Abstract
Several N-substituted maleimides containing substituents of varying bulkiness and polarity were synthesised and tested for antimicrobial and cytostatic activity. Neutral maleimides displayed relatively strong antifungal effect minimum inhibitory concentrations (MICs in the 0.5–4 µg ml−1 range); their antibacterial activity was structure dependent and all were highly cytostatic, with IC50 values below 0.1 µg ml−1. Low antimicrobial but high cytostatic activity was noted for basic maleimides containing tertiary aminoalkyl substituents. Chemical reactivity and lipophilicity influenced antibacterial activity of neutral maleimides but had little if any effect on their antifungal and cytostatic action. N-substituted maleimides affected biosynthesis of chitin and β(1,3)glucan, components of the fungal cell wall. The membrane enzyme, β(1,3)glucan synthase has been proposed as a putative primary target of N-ethylmaleimide and some of its analogues in Candida albicans cells.
Introduction
Microbial resistance to antimicrobials is an emerging challenge for clinicians and pharmaceutical industry. The multi-drug-resistant bacteria and fungi are the major cause of failure in chemotherapy of infectious diseases. Thus, the need for novel antimicrobial agents is especially urgent. Among different strategies of searching for new potential drugs, identification of novel, unexploited molecular targets and their inhibitors seems one of the most promising. These days many researchers concentrate on bacterial and fungal enzymes that catalyse important biochemical reaction in microbial cells. Bioactive compounds that selectively inactivate such proteins and block metabolic pathways in human pathogenic bacteria and fungi could be potential antimicrobial drugs.
Although N-ethyl maleimide (NEM) may react with amines and imidazole derivatives to form N-acylation products, it is widely known as a thiol-reacting compoundCitation1 and as such is an effective inhibitor of several enzymes containing reactive cysteinyl residues, essential for their catalytic activityCitation2–4. Because many of these enzymes are important for growth and survival of micro-organisms, antimicrobial activity of NEM is not surprising. Antimicrobial properties of NEM and other N-substituted maleimides have been already reportedCitation5–9; however, none of those studies related antimicrobial activity of substituted maleimides to their physicochemical properties, including chemical reactivity and lipophilicity. In the present work, such correlation has been attempted for 12 various N-substituted maleimides. Moreover, novel information on molecular mechanism of antifungal action of compounds studied has been provided.
Materials and methods
Chemistry
Solvents and reagents were purchased from Sigma-Aldrich. Melting points are uncorrected. 1H NMR spectra were recorded on the Varian Gemini 200 and 500 MHz spectrometers. UV–vis spectra were recorded in an UV–vis Lambda 45, Perkin Elmer spectrophotometer. Thin layer chromatography (TLC) was performed on silica gel (Merck) in a solvent system benzene:ethyl acetate:ethanol (50:15:5). Elementary analysis (EA) was run on the Carlo Erba, type EA 1108 analyser.
General procedure for the synthesis of maleimides 3–10
Maleic anhydride (20 mmol) was dissolved in 20 ml of CH2Cl2. The stirred solution was cooled to 0–5°C and equimolar amount of an appropriate amine dissolved in 15 ml of CH2Cl2 was added to the mixture. The resulting suspension was refluxed for 2 h and then cooled to room temperature. The precipitated solids were filtered off and washed twice with ethyl ether. The maleamic acids formed in the first step (15 mmol) were dissolved in 24 ml of acetic anhydride and anhydrous sodium acetate (10 mmol) was added to the mixture. The resulting suspension was refluxed and reaction progress was followed by TLC. When the reaction was completed, the mixture was cooled and 100 ml of water was added. The resulting solution of maleimide in water was extracted with ethyl acetate (3 × 50 ml), extracts were combined, dried with MgSO4, filtered and the solvent was evaporated. Crude products were re-crystallised from the solvent or distilled.
Basic maleimides 11 and 12 were synthesised according to the described proceduresCitation10,Citation11.
N-cyclohexylmaleimide (3)
Yield 70% and m.p. 84°C. 1H NMR (CDCl3): δ [ppm]: 7.29 (s, 1H, = CH); 6.65 (s, 1H, HC=); 3.93 (t, 1H, J = 3.9 Hz, CH); 2.08 (qd, 2H, J = 12.9 Hz and 3.9 Hz, CH2); 1.86 (d, 2H, J = 13.2 Hz, CH2); 1.68 (m, 3H, CH2), 1.3 (m, 3H, CH2). Analytically found (Anal. Found): C 66.93, H 7.27, N 7.78%. Calculated for C10H13NO2: C 67.02, H 7.31, N 7.82%.
N-benzylmaleimide (4)
Yield 58% and m.p. 69°C. 1H NMR (CDCl3) δ [ppm]: 7.35 (m, 5H, C6H5); 6.72 (s, 2H, CH=CH); 4.69 (s, 2H, CH2). Anal. found: C 71.48, H 5.01, N 7.42%. Calculated for C11H9NO2: C 71.48, H 4.85, N 7.48%.
