Abstract
A series of novel conformationally restricted N1-arylsulfonyl-3-aminoalkoxy indoles were designed and synthesized as 5-HT6 receptor (5-HT6R) ligands. Many of the synthesized compounds have moderate in vitro-binding affinities at 5-HT6R. The lead compound 8b (% inhibition = 97.2 at 1 μM) from this series has good pharmacokinetic profile in male Wister rats and is active in animal model of cognition like Morris water maze. The details of chemistry, SAR, pharmacokinetics and pharmacological data constitute the subject matter of this report.
Introduction
5-HT6R was first identified by molecular cloning in the ratCitation1,Citation2, then in manCitation3 and subsequently in mouseCitation4. These receptors are predominantly expressed in the central nervous system, including the olfactory tubercle, nucleus accumbens, striatum, hippocampus and cerebral cortex, with a notably higher level of expression in the striatum of both rat and human brains. Due to the high presence of 5-HT6R in brain, the 5-HT6R active ligands play an important role in CNS disorders such as schizophrenia, dementia, Alzheimer’s disease, Parkinson’s disease and other neurodegenerative disorders. Various structurally diverse small molecules containing the basic nitrogen (for primary binding) and the two other aromatic sites (for secondary binding) have been known to bind selectively at 5-HT6RCitation5–8. In the past decade various molecules like SB-742457, SUVN-502, PRX-07034, SAM-531, SAM-315, LY-483518 and Lu AE58054 were reported as 5-HT6R ligands with positive clinical dataCitation9–13.
Among these reported 5-HT6R ligands, a few were reported with conformationally constrained features. There are reports by Glennon et al. with fused tryptamine side chain in six member ring (fused cyclohexyl type) that makes it conformationally restricted,Citation14 viz., tetrahydrocarbazole derivatives (Compound 1 in ). Although there was change in their spatial orientations, the compounds were active towards 5-HT6R. Similarly, GSK and Wyeth (Compound 4 and 5 in ) also reported potent 5-HT6R ligands with fused indole scaffoldCitation15,Citation16; their focus also was mainly on side chain and indole template. Although there was substantial amount of work reported with constrained or fused tryptamines, not much work was reported around conformationally restricted sulfonamides. Recently, we at Suven reported constrained pyrrolidinyl indolesCitation17 and indole sulfonamidesCitation18,Citation19, as 5-HT6R ligands (Structures 2 and 3 in ). These series of compounds have good affinity towards 5-HT6R with selectivity over other known GPCRs. The compounds were active in in vivo models of cognition like Morris water maze and NORT. These compounds have acceptable pharmacokinetic profile for further development. Based on this knowledge and observations, we have designed and synthesized a series of 3-aminoalkoxy indole derivatives with constrained sulfonamide ring (Compound 8b in ). The lead compound, 8b was evaluated for pharmacokinetic parameters, % surrogate metabolism, human CYP3A4 and 2D6 liabilities. Further, the compound 8b was tested in animal model of cognition, viz., Morris water maze. The results of these studies are discussed in this communication.
Results and discussion
Structure activity relationship
As a part of our SAR studies compounds 8a–8o were synthesized and evaluated for their binding affinities at human 5-HT6 receptor at 1 μM concentration and a few of them for Ki values (). Among these compounds, the derivatives 8a, 8b, 8g, 8j and 8n were found to have highest binding affinities with percent inhibition, at 1 μM concentration, being 82.3%, 97.2%, 74.13%, 74.28% and 86.38%, respectively.
Table 1. 5-HT6R binding affinities.
Substitutions tried on ring A were mostly 2-alkoxy and 2-halo. The compound 8d, with 2-methoxy substitution, was active with Ki of 127 nM, while the compound 8c, with 2-chloro substitution has a Ki of 464 nM. This shows that 2-chloro substitution was detrimental for the activity.
Based on our SAR efforts in different tryptamine type of compoundsCitation20, modification was carried out in N,N-dimethylaminoethoxy side chain at C-3 of indole with the insertion of methyl at α or β positions with respect to terminal nitrogen. Among the various derivatives prepared with this modified side chain, the most active derivative 8n (86.38% inhibition) has 2-methoxy substitution with methyl at β position in side chain. Its analogue with methyl substitution at α position with respect to the tertiary nitrogen (8f) has less binding affinity (68.26% inhibition) towards 5-HT6R. The compound 8a, without any substitution in the side chain, has 82.3% inhibition, while the α-methyl analogue 8j has slightly reduced potency, with 74.28% inhibition. The derivative 8m with 3-fluoro substitution on ring A and α-methyl group in the side chain was least active with 46.68% inhibition at 5-HT6R. Its analogue 8g, with β-methyl group in the side chain was found to have better binding affinity (74.13% inhibition), indicating that the position of methyl group in the side chain has an important role to play regarding a favourable conformation for binding at 5-HT6R.
Furthermore, some of the selected compounds were profiled for their selectivity against a few receptors including 5-HT receptor subtypes like 5-HT4, 5-HT7 and Muscarinic M1. Compounds were found to have IC50 > 10,000 nM for these panel of CNS receptors ().
Table 2. Selectivity profile of compounds on other receptors.
The compound 8b was tested in cell-based assay for 5-HT4 receptor binding along with the standard, GR113808 (IC50 = <0.1 nM). The compound 8b has no affinity (IC50 ≥ 10,000 nM) towards this receptor (see ).
