Abstract
New series of 2,4-diaryl-3-azabicyclo[3.3.1]nonan-9-one 4′-phenylthiosemicarbazones (compounds 9–16) was obtained from the corresponding 2,4-diaryl-3-azabicyclo[3.3.1]nonan-9-ones. The synthesized compounds have been characterized by their elemental, analytical, and spectral studies. Besides, these reported compounds were screened for their antibacterial and antifungal activities against a spectrum of microbial organisms. These studies proved that against bacteria, compounds 10 and 11 against Bacillus subtilis, compound 13 against Salmonella typhi, show maximum inhibition potency at low concentration (6.25 μg/mL), whereas against fungal, compounds 11, 13, and 16 against Candida albicans and compounds 12 and 13 against Cryptococcus neoformans, showed beneficial antifungal activity at minimum concentration (6.25 μg/mL).
Introduction
Microbial infections are associated with rates of attributable morbidity and mortality [Citation1]. The resistance of common pathogens to standard antibiotic therapies is rapidly becoming a major public health problem throughout the world. The incidence of multidrug-resistant Gram-positive and Gram-negative bacteria is increasing and the infections caused by them are becoming problematic now-a-days [Citation2]. There is an urgent need for the discovery of new compounds endowed with antimicrobial activity.
Many compounds with a thiosemicarbazone moiety exhibit significant biological properties [Citation3] because thiourea unit (NHCSNH) can easily form chelation with metal ions like iron, zinc, magnesium, and so on. Thiosemicarbazones are a class of small molecules that have been evaluated against Plasmodium falciparum, Trypanosoma brucei, and Trypanosoma cruzi for various diseases. The effectiveness of thiosemicarbazone analogues in treating these diseases is reported due to their activity against cysteine proteinases including rhodesain [Citation4–10]. Moreover compounds with a thiosemicarbazones structure are known to possess tranquilizing, muscle relaxing, psychoanaleptic, hypnotic, ulcerogenic, antidepressant, antibacterial, antifungal, analgesic, and anti-inflammatory properties [Citation11–15].
Jeyaraman and Avila [Citation16] have reviewed the importance of bicyclic compounds as intermediates in the synthesis of a several physiologically active compounds. Similarly, Lijinsky and Taylor [Citation17] have found that the presence of substituents at both the α-positions to that of “N” in piperidin-4-one is important to exert marked biological properties. In addition, several interesting investigations have been made through piperidine-based heterocyclic compounds and exhibited numerous biological properties such as antibacterial, antifungal, antitumor, antiarrhythmic, antithrombic, calcium antagonist, hypotensive, and neuroleptic activities [Citation18,Citation19]. An essential component of the search for new leads in drug-designing program is the synthesis of molecules that are novel and resemble known biologically active molecule by virtue of the presence of some pharmacophoric groups. Certain small heterocyclic molecules act as highly functionalized scaffolds and are known pharmacophores of a number of biologically active and useful molecules. Apart from the biological importance, these bridged bicyclic compounds exhibit twin-chair, chair-boat, or twin-boat conformations possessing interesting stereochemistry. In connection with our earlier work and as part of our ongoing research programme [Citation20–25], we have planned to design a system that combines both bioactive azabicyclo[3.3.1]nonane and thiosemicarbazone components together to give a compact structure like title compounds.
Experimental
Microbiology
Materials. The bacterial strains, viz., Staphylococcus aureus (ATCC-25825), Bacillus subtilis (ATCC-451), Salmonella typhi (ATCC-24915), Escherichia coli (ATCC-25835), and Klebsiella pneumonia (ATCC-15490) and antifungal strains, viz., Cryptococcus neoformans (ATCC-3312), Candida albicans (ATCC-3122), Rhizopus oryzae (ATCC-9363), Aspergillus niger (ATCC-598), and Aspergillus flavus (ATCC-485) are procured from National Chemical Laboratory, Pune, India.
In vitro antibacterial and antifungal activity
The in vitro activities of the compounds were tested in Sabourauds dextrose broth (SDB; Hi-media, Mumbai, India) for fungi and in Nutrient broth (NB, Hi-media) for bacteria by the 2-fold serial dilution method [Citation26]. The test compounds were dissolved in dimethyl sulphoxide (DMSO) to obtain 1 mg/mL stock solutions. Seeded broth (broth containing microbial spores) was prepared in NB from 24-h-old bacterial cultures on nutrient agar (Hi-media) at 37°C ± 1°C, whereas fungal spores from 24-h to 7-day-old Sabourauds agar slant cultures were suspended in SDB. The colony-forming units (cfu) of the seeded broth were determined by plate count technique and adjusted with the help of McFarland standards in the range of 104–105 cfu per mL. The final inoculum size was 105 cfu/mL for antibacterial assay and 1.1–1.5 × 102 cfu/mL for antifungal assay. Testing was performed at pH 6.5 ± 0.2 for bacteria and pH 5.6 ± 0.2 for fungi. Exactly 0.2 mL of the solution of test compound was added to 1.8 mL of seeded broth to form the first dilution. One millilitre of the first dilution solution was diluted with a further 1 mL of the seeded broth to give the second dilution and so on till six such dilutions were obtained. A set of assay tubes containing only seeded broth was kept as control and likewise solvent controls were also run simultaneously. The tubes were incubated in biological oxygen demand (BOD) incubators at 37°C ± 1°C for bacteria and 28°C ± 1°C for fungi. The minimum inhibitory concentrations (MICs) were recorded by visual observations after 24 h (for bacteria) and 72–96 h (for fungi except C. albicans) of incubation. Ciprofloxacin and amphotericin B were used as standards for bacterial and fungal studies, respectively.
Chemistry
Thin-layer chromatography (TLC) was carried out to monitor the course of the reaction and purity of the product. The melting points were recorded in open capillaries and are uncorrected. IR spectra were recorded in KBr (pellet forms) on AVATAR-330 FT-IR spectrophotometer (Thermo Nicolet) and only noteworthy absorption levels (reciprocal centimetres) are listed. 1H NMR spectra were recorded at 500 MHz on BRUKER AMX 500 MHz spectrophotometer using CDCl3 as solvent and TMS as internal standard. 13C NMR spectra were recorded at 125.76 MHz on BRUKER AMX 500 MHz spectrometer in CDCl3. For compounds 1–7 (except compound 5), GCMS were recorded on GC model: Varian GC-MS-Saturn 2200 Thermo, capillary column VF5MS (5% phenyl–95% methyl polysiloxane), 30 m length, 0.25 mm internal diameter, 0.25 µm film thickness, temperature of column range from 50°C to 280°C (10°C/min), and injector temperature 250°C. For compounds 9–16, ES-HRMS was recorded on QTof-Mico YA 263 instrument except compounds 11 and 13.
By employing the literature precedent of Baliah and Jeyaraman [Citation27], all the parent 2,4-diaryl-3-azabicyclo[3.3.1]nonan-9-ones (compounds 1–8) were prepared by the condensation of appropriate ketones, aldehydes, and ammonium acetate in 1:2:1 ratio using ethanol as solvent.
