Abstract
Two mononuclear copper(II) complexes, [Cu(C15H16NO2)2] (1) and [Cu(C6H9N2O4)2·3H2O] (2·3H2O), were synthesised and structurally characterised by single-crystal X-ray analysis. The copper(II) atom adopts a square-planar environment in complex 1, while the geometry in 2·3H2O could be described as the distorted square pyramidal. Complexes 1 and 2·3H2O were evaluated for their inhibitory activities against Helicobacter pylori (H. pylori) urease in vitro. They both were found to have strong inhibitory activities against H. pylori urease comparable to that of acetohydroxamic acid (AHA). A docking simulation was performed to position 2 into the H. pylori urease active site to determine the probable binding conformation.
Keywords::
Introduction
Urease, a nickel-dependent metalloenzyme, is found in bacteria, fungi, yeast and plantsCitation1,Citation2. Urease catalyses the hydrolysis of urea to form ammonia and carbamic acidCitation3,Citation4. The amino acid sequences of the active site are principally conserved in all known ureases, and the catalytic mechanism of their action is believed to be the same. Benini and co-workers proposed the most reliable enzymatic reaction mechanism on the basis of several crystal structures of complexes of Bacillus pasteurii ureaseCitation5. The active site of the native enzyme binds three water molecules and a hydroxide ion bridged between two nickel ions. H. pylori is a Gram-negative microaerophilic bacterium that infects up to 50% of the world’s human populationCitation6. The H. pylori urease could play an important role in the pathogenesis of gastric and peptic ulcerCitation7–9. In the past decades, some urease inhibitors have been reported such as phosphorodiamidates, hydroxamic acid derivatives and imidazolesCitation10. Some of urease inhibitors cannot be used in vivo because of their toxicity or instability. Thus, it is interesting to seek novel urease inhibitors with good bioavailability and low toxicity.
Recently, we have reported some silver(I) complexes showing potent inhibitory activities against ureaseCitation11. In this paper, we synthesised two new Cu(II) complexes with carboxylate, [Cu(C15H16NO2)2] (1) and [Cu(C6H9N2O4)2·3H2O] (2·3H2O), and investigated their inhibitory activities against H. pylori urease. Docking simulation using AUTODOCK 4.0 was performed to position complex 2 into the active site of H. pylori urease to determine the probable binding conformationsCitation12.
Experimental
Materials and methods
5-Methylpyrazine-2-carboxylic acid, 4-methylbenzoic acid and benzylamine were purchased from Aldrich and used without further purification. Elemental analyses for C, H and N were carried out on a Perkin–Elmer 2400 analyser. X-ray crystallography was carried out using a Bruker SMART APEX II CCD diffractometer. All chemicals and reagents used in this study were of analytical grade. Protease inhibitors (Complete mini EDTA-free) were purchased from Roche Diagnostics GmbH (Mannheim, Germany) and brucella broth was purchased from Becton-Dickinson (Cockeysville, MD). Horse serum was purchased from Hyclone (Utah, USA).
Synthesis of complex 1
A methanol solution (10 mL) of Cu(NO3)2•3H2O (121 mg, 0.5 mmol) was added to a solution of benzylamine (1.0 mmol) in methanol (5 mL), followed by an ammonia solution (10 mL, 13 mol/L) of 4-methylbenzoic acid (1.0 mmol). The mixture was stirred for 5 min at room temperature. After one week, blue crystals of 1 were formed. The crystals were filtered off and dried in a vacuum desiccator containing anhydrous CaCl2. Yield: 156 mg, 57% (based on Cu). Anal. Calc. for C30H32CuN2O4: C, 65.74; H, 5.88; N, 5.11. Found: C, 65.61; H, 5.97; N, 5.43%.