Similarly, the compound 8b was tested in cell-based assays for 5-HT7 receptor and Muscarinic M1 receptor binding, the standards being Clozapine (IC50 = 1 nM) and Atropine (IC50 = 1 nM), respectively (see and ). As can be seen the compound was inactive towards these receptors.
Before testing the analogs in rodent behavioral assays in vivo, the Pharmacokinetic and ADME surrogate properties were determined in rat, the species ultimately used for behavioral models.
The active compounds, 8a and 8b from this series, were tested for their human cytochrome P450 inhibitory potential. The selected compound, 8b was found to have an IC50 value of 5.06 μM and 37.05 μM for CYP2D6 and CYP3A4 respectively, while the compound 8a was having IC50 values 7.65 μM and >45 μM for CYP2D6 and CYP3A4 respectively. Further, the compounds 8a and 8b were evaluated for their surrogate % metabolism in rat and human liver microsomes. The compound 8b has high metabolism (95.59%) in human liver microsomes, while it has relatively lower metabolism in rat liver microsomes (77.54%). This is in contrast to the metabolism shown by compound 8a. The compound 8a has low metabolism in human liver microsomes (50.98%), whereas it has high metabolism in rat liver microsomes (100%) ()
Table 3. Human cytochrome P450 inhibitory data and % surrogate metabolism for compounds 8a and 8b.
The most potent 5-HT6R ligand, 8b from this series was further evaluated for its pharmacokinetic profile in male Wister rats (). The compound has high volume of distribution (17.5 l/kg) with high systemic clearance (8.2 ± 2.8 l/h/kg). The dose of 10 mg/kg was rapidly absorbed in rats and it had an elimination half life (t1/2) of 1.49 ± 0.26 h, when it was administered orally. The bioavailability (%F) was found to be 35 ± 5% and the observed Cmax value was 0.31 ± 0.05 μg/ml.
Table 4. Pharmacokinetic profile of compound 8b in male Wister rats.
Since brain penetration was an important requirement for agents targeting CNS disorders, the steady state brain penetration study for compound 8b was carried out in male Wister rats (n = 4). 5 ml/h/kg constant infusion in 50% PEG was carried out over a period of 6 h. The steady state brain and plasma ratio (Cb/Cp) in male Wister rats was 9.04 ± 1.86, confirming high brain penetration for this compound.
The compound 8b was further evaluated for its efficacy in animal model of cognition like Morris water maze testCitation21.
Morris water maze test
The following protocol was followed for the experiment.
Protocol: Wister rats 10–12 weeks old were given 4 trials/day for 5 days; each trial was of 60 s with an ITI of 600 s. Each trial started with the rat placed facing the wall of the maze at one of the eight designated locations (0°, 90°, 180°, 270°) on 1st, 3rd and 5th day and (45°, 135°, 225°, 315°) on 2nd and 4th day. Compound 8b was administered by gavages during the acquisition phase. Scopolamine was administered at a dose of 0.6 mg/kg on the first day and the dose of scopolamine was gradually increased at a rate of 0.05 mg/kg/day. Compound 8b was administered 30 min before the administration of scopolamine. Compound 8b significantly reversed the spatial memory deficit induced by scopolamine in the Morris water maze trial indicating cognitive improvement potential of the compound ( and ).
Figure 5. Latency to target in Morris water maze task. Data represents mean ± SEM of latency to target recorded as time taken to locate the hidden platform in water. Compound 8b at 10 mg/kg p.o. reversed scopolamine-induced deficit and significant difference from scopolamine-treated group is indicated with asterisk (unpaired t-test; *p < 0.05).
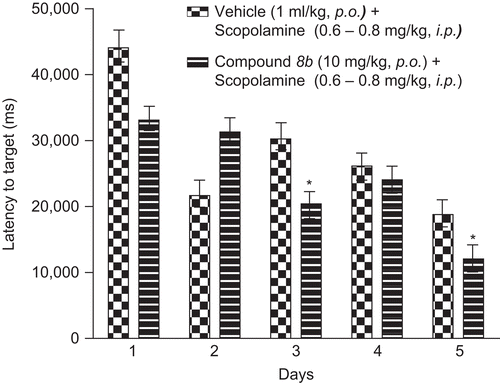
Figure 6. Path length to target in Morris water maze task. Data represents mean ± SEM of path length recorded as path taken to locate the hidden platform in water. Compound 8b at 10 mg/kg p.o. reversed scopolamine-induced deficit and significant difference from scopolamine-treated group is indicated with asterisk (unpaired t-test *p < 0.05; **p < 0.01).
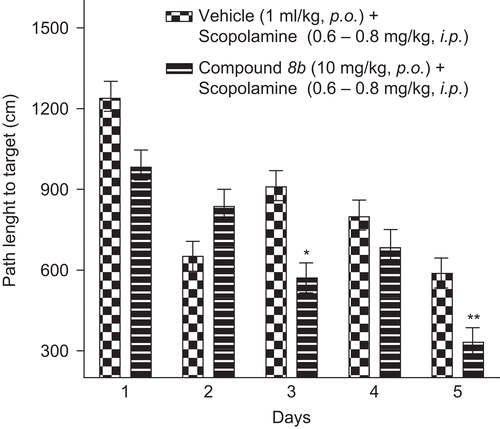
Materials and Methods
Infra red spectra were recorded on KBr disc and in solid state using Perkin-Elmer model 1600 FT-IR spectrophotometer (Perkin-Elmer, Norwalk, CT). Electrospray ionization mass spectra were recorded on a API 4000 triple quadrupole instrument (MDSSCIEX, Concord, Ontario, Canada). 1H-NMR spectra were obtained on a Bruker proton NMR spectrometer (Fallanden, Switzerland) at 400 MHz. Deuterated reagents were used as solvents and were commercially procured. Tetramethylsilane (TMS) was used as an internal standard. Chemical shift values are expressed in parts per million (d) and coupling constants are expressed in Hz. Chromatography refers to column chromatography performed using 60–120 mesh silica gel and executed under nitrogen pressure (flash chromatography) conditions. All the reagents and chemicals used were of ‘reagent grade’. Substituted benzene sulfonyl chlorides were synthesized in-house from the substituted benzene by chlorosulfonation or from the corresponding amines via the diazo intermediates.