N-(2,6-dimethylphenyl)maleimide (5)
Yield 51% and m.p. 105–107°C. 1H NMR (CDCl3) δ [ppm]: 7.1 (m, 3H, C6H5); 6.8 (s, 2H, CH=CH); 2.2 (s, 6H, CH3). Anal. found: C 69.34, H 5.58, N 6.83%. Calculated for C12H11NO2: C 69.63, H 5.51, N 6.95%.
N-(2,4,6-trimethylphenyl)maleimide (6)
Yield 64% and m.p. 99–100°C. 1H NMR (CDCl3) δ [ppm]: 6.7 (m, 5H, C6H5); 6.6 (s, 2H, CH=CH); 2.1 (s, 3H, CH3); 2.7 (s, 6H, CH3). Anal. found: C 72.21, H 6.11, N 6.45%. Calculated for C13H13NO2: C 72.54, H 6.09, N 6.51%.
N-(2-tert-butylphenyl)maleimide (7)
Yield 85% and m.p. 90°C. 1H NMR (CDCl3) δ [ppm]: 7.2 (d, 1H, J = 8 Hz, C6H5); 7.1 (t, 1H, J = 7 Hz, C6H5); 7.9 (s, 1H, = CH); 6.93 (d, 1H, C6H5); 6.2 (s,1H, CH=); 1.1 (s, 9H, (CH3)3C). Anal. found: C 73.11, H 6.54, N 6.07%. Calculated for C14H15NO2: C 73.34, H 6.59, N 6.11%.
N-(4-bromophenyl)maleimide (8)
Yield 53% and m.p. 118–119°C. 1H NMR (CDCl3) δ [ppm]: 7.73 (m, 2H, C6H5); 7.71 (m, 2H, C6H5); 6.7 (s, 2H, CH=CH). Anal. found: C 47.66, H 2.51, N 5.32%. Calculated for C10H6NO2Br: C 47.65, H 2.40, N 5.36%.
N-(4-methoxyphenyl)maleimide (9)
Yield 58% and m.p. 150–151°C. 1H NMR (CDCl3) δ [ppm]: 7.5 (d, 2H, J = 8.8 Hz, C6H5); 7.2 (d, 2H, J = 8.8 Hz, C6H5); 6.5 (s, 2H, CH=CH); 3.4 (s, 3H,−OCH3). Anal. found: C 64.98, H 4.48, N 6.79%. Calculated for C11H9NO3: C 65.02, H 4.46, N 6.89%.
N-(4-nitrophenyl)maleimide (10)
Yield 70% and m.p. 161°C. 1H NMR (CDCl3) δ [ppm]: 8.33 (d, 2H, J = 2 Hz, C6H5); 7,.8 (d, 2H, J = 2 Hz, C6H5); 6.3 (s, 2H, CH=CH). Anal. found: C 55.04, H 2.75, N 12.77%. Calculated for C10H6N2O4: C 55.05, H 2.77, N 12.84%.
N-[2-(N,N-dimethylamino)ethyl]maleimide (11)
Yield 52% and m.p. 175–176°C. 1H NMR (CDCl3): δ [ppm]: 6.67 (s, 2H, CH=CH), 3.61 (t, J = 6.3 Hz, 2H, CH2), 2.47 (t, J = 6.4 Hz, 2H, CH2), 2.23 (s, 6H, CH3). Anal. found: C 56.99, H 7.09, N 16.51%. Calculated for C8H12N2O2: C 57.14, H, 7.14, N 16.67%.
N-[2-(N-morpholinoethyl]maleimide (12)
Yield 33% and m.p. 132°C. 1H NMR (CDCl3): δ [ppm]: 6.65 (s, 2H, CH=CH), 3.55 (t, J = 6.8 Hz, 2H, CH2), 2.49 (t, J = 6.8 Hz, 2 H, CH2), 2.42 (m, 8H, CH2). Anal. found: C 56.99, H, 6.48, N 13.17%. Calculated for C10H14N2O3: C 57.14, H 6.67, N 13.33%.
Determination of the model reaction rate
Progress of the reaction between maleimides and N-acetyl methyl cysteinate (ACME) was determined spectrophotometrically, measuring decrease in the absorption at 300 nm (A300). Stock solutions of maleimides (50 µmol ml−1 in dimethyl sulfoxide) and ACME (50 µmol ml−1 in water) were prepared. Sample of the maleimide solution (50 µl) was added to 1400 µl of the sodium phosphate buffer (pH 5.0 or 7.0) and A300 of the resulting solution was recorded. Reaction was initiated by addition of 50 µl of the ACME solution and measurement of A300 was continued at room temperature. Concentration of a particular maleimide in the reaction mixture at the given moment was calculated from the standard curves A300 = f(c) determined separately for each compound from the 1–12 series. Data were plotted as 1/c versus time, to give the second order rate constants k as slopes of the linear plots.