2,4-Diphenyl-3-azabicyclo[3.3.1]nonan-9-one (compound 1)
IR (cm−1): 3317 (N–H stretching), 3057, 3026, 2926, 2857, 2794 (C–H stretching), 1708 (C=O stretching), 1598 (C=C stretching-Ph). 1H NMR (δ ppm): 7.54–7.30, (m, 10H, aryl protons); 4.39 (d, 2H, J = 2.40 Hz, H-2a and H-4a); 2.89 (m, 1H, H-7a); 2.47 (br s, 1H, H-1, H-5); 1.93 (dd, 1H, J = 5.86, 1.46 Hz, H-8e); 1.90 (dd, 1H, J = 5.88, 1.48 Hz, H-6e); 1.87 (br s, 1H NH); 1.74–1.64 (m, 2H, H-6a and H-8a); 1.38 (quin, 1H, H-7e). 13C NMR (δ ppm): 217.58 (C-9), 141.37 (C-2′ and C-4′), 128.61(C-2″ and C-4″), 127.62 (C-2″″ and C-4″″), 126.96 (C-2′″ and C-4′″); 64.81 (C-2 and C-4); 54.05 (C-1 and C-5); 29.14 (C-6 and C-8); 21.21 (C-7). GCMS: m/z = 292.22 (M+1).
2,4-Bis(4-fluorophenyl)-3-azabicyclo[3.3.1]nonan-9-one (compound 2)
IR (cm−1): 3318 (N–H stretching); 3071, 2970, 2921, 2852 (C–H stretching); 1707 (C=O stretching); 1603 (C=C stretching-Ph). 1H NMR (δ ppm): 7.10–7.51 (m, 8H, aromatic protons); 4.40 (d, 2H, J = 2.20 Hz, H-2a and H-4a); 2.83 (m, 1H, H-7a), 2.44 (br s, 2H, H-1 and H-5), 1.92 (d, 1H, J = 5.48 Hz, H-8e), 1.88 (d, 2H, J = 5.48 Hz, H-6e and NH); 1.76–1.67 (m, 2H, H-6a and H-8a); 1.41 (quin, 1H, H-7e). 13C NMR (δ ppm): 216.90 (C-9); 163.46, 161.01 (C-2″″ and C-4″″), 136.94, 136.92 (C-2′ and C-4′), 128.49, 128.41 (C-2″ and C-4″), 115.61, 115.40 (C-2′″ and C-4′″); 64.17 (C-2, C-4); 53.95 (C-1, C-5); 28.97 (C-6, C-8); 21.15 (C-7). GCMS m/z = 328.17 (M+1).
2,4-Bis(3-fluorophenyl)-3-azabicyclo[3.3.1]nonan-9-one (compound 3)
IR (cm−1): 3315 (N–H stretching); 3073, 2978, 2930, 2910, 2852, 2815 (C–H stretching); 1712 (C=O stretching); 1614, 1589 (C=C stretching-Ph). 1H NMR (δ ppm): 7.40–7.02 (m, 8H, aryl protons); 4.42 (d, 2H, J = 1.60 Hz, H-2a and H-4a); 2.80 (m, 1H, H-7a); 2.49 (br s, 2H, H-1, H-5); 1.94 (d, 2H, J = 4.80 Hz, H-8e, NH); 1.90 (d, 1H, J = 5.20 Hz, H-6e); 1.78–1.68 (m, 2H, H-6a and H-8a), 1.42 (quin, 1H, H-7e). 13C NMR (δ ppm): 216.48 (C-9); 164.40, 161.95 (fluoro bearing C-2′″, C-4′″); 143.88, 143.82 (C-2′, C-4′), 130.31, 130.23 (C-2′″, C-4′″); 122.58, 122.56 (C-2″, C-4″), 114.75, 114.54, 114.04, 113.82 (C-4″″); 64.19 (C-2 and C-4); 53.78 (C-1 and C-5); 29.14 (C-6 and C-8); 21.17 (C-7). GCMS m/z = 327.42 (M+).
2,4-Bis(4-chlorophenyl)-3-azabicyclo[3.3.1]nonan-9-one (compound 4)
IR (cm−1): 3314 (N–H stretching); 3063, 2921, 2852, 2800 (C–H stretching); 1709 (C=O stretching); 1590 (C=C stretching-Ph). 1H NMR (δ ppm): 7.48–7.38 (m, 8H, aromatic protons); 4.39 (d, 2H, J = 2.20 Hz, H-2a and H-4a); 2.80 (m, 1H, H-7a); 2.44 (br s, 2H, H-1 and H-5); 1.90 (d, 1H, J = 4.76 Hz, H-8e); 1.87 (d, 2H, J = 4.76 Hz, H-6e and NH); 1.76–1.66 (m, 2H, H-6a and H-8a); 1.40 (quin, 1H, H-7e). 13C NMR (δ ppm): 216.64 (C-9); 139.63 (C-2′, C-4′); 133.43 (C-2″″ and C-4″″); 128.88 (C-2″ and C-4″); 128.28 (C-2′″ C-4′″); 64.17 (C-2, C-4); 53.77 (C-1, C-5); 29.00 (C-6, C-8); 21.14 (C-7). GCMS m/z = 359.13 (M+); m/z = 361 (M+2 isotopic peak).
2,4-Bis(2-chlorophenyl)-3-azabicyclo[3.3.1]nonan-9-one (compound 5)
IR (cm−1): 3305 (N–H stretching); 3063, 2973, 2931, 2905, 2852, 2821 (C–H stretching); 1706 (C=O stretching); 1589, 1567 (C=C stretching-Ph. 1H NMR (δ ppm): 4.85 (d, 2H, J = 2.40 Hz, H-2a, H-4a); 2.88 (m, 1H, H-7a); 2.77 (br s, 2H, H-1, H-5); 1.90 (d, 1H, J = 4.80 Hz, H-8e); 1.87 (dd, 1H, J = 4.80, 1.60 Hz, H-6e); 1.81–1.71 (m, 2H, H-6a, H-8a); 1.66 (br s, 1H, NH); 1.41 (quin, 1H, H-7e); 8.05–7.27 (m, 8H, aromatic protons). 13C NMR (δ ppm): 216.34 (C-9); 138.17 (C-2′ and C-4′), 132.69 (chloro bearing C-2″ and C-4″); 130.17 (C-2″ and C-4″); 128.78 (C-2″″ and C-4″″); 128.90, 126.92 (other aromatic carbons); 61.01 (C-2 and C-4); 49.67 (C-1 and C-5); 29.65 (C-6 and C-8); 20.93 (C-7).
2,4-Bis(4-methylphenyl)-3-azabicyclo[3.3.1]nonan-9-one (compound 6)
IR (cm−1): 3315 (N–H stretching); 3024, 2939, 2859 (C–H stretching); 1714 (C=O stretching); 1599, 1564 (C=C stretching-Ph). 1H NMR (δ ppm): 7.43–7.21 (m, 8H, aromatic protons); 4.37 (d, J = 2.20 Hz, H-2a, H-4a); 2.89 (m, H-7a), 2.44 (br s, H-1, H-5); 2.36 (s, CH3 at C-2″″ and C-4″″); 1.95 (dd, J = 5.86, 1.46 Hz, H-8e); 1.92 (dd, J = 5.32, 1.64 Hz, H-6e, NH); 1.75–1.65 (m, H-6a, H-8a); 1.37 (quin, H-7e). 13C NMR (δ ppm): 217.99 (C-9); 138.46 (C-2′ and C-4′); 137.22 (C-2″″ and C-4″″); 129.29, 126.90 (other aromatic carbons); 64.71 (C-2, C-4); 54.21 (C-1, C-5); 29.18 (C-6, C-8); 21.27 (C-7); 21.22 (CH3 at C-2″″ and C-4″″). GCMS m/z = 320.21(M+1).
