Abstract
The methanolic extract of Dictamnus dasycarpus root barks afforded one new glycosidic quinoline alkaloid, 3-[1β-hydroxy-2-(β-D-glucopyranosyloxy)-ethyl)-4-methoxy-2(1H)-quinolinone (1), together with nine known compounds, preskimmianine (2), 8-methoxy-N-methylflindersine (3), dictamine (4), γ-fagarine (5), halopine (6), skimmianine (7), dictangustine-A (8), iso-γ-fagarine (9), isomaculosidine (10). The isolated alkaloids significantly inhibited nitric oxide (NO) production in lipopolysaccharide (LPS)-stimulated BV2 cells. Among them, compounds 3 and 7 showed the most potent inhibitory activities on LPS-induced NO production.
Introduction
Nitric oxide (NO) is a gaseous signalling molecule that regulates various physiological and pathological responses depending on the relative concentration of NO and the surrounding milieu in which NO is produced. NO modulates central nervous system (CNS) functions by regulating blood flow in the brain and acts as a neurotransmitter in nonadrenergic noncholinergic (NANC) neuronCitation1,Citation2. However, the excessive NO, which is produced in response to inflammation by the activated microglia in CNS has been reported to be involved in a number of neurodegenerative diseases such as Alzheimer’s disease, Parkinson’s disease, and ischemiaCitation3–5. Hence, the inhibition of overproduction of NO has been suggested as an important therapeutic approach for prevention and/or treatment of neurodegenerative diseasesCitation6. In the course of searching for compounds modulating the overproduction of NO from natural sources using LPS-stimulated BV2 cells as an in vitro assay system, it was found that the methanolic extract of Dictamnus dasycarpus root barks (Rutaceae) significantly inhibited LPS-induced NO production. D. dasycarpus is widely distributed in Asia, and the root bark of this plant has been used for treatment of various ailments such as skin inflammation, eczema, rubella, scabies, acute rheumatoid arthritis, jaundice, cold, and headache in Korean traditional medicineCitation7. In the previous study in our lab, the neuroprotective activity of D. dasycarpus root bark was first reported through the isolation of neuroprotective limonoids, and identification of their action mechanisms against glutamate-induced neurotoxicity in primary cultures of rat cortical cellsCitation8,Citation9. Even though quinoline and furoquinoline alkaloids have been reported as constituents of D. dasycarpus root barkCitation10–12, there have been few reports related to neuroprotective effects of these alkaloids. In the present study, we report the isolation and structural elucidation of compounds 1–10 and their inhibitory activities on NO production in LPS-stimulated BV2 microglial cells.
Methods and materials
Plant material
Root barks of D. dasycarpus were purchased from Kyungdong Oriental Herbal Market, Seoul, Korea in April, 2006 and identified by Dr. Jong Hee Park, a professor of the College of Pharmacy, Pusan National University. A voucher specimen (SNU-0189) has been deposited in Herbarium of the Medicinal Plant Garden, College of Pharmacy, Seoul National University.