Calculation of logP
Values of the theoretical 1−octanol/water partition coefficients (logP) were calculated for all compounds using the ALOGPS 2.1 softwareCitation12.
Determination of antimicrobial activity
Antifungal activity was determined by the M27-A2 method specified by CLSI (formerly NCCLS) in RPMI-1640 medium buffered to pH 7.013. The test micro-organisms were Candida albicans ATCC 10231, Candida glabrata DSM 11226, Candida tropicalis KKP 334 and Saccharomyces cerevisiae ATCC 9763. Antibacterial activity was determined by the microtitre plate method in Tryptic Soy Broth medium, pH 7.0 (GibcoBRL) against Gram-negative Escherichia coli ATCC 10536 and Gram-positive Staphylococcus aureus ATCC 6538 and Bacillus subtilis ATCC 6633. Wells containing serially diluted examined compounds and compound-free control were inoculated with 12-h cultures of tested strains to the final concentration of 104 colony-forming units (cfu) ml−1. Plates were incubated for 24 h at 37°C and growth was then quantified by measuring an optical density at 531 nm, using the microplate reader (VictorCitation3V; Perkin Elmer, Centre of Excellence ChemBioFarm). The MIC was defined as the lowest drug concentration at which at least 80% decrease in turbidity, in comparison to the drug-free control, was observed.
The number of viable yeast cells was determined by subculturing diluted cell suspensions on agar plates. Samples of 0.05 ml were collected from microtitre plate wells, appropriately diluted with sterile water and aliquots of 0.1 ml were spread onto the surface of Sabouraud agar plates. Plates were incubated at 30°C for 24 h and colonies were counted to give the cfu values.
Morphological observations
C. albicans cells from the overnight cultures in Sabouraud medium were harvested, washed with sterile saline and suspended in RPMI-1640 medium to the final cell density of ≈105 cfu ml−1. Tested compounds were added at appropriate concentrations and cultures were incubated for 6 h at 30°C, with shaking. At time intervals, samples of 0.1 ml were collected and cells were stained for chitin or glucan. For chitin staining, the culture sample was combined with 0.1 ml of the Calcofluor White solution (0.3 mg ml−1 in 25 mM phosphate-buffered saline (PBS), pH 7.0) and incubated for 5 min at 25°C. The cells were then harvested, washed four times with PBS, re-suspended in 0.1 ml of the fresh PBS and transferred to a microscopic slide. Staining for glucan was performed using an aniline blue dye, as described by Kippert and LloydCitation14. Aliquots of the cell suspension (0.1 ml) were harvested, washed four times with PBS (pH 7.5), mounted in the aniline blue solution (0.5 mg ml−1 in PBS) and transferred to a microscopic slide. The stained cells were examined with the Olympus BX 60 F5 fluorescence microscope and photographs were taken using the Studio Lite software (Pixera Corporation).
Preparation of the mixed membrane fraction and glucan synthase activity assay
The mixed membrane fractions (MMFs) of C. albicans cells were prepared using the method of Cabib and KangCitation15. Activity of β(1,3)-glucan synthase present in MMF was measured with uridine-5′-diphospho-d-glucose as a substrate, using the microtitre plate fluorescence method described by Shedletzky et al.Citation16, based on the quantification of the aniline blue–glucan complex. The fluorescence was measured with the microplate reader at the excitation wavelength of 400 nm and the emission wavelength of 460 nm.
Determination of chitin synthase activity and measurement of chitin content
Chitin synthase activity was determined in MMF of C. albicans cells by the method of Munro et al.Citation17 The MMFs were activated by 5-min incubation with 100 ng trypsin μl−1 MMF at 30°C and the reactions were stopped by addition of 150 ng soybean trypsin inhibitor μl−1 MMF. Standard reactions for measuring chitin synthase activity were carried out in a 50 μl volume and were composed of: 50 μg MMF protein, 25 mM N-acetyl-D-glucosamine, 1 mM UDP-N-acetyl-D-glucosamine that included 25 nCi UDP-[U-14C] N-acetyl-D-glucosamine, 50 mM Tris–HCl pH 7.5, 10 mM MgCl2 and an inhibitor tested at appropriate concentration (0–200 μg ml−1 range). Incubations were carried out at 30°C for 30 min and the reaction was stopped by addition of 1 ml of 66% (v/v) ethanol. The reaction mixture was then filtered through GF/C filter discs (Whatman), which had been pre-soaked in 10% (v/v) trichloroacetic acid. The reactions tubes were rinsed out with 2 × 1 ml of 1% (v/v) Triton X-100 and each filter was then washed with 4 × 2 ml of 66% (v/v) ethanol. The radio-labelled chitin synthesised in the reaction was trapped on the filters and unincorporated substrate was removed by washing. Filters were dried at 80°C and their radioactivity counted in a scintillation counter.