Radioligand binding assay for human 5-HT6 receptor
Compounds were investigated by the reported procedureCitation22,Citation23. Briefly, receptor source and radioligand used were human recombinant expressed in HEK-293 cells and [3H] LSD (60–80 Ci/mmol), respectively. The final ligand concentration was 1.5 nM and non-specific determinant was methiothepin mesylate (0.1 µM). The reference compound and positive control was methiothepin mesylate. Reactions were carried out in 50 mM TRIS-HCl (pH 7.4) containing 10 mM MgCl2, 0.5 mM EDTA for 60 min at 37 °C. The reaction was terminated by rapid vacuum filtration onto glass fibre filters. Radioactivity trapped onto the filters was determined and compared to control values in order to ascertain any interactions of test compound(s) with the cloned serotonin-5-HT6 binding site.
Determination of IC50 values for 5-HT4 receptor binding, using cell-based functional assay
Recombinant CHO cells were established by transfecting an expression vector encoding human 5-HT4a gene and CRE-Luc reporter system. Isolated colonies were screened with 1000 nM 5-HT for the induction of luciferase reporter gene. The clone demonstrating highest 5-HT induced reporter gene was used in the evaluation of the known and novel compounds. Increasing concentrations of the compound were incubated with or without 1000 nM 5-HT for antagonist or agonist mode, respectively, for 5 h in 96 well plate. After incubation, cells were harvested, lysed and reporter gene activity was determined. The data was analyzed using Prism GraphPad software.
Determination of IC50 values for 5-HT7 receptor binding, using cell-based functional assay
CHO cells were transfected with an expression vector encoding rat 5-HT7 gene and CRE-Luc reporter system. Colonies were isolated and screened with 1000 nM 5-HT for the induction of luciferase reporter gene. The clone demonstrating highest 5-HT induced reporter gene was used in the evaluation of the known and novel compounds. Increasing concentrations of the compound were incubated with or without 1000 nM 5-HT for antagonist or agonist mode respectively for 5 h in 96 well plate. After incubation, cells were harvested, lysed and reporter gene activity was measured. The data was analyzed using Prism GraphPad software.
Determination of IC50 values for acetylcholine receptor M1 binding, using cell-based functional assay
Muscarinic acetylcholine receptor M1 was cloned into an expression vector and transfected in CHO cells along with CRE-Luc reporter system. Isolated colonies were screened with 10,000 nM acetylcholine. Clones exhibiting highest acetylcholine dependent reporter gene induction were utilized in the evaluation of known and novel compounds. Increasing concentrations of the compound were incubated with or without 10,000 nM acetylcholine for antagonist or agonist mode, respectively, for 5 h in 96 well plate. After incubation, cells were harvested, lysed and reporter gene activity was determined. The data was analyzed using Prism GraphPad software.
Synthesis
The general synthetic strategy followed for the preparation of the title compounds was summarized in . Various substituted indoxyls were synthesized using literature methodsCitation24,Citation25. More often, the indoxyls were synthesized starting from the appropriately substituted 2-bromobenzoic acids. The substituted 2-bromobenzoic acids were treated with Glycine to obtain the diacids (2). These diacids were then subjected to cyclization in presence of acetic anhydride and N,N-dimethylformamide at higher temperature (120 °C) followed by, in situ, decarboxylation to obtain the corresponding 1-acetyl-3-acetyloxy indoles (3). The compounds (3) were deacetylated under mild conditions using sodium sulfite and methanol/water (1:1) at room temperature to obtain indoxyls (4), which were used after purification for further synthesis.
Scheme 1. Reagents: (a) Glycine, water, NaOH; (b) DMF, NaOAc, Ac2O; (c) Na2SO3, MeOH/water; (d) THF, K2CO3, Me2NCH2CH2Cl, reflux; (e) NaOH, MeOH, reflux; (f) 2-Bromobenzenesulfonyl chloride, THF, KOH powder; (g) DMA, CH3COOK, P(PPh3)4Pd, 120°C.
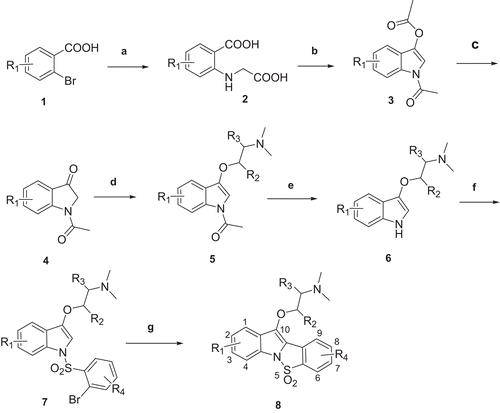
Various alternative methods were used for the etherification of indoxyls; the best system was found to be the one involving dimethylaminoethyl chloride in tetrahydrofuran in presence of potassium carbonate. Further, for the derivatization of N1 of indole, potassium hydroxide powder and tetrahydrofuran was found to be the best condition for sulfonylation. The N1 sulfonylated compound was further treated with dry potassium acetate and tetrakis triphenyl phosphino palladium (0) catalyst in N,N-dimethyl acetamide (DMA) solvent to obtain the desired compounds 8a–8r.
