr) of 0.1, 1 and 4 min−1. The hatched area resembles the range of clinically used oxime concentrations. In addition, necessary oxime concentrations of selected oximes and organophosphorus compounds were included using measured reactivation rate constants (cf. ): Cyclosarin and pralidoxime (+), obidoxime (*), and HI-6 (▪).
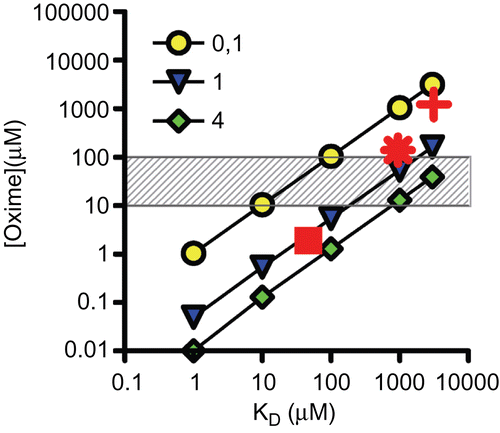
Affinity of oximes towards native AChE and therapeutic concentration
Oximes inhibit reversibly AChECitation7 and the dissociation constant Ki of the oxime from the substrate-free human AChE was determined to be in the range of 150–500 µM for obidoxime, pralidoxime, HI-6, TMB-4, and MMB-4Citation2,Citation37–40. The consequence of the intrinsic inhibitory potency of oximes, which may contribute to the observed oxime toxicity at high dosesCitation41,Citation42, is a limitation of the therapeutic oxime dose and the resulting in vivo oxime concentration. Consequently, recommended therapeutic obidoxime and pralidoxime concentrations are in the range of 10–20 and 100 µM, respectivelyCitation3,Citation36.
The relationship between the affinity towards native and OP-inhibited human AChE was investigated by Pang and co-workers with a homologous series of alkylene-linked bis-pyridinium aldoximes, i.e. Ortho-3 to Ortho-9Citation43. It could be shown that the increase of affinity of the oximes towards diethyl-phosphoryl-inhibited AChE, i.e. KD from 21 (Ortho-3) to 0.6 µM (Ortho-9), was accompanied by an increasing inhibitory potency of the compounds, i.e. Ki from 65 (Ortho-3) to 0.3 µM (Ortho-9). Consequently, the LD50 of Ortho-7 (Ki 1.3 µM) was less than 5 µmol/kg in ratsCitation44 while the LD50 of pralidoxime in rats is approximately 1 mmol/kgCitation42.
These data demonstrate that a simultaneous increase of oxime affinity towards native and OP-inhibited AChE in the absence of a substantial increase in reactivity does not necessarily result in an improved therapeutic benefit due to the reduced side effect-free oxime concentration. Desirable would be a selective affinity of an oxime towards OP-inhibited AChE, a requirement that seems difficult to be accomplished in view of the huge number of structurally different OP.
Conclusions
The theoretical considerations based on the kinetic properties of oximes indicate that oximes presently used clinically (obidoxime, pralidoxime) or under development for human use (HI-6, MMB-4) should be adequate reactivators for a larger number of OP pesticides and nerve agents. Established and experimental oximes are inadequate against particular OP, e.g. tabun, fenamiphos, and methylfluorophosphonylcholines, due to low affinity and reactivity. Future developments of more effective oximes should consider kinetic demands by attempting to achieve an at least moderate reactivity and affinity, preferentially towards OP-inhibited AChE. A minimal kr of 0.1 min−1 and a KD lower than 100 µM would be the minimal requirements for a rapid and sufficient reactivation of inhibited AChE.
The availability of effective reactivators does not necessarily imply that such oximes are effective antidotes in vivo. Presence of high OP concentrations, e.g. in intentional self-poisoning by pesticides, may lead to re-inhibition of reactivated AChE thus impairing net reactivationCitation45. In addition, premature and ongoing aging of OP-inhibited AChE affects oxime effectivenessCitation46. Finally, the in vivo efficacy of oximes is significantly determined by a proper dosing and an adequate duration of oxime administrationCitation26.
Declaration of interest
The authors declare that they do not have any conflicts of interest
References
- Namba T, Hiraki K. PAM (pyridine-2-aldoxime methiodide) therapy for alkylphosphate poisoning. JAMA 1958;166:1834–1839.
- Eyer P. The role of oximes in the management of organophosphorus pesticide poisoning. Toxicol Rev 2003;22:165–190.
- Eddleston M, Eyer P, Worek F, Juszczak E, Alder N, Mohamed F, Senarathna L, Hittarage A, Azher S, Jeganathan K, Jayamanne S, von Meyer L, Dawson AH, Sheriff MH, Buckley NA. Pralidoxime in acute organophosphorus insecticide poisoning–a randomised controlled trial. PLoS Med 2009;6:e1000104.