For the quantitative determination of chitin content in cells cultivated for 24 h in the presence of maleimides, the amount of N-acetyl-D-glucosamine released by chitinase/cytohelicase was measured by the colorimetric method described previouslyCitation18. Reaction mixtures contained 0.1 U ml−1 of chitinase from Streptomyces griseus and 1 mg ml−1 of cytohelicase from Helix pomatia.
Determination of haemolytic effect
Human red cells were re-suspended in cold saline to the final number of 2 × 107 cells ml−1. Compounds were added to the cell suspensions, samples were incubated at 37°C for 30 min and then centrifuged (1700g, 10 min, 4°C). Supernatants were collected and absorbance at 540 nm was measured to determine haemoglobin concentration. The maximal possible haemolysis level (positive control) was determined in supernatants obtained from cell suspensions pre-treated with 0.1 % Triton X-100. Values of drug concentration causing 50% haemolysis in comparison with the positive control (EH50) were read from the A540 = f(concentration) plots.
Cytotoxicity assay
Human cervix carcinoma HeLa S3 cells were maintained in high glucose Dulbecco’s modified Eagle’s medium (pH 7.0) supplemented with 10% foetal bovine serum, 2 mM l-glutamine and antibiotics (100 units ml−1 penicillin and 100 mg ml−1 streptomycin) at 37°C in 10% CO2/air atmosphere. Cells were screened routinely for Mycoplasma by the PCR method with Mycoplasma Plus PCR Primer Set (Stratagene, La Jolla, CA). The cytotoxicity was determined by the MTT assay. Briefly, exponentially growing cells were attached at 3 × 104 cells per well in a 24-well multi-well plates and the cellular viability was determined after 120 h of continuous exposure to different drug concentrations. Cells were incubated with the MTT (3-(4,5-dimethylthiazol-2-yl)-2,5-diphenyltetrazolium tetrazolium salt) for 4 h at 37°C, and formazan formation was measured by a microplate reader. The concentrations required to inhibit cell growth by 50% compared to untreated controls (IC50) were determined from the curves plotting survival as a function of dose by use of the Slide Write program. All values are averages of at least two independent experiments, each done in duplicate.
Results
Synthesis of N-substituted maleimides
N-Ethylmaleimide 1 (NEM) and N-tert-butylmaleimide 2 were purchased from Sigma-Aldrich. N-substituted maleimides 3–10 were synthesised in a two-step procedure (). In the first step, N-substituted maleamic acid derivatives were formed upon reaction of maleic anhydride and an appropriate amine. Cyclisation to N-substituted maleimides 3–10 was achieved by heating of maleamic acid derivatives in the presence of acetic anhydride containing catalytic amounts of sodium acetate. N-[2-(N,N-dimethylamino)ethyl]maleimide 11 and N-[2-(N-morpholinoethyl]maleimide 12 were synthesised according to the literature procedures.
Chemical reactivity and lipophilicity of maleimides
Chemical reactivity of N-substituted maleimides 1–12 was determined in the model reaction with ACME, mimicking cysteinyl residues present at the enzyme active centres. Reactions were carried out at room temperature, in buffered aqueous media, at pH 5.0 and 7.0. Reagents were present in equimolar amounts. All reactions proceeded according to , leading to the formation of respective products of nucleophilic 1,4 addition, as was confirmed by high-performance liquid chromatography (HPLC) and 1H NMR analysis. The NMR data of products formed in the reactions of ACME with maleimides 1–12 were consistent with structures of general formula shown in . For example, reaction of ACME with N-benzylmaleimide 4 afforded product with the following spectral data: 1H NMR (CDCl3) δ [ppm]: 8.19 (m, 1H, NH); 7.35 (m, 5H, C6H5); 4.71 (s, 2H, CH2Ph); 4.38 (m, 1H, CHC(O)); 3.74 (t, 1H, CHS); 3.58 (s, 3H, CH3O); 3.01 (m, 2H, CH2C(O)); 2.84 and 2.74 (AB, 2H, CH2S); 1.92 (s, 3H, CH3C(O)). Kinetics of reactions of ACME with maleimides was also studied. The reaction progress was monitored spectrophotometrically at 300 nm, by measuring decrease of absorption deriving from the maleimide chromophore. The determined k values are summarised in . As expected for the nucleophilic 1,4-addition of thiols to the conjugated double bond system, reactions of ACME with maleimides were faster at higher pH, due to the enhanced thiol nucleophilicity. This finding confirms the previous observations concerning reaction of NEM with reduced glutathioneCitation19. Differences in relative reactivity of maleimides at the given pH were noted. The most reactive compounds exhibited velocity constants more than an order of magnitude higher than the least reactive ones. Relatively high reactivity was observed for NEM 1 and maleimides with benzyl or phenylalkyl substituents at the nitrogen atom (4–7), while lower for compounds containing polar fragments in their N-aryl or N-alkyl substituents (8–12) and for bulky N-tert-butyl maleimide 2 and N-cyclohexyl maleimide 3.