Extraction and isolation
Root barks of D. dasycarpus (21 kg) were extracted with 80% MeOH three times in an ultrasonic apparatus. Upon removal of the solvent under vacuum, the methanolic extract yielded 2.18 kg of material (10.4% by dry weight). The methanolic extract was suspended in H2O and partitioned successively with n-hexane, CHCl3, ethylacetate (EtOAc) and n-butanol. n-hexane soluble fraction was subjected to column chromatography (CC) over silica gel eluted with n-hexane/EtOAc gradient (100:0 to 0:100) to obtain sixteen fractions (hfr.1−hfr.16). Compound 4 (1.0 g) was purified by recrystallization with MeOH from hfr.7. The CHCl3 fraction (220.2 g) was subjected to CC over silica gel eluted with CHCl3/MeOH gradient (100:0 to 0:100) to obtain ten fractions (cfr.1−cfr.10). The cfr.3 was chromatographed on a silica gel column eluting with n-hexane-EtOAc mixture to afford five fractions (cfr.3-1−cfr.3-5). The cfr.3-4 was subjected to reverse phase (RP) CC with MeOH−H2O step gradient (40% MeOH to 100% MeOH) to yield seventeen subfractions (cfr.3-4-1−cfr.3-4-17). The cfr.3-4-7 was subjected to reversed-phase HPLC (YMC-Pack Pro C18, 10 × 250 mm) using CH3CN:H2O (60:40, 2 mL/min) as an eluent to give compounds 6 (11.9 mg) and 9 (12.5 mg). Compound 10 (90.0 mg) was purified by recrystallization with MeOH from cfr.3-4-12. The cfr.4 was chromatographed on a silica gel column eluting with n-hexane-EtOAc mixture to afford fifteen fractions (cfr.4-1−cfr.4-15). The cfr.4-8 was subjected to reverse phase (RP) CC with MeOH−H2O step gradient (30% MeOH to 100% MeOH) to yield eight subfractions (cfr.4-8-1−cfr.4-8-8). The cfr.4-8-2 was subjected to reversed-phase HPLC (YMC-Pack Pro C18, 10 × 250 mm) using CH3CN:H2O:MeOH (30:65:5, 2 mL/min) as an eluent to give compound 5 (5.8 mg). The cfr.4-8-4 was subjected to reversed-phase HPLC (YMC-Pack Pro C18, 10 × 250 mm) using CH3CN:H2O (35:65, 2 mL/min) as an eluent to give compound 7 (2.6 mg). The EtOAc fraction (36 g) was subjected to silica gel CC eluting with a gradient of CHCl3/MeOH (100:0 to 0:100) to yield eight fractions (efr.1−efr.8). The efr.4 was subjected to CC over Sephadex LH-20 using MeOH to yield six subfractions (efr.4-1−efr.4-6). The efr.4-2 was subjected to reversed-phase HPLC (YMC-Pack Pro C18, 10 × 250 mm) using CH3CN:H2O (47:53, 2 mL/min) as an eluent to give compound 2 (5.6 mg). The efr.4-6 was subjected to reversed-phase HPLC (YMC-Pack Pro C18, 10 × 250 mm) using CH3CN:H2O (40:60, 2 mL/min) as an eluent to give compound 1 (12.9 mg). The efr.5 was subjected to RP CC with MeOH−H2O step gradient (10% MeOH → 100% MeOH) to yield nineteen subfractions (efr.5-1−efr.5-19). The efr.5-5 was subjected to reversed-phase HPLC (YMC-Pack Pro C18, 10 × 250 mm) using CH3CN:H2O (33:67, 2 mL/min) as an eluent to give compound 8 (7.0 mg). The efr.5-6 was subjected to reversed-phase HPLC (YMC-Pack Pro C18, 10 × 250 mm) using CH3CN:H2O (47:53, 2 mL/min) as an eluent to give compound 3 (5.8 mg).
3-[1β-hydroxy-2-(β-D-glucopyranosyloxy)-ethyl)-4-methoxy-2(1H)-quinolinone
Yellowish gum; [α]25D +21.0° (c 0.1, MeOH); UV (MeOH) λmax (logϵ) 224 (3.19), 270 (2.43), 324 (2.36) nm; IR: νKBr max cm−1: 3355, 2254, 2129, 1652, 1025, 825, 765, 620; 1H-NMR (400 MHz, DMSO-d6): δ 3.18 (1H, m, H-2′), 3.35 (1H, m, H-3′), 3.39 (1H, dd, J = 5.6, 11.6 Hz, H-6′a), 3.28 (1H, m, H-4′), 3.77 (1H, m, H-5′), 3.62 (1H, brd, J = 11.6 Hz, H-6′b), 3.95 (3H, s, H-11), 3.97 (2H, m, H-13), 4.23 (1H, d, J = 7.8 Hz, H-1′), 5.08 (1H, t, J = 6.1 Hz, H-12), 7.23 (1H, t, J = 7.8 Hz, H-6), 7.31 (1H, d, J = 7.9 Hz, H-8), 7.53 (1H, t, J = 7.6 Hz, H-7), 7.73 (1H, d, J = 7.4 Hz, H-5); 13C-NMR (100 MHz, DMSO-d6): δ 60.9 (C-6′), 62.9 (C-11), 65.7 (C-12), 69.5 (C-4′), 72.3 (C-13), 73.5 (C-2′), 76.5 (C-5′), 76.8 (C-3′), 103.4 (C-1′), 115.4 (C-8), 115.9 (C-10), 121.4 (C-3), 122.0 (C-6), 123.0 (C-5), 130.8 (C-7), 138.2 (C-9), 162.4 (C-4), 163.3 (C-2); HRFABMS (positive) m/z 398.1456 [M+H]+ (Calcd for C18H23O9N, 398.1450).