2,4-Bis(4-methoxyphenyl)-3-azabicyclo[3.3.1]nonan-9-one (compound 7)
IR (cm−1): 3307 (N–H stretching); 3005, 2968, 2933, 2831 (C–H stretching); 1705 (C=O stretching); 1610, 1584 (C=C stretching-Ph). 1H NMR (δ ppm): 7.46–6.93 (m, 8H, aromatic protons); 4.34 (d, 2H, J = 2.56 Hz, H-2a and H-4a); 3.28 (s, 6H, OCH3 at C-2″″ and C-4″″); 2.88 (m, 1H, H-7a); 2.41 (br s, 2H, H-1 and H-5); 1.96 (d, 1H, J = 4.40 Hz, H-8e); 1.92 (d, 1H, J = 4.76 Hz, H-6e); 1.87 (br s, 1H, NH); 1.74–1.64 (m, 2H, H-6a, H-8a); 1.38 (quin, 2H, H-7e). 13C NMR (δ ppm): 217.88 (C-9); 158.98 (C-2″″ and C-4″″), 136.51(C-2′ and C-4′), 132.27(C-2″ and C-4″), 113.89 (C-2′″ and C-4′″); 64.36 (C-2 and C-4); 55.29 (OCH3 at C-2″″ and C-4″″); 54.24 (C-1 and C-5); 29.05 (C-6 and C-8); 21.22 (C-7). GCMS m/z = 351.38 (M+).
2,4-Bis(3-methoxyphenyl)-3-azabicyclo[3.3.1]nonan-9-one (compound 8)
IR (cm−1): 3310 (N–H stretching); 3073, 2933, 2930, 2826 (C–H stretching); 1708 (C=O stretching); 1609, 1583 (C=C stretching-Ph). 1H NMR (δ ppm): 7.31–6.84 (m, 8H, aromatic protons); 4.37 (d, J = 2.20 Hz, H-2a, H-4a), 3.84 (s, 6H, OCH3 at C-2′″ and C-4′″); 2.86 (m, H-7a); 2.48 (br s, H-1, H-5); 1.97 (dd, J = 5.68, 1.48 Hz, H-8e); 1.93 (dd, J = 4.76, 1.48 Hz, H-6e); 1.90 (s, NH), 1.75–1.65 (m, H-6a, H-8a); 1.38 (quin, H-7e). 13C NMR (δ ppm): 217.55 (C-9); 159.83 (methoxy bearing C-2′″ and C-4′″); 143.03 (C-2′ and C-4′); 129.61(C-2′″ and C-4′″), 119.27, 112.97(C-2″ and C-4″), 114.6 (C-2″″ and C-4″″); 64.65 (C-2 and C-4); 55.29 (OCH3 at C-2′″ and C-4′″); 54.03 (C-1 and C-5); 29.23 (C-6 and C-8); 21.18 (C-7).
General method for synthesis of N′-(2,4-diaryl-3-azabicyclo[3.3.1]nonan-9-ylidine)bezenecarbothiohydrazides (compounds 9–16)
A mixture of 2,4-diaryl-3-azabicyclo[3.3.1]nonan-9-one (0.01 mol) and 4′-phenylthiosemicarbazide (0.01 mol) in ethanolic chloroform (1:1 mixture, 50 mL) was refluxed for 4 h in acidic medium. After cooling, the solid product was filtered and washed with excess of water and recrystallized from methanol. The recrystallized product further purified by column chromatography n-hexane:ethyl acetate (4:1) as eluent to get pure thiosemicarbazones.
N′-(2,4-diphenyl-3-azabicyclo[3.3.1]nonan-9-ylidine)bezenecarbothiohydrazides (compound 9)
IR (cm−1): 3352, 3282, 3258 (N–H stretching); 3058, 3039, 2964, 2912 (C–H stretching); 1616 (C=N stretching). 1H NMR (δ ppm): 9.21 (s, 1H, NHCS); 8.43 (s, 1H, CSNHPh); 7.61–7.23 (m, 15H, aryl protons); 4.41 (d, J = 2.0 Hz, 1H, H-2a); 4.29 (d, J= 2.0 Hz, 1H, H-4a); 3.11 (s, 1H, H-5); 2.83 (m, 1H, H-7a); 2.48 (s, 1H, H-1); 1.79 (dd, 3H, J = 5.88, 1.48 Hz, H-6e, H-8e and NH); 1.46 (m, 3H, H-6a, H-8a and H-7e). 13C NMR (δ ppm): 172.19 (C=S); 169.68 (C=N); 142.67 (C-2′); 142.39 (C-4′); 138.46 (C-4a); 128.42 (C-4b), 128.39 (C-4c), 127.61, 127.51 (C-2″ and C-4″); 127.31, 127.14 (C-2″″ and C-6″″); 126.89 (C-2′″ and C-6′″); 123.67 (C-4d); 65.01 (C-2); 63.98 (C-4); 45.01 (C-1); 36.34 (C-5); 29.08 (C-8); 25.98 (C-6); 20.51 (C-7). HRMS (ES): m/z = 441.1119 (M+H).
N′-(2,4-Bis(4-fluorophenyl)-3-azabicyclo[3.3.1]nonan-9-ylidine)bezenecarbothiohydrazides (compound 10)
IR (cm−1): 3352, 3261, 3158 (N–H stretching); 3059, 2960, 2936, 2892, 2856, 2798 (C–H stretching); 1638 (C=N stretching). 1H NMR (δ ppm): 9.21 (s, 1H, NHCS); 8.43 (s, 1H, NHPh); 7.27–7.56 (m, 13 H, aryl protons); 7.11 (q, 4H, protons meta to fluorine atom); 4.36 (d, J = 2.5 Hz, 1H, H-2a); 4.22 (d, J = 1.88 Hz 1H, H-4a); 2.96 (s, 1H, H-5); 2.51 (s, 1H, H-1); 2.80 (m, 1H, H-7a); 1.81 (m, 3H, H-6e, H-8e and NH); 1.36–1.55 (m, 3H, H-6a and H-8a and H-7e). 13C NMR (δ ppm): 176.12 (C=S); 167.05 (C=N); 163.89, 161.65 (C-2″″ and C-4″″); 138.07, 136.28 (C-2′ and C-4′); 139.06 (C-4a); 128.62 (C-4b), 128.38 (C-4c), 128.24, 127.88 (C-2″ and C-6″), 127.67 (C-4d), 115.61, 115.11 (C-2′″ and C-6′″); 64.88 (C-2); 63.44 (C-4); 46.56 (C-1); 35.12 (C-5); 29.91 (C-8); 26.87 (C-6); 25.42 (C-7). HRMS (ES): m/z = 477.2324 (M+H).