Table 1. Lipophilicity and chemical reactivity of maleimides.
also contains values of logP parameters, calculated for compounds 1–12, using the ALOGPS 2.1 software. The maleimides under study differed markedly in terms of their lipophilicity, reflected by the calculated values of logP. Although NEM 1 was only about two times better soluble in octanol than in water, solubility of the most hydrophobic N-(2-tert-butylphenyl)maleimide 7 in octanol was almost 500 times higher than that in water. Generally, compounds 1–10 should be considered moderately or highly hydrophobic, whereas 11 and 12 are moderately hydrophilic.
The maleimide ring is known to undergo hydrolysis to maleamic acid in a pH-dependent manner, especially quickly in alkaline media19, 20. HPLC analysis of buffered aqueous solutions of maleimides 1–12 revealed that these compounds were completely stable at pH 4–6.5 for at least 24 h and only a very slow decomposition to maleamic acid derivatives was observed at pH 6.5–7.0, with 5–11% of the decomposition products observed at pH 7.0 after 24 h. Therefore, a possibility of substantial decomposition of maleimides under conditions of determination of their antimicrobial, haemolytic and cytostatic activity (pH ≤ 7.0) should be excluded.
Antimicrobial activity
The N-substituted maleimides 1–12 were tested for growth inhibitory activity against four yeast and three bacterial strains. MICs of the studied compounds were determined by the microplate serial dilution method. Data shown in indicate that all compounds but 11 and 12 exhibited strong antifungal activity, with MICs ranging between 0.5 and 4 µg ml−1. This activity was comparable to that of known antifungals, Nystatin and Fluconazole. Compounds 11 and 12 were notable exceptions, demonstrating much lower antifungal activity. On the other hand, antibacterial activity of 1–12 was differentiated but generally low, with MICs between 1 and 128 µg ml−1. Especially high MIC values were noted against Gram-negative E. coli. N-(4-bromophenyl)maleimide 8, N-(4-methoxyphenyl)maleimide 9, N-(4-nitrophenyl)maleimide 10 and two basic maleimides 11 and 12 showed the lowest antibacterial activity among the all compounds tested, whereas the highest was observed for 1.
Table 2. Antibacterial and antifungal in vitro activity of maleimide compounds and reference antifungals.
Effect of maleimides action on C. albicans viability and morphology of cells
C. albicans cells grown in RPMI-1640 medium were treated with malemides 1–10, 8 µg ml−1, i.e., concentration exceeding their MIC values. Cells were counted and changes in cell morphology were monitored by fluorescence microscopy after staining of fungal cell wall components with fluorescent dyes. Action of all compounds resulted in a substantial reduction of the cell number, reflected by the cfu ml−1 value, dropped from 105 cfu ml−1 to 1–1.4 × 104 cfu ml−1. The most striking changes in cell morphology shown in included apparent “chaining” of the cells, most likely resulting from cell agglutination or formation of pseudohyphal forms and in some cases evident shrinking and distortion of dye-stained cell wall constituents, especially those stained with the β(1,3)-glucan-specific aniline blue, was observed.
Figure 1. Visualisation of the effect of maleimides on morphology of Candida albicans yeast cells. (A–C) Cells stained for chitin with Calcofluor White. (A) Control, (B) cells treated with 1, 5 µg ml−1; (C) cells treated with 7, 5 µg ml−1. (D–F) Cells stained for glucan with aniline blue. (D) Control, (E) cells treated with 1, 5 µg ml−1; (F) cells treated with 7, 5 µg ml−1.
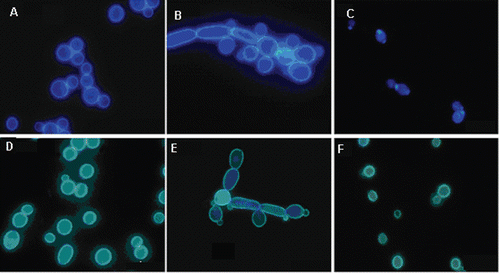
Influence of maleimides on chitin content of C. albicans cells and activity of chitin synthase and β(1, 3)-glucan synthase
Action of maleimides on C. albicans slightly affected chitin content in the yeast cells. Compared to the control untreated cells, cell treated with maleimides 1–10 at 5 µg ml−1 contained 10–12% less chitin, while the treatment with maleimides at 50 µg ml−1 reduced the chitin content by 20–25%. No reduction of chitin content was observed for cells treated with 11 or 12 at 50 µg ml−1. Slight inhibition of chitin synthase activity present in the MMF of C. albicans cells by maleimides tested was noted. Enzyme activity was 14–21% lower in samples containing malemides 1–12 at 50 µg ml−1 but none of the compounds studied reached 50% inhibition of chitin synthase activity at concentration <200 µg ml−1. In the parallel determination, IC50 = 9.5 ± 0.5 µg ml−1 was determined for nikkomycin Z, a well-known inhibitor of chitin synthaseCitation21.