Synthesis of complex 2•3H2O
5-Methylpyrazine-2-carboxylic acid (2.0 mmol) was dissolved in ammonia solution (20 mL, 13 mol/L). The solution was stirred for 1 h and added to a stirred methanol solution (10 mL) of Cu(NO3)2•3H2O (1.0 mmol). The mixture was stirred for 10 min at room temperature and then filtered. The filtrate was kept in air for 1 week, and green crystals of 2•3H2O were formed. The crystals were isolated and dried in a vacuum desiccator containing anhydrous CaCl2. Yield: 184 mg, 45% (based on Cu). Anal. Calc. for 2•3H2O (C12H18CuN4O8): C, 35.17; H, 4.43; N, 13.67. Found: C, 35.00; H, 4.58; N, 13.51%.
Crystal structure determinations
X-ray crystallographic data were collected on a Bruker SMART Apex II CCD diffractometer using graphite-monochromated Mo-Kα (λ = 0.71073 Å) radiation. The collected data were reduced using the SAINT programCitation13, and empirical absorption corrections were performed using the SADABS programCitation14. The structures were solved by direct methods and refined against F2 by full-matrix least-squares methods using the SHELXTL version 5.1. All of the non-hydrogen atoms were refined anisotropically. All other hydrogen atoms were placed in geometrically ideal positions and constrained to ride on their parent atoms.
Measurement of inhibitory activity against H. pylori urease
H. pylori (ATCC 43504; American Type Culture Collection, Manassas, VA) was grown on Columbia Agar supplemented with 7% sheep blood and cultured for 3 days at 37°C under microaerobic conditions (5% O2, 10% CO2 and 85% N2), as previously describedCitation15.
The method of preparation of H. pylori urease was followed by Mao16. Briefly, 50 mL broth cultures (2.0 × 108 CFU/mL) were centrifuged (5000g, 4°C) to collect the bacteria, and after washing twice with phosphate-buffered saline (pH 7.4), the H. pylori precipitation was stored at −80°C. H. pylori was returned to room temperature, and after the addition of 3 mL of distilled water and protease inhibitors, sonication was performed for 60 s. Following centrifugation (15,000g, 4°C), the supernatant was desalted through SephadexG-25 column (PD-10 columns, Amersham–Pharmacia Biotech, Uppsala, Sweden). The resultant crude urease solution was added to an equal volume of glycerol and stored at 4°C until use in the experiment. The mixture, containing 25 μL (4U) of H. pylori urease (100 mM HEPES, pH 6.8) and 25 μL of the test compounds of various concentrations, was preincubated for 3 h at room temperature in a 96-well assay plate. After preincubation, 0.2 mL of 100 mM HEPES (pH 6.8) buffer containing 500 mM urea and 0.002% phenol red were added and incubated at 37°C. Urease activity was determined by measuring ammonia production using the indophenol method as described by WeatherburnCitation17.
Docking simulations
Molecular docking of the complex into the three-dimensional X-ray structure of H. pylori urease structure (entry 1E9Z in the Protein Data Bank) was carried out using the AUTODOCK 4.0 software as implemented through the graphical user interface AutoDockTools (ADT 1.4.6)Citation18. The procedure of the molecular docking was determined as described in our groupCitation17,Citation19.
Results and discussion
Crystal structure description
The crystal structures of complexes 1 and 2•3H2O consist of discrete [Cu(4-methylbenzoate)2(benzylamine)2] and [Cu(5-methylpyrazine-2-carboxylate)2-(3H2O)] moieties, respectively. Complex 2•3H2O involves a lattice molecule of water. X-ray single crystal diffraction reveals that both 1 and 2•3H2O crystallise in triclinic space group P-1. As shown in , complex 1 has a four-coordinate Cu(II) ion in a square-planar geometry defined by two carboxylate O atoms from two 4-methylbenzoate groups and two N atoms from two benzylamine molecules. The bond distances of Cu–O and Cu–N are 2.001(2) and 2.005(2) Å, respectively. Analogous Cu(II) species were previously reported in the literatures 20–22. Interestingly, there are two intermolecular N−H···O hydrogen-bonding interactions between the coordinated benzylamine molecule and the uncoordinated oxygen atom of carboxylate (), which associate the adjacent CuO2N2 chromophores to give a one-dimensional (1D) structure ().