N′-(2,4-Bis(3-fluorophenyl)-3-azabicyclo[3.3.1]nonan-9-ylidine)bezenecarbothiohydrazides (compound 11)
IR (cm−1): 3397, 3251, 3142 (N–H stretching); 3068, 2989, 2946, 2866 (C–H stretching); 1639 (C=N stretching). 1H NMR (δ ppm): 9.21 (s, 1H, NHCS); 8.62 (s, 1H, CSNHPh); 6.96–7.44 (m, 13H, protons ortho and meta to the fluorine atom); 4.43 (d, J = 2.5 Hz, 1H, H-2a); 4.24 (d, J = 2.5 Hz, 1H, H-4a); 2.94 (s, 1H, H-5); 2.72 (m, 1H, H-7a); 2.54 (s, 1H, H-1); 1.80 (b s, 3H, H-6e, H-8e and NH); 1.39–1.57 (m, 2H, H-6a and H-8a); 1.44 (quintet, 1H, H-7e). 13C NMR (δ ppm): 169.98 (C=S); 161.79 (C=N); 164.35, 161.56 (C-2′″ and C-4′″); 144.13 (C-2′); 143.76 (C-4′); 138.27 (C-4a); 130.96, 122.76, 122.41, 121.76, 114.74, 114.63, 114.20, 113.93, 113.69 (other aryl carbons); 64.16 (C-2); 63.22 (C-4); 46.24 (C-1); 39.15 (C-5); 28.67 (C-8); 27.57 (C-6); 21.41 (C-7).
N′-(2,4-Bis(4-chlorophenyl)-3-azabicyclo[3.3.1]nonan-9-ylidine)bezenecarbothiohydrazides (compound 12)
IR (cm−1): 3379, 3245 (N–H stretching); 3056, 2997, 2948, 2892, 2735 (C–H stretching); 1641 (C=N stretching). 1H NMR (δ ppm): 9.91(s, 1H, NHCS); 8.91 (s, 1H, CSNHPh); 7.01–7.50 (13H, aromatic protons); 4.39 (d, J =2.5 Hz, 1H, H-2a); 4.21 (d, J = 2.0 Hz, 1H, H-4a); 2.85 (s, 1H, H-5); 2.49 (s, 1H, H-1); 2.78 (m, 1H, H-7a); 1.84 (dd, 3H, H-6e, H-8e and NH); 1.40–155 (m, 2H, H-6a and H-8a); 1.45 (quintet, 1H, H-7e). 13CNMR (δ ppm): 169.46 (C=S); 159.99 (C=N); 140.28 (C-2′); 138.27 (C-4′); 138.96 (C-4a); 133.68, 133.35 (C-2″″ and C-4″″) 128.93 (C-4b); 128.75 (C-4c); 128.43, 128.19 (C-2″ and C-6″) 127.96 (C-4d); 126.23 (C-2′″ and C-6′″); 64.87 (C-2); 63.42 (C-4); 46.41 (C-1); 39.13 (C-5); 28.53 (C-8); 27.32 (C-6); 21.34 (C-7). HRMS (ES): m/z = 509.1408 (M+H); m/z = 510.1392 (M+2).
N′-(2,4-Bis(2-chlorophenyl)-3-azabicyclo[3.3.1]nonan-9-ylidine)bezenecarbothiohydrazides (compound 13)
IR (cm−1): 3386, 3247, 3143 (N–H stretching); 3042, 2976, 2967, 2946, 2883 (C–H stretching); 1629 (C=N stretching). 1H NMR (δ ppm): 9.87 (s, 1H, NHCS); 8.29 (s, 1H, CSNHPh); 8.01 (t, 2H, protons ortho to chlorine atom); 7.11–7.42 (m, 11H, other aryl protons); 4.72 (d, J = 2.5 Hz, 1H, H-2a); 4.62(d, J = 2.0 Hz 1H, H-4a); 3.18 (d, J = 2.0 Hz, 1H, H-5); 2.79 (m, 2H, H-1 and H-7a); 1.79 (dd, 3H, H-6e, H-8e and NH); 1.61 (m, 2H, H-6a and H-8a); 1.39 (quintet, 1H, H-7e). 13C NMR (δ ppm): 169.34 (C=S); 159.15 (C=N); 138.65 (C-2′); 138.03 (C-4′); 139.01 (C-4a); 133.01, 130.10 (chloro bearing C-2″ and C-6″); 128.95, 128.56, 127.43, 126.19, 126.59, 125.01, 121.43 (other aryl carbons); 62.43 (C-2); 60.58 (C-4); 42.19 (C-1); 35.03 (C-5); 29.18 (C-8); 27.20 (C-6); 21.32 (C-7).
2,4-Bis(4-methylphenyl)-3-azabicyclo[3.3.1]nonan-9-one 4′-phenylthiosemicarbazones (compound 14)
IR (cm−1): 3392, 3256, 3141 (N–H stretching); 3068, 2992, 2972, 2932, 2888, 2797 (C–H stretching); 1642 (C=N stretching). 1H NMR (δ ppm): 9.90 (s, 1H, NHCS); 8.47 (s, 1H, CSNHPh); 7.01–7.44 (m, 13H, aryl protons); 4.38 (d, J = 2.5 Hz, 1H, H-2a); 4.23 (d, J = 2.0 Hz, 1H, H-4a); 2.91 (s, 1H, H-5); 2.47 (s, 1H, H-1); 2.79 (m, 1H, H-7a); 2.31, (s, 6H, CH3 at C-2″″ and C-4″″); 1.86 (dd, 3H, H-6e, H-8e and NH); 1.38–1.52 (m, 2H, H-6a and H-8a), 1.41 (quintet, 1H, H-7e). 13C NMR (δ ppm): 168.42 (C=S); 157.73 (C=N); 138.10, 137.23 (C-2′ and C-4′); 139.06 (C-4a); 136.90 (C-2″″ and C-4″″), 129.38 (C-4b), 129.05 (C-4c), 127.19 (C-2″ and C-6″), 126.76(C-2′″ and C-6′″), 125.12 (C-4d) (other aryl carbons); 65.43 (C-2); 63.91 (C-4); 46.57 (C-1); 39.32 (C-5); 29.47 (C-8); 28.14 (C-6); 21.29 (C-7); 21.09 (CH3 at C-2″″ and C-4″″); HRMS (ES): m/z = 469.1983 (M+H).