On the other hand, a notable in vitro inhibition by maleimides 1–10 of β(1,3)-glucan synthase activity present in MMF was noted. Exemplary data shown in clearly indicate that at 5 µg ml−1, i.e., concentration slightly higher than the MICs of these maleimides, enzyme activity was inhibited in about 35%, while at 50 µg ml−1 the extent of inhibition was enhanced to 60–65%. Concentrations causing 50% inhibition of the enzyme activity (IC50) were: 8.5 ± 1.1 µg ml−1 for 1, 13.7 ± 2.3 µg ml−1 for 2 and 21.2 ± 2.5 µg ml−1 for 8, respectively. Similar enzyme inhibitory potency, with IC50 values within the 8–25 µg ml−1 range, was noted for other maleimides, except for 11 and 12, where only 20–24% inhibition was observed at 100 µg ml−1. The known glucan synthase inhibitor, trans-cinnamaldehydeCitation22, inhibited the enzyme with IC50 = 140 ± 8 µg ml−1.
Haemolytic and cytostatic activity
N-substituted maleimides 1–12 were tested for haemolytic activity against human erythrocytes. None of the studied compounds at concentrations <300 µg ml−1 induced haemoglobin release from red blood cells at a measurable rate. Higher concentrations could not be tested because of the solubility problems. In the parallel determination, a well-known membrane-active antifungal antibiotic Nystatin caused rapid haemolysis and disruption of 50% of erythrocytes was noted at 80 µg ml−1 of this compound. It may be therefore concluded that N-substituted maleimides are not haemolytic and do not disturb membrane function in erythrocytes.
The maleimide derivatives were also tested for cytostatic activity in tissue culture against HeLa S3 cells. All compounds appeared highly cytostatic. The ED50 values for 1–12 were lower than 0.1 µg ml−1, for example: 0.068 ± 0.007 µg ml−1 for 1, 0.045 ± 0.008 µg ml−1 for 4, and 0.05 ± 0.01 µg ml−1 for 9, while for the reference, known cytostatic agent doxorubicin, the respective value was 0.011 ± 0.009 µg ml−1 −
Discussion
A series of neutral N-substituted maleimides (1–10) studied in this work displayed reasonably high antifungal activity, with very little if any structure–antifungal activity relationship. On the other hand, the antibacterial activity of NEM 1 and its derivatives 2–12 was generally lower and structure dependent. The especially low activity of all compounds studied but NEM against Gram-negative E. coli seems to be related to their lipophilicity and bulkiness, as high MICs (<32 µg ml−1) were found for the compounds 2–10 exhibiting logP values higher than 0.8 and containing relatively large substituents, while the lowest for the least lipophilic and the smallest NEM 1. Biological basis of this difference is not known but one may assume that the lipophilic, relatively large maleimide derivatives do not cross the bacterial outer membrane and remain captured there, unable to reach any intracellular targets, whereas the smallest and less hydrophobic NEM easily penetrates the outer barrier and reaches an unidentified target in the periplasmic space, cytoplasmic membrane or cytosol. The low activity of moderately hydrophilic basic maleimides 11 and 12 against E. coli seems to be an exception to this rule; however, their relative bulkiness and presence of several hydrophobic regions in these molecules may be a reason for the strongly reduced activity against Gram-negative bacteria. In the case of Gram-positive bacteria, three compounds, namely 8, 9 and 10, bearing more or less polar substituents in the phenyl ring, as well as the basic maleimides 11 and 12, were apparently less active than the other ones. Reason for this difference is not known, although it does not seem to be directly related to the general lipophilicity. Because compounds 8–12, demonstrating the lowest activity against Gram-positive bacteria, were found to be the least reactive in the model reaction with ACME, it is possible that their low growth inhibitory potency against B. subtilis and S. aureus results from poor reactivity against an unidentified target containing essential cysteinyl residue.
Undoubtedly, differences in chemical reactivity and lipophilicity did not influence the antifungal activity of the maleimide derivatives tested, except for the two compounds containing N-aminoalkyl substituents 11 and 12, demonstrating much lower antifungal efficacy. Reduction of the cell number and changes in morphology of C. albicans cells treated with highly active compounds at concentrations exceeding their MIC values indicated the cell lysis and suggested a possible effect of these maleimides on formation of cell wall components. This was checked in two ways: (1) quantification of possible influence on chitin biosynthesis by monitoring the total chitin content in C. albicans cells treated with maleimides and (2) in vitro effect of maleimides on activity of C. albicans chitin synthase and β(1,3)-glucan synthase in MMF. Although the compounds studied only slightly affected chitin content and chitin synthase activity, a substantial inhibition of β(1,3)-glucan synthase was noted. All neutral maleimides at concentrations slightly exceeding their MIC values inhibited enzyme activity by more than 50%. In this respect, it is worth mentioning that fungal β(1,3)-glucan synthases contain sulfhydryl groups, important for their activity, localised most probably in the GTP-ase part of the enzyme complexCitation23. Our present finding confirms previous reports on inhibition of β(1,3)glucan synthase activity by other sulfhydryl-acting compoundsCitation24. The previously reported inhibition of S. cerevisiae β(1,3)-glucan synthase by N-phenylpropyl-3,4-dichloromaleimideCitation9 also supports our findings, as that compound should be considered a good electrophilic acceptor for nucleophilic 1,4-addition of reactive sulfhydryl group(s) present in glucan synthase.