Synthesis of compounds with α-methyl and β-methyl substituents in the side chain were obtained following essentially the same route, using appropriately substituted dimethylamino ethyl chlorides, that were procured commercially.
Experimental
General (representative) procedure for the synthesis of compound 8d
Potassium carbonate (872 mmol) was added to a solution of 1-acetyl-6-chloro indoxyl (4) (436.3 mmol) in tetrahydrofuran (950 ml) and stirred at 25–30°C for 90 min. The free base of (2-Chloroethyl)dimethylamine hydrochloride (1308 mmol) was liberated and extracted into toluene by stirring over 350 ml toluene and a solution of sodium hydroxide (1308 mmol) in water (250 ml). The organic layer containing the free base was then separated and transferred to the above reaction mixture. The reaction mass was heated to reflux at 90–95°C and maintained for 4 h. After completion of the reaction (TLC), the reaction mass was cooled to 25–30°C and filtered the solid at suction and washed with ethyl acetate (250 ml × 3). The filtrate was collected and concentrated under reduced pressure to obtain oily product. The residue, thus obtained, was purified by flash chromatography over silica gel using 1% triethylamine (TEA) in ethyl acetate to get intermediate 5.
The intermediate 5 (61.5 mmol) dissolved in methanol (100 ml) and was added to a solution of sodium hydroxide (123 mmol) in water (100 ml), heated to 60–65°C and maintained for 2 h. After completion of the reaction (TLC), the mass was cooled to 25–30°C and distilled off methanol and water mixture to obtain oily mass. Water (1000 ml) was added to the residual mass and extracted the product with ethyl acetate (3 × 100 ml). The separated ethyl acetate layers were combined, washed with brine solution (100 ml), dried over anhydrous sodium sulphate and concentrated under reduced pressure to obtain a thick oily mass. The residue, thus obtained, was purified by flash chromatography over silica gel using 1% TEA in ethyl acetate to get intermediate 6, which was identified by IR, NMR and mass spectral analysis.
Potassium hydroxide powder (60.35 mmol) was added to a solution of intermediate 6 (30.1 mmol) in tetrahydrofuran (150 ml) and stirred the reaction mass for 1 h at 25–30 °C under nitrogen atmosphere. 2-Bromobenzenesulfonyl chloride (45.2 mmol) dissolved in tetrahydrofuran (100 ml) was added to the reaction mass drop wise over a period of 15 min. The reaction mass was stirred at 25–30 °C for 60 min. After completion (TLC) of the reaction, the solvent was distilled off completely under reduced pressure to get oil. Water (250 ml) was added to the residual mass and extracted the product with dichloromethane (2 × 200 ml). The combined organic layer was washed with brine solution, dried over anhydrous sodium sulphate and concentrated under reduced pressure. The residual mass, so obtained, was purified by flash chromatography over silica gel using 1% TEA in ethyl acetate to get intermediate 7, which was identified by IR, NMR and mass spectral analyses.
Dry potassium acetate (2.006 mmol) was added to a solution of intermediate 7 (1.18 mol) in DMA followed by tetrakis triphenyl phosphine palladium (0) catalyst (0.059 mmol) under nitrogen atmosphere. The reaction mass was heated to 110–120 °C and stirred for 1 h. After completion of the reaction (TLC), the reaction mass was cooled to 25–30 °C. Water (50 ml) was added and extracted product into ethyl acetate (100 ml × 3). The combined organic layer was washed with brine solution, dried over anhydrous sodium sulphate and concentrated under reduced pressure to obtain oily product. The residue, thus obtained, was purified by flash chromatography over silica gel using 1% methanol in chloroform to get compound 8d, which was identified by IR, NMR and mass spectral analysis.
10-(2-N,N-Dimethylaminoethoxy)-5-Thia-4b-azaindeno [2,1-a]indene-5,5-Dioxide (8a). Thick oil; IR (KBr, cm−1): 1179, 1334, 1441, 1620, 2944; mass (m/z): 343.2 [M + H]+; 1H-NMR (ppm): 2.37 (6H, s, N(CH3)2), 2.75–2.78 (2H, t, CH2-N(CH3)2), 4.38–4.41 (2H, t, CH2O), 7.24–7.26 (1H, m, C2-H), 7.38–7.46 (2H, m, C3-H and C7-H), 7.66–7.69 (3H, m, C1-H, C4-H and C8-H), 7.79–7.81 (1H, d, J = 7.84 Hz, C9-H), 7.93–7.94 (1H, d, J = 7.84 Hz, C6-H); HRMS: [M + H]+ C18H19N2O3S calc. 343.1116, found. 343.1112.
3-Chloro-10-(2-N,N-Dimethylaminoethoxy)-5-Thia-4b-aza indeno [2,1-a]indene-5,5-Dioxide (8b). M.R (°C): 104.5–109.8; IR (KBr, cm−1): 1175, 1310, 1397, 1630, 2789, 3620; mass (m/z): 377.1 [M + H]+; 1H-NMR (ppm): 2.36 (6H, s, N(CH3)2), 2.74–2.77 (2H, t, CH2-N(CH3)2), 4.35–4.38 (2H, t, CH2O); 7.20–7.23 (1H, dd, J = 8.50, 1.77 Hz, C2-H), 7.46–7.48 (1H, m, C8-H), 7.57–7.60 (1H, d, J = 8.47 Hz, C1-H), 7.67–7.69 (2H, m, C4-H and C7-H), 7.79–7.81 (1H, d, J = 7.88 Hz, C6-H), 7.92–7.94 (1H, d, J = 7.83 Hz, C9-H); HRMS: [M + H]+ C18H18ClN2O3S calc. 377.0726, found. 377.0730.