N′-(2,4-Bis(4-methoxyphenyl)-3-azabicyclo[3.3.1]nonan-9-ylidine)bezenecarbothiohydrazides (compound 15)
IR (cm−1): 3335, 3238, 3186 (N–H stretching); 3012, 2998, 2947, 3286, 2849 (C–H stretching); 1629 (C=N stretching). 1H NMR (δ ppm): 9.86 (s, 1H, NHCS); 8.88 (s, 1H, CSNHPh); 6.93–7.47 (m, 13H, aryl protons); 4.31 (d, J = 2.5 Hz, 1H, H-2a); 4.21 (s, 1H, H-4a); 3.82 (d, 6H, OCH3 at C-2″″ and C-4″″); 2.81 (s, 1H, H-5); 2.78 (m, 1H, H-7a); 2.45 (s, 1H, H-1); 1.90 (dd, 3H, H-6e, H-8e and NH); 1.45 (m, 3H, H-6a and H-8a and H-7e). 13C NMR (δ ppm): 167.19 (C=S); 158.56, 159.01 (C-2″″ and C-4″″); 156.32 (C=N); 138.06 (C-4a); 134.12, 133.31 (C-4′ and C-2′); 129.91(C-4b); 127.81(C-4c); 127.56 (C-2″ and C-6″); 126.11 (C-4d); 113.92, 113.80 (C-2′″ and C-6′″); 65.01(C-2); 63.36 (C-4); 55.01 (OCH3 at C-2″ and C-4″″); 46.43 (C-1); 39.12 (C-5); 29.06 (C-8); 27.31 (C-6); 22.13 (C-7). HRMS (ES): m/z = 501.2298 (M+H).
2,4-Bis(3-methoxyphenyl)-3-azabicyclo[3.3.1]nonan-9-one 4′-phenylthiosemicarbazones (compound 16)
IR (cm−1): 3359, 3278, 3143 (N–H stretching); 3043, 3021, 2968, 2943, 2879, 2874, 2768 (C–H stretching); 1612 (C=N stretching). 1H NMR (δ ppm): 9.81 (s, 1H, NHCS); 8.83 (s, 1H, CSNHPh); 6.81–7.43; (m, 13H, aryl protons). 4.36 (d, 1H, H-2a); 4.23 (d, 1H, H-4a); 3.78 (s, 6H, OCH3 at C-2′″ and C-4′″); 2.83 (m, 1H, H-7a); 2.94 (s, 1H, H-5); 2.49 (s, 1H, H-1); 1.82 (dd, 3H, H-6e, H-8e and NH); 1.43–1.54 (m, 2H, H-6a, H-8a and H-7e). 13C NMR (δ ppm): 168.14 (C=S); 159.13, 159.07 (C-2′″ and C-4′″); 156.09 (C=N); 143.32, 142.98 (C-2′ and C-4′); 138.90 (C-4a); 129.48 (C-4b), 129.05 (C-4c), 126.25 (C-4d), 125.97, 119.23, 119.06, 113.34, 112.98 (other aryl carbons); 65.09 (C-2); 63.41 (C-4); 55.21 (OCH3 at C-2′″ and C-4′″); 46.39 (C-1); 39.35 (C-5); 28.76 (C-8); 27.47 (C-6); 21.25 (C-7); HRMS (ES): m/z = 500.9981 (M+H).
Results and discussion
Chemistry
The parent bicyclic ketones (2,4-diaryl-3-azabicyclo [3.3.1]nonan-9-ones) were prepared according to the literature precedent by Baliah and Jeyaraman [Citation27]. The ketones upon treatment with 4′-phenylthiosemicarbazide in the presence of few drops of mineral acid afforded two different products, viz., 2,4-Diaryl-3-azabicyclo[3.3.1]nonan-9-one thiosemicarbazones (9–16) and arylthiosemicarbazones (9a–16a) (). The formation of by-product (arylthiosemicarbazones) is due to poor mass balance while using mineral acids (HCl or H2SO4) as catalyst in the condensation of high molecular parent ketones with thiosemicarbazide. The cleavages of the products were studied by retro mannich mechanism followed by condensation reaction. Further, no more by-products were found to be formed after workup. The analytical data of the compounds 1–16 are given in .
Table 1. Analytical data for compounds 1–16.
Scheme 1. Reagent and conditions: (a) EtOH/warm, room temperature, 2–10 days and (b) MeOH-CHCl3, H2NNHCSNHPh/H+, reflux, 90–100°C 4 h.
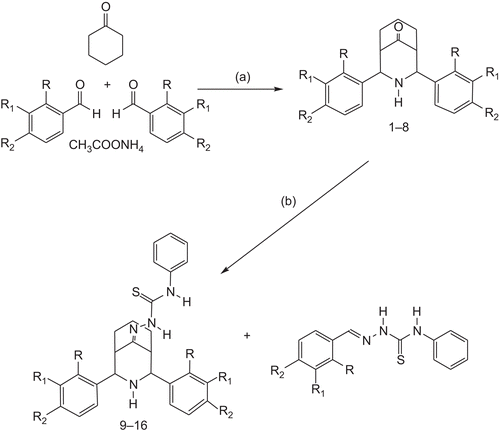
In order to investigate the spectral assignments of reported compounds 9–16, compound 9 is taken as the representative compound. The IR spectra of compound 9 show a collective absorption bands appeared in the region 3352–3258 cm−1, which is assigned to NH stretching frequency, and C=N stretching frequency appeared as strong and intense bands at 1616 cm−1, respectively.
For the representative compound 9, H,H-COSY, NOESY, and HSQC were recorded to assign all the signals unambiguously, and to establish the stereochemistry. The H,HCOSY/NOESY and HSQC correlations are reproduced in and .
Table 2. Correlations in the H,H-COSY and NOESY spectra of compound 9.
Table 3. Correlations in the HSQC spectrum of compound 9.
Assignment of proton chemical shifts and stereochemistry
In compound 9, two broad singlets at 8.43 ppm (1H) and 9.21 ppm (1H) are due to NH protons of thiosemicarbazone moiety, which are disappeared in the D2O exchange NMR spectrum. Of the two singlets, the upfield signal at 8.43 has NOE with the proton signal at 3.11 ppm (H-5); hence, the broad singlet at 8.43 assigned to NH proton at 2′ of thiosemicarbazone moiety. Consequently, the broad singlet at 9.21 ppm unequivocally assigned to the NH proton at 4′ of thiosemicarbazone moiety. According to the D2O exchange NMR, the multiplet centred at 1.79 ppm corresponding to three protons is reduced to two protons, because of D2O exchange, which indicates that the NH proton resonance also overlapped the multiplet.
There are two broad singlets at 3.11 (1H) and 2.48 (1H) ppm with the half-width W1/2 of 11.40 and 11.38 Hz, respectively. The broad singlet nature and half-width values suggest that they are due to the bridgehead protons H-1 and H-5. Further, the observed weak correlation between 3.11 and 2.48 ppm strongly implies that the correlation is due to long-range coupling between the bridgehead protons because of the “W” arrangement of those protons ().
Figure 1. Correlations between the protons that are in “W” arrangements, from the H,H-COSY spectrum of compound 9.
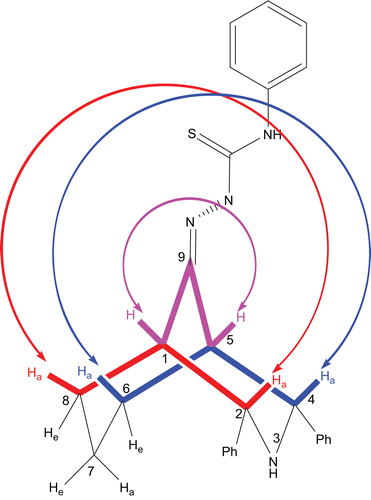
The difference between H-1 and H-5, that is, Δδ, is 0.63 ppm whereas in the corresponding bicyclic ketone, the bridgehead protons H-1 and H-5 appear together at 2.47 ppm (2H) as a broad singlet with a W1/2 of 11.46 Hz. Because of nonbonding interaction between the N–N(2′) and C(5)-H bonds (), the H-5 proton is deshielded, the deshielding magnitude is quite large (0.64 ppm). But there is no change in another bridgehead proton H-1, except for slight deshielding of 0.01 ppm. The positions, appearance, spectral width, and correlations strongly suggest that the signals at 3.11 and 2.48 ppm are due to the bridgehead protons H-5 (syn α) and H-1 (anti α). Though the bridgehead protons appear as two separate signals, both are broad singlets and their half-widths are also similar to that of its parent ketone 1. Hence, this suggests that the thiosemicarbazone derivative also adopts the twin-chair conformation.
