Abstract
Terminalia chebula fruit extracts were prepared sequentially with hexane, ethyl acetate, methanol and methanol–water (70:30) and tested for their α-glucosidase inhibitory and antioxidant potential. The study resulted in the formulation of an extract with high α-glucosidase inhibitory potential (IC50 0.19 ± 0.03 µg mL−1) enriched with hydrolysable tannins. Also, each of the extract was chemically characterized by reversed-phase high-performance liquid chromatography on the basis of their marker compounds chebulagic acid, chebulinic acid and corilagin in order to give explanation to the significant activity shown by the extracts. The antioxidant potential of the highly active extract was evaluated in the cellular level also using superoxide dismutase, glutathione S-transferase and induced oxidative stress assays. The results indicated the possibility of using the extract as a nutraceutical health supplement in the management of type 2 diabetes.
Introduction
Diabetes mellitus is a major chronic disease caused by the improper balance of glucose homeostasis. An abnormal postprandial increase in blood glucose level has been linked to the onset of type 2 diabetes and associated with oxidative dysfunction and failure of various organs. Hydrolysis of dietary carbohydrate such as starch is a major source of glucose in blood. This hydrolysis is carried out by a group of enzymes that include pancreatic α- amylase and intestinal α-glucosidase. It is believed that inhibition of this enzyme activity should be an important strategy for management of type 2 diabetes, wherein α-glucosidase inhibitors could retard the rapid utilization of dietary carbohydrate and suppress postprandial hyperglycemia. Currently, therapeutic drugs, such as acarbose are shown to effectively reduce intestinal absorption of sugars in humanCitation1. Oxidative stress due to hyperglycemia and hyperlipidemia also play an important role in complications and other adverse effect due to diabetes.
Herbal medicine is a major component in all indigenous people’s traditional medicine and a common element in ayurvedic, homeopathic and naturopathic medicine. World health organization notes that out of 119 plant-derived pharmaceutical medicines, about 74% are used in modern medicine. Medicinal plants have the advantage of having little or no side effects. Long before, till the use of insulin became common, indigenous remedies were used for the treatment of diabetes mellitus that made scientist look towards hypoglycemic agents of plant origin.
Free radicals have been implicated in the causation of several diseases such as liver cirrhosis, atherosclerosis, cancer, diabetes and compounds that can scavenge free radicals have great potential in ameliorating these disease processesCitation2. Antioxidants thus play an important role to protect the human body against damage by reactive oxygen species (ROS)Citation3. Increased oxidative stress has been postulated in the diabetic state. Oxygen free radical activity can initiate peroxidation of lipids, which in turn stimulates glycation of protein, inactivation of enzymes and alterations in the structure and function of collagen, basement and other membranes and plays a role in the long-term complications of diabetesCitation4. Oxidative stress in diabetes coexists with a reduction in the antioxidant statusCitation5, which can increase the deleterious effects of free radicals. Supplementation with non-toxic antioxidants may have a chemoprotective role in the diabetesCitation6.
Terminalia chebula belongs to the family Combretaceae and is found throughout India. Fruits of T. chebula contain 25–30% of hydrolysable tanninsCitation7. The chief constituents are chebulic acid, chebulagic acid, corilagin and gallic acidCitation8,Citation9. T. chebula has been reported to exhibit a variety of biological activity, including anticancerCitation10, antidiabeticCitation11, antibacterialCitation12, antifungalCitation13, antiviralCitation14,Citation15, antimutagenicCitation16 activities, etc. T. chebula fruits are the part of well-known traditional formulation Triphala used in the treatment of many chronic diseases such as ageing, heart ailments and hepatic diseasesCitation11,Citation17. Most of the earlier studies regarding the biological activities of T. chebula fruits were carried out using crude extracts. In the present study, we have sequentially extracted the fruit powder with different solvents in the increasing order of polarity and studied how these extracts affected the α-glucosidase inhibitory and antioxidant activity.
Materials and methods
Plant material
T. chebula fruits were collected from forest near Vithura, Trivandrum, Kerala. The samples were identified by Dr. A.G. Pandurangan in tropical botanical garden and research institute (Palode, Trivandrum, Kerala, India) and a voucher specimen (No: 76487/AGP/09) was kept in herbarium for future reference. The samples were powdered to an average particle size of 0.5 mm and stored at 4°C in air tight containers.