The facts that maleimides tested strongly inhibited β(1,3)-glucan synthase activity at concentrations close to those inhibiting fungal growth, they were fungicidal and induced morphological alterations characteristic for the consequences of cell wall inhibition, suggested that the enzyme might be a primary molecular target of these compounds in yeast cells. Because glucan synthase, responsible for the formation of the main component of C. albicans cell wall and thus essential for cell viability, is a cytoplasmic membrane-localised protein, it is supposed to be especially exposed to the action of hydrophobic inhibitors that may accumulate and concentrate in the membrane lipidic environment. It is worth mentioning, therefore, that this enzyme is inhibited by many structurally unrelated but generally hydrophobic compounds, like glycolipidic papulacandins, acid terpenoids and N-acylated cyclic hexapeptidesCitation25, but also by the trans-cinnamaldehyde as well as by chalcone derivatives, including those developed in our laboratoryCitation22,Citation26,Citation27, both containing the conjugated double bond systems, that could serve as electrophilic acceptors in nucleophilic 1,4-addition. Many of the above-mentioned compounds targeting glucan synthase inhibit enzyme activity in vitro by more than 50%, at concentrations slightly higher than their MICsCitation27,Citation28. The same phenomenon was found by us for maleimides 1–10 what additionally points at glucan synthase as the primary target of these compounds in C. albicans. Much lower antifungal activity of moderately hydrophilic basic maleimides 11 and 12 and low inhibitory potential of these compounds against β(1,3)-glucan synthase present in the membrane fraction could be explained by their hydrophilicity and possible low accumulation in the membrane, although the presence of a positively charged aminoalkyl substituent may also directly affect affinity to the binding site at the enzyme molecule.
The maleimides tested appeared strongly cytostatic against mammalian cells in tissue culture, despite the fact that they did not induce lysis of human erythrocytes. However, the latter can be easily explained by the fact that erythrocytes are unusual cells, rather “biological sacs” filled with haemoglobin, so that one can hardly find there any potential target for maleimides. Lack of the haemolytic effect thus only indicates that maleimides are not membrane-disrupting compounds. On the other hand, in complete mammalian cells, there are many enzymes containing thiol groups that may be targeted by maleimides. Most of them are localised in the cell interior, so that one may suppose that all maleimides used in our studies eventually crossed the mammalian cell membrane, not targeting β(1,3)-glucan synthase, as this enzyme is absent in mammalian cells. Most probably, the observed inhibition of growth of HeLa cells is a consequence of the previously reported anti-mitotic activity of maleimidic compoundsCitation29.
Mammalian toxicity of maleimides disqualifies these compounds as drug candidates for chemotherapy of disseminated fungal infections, but their use for the treatment of superficial mycoses seems possible, as was previously shown for some other maleimide derivatives in an animal model of trichophytosisCitation30. Therefore, it seems that hydrophobic maleimides have potential to become components of medicinal preparations for the treatment of cutaneous fungal infections.
Declaration of interest
The authors report no conflict of interests.
References
- Smyth DG, Nagamatsu A, Fruton JS. Some reactions of N-ethylmaleimide. J Am. Chem Soc 1960;82:4600–4804.
- Han KK, Delacourte A, Hemon B. Chemical modification of thiol group(s) in protein: Application to the study of anti-microtubular drugs binding. Comp Biochem Physiol, B 1987;88:1057–1065.
- Shaw E. Cysteinyl proteinases and their selective inactivation. Adv Enzymol Relat Areas Mol Biol 1990;63:271–347.
- Matuszak N, Muccioli GG, Labar G, Lambert DM. Synthesis and in vitro evaluation of N-substituted maleimide derivatives as selective monoglyceride lipase inhibitors. J Med Chem 2009;52:7410–7420.
- Takatori K, Hasegawa T, Nakano S, Kitamura J, Kato N. Antifungal activities of N-substituted maleimide derivatives. Microbiol Immunol 1985;29:1237–1241.
- Igarashi Y, Yagami K, Imai R, Watanabe S. Antimicrobial activities of some N-aklylmaleimides. J Industr Microbiol 1990;6:223–225.
- Zentz F, Valla A, Le Guillou R, Labia R, Mathot AG, Sirot D. Synthesis and antimicrobial activities of N-substituted imides. Farmaco 2002;57:421–426.
- Sortino M, Cechinel Filho V, Corrêa R, Zacchino S. N-Phenyl and N-phenylalkyl-maleimides acting against Candida spp.: Time-to-kill, stability, interaction with maleamic acids. Bioorg Med Chem 2008;16:560–568.