Enzymatic Hydrolyses of compound 1
Compound 1 (4.0 mg) was hydrolyzed with 8.0 mg of β-glucosidase (Almonds Lot 1264252, Sigma-Aldrich) in 1.5 mL of H2O at 37 °C for 12 h. The hydrolyzed product of 1 (compound 1a) was obtained from the organic phase. The aqueous phase of hydrolyzate of 1 was dried using a stream of N2 and then subjected to CC over silica gel eluted with CHCl3−MeCN (3:1) to yield glucose (1.5 mg) from 1, [α]20D +48.2° (c 0.5, H2O).
Cell cultures
BV2 mouse microglia cell line originally developed by Dr. Bocchini at University of Perugia (Perugia, Italy) was generously provided by Dr. Sun-yeou Kim at Kyunghee University (Suwon, Korea). The cell line was maintained in DMEM containing 10% FBS with penicillin (100 IU/mL) and streptomycin (10 mg/mL) at 37°C in a humidified atmosphere of 95% air-5% CO2.
Determination of NO content
Compounds were dissolved in DMSO (final concentration in cultures, 0.1%). To remove any trace of phenol red, the cell cultures were washed and the medium was replaced with phenol red-free DMEM. Then BV2 microglia cells (2 × 105) were treated with samples for 1 h, before exposure to 100 ng/mL of LPS. After 24 h incubation, nitrite in culture media was measured to assess NO production in BV2 cells using Griess reagent. In 96-well plate, 100 µl of sample aliquots was mixed with 100 µl of Griess reagent (1% sulfanilamide, 0.1% naphthylethylenediamine dihydrochloride, 2% phosphoric acid) and incubated at room temperature for 15 min. The absorbance (abs) at 550nm was measured on a microplate reader. The concentration was determined using nitrite standard curve.
Estimation of cell viability
After 100 µl of sample aliquot was collected for Griess assay, MTT (0.2 mg/ml) was directly added to cultures, followed by incubation at 37°C for 2 h. The supernatant was then aspirated and 100 µl of DMSO was added to dissolve the formazan. After insoluble crystals were completely dissolved, absorbance at 540 nm was measured using a microplate reader. Data were expressed as percent cell viability relative to control cultures.
Statistical analysis
Data were evaluated for statistical significance using an analysis of variance (ANOVA) with a computerized statistical package. The data were considered to be statistically significant if the probability value was <0.05.
Results and discussion
The methanolic extract of D. dasycarpus root barks was suspended in water, and successively partitioned into n-Hexane, CHCl3, EtOAc, n-BuOH, and water fractions. Each fraction was evaluated for its inhibitory activity on NO production in LPS-stimulated BV2 cells (). The n-hexane and EtOAc fractions, which showed the potent inhibitory effects on NO production without cytotoxicity, were further subjected to repeated column chromatography to yield nine alkaloids (1–10) including one new glycosidic quinoline alkaloid (1).
Figure 1. The inhibitory effects of fractions of D. dasycarpus on LPS-induced NO production in BV2 cells. BV2 cells were pre-treated with each fraction for 1 h before exposure to LPS for 24 h. The concentration of nitrite in culture medium was measured as described in the methods. The values shown are mean ± SD of data from three independent experiments. Results differ significantly from the LPS-treated, *p < 0.001.