Chemicals and reagents
The compounds 2,2′-azinobis (3-ethylbenzothiazoline-6-sulphonic acid) diammonium salt (ABTS), 1,1-diphenyl-2-picrylhydrazyl (DPPH) as free radical, α-glucosidase (from Saccharomyces cerevisiae), p-nitrophenyl α-d-glucopyranoside (PNPG), gallic acid, 6-hydroxy-2,5,7,8-tetramethylchroman-2-carboxylic Acid (Trolox), phenazine methosulphate (PMS), b-nicotinamide adenine dinucleotide (NADH), 20,70-dichlorodihydrofluoresceindiacetate (DCFH-DA), catechin hydrate, gallic acid were supplied from Sigma–Aldrich (St. Louis, MO, USA). Acarbose was obtained from SERVA Electrophoresis GmbH (Mannheim, Germany). Glutathione S-Transferase (GST) Assay Kit was procured from Cayman chemical company, Ann Arbor, USA. Folin–Ciocalteu reagent, potassium persulphate, sodium phosphate, and sodium carbonate, ethyl alcohol, hexane and chloroform were analytical grade and procured from Merck (Darmstadt, Germany). Reference compounds chebulagic acid, chebulinic acid and corilagin were purchased from Natural Remedies (Bangalore, India). All other reagents and solvents used were of Analytical and AR grade.
Extraction of plant material
Powdered T. chebula fruits (500 g) were extracted successively with 1 Litre each of hexane, ethyl acetate, methanol and methanol–water (70:30) in the increasing order of polarity. The hexane (TC-1), ethyl acetate (TC-2) and methanol (TC-3) extracts were then filtered and concentrated under reduced pressure below 45°C. The methanol–water extract (TC-4) was lyophilised and kept at −20°C in sealed bottles till analysed.
Total phenolics assay
The total phenolic content of the extracts were determined by the Folin–Ciocalteu method with gallic acid as standardCitation18. Stock solution (1 mg mL−1) was diluted with methanol to have sample solutions of varying dilutions (2–10 μg mL−1). Sample solutions (0.5 mL) were mixed with 0.5 mL of Folin–Ciocalteu reagent and 1 mL of sodium carbonate (20% w/v), adjusting with water so as to have a total volume of 5 mL, the contents were mixed and allowed to stand for 30 min. Absorption at 760 nm was measured in a Shimadzu 1601 UV–VIS Spectrophotometer. The total phenolic content was expressed as gallic acid equivalents (GAE) in milligrams per gram of sample.
α-Glucosidase inhibition assay
The enzyme reactionCitation19 was performed using PNPG as a substrate in phosphate salt buffer. The α-glucosidase inhibiting effect of the extractant was assayed according to the following procedure. Different volume of the extractant (0.1 mg mL−1) was added into the phosphate salt buffer (pH 6.8), after adding 20 µL α-glucosidase (1 U mL−1), the mixture was incubated at 37°C for 5 min. Then 200 µL PNPG was added as a substrate into the buffer, and after 20-min reaction, 200 µL sodium carbonate was added to terminate the reaction. Enzymatic activity was quantized by measuring the p-nitrophenol released from PNPG at 405 nm wavelength using multiplate reader (Synergy 4, BioTek Instruments Inc., Vermont, USA). Acarbose was taken as reference compound.
Evaluation of antioxidant activity
DPPH radical scavenging activity
DPPH is a free radical compound and has been widely used to test the free radical scavenging ability of samples. The hydrogen atom or electron donation ability of the extracts was measured from the bleaching of purple coloured methanol solution of 2,2-diphenyl-1-picrylhydrazylCitation20. Methanolic stock solutions (5.0 mg mL−1) of extracts were diluted to final concentrations ranging from 2 to 10 μg mL−1. Various concentrations (100 µL) of the extracts were mixed with 2.9 mL of a 0.3 mM DPPH solution and allowed to react at room temperature in dark. After 30 min, the absorbance values were measured at 517 nm. Gallic acid was used as positive control and the percentage radical scavenging activity is calculated using the following equation.
where A0 is the absorbance of the control and A1 is the absorbance of the sample.
Experiments were carried out in triplicate.