- Eddleston M, Buckley NA, Eyer P, Dawson AH. Management of acute organophosphorus pesticide poisoning. Lancet 2008;371:597–607.
- Jokanovic M. Medical treatment of acute poisoning with organophosphorus and carbamate pesticides. Toxicol Lett 2009;190:107–115.
- Finkelstein Y, Kushnir A, Raikhlin-Eisenkraft B, Taitelman U. Antidotal therapy of severe acute organophosphate poisoning: a multihospital study. Neurotoxicol Teratol 1989;11:593–596.
- Eyer P, Worek F. Oximes. In: Marrs TC, Maynard RL, Sidell FR, eds. Chemical Warfare Agents: Toxicology and Treatment. Chichester: John Wiley & Sons Ltd., 2007:305–29.
- Erdmann WD, von Clarmann. A new esterase reactivator for the treatment of alkylphosphate poisonings. Dtsch Med Wochenschr 1963;88:2201–2206.
- Xue SZ, Ding XJ, Ding Y. Clinical observation and comparison of the effectiveness of several oxime cholinesterase reactivators. Scand J Work Environ Health 1985;11(Suppl 4):46–48.
- Kusic R, Jovanovic D, Randjelovic S, Joksovic D, Todorovic V, Boskovic B, Jokanovic M, Vojvodic V. HI-6 in man: efficacy of the oxime in poisoning by organophosphorus insecticides. Hum Exp Toxicol 1991;10:113–118.
- Worek F, Eyer P, Aurbek N, Szinicz L, Thiermann H. Recent advances in evaluation of oxime efficacy in nerve agent poisoning by in vitro analysis. Toxicol Appl Pharmacol 2007;219:226–234.
- Reiner E, Simeon-Rudolf V. Pyridinium, imidazolium and quinuclidinium compounds: toxicity and antidotal effects against the nerve agents Tabun and Soman. Arh Hig Rada Toksikol 2006;57:171–179.
- Hobbiger F. Reactivation of phosphorylated acetylcholinesterase. In: Koelle GB, ed. Cholinesterases and Anticholinesterase Agents. Berlin: Springer-Verlag, 1963:921–988.
- Hagedorn I, Gündel WH, Schoene K. Reaktivierung phosphorylierter acetylcholin-esterase mit oximen: beitrag zum studium des reaktionsablaufes. Drug Res 1969;19:603–606.
- Bismuth C, Inns RH, Marrs TC. Efficacy, toxicity and clinical use of oximes in anticholinesterase poisoning. In: Ballantyne B, Marrs TC, eds. Clinical and Experimental Toxicology of Organophosphates and Carbamates. Oxford: Butterworth & Heinemann, 1992:555–577.
- Worek F, Thiermann H, Szinicz L, Eyer P. Kinetic analysis of interactions between human acetylcholinesterase, structurally different organophosphorus compounds and oximes. Biochem Pharmacol 2004;68:2237–2248.
- Worek F, Reiter G, Eyer P, Szinicz L. Reactivation kinetics of acetylcholinesterase from different species inhibited by highly toxic organophosphates. Arch Toxicol 2002;76:523–529.
- Worek F, Thiermann H, Szinicz L. Reactivation and aging kinetics of human acetylcholinesterase inhibited by organophosphonylcholines. Arch Toxicol 2004;78:212–217.
- Bartling A, Worek F, Szinicz L, Thiermann H. Enzyme-kinetic investigation of different sarin analogues reacting with human acetylcholinesterase and butyrylcholinesterase. Toxicology 2007;233:166–172.
- Worek F, Aurbek N, Koller M, Becker C, Eyer P, Thiermann H. Kinetic analysis of reactivation and aging of human acetylcholinesterase inhibited by different phosphoramidates. Biochem Pharmacol 2007;73:1807–1817.
- Aurbek N, Thiermann H, Szinicz L, Eyer P, Worek F. Analysis of inhibition, reactivation and aging kinetics of highly toxic organophosphorus compounds with human and pig acetylcholinesterase. Toxicology 2006;224:91–99.
- Worek F, Aurbek N, Wetherell J, Pearce P, Mann T, Thiermann H. Inhibition, reactivation and aging kinetics of highly toxic organophosphorus compounds: pig versus minipig acetylcholinesterase. Toxicology 2008;244:35–41.
- Worek F, Herkert NM, Koller M, Aurbek N, Thiermann H. Interaction of pentylsarin analogues with human acetylcholinesterase: a kinetic study. Toxicol Lett 2009;187:119–123.
- Eddleston M, Eyer P, Worek F, Mohamed F, Senarathna L, von Meyer L, Juszczak E, Hittarage A, Azhar S, Dissanayake W, Sheriff MH, Szinicz L, Dawson AH, Buckley NA. Differences between organophosphorus insecticides in human self-poisoning: a prospective cohort study. Lancet 2005;366:1452–1459.