Table 1. Hydrogen bonds arrangement in 1 and 2•3H2O (Å, °).
Figure 1. The structure of complex 1. (a) An ORTEP diagram showing the structure of 1 with selected atom-labelling and thermal ellipsoids plotted at 30% probability. (b) One-dimensional (1D) structure of 1 formed by intermolecular N−H O hydrogen bonds.
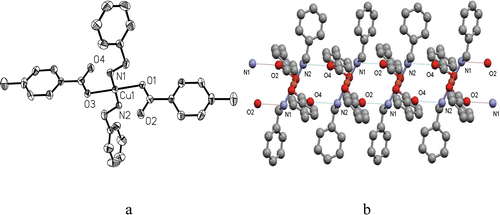
The crystal structure of complex 2·3H2O has been reported in previous workCitation23. The crystallographic data for complexes and the selected bond lengths and angles are provided in the supplementary information.
Inhibitory bioactivity against urease
The abilities of the ligands, Cu2+ and the complexes in inhibiting urease were studied by the IC50 values of the material tested against H. pylori urease. From , benzylamine, 4-methylbenzoic acid and 5-methylpyrazine-2-carboxylic acid as enzyme inhibitors had no ability to inhibit urease (IC50 > 100 μM). This indicated the ligands had less influence on the activity of H. pylori urease. Under the same condition, both the two Cu(II) complexes had much stronger urease inhibitory activities with the IC50 values of 3.23 µM for 1 and 1.05 µM for 2·3H2O, respectively, compared with that of the standard inhibitor acetohydroxamic acid which had IC50 values of 42.47 µM, as shown in . The two copper(II) complexes exhibited stronger ability to inhibit urease probably due to the strong Lewis acid properties of copper metal ions. This showed that inhibitory efficiency of the complex toward urease may be influenced not only by the transition metal ion but also by ligands. Complex 2 3H2O exhibits stronger ability to inhibit urease than complex 1 probably because of copper metal ion being in the square-pyramidal coordination and simple molecular structure. The Cu(II) ion showed weaker inhibitory activity against urease than the complexes because the ligands probably strengthened inhibitory activity of the complexes. At the same time, the IC50 of the Cu(II) ion was higher than that of the literatureCitation24,Citation25.
Table 2. Inhibition of H. pylori urease by 1, 2, ligands and Cu(II) ion.
The results are agree with the data of the Cu(II) complex and AHA reported previouslyCitation26, but are much stronger than the activity of the silver(I) carboxylate complexCitation11.
Molecular docking study
In the X-ray structure available for the native H. pylori urease (entry 1E9Z in the Protein Data Bank), the two nickels were coordinated by His136, His138, Kcx219, His248, His274, Asp362 and water moleculesCitation27, while in the AHA-inhibited H. pylori urease (entry 1E9Y in the Protein Data Bank), these water molecules were replaced by AHA28.
The binding mode of diamidophosphate (DAP), the hydrolytic product of phenylphosphorodiamidate (PPD), a known efficient urease inhibitor, in the enzyme active site was modeledCitation7,Citation16. In the binding model, the oxygen of DAP formed a hydrogen bond with the amino hydrogen of His221 (length of the hydrogen bond: His221N–H…ODAP = 2.244 Å; angle of the hydrogen bond: His221N–H…ODAP = 134.574°). The benzene ring of DAP probably forms hydrophobic interactions with Ala169 and Ala365.
The binding model of 2 with H. pylori urease is depicted in . All the amino acid residues which had interactions with H. pylori urease are shown. In the binding model of 2 with urease, one hydrogen bond was formed. The nitrogen atom of 2 formed hydrogen bond with the amino hydrogen of His221 as shown in (length of the hydrogen bond: His221 N–H…Ncomplex2 = 2.205 Å;angle of the hydrogen bond: His221 N–H…Ncomplex2 = 135.264 °) similar to DAP, but different to the Schiff-base copper(II) complex which was previously reported19. Moreover, 2 might form hydrophobic interaction with Ala 365 of H. pylori urease. Its urease inhibitory property may be attributed to the above hydrogen bond and hydrophobic interactions formed between the copper complexes and H. pylori urease.