ABTS radical cation decolourisation assay
An improved ABTS decolorization assayCitation21 in which ABTS+• were generated by the oxidation of ABTS with potassium persulphate. The ABTS+• solution was diluted to an absorbance of 0.7 ± 0.05 at 734 nm (Shimadzu UV–VIS Spectrophotometer, Model 2100). Absorbance was measured 7 min after the initial mixing of different concentrations of the methanolic bark extracts with 1 mL of ABTS+• solution. Trolox was used as a reference standard.
HPLC estimation of major phenolics
Stock solutions (5 mg mL−1) of TC-2, TC-3, and TC-4 were prepared and the solutions were filtered through a 0.45-µm filter. The stock solutions were then diluted to a concentration of 1 µg µL−1 and 20 µL of the solutions were injected into the high-performance liquid chromatography (HPLC) column. Reference compounds chebulagic acid, chebulinic acid, corilagin and gallic acid in methanol were used to calibrate standard curves and retention times.
Cell culture and treatment
HepG2 cells (hepatocellular carcinoma cell line) obtained from the National Centre for Cell Science (Pune, India) were maintained in culture in 75 cm2 polystyrene flasks (Falcon) with DMEM containing 10% fetal bovine serum and 100 μg mL−1 penicillin–streptomycin at 37°C in a humidified atmosphere of 95% air and 5% CO2. Cultures (2.5 × 106 cells per well) were grown for 3 days in complete DMEM until they reached the post-confluence stage. Cells were incubated with different concentrations of TC-2 extract (10, 50, 100 and 200 µg mL−1) medium for 24 h.
Superoxide radical scavenging and SOD activity
Superoxide radicals are generated non-enzymatically in PMS–NADH systems by the oxidation of NADH and assayed by the reduction of NBTCitation22. The superoxide radicals were generated in 1 mL of Tris–HCl buffer (16 mM, pH 8.0) containing NBT (50 μM) and NADH (78 μM), PMS (10 μM) was added to the mixture and incubated at 25°C for 5 min, and the absorbance was measured at 560 nm against blank samples.
To test whether TC-2 extract can alter the activity of intracellular superoxide dismutases (SODs), SOD activity in the cell lysates was measured using the nitroblue tetrazolium assay (NBT assay, which measures the conversion of NBT to blue formazanCitation23). Superoxide radicals were generated intracellularly by phorbol myristate acetate (PMA). The formazan formed after incubation of the cells with 0.5 mg mL−1 NBT was solubilized by adding 300 mL of KOH/dimethylsulphoxide (DMSO) per well (mix 1 part of 2 M KOH with 1.167 parts of DMSO just before use) and absorbance was measured at 620 nm using a microplate reader (Synergy 4, BioTek Instruments Inc., Vermont, USA).
Assay of glutathione S-transferase activity
GST activity of the samples was measured using the Cayman Chemical GST Assay Kit (Cayman chemical company, Ann Arbor, USA). Total GST activity (cytosolic and microsomal) was measured by the conjugation of 1-chloro-2,4-dinitrobenzene with reduced glutathione. The conjugation is accompanied by an increase in absorbance at 340 nm. The rate of increase is directly proportional to the GST activity in the sampleCitation24.
Evaluation of induced oxidative stress inhibition
Cell culture and treatment
C2C12 cells purchased from the National Centre for Cell Science (Pune, India) were cultured in Dulbecco’s modified Eagle’s medium supplemented with 10% fetal calf serum, 100 U mL−1 penicillin and 100 mg mL−1 streptomycin. Cultures were maintained at 37°C in a 5% carbon dioxide incubator. When the cells were about to cover 80% of the flask area, they were detached and seeded on 24-well plates. After attaining, 70–80% confluency, the cells were rinsed twice with phosphate-buffered saline (PBS) and changed with medium containing extracts at different concentrations. After 24 h incubation, the cells were washed twice with PBS and 50 mM H2O2 was maintained in individual wells for 1 h at 37°C. These cells were detached by trypsin to assay by flow cytometry.
Evaluation of oxidative stress inhibition
Cytoprotective effect against the oxidative stress-induced by H2O2 was measured by determining the intracellular content of ROS. Intracellular ROS levels were measured employing DCFH-DACitation25. Non-fluorescent DCFH-DA, after cellular incorporation, is hydrolysed by cellular esterase to DCFH, which in the presence of strong oxidants such as H2O2 and others, is oxidised to fluorescent 2',7'-dichlorofluorescein which were analysed with Fluorescence-activated cell sorting (FACS) Aria II (BD Bioscience, San Jose, CA, USA). C2C12 cells pre-treated with TC-2 extract were incubated with DCFH-DA at 37°C for 20 min and then analysed by the FACS Aria II.