2-Chloro-10-(2-N,N-Dimethylaminoethoxy)-5-Thia-4b-aza indeno [2,1-a]indene-5,5-Dioxide (8c). Thick oil; IR (KBr, cm−1): 1174, 1317, 1433, 1621, 3430; mass (m/z): 377.1 [M + H]+; 1H-NMR (ppm): 2.38 (6H, s, N(CH3)2), 2.75–2.78 (2H, t, CH2-N(CH3)2), 4.34–4.37 (2H, t, CH2O); 7.32–7.35 (1H, dd, J = 8.65, 1.86 Hz, C3-H), 7.48–7.50 (1H, m, C8-H), 7.58–7.60 (1H, d, J = 8.77 Hz, C4-H), 7.65–7.68 (2H, m, C1-H and C7-H), 7.80–7.82 (1H, d, J = 7.86 Hz, C6-H), 7.94–7.96 (1H, d, J = 7.84 Hz, C9-H); HRMS: [M + H]+ C18H18ClN2O3S calc. 377.0726, found. 377.0732.
2-Methoxy-10-(2-N,N-Dimethylaminoethoxy)-5-Thia-4b-azaindeno[2,1-a]indene-5,5-Dioxide (8d). Thick oil; IR (KBr, cm−1): 1155, 1170, 1324, 1442, 1614, 3436; mass (m/z): 373.1 [M + H]+; 1H-NMR (ppm): 2.37 (6H, s, N(CH3)2), 2.74–2.77 (2H, t, CH2-N(CH3)2), 3.85 (3H, s, OCH3), 4.33–4.36 (2H, t, CH2O); 6.98–7.01 (1H, dd, J = 8.8, 2.0 Hz, C3-H), 7.10–7.11 (1H, d, J = 2.36 Hz, C1-H), 7.44–7.45 (1H, m, C7-H), 7.55–7.57 (1H, d, J = 8.88 Hz, C4-H), 7.63–7.67 (1H, m, C8-H), 7.78–7.80 (1H, d, J = 7.8 Hz, C6-H), 7.90–7.92 (1H, d, J = 7.8 Hz, C9-H); HRMS: [M + H]+ C19H21N2O4S calc. 373.1222, found. 373.1218.
2-Isopropoxy-10-(2-N,N-Dimethylaminoethoxy)-5-Thia-4b-azaindeno[2,1-a]indene-5,5-Dioxide (8e). M.R (°C): 70.4–74.1; IR (KBr, cm−1): 1177, 1333, 1461, 1686, 2975, 3461; mass (m/z): 401.4 [M + H]+; 1H-NMR (ppm): 1.35–1.37 (6H, d, (CH3)2CH-), 2.37 (6H, s, N(CH3)2), 2.74–2.77 (2H, t, CH2-N(CH3)2), 4.33–4.36 (2H, t, CH2O); 4.53–4.59 (1H, septet, CH(CH3)2), 6.97–7.00 (1H, dd, J = 8.87. 2.42 Hz, C3-H), 7.12 (1H, d, J = 2.29 Hz, C1-H), 7.43–7.45 (1H, m, C8-H), 7.54–7.56 (1H, d, J = 8.82 Hz, C4-H), 7.64 (1H, m, C7-H), 7.77–7.79 (1H, d, C6-H), 7.90–7.92 (1H, d, C9-H); HRMS: [M + H]+ C21H25N2O4S calc. 401.1535, found. 401.1530.
(±) 2-Methoxy-10-(2-N,N-Dimethylamino-2-methylethoxy)-5-Thia-4b-azaindeno[2,1-a]indene-5,5-Dioxide (8f). M.R (°C): 77.6–80.1; IR (KBr, cm−1): 1175, 1324, 1437, 1626, 2962, 3431; mass (m/z): 387.3 [M + H]+; 1H-NMR (ppm): 1.15–1.17 (3H, d, CH3-CH-), 2.37 (6H, s, N(CH3)2), 3.01–3.09 (1H, m, CH-CH3), 3.87 (3H, s, OCH3), 4.09–4.11 (1H, dd, CH2-CH), 4.30–4.34 (1H, dd, O-CH2), 6.98–7.01 (1H, dd, J = 8.8, 2.36 Hz, C3-H), 7.09–7.10 (1H, d, J = 2.36 Hz, C1-H), 7.43–7.45 (1H, m, C8-H), 7.55–7.58 (1H, d, J = 8.80 Hz, C4-H), 7.63–7.65 (1H, m, C7-H), 7.78–7.80 (1H, d, J = 7.8 Hz, C6-H), 7.88–7.90 (1H, d, J = 7.76 Hz, C9-H); HRMS: [M + H]+ C20H23N2O4S calc. 387.1378, found. 387.1375.