Conclusion
The present report describes the syntheses, X-ray crystal structures and inhibitory enzyme activity of the Cu(II) complexes. The complexes exhibited ideal ability to inhibit urease, although their ligands had no ability to inhibit urease (IC50 > 100 μM). Docking simulation was performed to position complex 2 into the H. pylori urease active site to determine the probable binding conformations and the results indicated that the complex was a potent inhibitor of H. pylori urease. Detailed investigations are continuing to study the mechanisms of the inhibitory activity reported here.
Supporting material
Crystallographic data in CIF format for the structural analysis have been deposited with the Cambridge Crystallographic Data Centre No. 800150 for complex [Cu(C15H16NO2)2] and No. 800151 for complex [Cu(C6H9N2O4)2·3H2O]. Copies of this information can be obtained free of charge from The Director, CCDC, 12 Union Road, Cambridge CB2 1EZ, UK (Fax: +44 1223 336 033; Email: [email protected]. ac.uk or www: http://www.ccdc.cam.ac.uk).
Declaration of interest
This work was financed by grant from National Natural Science Foundation of China (50978208), Hubei National Science Foundation (2009CDA022), Education Office of Hubei Province (D20091704 & Q20101610) and Special Fund for Basic Scientific Research of Central Colleges, South-Central University for Nationalities (Project No. CZY10007).
Supplementary Material
Download PDF (40.5 KB)References
- Bacanamwo M, Witte CP, Lubbers MW, Polacco JC. Activation of the urease of Schizosaccharomyces pombe by the UreF accessory protein from soybean. Mol Genet Genomics 2002;268:525–534.
- Krajewska B., Ureases I. Functional, catalytic and kinetic properties: a review. J Mol Catal B Enzym 2009;59: 9–21.
- Dixon NE, Gazzola TC, blakeley RL, Zermer B. Letter: Jack bean urease (EC 3.5.1.5). A metalloenzyme. A simple biological role for nickel? J Am Chem Soc 1975;97:4131–4133.
- Karplus PA, Pearson MA, Hausinger RP. 70 Years of crystalline urease: what have we learned? Acc Chem Res 1997;30: 330–337.
- Benini S, Rypniewski WR, Wilson KS, Miletti S, Ciurli S, Mangani S. A new proposal for urease mechanism based on the crystal structures of the native and inhibited enzyme from Bacillus pasteurii: why urea hydrolysis costs two nickels. Structure 1999;7:205–216.
- Covacci A, Telford JL, Del Giudice G, Parsonnet J, Rappuoli R. Helicobacter pylori virulence and genetic geography. Science 1999;284:1328–1333.
- Mobley HLT, Mendz GL, Hazell SL. (2001). Helicobacter pylori: physiology and genetics. Washington, USA: ASM Press.
- Crespo A, Suh B. Helicobacter pylori infection: epidemiology, pathophysiology, and therapy. Arch Pharm Res 2001;24:485–498.
- Montecucco C, Rappuoli R. Living dangerously: how Helicobacter pylori survives in the human stomach. Nat Rev Mol Cell Biol 2001;2:457–466.
- Follmer C. Ureases as a target for the treatment of gastric and urinary infections. J Clin Pathol 2010;63:424–430.
- Cheng K, Zhu HL, Li YG. Syntheses and characterizations of two novel silver polymers from assembly 5-bromosalicylic acid and 2-aminobenzoic acid, with high inhibitory activities against jack bean urease. Z Anorg Allg Chem 2006;632:2326–2330.