Statistical analysis
The results were analysed using Microsoft excel 2007 and Origin Pro 8 softwares. All the data are expressed as mean ± SD (n = 3). Student’s t-test was used to compare means, and values were considered significant at p < 0.05.
Results and discussion
Total phenolic content of Terminalia chebula extracts
The phenolic content was found to be maximum (718 ± 24.5 mg g−1 GAE) in TC-2 followed by TC-3 (623.8 ± 12 mg g−1 GAE) (). But the hexane extract TC-1 was found to be devoid of phenolic compounds. Therefore, it was exempted from further studies.
Table 1. TPC content and HPLC analysis of individual phenolic compounds in ethyl acetate, methanol and 70% methanol–water extracts of T. chebula.
Inhibitory effect on α-glucosidase
The hydrolysable tannins rich extracts of T. chebula were tested for their ability to inhibit α-glucosidase. It was found that all the three extracts showed strong inhibition against α-glucosidase. A low IC50 translates to a stronger α-glucosidase inhibition. The IC50 values of different extracts were shown in . α-Glucosidase inhibitory potential of extracts varies as TC-2>TC-3>TC-4 (). As compared to the standard acarbose (IC50 38.5 ± 3.2 µg mL−1), all the extracts of T. chebula showed significantly higher inhibition against α-glucosidase. However, among the extracts, TC-2 showed highest inhibitory potential against α-glucosidase (IC50 0.19 ± 0.03 µg mL−1) followed by TC-3 with an IC50 value of 0.28 ± 0.02 µg mL−1. This may be attributed to the high content of chebulagic and chebulinic acids. HPLC analysis showed that the TC-2 extract contained the highest quantity of chebulagic (242.8 ± 6.03 mg g−1) and chebulinic acid (358.6 ± 8.6 mg g−1) followed by the TC-3 extract (chebulagic acid – 159.0 ± 5.8 mg g−1 and chebulinic acid – 233.9 ± 7.3 mg g−1). The α-glucosidase inhibitory potential of hydrolysable tannins is directly proportional to the number of galloyl and chebuloyl groupsCitation26. Chebulinic acid contains three galloyl and one chebuloyl groups whereas chebulagic acid contains a galloyl, a chebuloyl and a hexahydroxydiphenoyl (HHDP) group. The HHDP unit have only a weak influence on the α-glucosidase inhibition in the case of monomeric hydrolysable tanninsCitation27. Therefore, it can be inferred that as the amount of chebulagic and chebulinic acid increases the α-glucosidase inhibitory potential also increases. This is clearly evident from the IC50 values obtained for different extracts (). A recent study on α-glucosidase inhibitory potential of Terminalia speciesCitation28 showed that T. chebula ethanol extract has an IC50 value of 6.32 ± 0.40 µg mL−1. Also, the α-glucosidase inhibitory power of chebulagic acid, one of the active principles in T. chebula, was studied by Gao et alCitation29 and the IC50 value is given as 47.7 µg mL−1.
Table 2. α-glucosidase inhibitory effect and antioxidant activity of different extracts of T. chebula on in vitro models, as expressed (µg mL−1) by inhibitory concentration (IC50). Each value represents the mean ± SD of three replicates.
Figure 1. Dose–response curve for the inhibitory effect of T. chebula extracts on the activity of α-glucosidase.
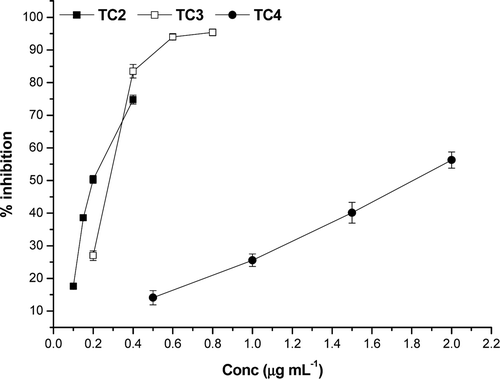
Normally, the inhibition of α-glucosidase enzyme reduces starch digestion and absorption, consequently lowering a postprandial hyperglycaemic response. This hypoglycemic effect is an established and effective target for type 2 diabetes prevention and treatmentCitation30. All the extracts studied here showed high level of α-glucosidase inhibition in comparison with the synthetic inhibitor, acarbose. But, these synthetic inhibitors have some side effects also. Therefore, the possibility of preventing the onset of diabetes using dietary supplements and herbal medicines has attracted increasing attention. Thus, making these extracts as suitable candidates as nutraceutical supplements for diabetes prevention.