(±) 3-Fluoro-10-(2-N,N-Dimethylamino-1-methylethoxy)-5-Thia-4b-aza indeno [2,1-a]indene-5,5-Dioxide (8g). M.R (°C): 82.6–85.5; IR (KBr, cm−1): 1184, 1337, 1617, 2721, 3434; mass (m/z): 375.2 [M + H]+; 1H-NMR (ppm): 1.33–1.35 (3H, d, J = 5.4 Hz, CH3-CH), 2.37 (6H, s, N(CH3)2), 2.49–2.54 (1H, m, CH2-N(CH3)2), 2.75–2.80 (1H, m, CH2-N(CH3)2), 4.55–4.60 (1H, m, J = 5.4 Hz, CH-CH3), 6.99–7.0 (1H, m, J = 2.2 Hz, C2-H), 7.39–7.40 (1H, dd, C4-H), 7.44–7.46 (1H, m, C7-H), 7.61–7.66 (2H, m, C1-H and C8-H), 7.79–7.81 (1H, d, J = 7.8 Hz, C9-H), 7.93–7.95 (1H, d, J = 7.8 Hz, C6-H); HRMS: [M + H]+ C19H20FN2O3S calc. 375.1178, found. 375.1175.
(±) 2-Chloro-10-(2-N,N-Dimethylamino-2-methylethoxy)-5-Thia-4b-aza indeno [2,1-a]indene-5,5-Dioxide (8h). Thick oil; IR (KBr, cm−1): 1172, 1331, 1317, 1437, 1620, 3435; mass (m/z): 391.3 [M + H]+; 1H-NMR (ppm): 1.15–1.16 (3H, d, J = 5.4 Hz, CH3-CH), 2.37 (6H, s, N(CH3)2), 3.01–3.11 (1H, m, CH-N(CH3)2), 4.10–4.11 (1H, m, O-CH2), 4.29–4.34 (1H, m, O-CH2), 7.32–7.35 (1H, dd, J = 8.60, 1.88 Hz, C3-H), 7.48–7.49 (1H, m, C7-H), 7.58–7.60 (1H, d, J = 8.70 Hz, C4-H), 7.64–7.65 (1H, d, J = 1.68 Hz, C1-H), 7.66–7.68 (1H, m, C8-H), 7.80–7.82 (1H, d, J = 7.80 Hz, C6-H), 7.91–7.93 (1H, d, J = 7.8 Hz, C9-H); HRMS: [M + H]+ C19H20ClN2O3S calc. 391.0883, found. 391.0886.
(±) 2-Chloro-10-(2-N,N-Dimethylamino-1-methylethoxy)-5-Thia-4b-aza indeno [2,1-a]indene-5,5-Dioxide (8i). M.R (°C): 88.6–92.0; IR (KBr, cm−1): 1177, 1346, 1443, 1598, 2931, 3436; mass (m/z): 391.2 [M + H]+; 1H-NMR (ppm): 1.34–1.35 (3H, d, J = 6.24 Hz, CH3-CH), 2.37 (6H, s, N(CH3)2), 2.49–2.53 (1H, m, CH2-N(CH3)2), 2.76–2.81 (1H, m, CH2-N(CH3)2), 4.52–4.57 (1H, m, J = 6.24 Hz, CH-CH3), 7.32–7.34 (1H, dd, J = 8.56, 1.92 Hz, C3-H), 7.47–7.49 (1H, m, C7-H), 7.58–7.60 (1H, d, J = 8.64 Hz, C4-H), 7.67–7.68 (2H, m, C1-H and C8-H), 7.80–7.82 (1H, d, J = 7.88 Hz, C6-H), 7.98–7.99 (1H, d, J = 7.84 Hz, C9-H); HRMS: [M + H]+ C19H20ClN2O3S calc. 391.0883, found. 391.0887.
(±) 10-(2-N,N-Dimethylamino-2-methylethoxy)-5-Thia-4b-aza indeno [2,1-a]indene-5,5-Dioxide (8j). M.R (°C): 60.1–63.3; IR (KBr, cm−1): 1180, 1335, 1441, 1619, 2967; mass (m/z): 357.1 [M + H]+; 1H-NMR (ppm): 1.15–1.17 (3H, d, CH3CH), 2.37 (6H, s, N(CH3)2), 3.01–3.09 (1H, m, CH-CH3), 4.09–4.11 (1H, dd, O-CH2), 4.30–4.34 (1H, dd, O-CH2), 7.32–7.34 (1H, dd, J = 8.56, 1.92 Hz, C3-H), 7.24–7.26 (1H, m, C2-H), 7.38–7.46 (2H, m, C6-H and C7-H), 7.66–7.69 (3H, m, C1-H, C4-H and C8-H), 7.79–7.81 (1H, d, J = 7.84 Hz, C9-H); HRMS: [M + H]+ C19H21N2O3S calc. 357.1273, found. 357.1270.
2-Ethoxy-10-(2-N,N-Dimethylaminoethoxy)-5-Thia-4b-aza indeno [2,1-a]indene-5,5-Dioxide (8k). M.R (°C): 101.2–103.8; IR (KBr, cm−1): 1175, 1234, 1315, 1441, 1622, 3615; mass (m/z): 387.5 [M + H]+; 1H-NMR (ppm): 1.40–1.46 (3H, t, CH3CH2O), 2.37 (6H, s, N(CH3)2), 2.74–2.77 (2H, t, CH2-N(CH3)2), 4.05–4.10 (2H, q, CH2O), 4.33–4.36 (2H, t, CH2O), 6.98–7.01 (1H, dd, J = 8.92, 2.44 Hz, C3-H), 7.09–7.10 (1H, d, J = 2.32 Hz, C1-H), 7.43–7.45 (1H, m, C7-H), 7.54–7.57 (1H, d, J = 8.80 Hz, C4-H), 7.62–7.64 (1H, m, C6-H), 7.77–7.79 (1H, d, J = 7.88 Hz, C8-H), 7.90–7.92 (1H, d, J = 7.84 Hz, C9-H); HRMS: [M + H]+ C20H23N2O4S calc. 387.1378, found. 387.1375.