- Morris GM, Goodsell DS, Halliday RS, Huey R, Hart WE, Belew RK, Olson AJ. Automated docking using a Lamarckian genetic algorithm and an empirical binding free energy function. J Comput Chem 1998;19:1639–1662.
- Bruker. (1998). SMART and SAINT (Version 5.0). Data collection and processing software for the SMART system. Madison, Wisconsin, USA: Bruker AXS Inc.
- Sheldrick GM. (1996). SADABS. Program for empirical. Absorption correction of area detector data. Germany: University of Göttingen.
- Li HQ, Xu C, Li HS, Xiao ZP, Shi L, Zhu HL. Metronidazole-flavonoid derivatives as anti-Helicobacter pylori agents with potent inhibitory activity against HPE-induced interleukin-8 production by AGS cells. Chemmedchem 2007;2:1361–1369.
- Mao WJ, Lv PC, Shi L, Li HQ, Zhu HL. Synthesis, molecular docking and biological evaluation of metronidazole derivatives as potent Helicobacter pylori urease inhibitors. Bioorg Med Chem 2009;17:7531–7536.
- Weatherburn MW. Phenol hypochlorite reaction lor determination of ammonia. Anal Chem 1967;39:971–974.
- Huey R, Morris GM, Olson AJ, Goodsell DS. A semiempirical free energy force field with charge-based desolvation. J Comput Chem 2007;28:1145–1152.
- Cui YM, Li YG, Cai YJ, Chen W, Zhu HL. Synthesis, molecular docking, and activity of Schiff-base copper(II) complex with N-n-butylsalicylaldiminate as Helicobacter pylori urease inhibitor. J Coord Chem 2011;64:610–616.
- Mak TCW, Yip WH, Smith G, O’Reilly EJ, Kennard CHL. Metal-phenoxyalkanoic acid interactions. Part 20. The crystal structures of diaquabis(2-carbamoylphenoxyacetato)copper(II) and tetraaquabis(2-carbamoylphenoxyacetato)nickel(II). Inorg Chim Acta 1986;112:53–57.
- Humphrey SM, Angliss TJP, Aransay M, Cave D, Gerrard LA, Weldon GF, Wood PT. Bimetallic metal-organic frameworks containing the [M(2,X-pdc)2-2] (M = Cu, Pd, Pt; x = 4, 5) building block − synthesis, structure, and magnetic properties†. Z Anorg Allg Chem 2007; 633: 2342–2353.
- Hao HQ, Zhang H. Diaquabis[(pyrimidin-2-ylsulfanyl)acetato]copper(II) dihydrate. Acta Crystallogr Sect 2006; E62: m30.
- Ptasiewicz-Bąk H, Leciejewicz J. The crystal structure of monoaquabis(trans-5--methylpyrazine-2-carboxylato-N,O)copper(II) Trihydrate. Polish J Chem 2000; 74: 877–883.
- Krajewska B, Zaborska W. Jack bean urease: the effect of active-site binding inhibitors on the reactivity of enzyme thiol groups. Bioorg Chem 2007;35:355–365.
- Krajewska B. Mono- (Ag, Hg) and di- (Cu, Hg) valent metal ions effects on the activity of jack bean urease. Probing the modes of metal binding to the enzyme. J Enzyme Inhib Med Chem 2008;23:535–542.
- Cheng K, You ZL, Zhu HL. New method for the synthesis of a mononucleating cyclic peptide ligand, crystal structures of its Ni, Zn, Cu, and Co complexes, and their inhibitory bioactivity against urease. Aust J Chem 2007;60:375–379.
- Ha NC, Oh ST, Sung JY, Cha KA, Lee MH, Oh BH. Supramolecular assembly and acid resistance of Helicobacter pylori urease. Nat Struct Biol 2001;8:505–509.
- Xiao ZP, Ma TW, Fu WC, Peng XC, Zhang AH, Zhu HL. The synthesis, structure and activity evaluation of pyrogallol and catechol derivatives as Helicobacter pylori urease inhibitors. Eur J Med Chem 2010;45:5064–5070.