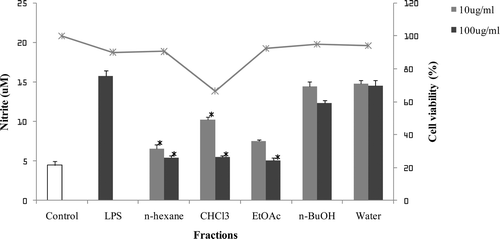
Compound 1 was isolated as a yellowish gum. The molecular formula was determined to be C18H23O9N from the HRFABMS at m/z 398.1456 [M+H]+ (calcd m/z 398.1450). The 1H NMR spectrum of 1 showed the presence of one methoxy group [δH 3.95 (3H, s)], and 1, 2-substituted aromatic ring [δH 7.23 (1H, t, J = 7.8 Hz), 7.31 (1H, d, J = 7.9 Hz), 7.53 (1H, t, J = 7.6 Hz), and 7.73 (1H, d, J = 7.4 Hz)]. In addition, the 13C spectrum had signals indicating one carbonyl group [δC 163.3 (C-2)], two quaternary carbon [δC 115.9 (C-10), and 138.2 (C-9)], together with signals characteristic for a glucose unit. The NMR spectra indicated that 1 has a 2(1H)-quinolinone alkaloid derivative by comparing its spectroscopic data with previously reported informationCitation13,Citation14. Hydrolysis of 1 with β-glucosidase yielded D-glucose ([α]20D +48.2°)Citation15. The glucose was determined to be at C-13 from the HMBC correlation between H-1′ and C-13 (). The 13C and DEPT spectra had signals indicating one oxymethine carbon [δC 65.7 (C-12)], and one oxymethylene carbon [δC 72.3 (C-13)]. HMBC correlations from oxymethine proton [δH 5.08 (1H, t, J = 6.1 Hz)] to C-3, C-2, C-4 and C-13 indicated that oxymethine was at C-3. Thus, compound 1 was determined as 3-[1β-hydroxy-2-(β-D-glucopyranosyloxy)-ethyl)-4-methoxy-2(1H)-quinolinone.
Nine known compounds were identified from their spectroscopic data by comparison with values reported in the literature as preskimmianine (2)Citation16, 8-methoxy-N-methylflindersine (3)Citation17, dictamine (4)Citation18,Citation19, γ-fagarine (5)Citation19, halopine (6)Citation20, skimmianine (7)Citation16, dictangustine-A (8)Citation21, iso-γ-fagarine (9)Citation21, isomaculosidine (10)Citation22. Among these compounds, compounds 3, 6 and 8 were isolated for the first time from this plant ().
Inhibitory effects of compounds 1−10 on LPS-induced NO production in BV2 microglia cells were evaluated using the Griess reaction described in Methods (). The treatment of LPS resulted in a significant increment of nitrite concentration in the medium as compared to control. The NO production induced by LPS was significantly inhibited by pretreatment of compounds 1–10. Among them, compound 1 which had glycoside moiety in its structure showed moderate activity compared to the other compounds at the concentration tested. However, compound 1a, the hydrolyzed product of compound 1 showed more potent inhibitory activity, which is comparable to compound 2. The inhibitory activity of 8-methoxy-N-methylflindersine (3) was most potent, which showed almost complete inhibition on LPS-induced NO production at the concentration of 12.25 µM. In 4-methoxyfuroquinoline alkaloids (compounds 4–7), the oxygenation at C-7 and C-8 resulted in a moderate increase in inhibitory activity on NO production, while weak activity was found in compounds 4 and 5, which had no substitution at C-7. Especially, the methoxy group at C-7 led to a significant increase in inhibitory potency: skimmianine (7), halopine (6), γ-fagarine (5) > dictamine (4). On the other hand, in 4-oxo-N-methylfuroquinoline alkaloids (compounds 8–10), the substitutions at C-6 or C-8 decreased the inhibitory activity at the concentration over 50 µM. To verify whether the reduced cell numbers caused by the cytotoxicity of these compounds resulted in decrease of NO production, cell viability was measured employing MTT assay. Cell viability was not significantly altered by the treatment of compounds at the concentrations used (data not shown).
Table 1. Inhibitory effects of compounds 1–10 isolated from D. dasycarpus on LPS-induced NO production in BV2 cells.
In the previous study, we reported that limonoids isolated from D. dasycarpus root barks significantly protected primary cultures of rat cortical cells against glutamate-induced neurotoxicityCitation8. In addition, it was revealed that neuroprotection by these limonoids was exhibited through inhibiting calcium influx, overproduction of cellular NO and reactive oxygen species, and to preserve mitochondrial membrane potential and activities of antioxidant enzymesCitation9. Limonoids and alkaloids isolated herein are thought to contribute to neuroprotective effects of D. dasycarpus, and these compounds are expected to be potential drug candidates for treatment of various neurodegenerative diseases.