(±) 10-(2-N,N-Dimethylamino-1-methylethoxy)-5-Thia-4b-aza indeno [2,1-a]indene-5,5-Dioxide (8l). Thick oil; IR (KBr, cm−1): 1180, 1335, 1441, 1614, 2940; mass (m/z): 357.2 [M + H]+; 1H-NMR (ppm): 1.34–1.36 (3H, d, J = 6.20 Hz, CH3-CH), 2.37 (6H, s, N(CH3)2), 2.51–2.55 (1H, m, CH2-N(CH3)2), 2.76–2.81 (1H, m, CH2-N(CH3)2), 4.61–4.65 (1H, m, J = 6.20 Hz, CH-CH3), 7.24–7.26 (1H, m, C2-H), 7.35–7.46 (2H, m, C3-H and C7-H), 7.63–7.69 (3H, m, C1-H, C4-H and C8-H), 7.79–7.81 (1H, d, J = 7.84 Hz, C9-H), 7.95–7.97 (1H, d, J = 7.84 Hz, C6-H); HRMS: [M + H]+ C19H21N2O3S calc. 357.1273, found. 357.1278.
(±) 3-Fluoro-10-(2-N,N-Dimethylamino-2-methylethoxy)-5-Thia-4b-aza indeno [2,1-a]indene-5,5-Dioxide (8m). M.R (°C): 108.9–111.9; IR (KBr, cm−1): 1154, 1184, 1336, 1618, 2778, 2968; mass (m/z): 375.2 [M + H]+; 1H-NMR (ppm): 1.15–1.17 (3H, d, CH3CH), 2.37 (6H, s, N(CH3)2), 3.04–3.09 (1H, m, CH-CH3), 4.12–4.16 (1H, dd, CH2-CH), 4.32–4.36 (1H, dd, CH2-CH), 6.99–7.0 (1H, m, J = 2.2 Hz, C3-H), 7.39–7.40 (1H, dd, C4-H), 7.44–7.46 (1H, m, C7-H), 7.59–7.68 (2H, m, C1-H and C8-H), 7.79–7.81 (1H, d, J = 7.8 Hz, C9-H), 7.87–7.89 (1H, d, J = 7.8 Hz, C6-H); HRMS: [M + H]+ C19H20FN2O3S calc. 375.1178, found. 375.1175.
(±) 2-Methoxy-10-(2-N,N-Dimethylamino-1-methylethoxy)-5-Thia-4b-aza indeno [2,1-a]indene-5,5-Dioxide (8n). Thick oil; IR (KBr, cm−1): 1180, 1335, 1441, 1614, 2940; mass (m/z): 387.2 [M + H]+; 1H-NMR (ppm): 1.34–1.36 (3H, d, J = 6.20 Hz, CH3-CH), 2.37 (6H, s, N(CH3)2), 2.51–2.55 (1H, m, CH2-N(CH3)2), 2.76–2.81 (1H, m, CH2-N(CH3)2), 3.85 (3H, s, OCH3), 4.61–4.65 (1H, m, J = 6.20 Hz, CH-CH3), 6.98–7.01 (1H, dd, J = 8.8, 2.0 Hz, C3-H), 7.10–7.11 (1H, d, J = 2.36 Hz, C1-H), 7.44–7.45 (1H, m, C7-H), 7.55–7.57 (1H, d, J = 8.88 Hz, C4-H), 7.63–7.67 (1H, m, C8-H), 7.78–7.80 (1H, d, J = 7.8 Hz, C6-H), 7.90–7.92 (1H, d, J = 7.8 Hz, C9-H); HRMS: [M + H]+ C20H23N2O4S calc. 387.1378, found. 387.1375.
1-Fluoro-10-(2-N,N-Dimethylaminoethoxy)-5-Thia-4b-aza indeno [2,1-a]indene-5,5-Dioxide (8o). Thick oil; IR (KBr, cm−1): 1179, 1334, 1441, 1620, 2944; mass (m/z): 361.1 [M + H]+; 1H-NMR (ppm): 2.37 (6H, s, N(CH3)2), 2.75–2.78 (2H, t, CH2-N(CH3)2), 4.38–4.41 (2H, t, CH2O), 6.72–6.73 (1H, m, C2-H), 7.1–7.21 (2H, m, C3-H and C4-H), 7.31–7.33 (1H, m, C8-H), 7.55–7.57 (1H, d, J = 8.88 Hz, C7-H), 7.78–7.80 (1H, d, J = 7.8 Hz, C6-H), 7.90–7.92 (1H, d, J = 7.8 Hz, C9-H); HRMS: [M + H]+ C18H18FN2O3S calc. 361.1022, found. 361.1017.
Conclusion
The results indicate that these analogues were having moderate activity profile towards 5-HT6R. The most potent compound 8b from this series was active in animal model of cognition like Morris water maze, having good oral bioavailability (%F = 35) and brain penetration. Further SAR modification to improve the binding affinities, metabolic stability and to make these compounds adequately brain penetrant, is under progress.
Declaration of interest
The authors report no conflicts of interest. The authors alone are responsible for the content and writing of the paper.