The signals at 4.41 (d, J = 2.0 Hz) and 4.33 ppm (d, J = 2.0 Hz) are due to benzylic protons and they only have correlations with the bridgehead protons H-1 and H-5; hence, we can unambiguously assign the signals to H-2 and H-4. Further, they have close proximity (NOE) with each other, hence indicating their orientation as axial. Except for axial orientation of the protons at C-2 and C-4, there is otherwise no possibility of NOE between them in the chair conformation. As a consequence of the observed NOE, we can conclude that the orientation of the phenyl groups on the same carbons should be equatorial. Moreover, 1,3-diequatorial orientation of the bulkier substituents is more favourable in the chair conformation. Further, the observed strong NOE of the ortho protons with H-7a/H-8e/H-6e also supports the equatorial orientation of the phenyl groups and the twin-chair conformation.
In addition to the “W” correlation, both H-1 and H-5 have correlations with the doublet at 1.79 ppm (3H, due to overlapped NH) and multiplet at 1.46 (3H) ppm. It is usually observed that the chemical shift difference of protons in the cyclohexane chair conformation is positive (Δδeq,ax = δeq − δax, is ′0.3 ppm), owing to the leading hyperconjugation of the C–C single bond [Citation28]. The multiplets with average difference of 0.33 ppm suggest that they are due to C-6 and C-8 protons. Further, the assignments and chair conformation of the cyclohexane part are confirmed by their NOE (). Moreover, the observed NOE between the multiplet at 2.83 ppm and the aryl protons supports attribution of the multiplet to H-7a (endocyclic proton, which is spatially very close to the ortho protons).
Figure 3. Twin-chair conformation with equatorial orientation of the phenyl groups at C-2 and C-4; supported by the NOE observed in the NOESY spectrum of compound 9. For clarity, the NOE between the ortho and ring protons is shown by red colour lines.
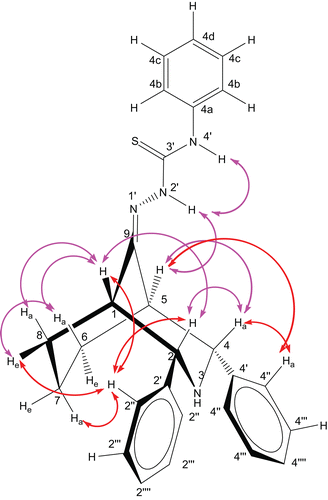
Of the five set of aromatic signals, the double doublet centred at 7.46 ppm (2H) coupled with the doublet at 7.59 (2H) and triplet at 7.21 ppm (1H). This observation suggests that the signals at 7.59, 7.46, and 7.21 ppm are due to the phenyl protons of thiosemicarbazone moiety 4b, 4c, and 4d, respectively. The remaining two sets of aryl signals, a multiplet centred at 7.36 (4H) and at 7.27(6H) ppm, and the multiplet at 7.36 ppm, have NOE with H-1/H-2a/H-7a/H-8e of the protons. Hence, the multiplet at 7.36 ppm is assigned to ortho protons (H-2″/H-4″). Consequently, the multiplet at 7.27 ppm is due to H-2′″/H-4′″, H-2″″/H-4″″ protons.
Overall, the chemical shifts, splitting pattern, H,H correlation, and NOE suggest that the compound is in twin-chair conformation with equatorial orientation of the phenyl groups, as depicted in . Further, the twin-chair conformation is evidenced by the W1/2 of H-1 and H-5; these values are in good agreement with the corresponding ketone and with literature values for the flattened twin-chair conformation of similar bicycles. If one of the cycles adopts a boat conformation, the resonances for the bridgehead protons H-1 and H-5 should be apparent doublets with coupling constants of about 18 Hz [Citation29]. In addition, the observed long-range couplings between H-2a and H-8a and between H-4a and H-6a are due to the “W” arrangement of those protons and this is only possible when the bicycle is in the twin-chair conformation (). Similarly, 1H NMR signals of other bicyclic thiosemicarbazones 10–16 are assigned and summarized in the “Experimental” section.
Assignment of carbon chemical shifts
All the proton-bonded carbon signals of the representative compound 9 were unambiguously assigned using HSQC spectrum. In the downfield region, five less intense signals that appear at 169.68, 172.19, 142.67, 142.39, and 138.46 ppm have no correlation with any proton signals of the molecule, which indicates that these signals are due to quaternary carbons. Of the five signals, 138.46, 142.67, and 142.39 ppm are due to the 4′-phenyl, C-2′, and C-4′ ipso carbons, respectively, and the signals at 169.68 and 172.19 ppm are due to the resonance of C=N and C=S carbons, respectively. The HSQC correlations are reproduced in .
Antimicrobial evaluation
In order to find the effect of potency of inhibitions in compounds 9–16 by in vitro method, we modified different substituents at phenyl groups in 2,4-diaryl-3-azabicyclo[3.3.1]nonan-9-one 4′-phenylthiosemicarbazones. The reported compounds were tested against bacterial strains, viz., S. aureus (ATCC-25825), B. subtilis (ATCC-451), S. typhi (ATCC-24915), E. coli (ATCC-25835), and K. pneumonia (ATCC-15490) using the literature precedent by Dhar et al., [Citation26], and their MIC values are depicted in .
Table 4. Antibacterial activity of compounds 9–16 against selected bacterial strains (MIC in μg/mL).
A glance at the MIC values in indicates that compound 9 against S. aureus exhibit minimum inhibition activity. However, para or meta fluorophenyl-substituted compound 10 against B. subtilis and S. typhi and compound 11 against B. subtilis exerted significant inhibition. The compound 10, which was inactive against K. pneumonia even at maximum concentration (200 μg/mL).
Surprisingly, replacement of fluorine function by chlorine in compounds 10 and 11 (compounds 12 and 13, respectively), compound 12 against K. pneumonia, was found to show superior activity than others. However, compound 12 against S. aureus/E. coli and B. subtilis shows a reversal in activity, by 8- and 4-fold, respectively, due to replacement of fluorine by chlorine. Instead of halogens, methyl or methoxy function substituted compound 14 against E. coli and compound 15 against K. pneumonia markedly elevated the maximum inhibition potency at minimum concentration (12.5 μg/mL).