- Dawson RM. Review of oximes available for treatment of nerve agent poisoning. J Appl Toxicol 1994;14:317–331.
- Thiermann H, Worek F, Eyer F, Eyer P, Felgenhauer K, Zilker T. Obidoxime in acute organophosphate poisoning: 2—PK/PD relationships. Clin Toxicol 2009;47:807–813.
- Eyer F, Worek F, Eyer P, Felgenhauer K, Haberkorn M, Zilker T, Thiermann H. Obidoxime in acute organophosphate poisoning: 1—clinical effectiveness. Clin Toxicol 2009;47:798–806.
- Peter JV, Moran JL, Graham P. Oxime therapy and outcomes in human organophosphate poisoning: an evaluation using meta-analytic techniques. Crit Care Med 2006;34:502–510.
- Cherian MA, Roshini C, Peter JV, Cherian AM. Oximes in organophosphorus poisoning. Ind J Crit Care Med 2005;9:155–163.
- de Silva HJ, Wijewickrema R, Senanayake N. Does pralidoxime affect outcome of management in acute organophosphorus poisoning? Lancet 1992;339:1136–1138.
- Pawar KS, Bhoite RR, Pillay CP, Chavan SC, Malshikare DS, Garad SG. Continuous pralidoxime infusion versus repeated bolus injection to treat organophosphorus pesticide poisoning: a randomised controlled trial. Lancet 2006;368:2136–2141.
- Holmstedt B. Pharmacology of organophosphorus cholinesterase inhibitors. Pharmacol Rev 1959;11:567–688.
- Eckert S, Eyer P, Herkert N, Bumm R, Weber G, Thiermann H, Worek F. Comparison of the oxime-induced reactivation of erythrocyte and muscle acetylcholinesterase following inhibition by sarin or paraoxon, using a perfusion model for the real-time determination of membrane-bound acetylcholinesterase activity. Biochem Pharmacol 2008;75:698–703.
- Herkert NM, Eckert S, Eyer P, Bumm R, Weber G, Thiermann H, Worek F. Identical kinetics of human erythrocyte and muscle acetylcholinesterase with respect to carbamate pre-treatment, residual activity upon soman challenge and spontaneous reactivation after withdrawal of the inhibitors. Toxicology 2008;246:188–192.
- Thiermann H, Zilker T, Eyer F, Felgenhauer N, Eyer P, Worek F. Monitoring of neuromuscular transmission in organophosphate pesticide-poisoned patients. Toxicol Lett 2009;191:297–304.
- Thiermann H, Szinicz L, Eyer F, Worek F, Eyer P, Felgenhauer N, Zilker T. Modern strategies in therapy of organophosphate poisoning. Toxicol Lett 1999;107:233–239.
- Mast U. Reaktivierung der erythrozyten-acetylcholinesterase durch Oxime. Thesis, University Munich, 1997.
- Kovarik Z, Calic M, Sinko G, Bosak A. Structure-activity approach in the reactivation of tabun-phosphorylated human acetylcholinesterase with bis-pyridinium para-aldoximes. Arh Hig Rada Toksikol 2007;58:201–209.
- Skrinjaric-Spoljar M, Kralj M. Reactivating and protective effects of pyridinium compounds in human erythrocyte acetylcholinesterase inhibition by organophosphates in vitro. Arch Toxicol 1980;45:21–27.
- Skrinjaric-Spoljar M, Simeon V, Reiner E, Krauthacker B. Bis-pyridinium compounds: inhibition of human erythrocyte acetylcholinesterase and protection of the enzyme against phosphylation. Acta Pharmacol Jugoslav 1988;38:101–109.
- Calesnick B, Christensen, Richter M. Human toxicity of various oximes. 2-Pyridine aldoxime methyl chloride, its methane sulfonate salt, and 1,1′-trimethylenebis-(4-formylpyridinium chloride). Arch Environ Health 1967;15:599–608.
- Marrs TC. Toxicology of oximes used in treatment of organophosphate poisoning. Adverse Drug React Toxicol Rev 1991;10:61–73.
- Pang YP, Kollmeyer TM, Hong F, Lee JC, Hammond PI, Haugabouk SP, Brimijoin S. Rational design of alkylene-linked bis-pyridiniumaldoximes as improved acetylcholinesterase reactivators. Chem Biol 2003;10:491–502.
- Hammond PI, Kern C, Hong F, Kollmeyer TM, Pang YP, Brimijoin S. Cholinesterase reactivation in vivo with a novel bis-oxime optimized by computer-aided design. J Pharmacol Exp Ther 2003;307:190–196.
- Eyer F, Meischner V, Kiderlen D, Thiermann H, Worek F, Haberkorn M, Felgenhauer N, Zilker T, Eyer P. Human parathion poisoning. A toxicokinetic analysis. Toxicol Rev 2003;22:143–163.
- Lotti M. Cholinesterase inhibition: complexities in interpretation. Clin Chem 1995;41:1814–1818.