References
- Monsma FJ Jr, Shen Y, Ward RP, Hamblin MW, Sibley DR. Cloning and expression of a novel serotonin receptor with high affinity for tricyclic psychotropic drugs. Mol Pharmacol 1993; 43:320–327.
- Ruat M, Traiffort E, Arrang JM, Tardivel-Lacombe J, Diaz J, Leurs R, Schwartz JC. A novel rat serotonin (5-HT6) receptor: molecular cloning, localization and stimulation of cAMP accumulation. Biochem Biophys Res Commun 1993; 193:268–276.
- Kohen R, Metcalf MA, Khan N, Druck T, Huebner K, Lachowicz JE, Meltzer HY, Sibley DR, Roth BL, Hamblin MW. Cloning, characterization, and chromosomal localization of a human 5-HT6 serotonin receptor. J Neurochem 1996; 66:47–56.
- Kohen R, Fashingbauer LA, Heidmann DE, Guthrie CR, Hamblin MW. Cloning of the mouse 5-HT6 serotonin receptor and mutagenesis studies of the third cytoplasmic loop. Brain Res Mol Brain Res 2001; 90:110–117.
- Liu KG, Robichaud AJ. 5-HT6 antagonists as potential treatment for cognitive dysfunction. Drug Dev Res 2009; 70:145–168.
- Werner JG, Cornelis JV. The serotonin 5-HT6 receptor: a viable drug target for treating cognitive deficits in Alzheimer’s disease. Exp Rev Neurother 2009; 9:1073–1085.
- Witty D, Mahmood A, Tsu TC. Advances in the design of 5-HT6 receptor ligands with therapeutic potential. Prog Med Chem 2009; 48:163–224.
- Gerard R, Herve S. 5-HT6 receptor antagonists as potential therapeutics for cognitive impairment. Curr Top in Med Chem 2010; 10:207–221.
- Zvartau-Hind M. 11th Int. Conf. on Alz. Dis. Rel. Dis. (July 26–31, Chicago), 2008; Abst 03–04–06.
- Mather-Edwards G. 11th Int. Conf. on Alz. Dis. Rel. Dis. (July 26–31, Chicago), 2008; Abst P4–341.
- Lesem M. 11th Int. Cong. of Schiz. Res. (March 28–April 1, Colorado Springs), 2007.
- Nirogi R, Kambhampati R, Shinde A, Kandikere V, Mudigonda K, Bhyrapuneni G, Jayarajan P, Abraham R, Mulla SM, Jasti V. 12th Int. Conf. on Alz. Dis. Rel. Dis. (July 11–16, Vienna), 2009; Abst P1–25.
- Maher-Edwards G, Zvartau-Hind M, Hunter AJ, Michael G, Gillian H, Gemma J, Maria D, Pauline W. Double-Blind, Controlled Phase II Study of a 5-HT6 receptor antagonist, SB-742457, in Alzheimer’s Disease. Curr Alz Res 2010; 7:374–385.
- Glennon RA, Lee M, Rangisetty JB, Dukat M, Roth BL, Savage JE, McBride A, Rauser L, Hufeisen S, Lee DK. 2-Substituted tryptamines: agents with selectivity for 5-HT(6) serotonin receptors. J Med Chem 2000; 43:1011–1018.
- Cole DC, Mahwah MA. Sulfonyltetrahydro-3H-benzo(E)indole-8-amine compounds as 5-HT6 ligands. US 2005/0101596.
- Liu KG, Lo JR, Comery TA, Zhang GM, Zhang JY, Kowal DM, Smith DL, Di L, Kerns EH, Schechter LE, Robichaud AJ. A regiospecific synthesis of a series of 1-sulfonyl azepinoindoles as potent 5-HT6 ligands. Bioorg Med Chem Lett 2008; 18:3929–3931.
- Ramakrishna VSN, Kambhampati RS, Shirsath VS, Jasti V. Novel indeno[2,1A] indanes and isoindolo[2,1-A]indoles WO 2005/066184.
- Jasti V, Ramakrishna VSN, Kambhampati RS, Battula SR, Veerareddy A, Rao VSVV. Novel tetracyclic arylsulfonyl indoles having serotonin receptor affinity useful as therapeutic agents, process for their preparation and pharmaceutical compositions containing them. WO 2004/000849.
- Ramakrishna VSN, Kambhampati RS, Shirsath VS, Jasti V. Benzothiazino indoles. WO 2005/005439.
- Ramakrishna VSN, Kambhampati RS, Shirsath VS, Jasti V. Tetracyclic 3-substituted indoles having serotonin receptor affinity. WO 2004/055026.
- Woolley ML, Bentley JC, Sleight AJ, Marsden CA, Fone KC. A role for 5-ht6 receptors in retention of spatial learning in the Morris water maze. Neuropharmacology 2001; 41:210–219.
- Assays performed at Novascreen Biosciences Corporation, Hanover, Maryland, USA.
- Monsma FJ Jr., Shen Y, Ward RP, Hamblin MW, Sibley DR. Molecular cloning and expression of novel serotonin receptor with high affinity for tricyclic psychotropic drugs. Mol Pharmacol 1993; 43:320–327.
- Conway SC, Gribble WG, Synthesis of 1-(Phenylsulfonyl)Indol-3-yl Trifluoromethanesulfonate. Heterocycles. 1990; 30:627–633.
- Su HCF, Tsou KC. Synthesis of bromo-substituted indoxyl esters for cytochemical demonstration of esterase activity. J Am Chem Soc 1960; 82:1187–1189.