- López SN, Castelli MV, de Campos F, Corrêa R, Cechinel Filho V, Yunes RA, Zamora MA, Enriz RD, Ribas JC, Furlán RL, Zacchino SA. In vitro antifungal properties structure-activity relationships and studies on the mode of action of N-phenyl, N-aryl, N-phenylalkyl maleimides and related compounds. Arzneimittelforschung 2005;55:123–132.
- Shults EE, Shakirov MM, Tolstikov GA, Kalinin VN, Schmidhammer G. Thebaine adducts with maleimides. Synthesis and transformations. Russian J Org Chem 2005;41:1132–1144.
- Coyle JD, Bryant LRB. Synthesis of diazaheterocycles with a bridgehead nitrogen by photocyclisation of N-substituted alicyclic imides. J Chem Soc Perkin Trans I 1983;2857–2865.
- Tetko IV, Tanchuk VY. Application of associative neural networks for prediction of lipophilicity in ALOGPS 2.1 program. J Chem Inf Comput Sci 2002;42:1136–1145.
- National Committee for Clinical Laboratory Standards. (2002). Reference method for broth dilution antifungal susceptibility testing of yeasts, 2nd edn. Approved standard M27-A2. Wayne, PA: NCCLS.
- Kippert F, Lloyd D. The aniline blue fluorochrome specifically stains the septum of both live and fixed Schizosaccharomyces pombe cells. FEMS Microbiol Lett 1995;132:215–219.
- Cabib E, Kang MS. Fungal 1,3-β-glucan synthase. Meth Enzymol 1987;138:637–642.
- Shedletzky E, Unger C, Delmer DP. A microtiter-based fluorescence assay for (1,3)-β-glucan synthases. Anal Biochem 1997;249:88–93.
- Munro CA, Schofield DA, Gooday GW, Gow NA. Regulation of chitin synthesis during dimorphic growth of Candida albicans. Microbiology (Reading, Engl) 1998;144:391–401.
- Bulawa CE, Slater M, Cabib E, Au-Young J, Sburlati A, Adair WL Jr, Robbins PW. The S. cerevisiae structural gene for chitin synthase is not required for chitin synthesis in vivo. Cell 1986;46:213–225.
- Gregory JD. The stability of N-ethylmaleimide and its reaction with sulfhydryl groups. J Am Chem Soc 1955;77:3922–3923.
- Niyaz Khan M. Kinetics and mechanism of the alkaline hydrolysis of maleimide. J Pharm Sci 1984;73:1767–1771.
- Georgopapadakou NH, Walsh TJ. Antifungal agents: Chemotherapeutic targets and immunologic strategies. Antimicrob Agents Chemother 1996;40:279–291.
- Bang KH, Lee DW, Park HM, Rhee YH. Inhibition of fungal cell wall synthesizing enzymes by trans-cinnamaldehyde. Biosci Biotechnol Biochem 2000;64:1061–1063.
- Frost DJ, Brandt K, Kaufmann T, Goldman R. Interaction of sulfhydryl reactive reagents with components involved in (1,3)-β-glucan synthesis from Candida albicans. Can J Microbiol 1995;41:692–698.
- Douglas CM. Fungal beta(1,3)-d-glucan synthesis. Med Mycol 2001;39 Suppl 1:55–66.
- Latgé JP. The cell wall: A carbohydrate armour for the fungal cell. Mol Microbiol 2007;66:279–290.
- López SN, Castelli MV, Zacchino SA, Domínguez JN, Lobo G, Charris-Charris J, Cortés JC, Ribas JC, Devia C, Rodríguez AM, Enriz RD. In vitro antifungal evaluation and structure-activity relationships of a new series of chalcone derivatives and synthetic analogues, with inhibitory properties against polymers of the fungal cell wall. Bioorg Med Chem 2001;9:1999–2013.
- Łącka I, Konieczny MT, Bułakowska A, Rzymowski T, Milewski, S. Antifungal action of the oxathiolone-fused chalcone derivative. Mycoses 2011 (in press). Available at: http://onlinelibrary.wiley.com/doi/10.1111/j.1439-0507.2010.01936.x/pdf
- Garcia-Effron G, Park S, Perlin DS. Correlating echinocandin MIC and kinetic inhibition of fks1 mutant glucan synthases for Candida albicans: Implications for interpretive breakpoints. Antimicrob Agents Chemother 2009;53:112–122.
- Friedmann E, Marrian DH, Simonreuss I. Antimitotic action of maleimide and related substances. Br J Pharmacol Chemother 1949;4:105–108.
- Matsui S, Yamazoe H, Watanabe I, Hayashi E, Konya K. [Studies on the experimental chemotherapy for dermatomycosis and candidiasis. IX. On the antifungal activity of various maleimide and succinimide compounds]. Yakugaku Zasshi 1984;104:1198–1206.