Declaration of interest
This research was supported by a grant (2011K000290) from Brain Research Center of the 21st Century Frontier Research Program funded by the Ministry of Education, Science and Technology, the Republic of Korea.
References
- Hibbs JB Jr. Infection and nitric oxide. J Infect Dis 2002;185 Suppl 1:S9–17.
- Moncada S. Nitric oxide gas: mediator, modulator, and pathophysiologic entity. J Lab Clin Med 1992;120:187–191.
- Bolaños JP, Almeida A, Stewart V, Peuchen S, Land JM, Clark JB et al. Nitric oxide-mediated mitochondrial damage in the brain: mechanisms and implications for neurodegenerative diseases. J Neurochem 1997;68:2227–2240.
- Beckman JS, Crow JP. Pathological implications of nitric oxide, superoxide and peroxynitrite formation. Biochem Soc Trans 1993;21:330–334.
- Wu DC, Jackson-Lewis V, Vila M, Tieu K, Teismann P, Vadseth C et al. Blockade of microglial activation is neuroprotective in the 1-methyl-4-phenyl-1,2,3,6-tetrahydropyridine mouse model of Parkinson disease. J Neurosci 2002;22:1763–1771.
- Moncada S, Higgs EA. Molecular mechanisms and therapeutic strategies related to nitric oxide. Faseb J 1995;9:1319–1330.
- Bae. The Medicinal Plants of Korea. Kyo-Hak Publishing Co. Seoul, 2000, p. 286.
- Yoon JS, Sung SH, Kim YC. Neuroprotective limonoids of root bark of Dictamnus dasycarpus. J Nat Prod 2008;71:208–211.
- Yoon JS, Yang H, Kim SH, Sung SH, Kim YC. Limonoids from Dictamnus dasycarpus protect against glutamate-induced toxicity in primary cultured rat cortical cells. J Mol Neurosci 2010;42:9–16.
- StorerR, Young DW. Constituents of the root of Dictamnus albus L. Tetrahedron 1973;29:1217–1222.
- Zhao W, Wolfender JL, Hostettmann K, Xu R, Qin G. Antifungal alkaloids and limonoid derivatives from Dictamnus dasycarpus. Phytochemistry 1998;47:7–11.
- Yang JL, Liu, LL, Shi YP. Limonoids and quinoline alkaloids from Dictamnus dasycarpus. Planta Med 2011;77:271–276.
- Su YF, Luo Y, Guo CY, Guo DA. Two new quinoline glycoalkaloids from Echinops gmelinii. J Asian Nat Prod Res 2004;6:223–227.
- Brown NMD, Grundon MF, Harrison DM, Surgenor SA. Quinoline alkaloids-XXI. The 13C NMR spectra of hemiterpenoid quinoline alkaloids and related prenylquinolines. Tetrahedron 1980;36:3579–3584
- Lin S, Wang S, Liu M, Gan M, Li S, Yang Y et al. Glycosides from the stem bark of Fraxinus sieboldiana. J Nat Prod 2007;70:817–823.
- Paulini H, Waibel R, Schimmer O. Mutagenicity and structure-mutagenicity relationships of furoquinolines, naturally occurring alkaloids of the Rutaceae. Mutat Res 1989;227:179–186.
- Funayama S, Murata K, Nozoe S. Quinoline alkaloids from Orixa japonica Phytochemistry 1994;36:525–528.
- Halstead CW, Forster PI, Waterman PG. Alkaloids from the stem bark of an Australian population of Zanthoxylum ovalifolium. Nat Prod Res 2006;20:940–945.
- Mizuta M, Kanamori H. Mutagenic activities of dictamnine and gamma-fagarine from dictamni radicis cortex (Rutaceae). Mutat Res 1985;144:221–225.
- Wondimu A, DagneE, WatermanPG. Quinoline alkaloids from the leaves of Teclea simplicifolia. Phytochemistry; 1988;27:959.
- Wu T-S, Li C-Y, Leu Y-L, Hu C-Q. Limonoids and alkaloids of the root bark of Dictamnus angustifolius. Phytochemistry 1999;50:509–512.
- Ayafor JF, Sondengam BL, Ngadjui BT. Quinoline and indopyridoquinazoline alkaloids from Vepris louisii. Phytochemistry 1982;21:2733–2736.