The in vitro antifungal activity of the reported compounds 9–16 were examined with five fungal strains, viz., C. albicans (ATCC-3122), R. oryzae (ATCC-9363), C. neoformans (ATCC-3312), A. niger (ATCC-598), and A. flavus (ATCC-485) and amphotericin B was used as standard drug. The obtained MIC values of the tested compounds and standard are depicted in that indicates all the tested compounds exhibit a varied range 6.25–200 μg/mL. Unsubstituted phenyl groups in compound 9 recorded minimum to moderate activity (100–200 μg/mL) against all the tested organisms except A. flavus, which did not show any inhibition potency even at maximum concentration at 200 μg/mL. However, due to introduction of fluorine atom at the meta or para position of phenyl groups in compound 9 (compounds 10 and 11) noticed minimum to moderate inhibition potency against all the tested fungal organisms with MIC ranging from 6.25 to 100 μg/mL, in which, compound 11 against A. albicans shows superior inhibition potency at minimum concentration (6.25 μg/mL). Modification of fluorine substituent by chlorine in compound 10 (compound 12) registered minimum antifungal activity against all the used strains. By changing the position of chloro substitution in compound 12 para to ortho (compound 12) 16- and 2-fold increased activity were noticed against C. albicans and R. oryzae. Replacement of one proton by methyl analogue in compound 9 (compound 14), the activity was increased against all the strains except against A. flavus, which is inactive even at maximum concentration (200 μg/mL). Due to modification of methyl analogue by methoxy group in compound 14, compounds 15 and 16 show moderate activity against the entire tested fungal strains. Among the compounds under the antifungal study, compounds 9 and 14 against A. flavus seldom show inhibition even at maximum concentration (200 μg/mL).
Table 5. Antifungal activity of compounds 9–16 against selected fungal strains (MIC in μg/mL).
From the close survey of the in vitro antibacterial and antifungal results against a panel of microbial organisms, it was revealed that the electron-withdrawing substituents at the ortho/meta/para position of the phenyl groups at C-2 and C-4 of the 3-azabicyclononane pharmacophore had remarkable activity against all the tested organisms.
A comparison of potency of the compounds 9–16 is given in the form of and by employing the following the equation.
Figure 4. Comparison of potency of compounds 9–16 with ciprofloxacin (as standard) against bacterial strains from serial dilution method. Scheme 1: Schematic diagram showing the synthesis of 2,4-diaryl-3-azabicyclo[3.3.1]nonan-9-one 4′-phenylthiosemicarbazones.
![Figure 4. Comparison of potency of compounds 9–16 with ciprofloxacin (as standard) against bacterial strains from serial dilution method. Scheme 1: Schematic diagram showing the synthesis of 2,4-diaryl-3-azabicyclo[3.3.1]nonan-9-one 4′-phenylthiosemicarbazones.](/cms/asset/4599d44a-f215-47e7-8db6-4af13cac0b1c/ienz_a_525508_f0005_b.gif)
Figure 5. Comparison of potency of compounds 9–16 with amphotericin B (as standard) against fungal strains from serial dilution method.
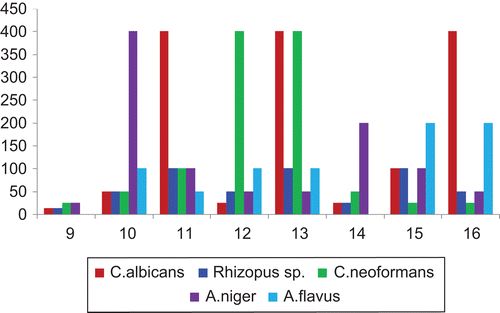
A close survey of the in vitro antibacterial and antifungal activity profile of the new 2,4-diaryl-3-azabicyclo[3.3.1]nonan-9-one 4′-phenylthiosemicarbazones (9–16) against the tested bacterial and fungal organisms gives a clear picture about the structure–activity correlations among compounds 9–16 under study. Compounds with electron-withdrawing groups (fluoro and chloro) and electron-donating groups (methyl or methoxy) function at the aryl groups present at the C-2 and C-4 positions of the azabicyclo[3.3.1]nonan-9-one moiety exerted a varied range of biological activities, while the activity was not significant for compound 9 without any substituent at the phenyl groups.
Among compounds 10–16, compounds 10 and 11 against B. subtilis, compound 13 against S. typhi, compound 14 against E. coli, compounds 12 and 15 against K. pneumonia were shown to be significant in their antibacterial potency at 6.25 and 12.5 µg/mL. Furthermore, their activity was also on a par with the standard drugs used and for some compounds was even higher than the activity of the standard drugs. Though most of the compounds studied exhibited moderate to significant antibacterial activity, compound 10 (with o-chlorophenyl group at the C-2 and C-4 positions) was found to exert a pronounced effect against all the tested organisms.
Similarly, against the tested fungal strains, compounds 11, 13, and 16 against C. albicans, compounds 11–13 against C. neoformans, compound 10 against A. niger recorded enhanced activity at 6.25 and 12.5 µg/ mL. However, the activity of compound 11 against all the tested organisms was found to be superior to the other compounds and with an even higher activity than the standard.
Conclusions
A close examination of the in vitro antibacterial and antifungal activity profile of the differently substituted novel 2,4-diaryl-3-azabicyclo[3.3.1]nonan-9-one 4′-phenylthiosemicarbazones (9–16) against the tested bacterial and the fungal strains provides a structure–activity relationship, which reveals that the compounds with fluorine or chlorine substituents were found to be more active against all the tested organisms. The method of action of these compounds is unknown. These observations may promote a further development of this group of 2,4-diaryl-3-azabicyclo[3.3.1]nonan-9-one 4′-phenylthiosemicarbazones (9–16), which may lead to compounds with better a pharmacological profile than standard drugs and serve as templates for the construction of better drugs to fight bacterial and fungal infections.
Declaration of interest
Authors have no declaration of interest.
References
- Zanatta, N., Alves, S.H., Coelho, H.S., Borchhardt, D.M., Machado, P., Flores, K.M., da Silva, F.M., Spader, T.B., Santurio, J.M., Bonacorso, H.G., Martins, M.A. Synthesis, antimicrobial activity, and QSAR studies of furan-3-carboxamides. Bioorg. Med. Chem. 2007, 15, 1947–1958.
- Khalafi-Nezhad, A., Soltani Rad, M.N., Mohabatkar, H., Asrari, Z., Hemmateenejad, B. Design, synthesis, antibacterial and QSAR studies of benzimidazole and imidazole chloroaryloxyalkyl derivatives. Bioorg. Med. Chem. 2005, 13, 1931–1938.
- Ghosh, S., Misra, A.K., Bhatia, G., Khan, M.M., Khanna, A.K. Syntheses and evaluation of glucosyl aryl thiosemicarbazide and glucosyl thiosemicarbazone derivatives as antioxidant and anti-dyslipidemic agents. Bioorg. Med. Chem. Lett. 2009, 19, 386–389.
- Siles, R., Chen, S.E., Zhou, M., Pinney, K.G., Trawick, M.L. Design, synthesis, and biochemical evaluation of novel cruzain inhibitors with potential application in the treatment of Chagas’ disease. Bioorg. Med. Chem. Lett. 2006, 16, 4405–4409.
- Fujii, N., Mallari, J.P., Hansell, E.J., Mackey, Z., Doyle, P., Zhou, Y.M., Gut, J., Rosenthal, P.J., McKerrow, J.H., Guy, R.K. Discovery of potent thiosemicarbazone inhibitors of rhodesain and cruzain. Bioorg. Med. Chem. Lett. 2005, 15, 121–123.
- Greenbaum, D.C., Mackey, Z., Hansell, E., Doyle, P., Gut, J., Caffrey, C.R., Lehrman, J., Rosenthal, P.J., McKerrow, J.H., Chibale, K. Synthesis and structure–activity relationships of parasiticidal thiosemicarbazone cysteine protease inhibitors against Plasmodium falciparum, Trypanosoma brucei, and Trypanosoma cruzi. J. Med. Chem. 2004, 47, 3212–3219.
- Du, X., Guo, C., Hansell, E., Doyle, P.S., Caffrey, C.R., Holler, T.P., McKerrow, J.H., Cohen, F.E. Synthesis and structure–activity relationship study of potent trypanocidal thio semicarbazone inhibitors of the trypanosomal cysteine protease cruzain. J. Med. Chem. 2002, 45, 2695–2707.
- Hall, I.H., Chen, S.Y., Barnes, B.J., West, D.X. The hypolipidemic activity of heterocyclic thiosemicarbazones, thioureas and their metal complexes in Sprague Dawley male rats. Met. Based Drugs 1999, 6, 143–147.
- Bermejo, E., Carballo, R., Castineiras, A., Dominguez, R., Liberta, E.A., Maichle-Mossmer, C, West, D.X. Complexes of group 12 metals with 2-acetylpyridine 4N-dimethylthiosemicarbazone and with 2-acetylpyridine-N-oxide 4N-dimethylthiosemicarbazone: synthesis, structure and antifungal activity. Z. Naturforsch. 1999, 54, 777–787.
- Pérez, J.M., Matesanz, A.I., Martín-Ambite, A., Navarro, P., Alonso, C., Souza, P. Synthesis and characterization of complexes of p-isopropyl benzaldehyde and methyl 2-pyridyl ketone thiosemicarbazones with Zn(II) and Cd(II) metallic centers. Cytotoxic activity and induction of apoptosis in Pam-ras cells. J. Inorg. Biochem. 1999, 75, 255–261.
- Reddy, K.H., Reddy, P.S., Babu, P.R. Synthesis, spectral studies and nuclease activity of mixed ligand copper(II) complexes of heteroaromatic semicarbazones/thiosemicarbazones and pyridine. J. Inorg. Biochem. 1999, 77, 169–176.
- Kelly, P.F., Slawin, A.Z.M., Soriano-Rama, A. Reaction of (Me3SiNSN)2S with palladium complexes; crystal structures of [PPh4]2[Pd2Br4(S3N2)] and [PPh4][PdBr2(S2N3)]. J. Chem. Soc. Dalton Trans. 1996, 53–60.
- West, X.D., Pardhye, B.S., Sonawane, B.P. Structural and physical correlation in the biological properties of transition metal N-hetero-cyclic thiosemicarbazones and S-alkyldithiocarbazate complexes. Struct. Bonding 1991, 76, 1–50.
- Liberta, E.A., West, X.D. Antifungal and antitumor activity of heterocyclic thiosemicarbazones and their metal complexes: current status. BioMetals. 1992, 5, 121–126.
- West, X.D., Liberta, E.A., Padhye, B.S., Chikate, C.R., Sonawane, B.P., Kumbhar, S.A., Yerande, G.R. Thiosemicarbazone complexes of copper(II): structural and biological studies. Coord. Chem. Rev. 1993, 123, 49–71.
- Jeyaraman, R., Avila, S. Chemistry of 3-azabicyclo[3.3.1]nonanes. Chem. Rev. 1981, 81, 149–174.
- Lijinsky, W., Taylor, H.W. Carcinogenicity of methylated nitrosopiperidines. Int. J. Cancer 1975, 16, 318–322.
- Cartoni, C., Figini, A., Carpi, A. [Antiarrhythmic action of certain 1,5-diphenyl-bispidin derivatives]. Ann. Ist. Super. Sanita 1968, 4, 333–335.
- El-Subbagh, H.I., Abu-Zaid, S.M., Mahran, M.A., Badria, F.A., Al-Obaid, A.M. Synthesis and biological evaluation of certain alpha, beta-unsaturated ketones and their corresponding fused pyridines as antiviral and cytotoxic agents. J. Med. Chem. 2000, 43, 2915–2921.
- Aridoss, G., Amirthaganesan, S., Kumar, N.A., Kim, J.T., Lim, K.T., Kabilan, S., Jeong, Y.T. A facile synthesis, antibacterial, and antitubercular studies of some piperidin-4-one and tetrahydropyridine derivatives. Bioorg. Med. Chem. Lett. 2008, 18, 6542–6548.
- Karthikeyan, P., Meena Rani, A., Saiganesh, R., Balasubramanian, K.K., Kabilan, S. Synthesis of 5,6-dihydrobenz[c]acridines: a comparative study. Tetrahedron 2009, 65, 811–821.
- Aridoss, G., Parthiban, P., Ramachandran, R., Prakash, M., Kabilan, S., Jeong, Y.T. Synthesis and spectral characterization of a new class of N-(N-methylpiperazinoacetyl)-2,6-diarylpiperidin-4-ones: antimicrobial, analgesic and antipyretic studies. Eur. J. Med. Chem. 2009, 44, 577–592.
- Aridoss, G., Balasubramanian, S., Parthiban, P., Kabilan, S. Synthesis, stereochemistry and antimicrobial evaluation of some N-morpholinoacetyl-2,6-diarylpiperidin-4-ones. Eur. J. Med. Chem. 2007, 42, 851–860.
- Aridoss, G., Balasubramanian, S., Parthiban, P., Kabilan, S. Synthesis and in vitro microbiological evaluation of imidazo(4,5-b)pyridinylethoxypiperidones. Eur. J. Med. Chem. 2006, 41, 268–275.
- Aridoss, G., Balasubramanian, S., Parthiban, P., Kabilan, S. Synthesis and NMR spectral studies of N-chloroacetyl-2,6-diarylpiperidin-4-ones. Spectrochim. Acta. A. Mol. Biomol. Spectrosc. 2007, 68, 1153–1163.
- Dhar, M.L., Dhar, M.M., Dhawan, B.N., Mehrotra, B.N., Ray, C. Screening of Indian plants for biological activity: I. Indian J. Exp. Biol. 1968, 6, 232–247.
- Baliah, V., Jeyaraman, R. Synthesis of some 3-azabicyclo[3.3.1]nonane. Indian J. Chem. 1971, 9, 1020–1022.
- Klod, S., Koch, A., Kleinpeter, E. Ab-initio quantum-mechanical GIAO calculation of the anisotropic effect of C–C and X–C single bonds—application to the 1H NMR spectrum of cyclohexane. J. Chem. Soc. Perkin. Trans. 2002, 2, 1506–1509.
- Iriepa, I., Madrid, A.I., Galvez, E., Bellanato, J. Synthesis, structural and conformational study of 3-methyl-3-azabicyclo[3.2.1]octan-8-one and 3-methyl-3-azabicyclo[3.3.1]nonan-9-one oximes. J. Mol. Struct. 2003, 651–653, 579–583.