DPPH radical scavenging activity
T. chebula extracts were subjected to the DPPH assay along with reference standards gallic acid and BHT. The DPPH scavenging activity with concentration is depicted in . The decrease in absorbance of the DPPH radical at 517 nm caused by antioxidant was due to the scavenging of the radical by hydrogen donation. A lower value of inhibitory concentration indicates a higher antioxidant activity. The samples showed high scavenging activity against DPPH. The lowest IC50 was obtained in the case of TC-2 (2.01 ± 0.08 µg mL−1) and it may be attributed to its high phenolic content (718 ± 24.5 mg g−1 GAE). The reference compounds gallic acid and BHT has IC50 values 1.4 and 7 µg mL−1, respectively. Linear correlation was found between the total phenolic content and DPPH IC50 of T. chebula extracts. IC50 values of extracts are given in .
ABTS radical cation decolourization assay
This method is an excellent tool for determining the antioxidant activity of hydrogen donating antioxidants and of chain breaking antioxidants. The T. chebula extracts showed good scavenging activity against ABTS•+. The IC50 values of the extracts were in the concentration range 2–5 µg mL−1 (). The values were compared with that of the reference compounds trolox (an ideal ABTS scavenger) (IC50 – 2.2 µg mL−1) and ascorbic acid (IC50 – 2.78 µg mL−1) and found to statistically significant (p ≤ 0.05) (). The higher activity of extracts against ABTS•+ can be due to the presence rich tannin content which is evident from the HPLC analysis of the extracts.
Superoxide radical anion scavenging assay
All the extracts showed scavenging activity in a dose-dependent manner. In the PMS/NADH-NBT system, superoxide anions derived from dissolved oxygen by PMS/NADH coupling reaction reduce NBT. The decrease of absorbance at 560 nm with antioxidants thus indicates the consumption of superoxide anions in the reaction mixture. The extracts exhibited superoxide scavenging activity () at all the concentrations (10–50 µg mL−1). The superoxide scavenging capacities of the extracts follow the order TC-2>TC-4>TC-3>catechin. Catechin, the natural superoxide scavenger has an IC50 value of 14.6 ± 0.08 µg mL−1.
HPLC analysis
The effect of T. chebula extracts on α-glucosidase inhibition and in vitro antioxidant chemical assays were very impressive. Therefore, HPLC analysis was carried out in order to characterize these extracts in terms of their chemical composition. Four compounds namely chebulagic acid, chebulinic acid, corilagin and gallic acid were identified and quantified by the HPLC analysis. Both chebulagic and chebulinic acid were present in considerable amounts in all the three extracts. The results are tabulated in . The TC-2 extract showed the highest amount of these two compounds.
Since the TC-2 extract showed strongest activity in all the in vitro chemical antioxidant assays, it was subjected to antioxidant activity studies using cell lines.
Superoxide dismutase assay
The effect of TC-2 extract on the SOD activity was measured in terms of the intracellular ROS levels. The ROS or superoxide anion levels in the normal cells were taken as zero. When these cells are treated with PMA (control cells), the ROS levels were increased by +24 ± 1.4%. The variation of ROS levels in the extract pre-treated cells were shown in the . Concentrated extract (200 µL) reduced the superoxide levels up to −19.5 ± 0.3%.
Glutathione S-transferase assay
The phase II metabolizing enzyme GST plays an important role in the conjugative detoxification of electrophiles as well as being an essential component of cellular antioxidant defence mechanism. The effect of T. chebula extract on the GST modulation was presented in . The normal untreated cells has shown GST activity of 7.38 nmol/mL/min/mL, whereas in the H2O2-treated control cells has reduced GST activity of 1.8 nmol/mL/min/mL the extract pre-treated cells has shown very significant increase in the GST activity. Extracts at different concentration (10, 50, 100 and 200 µg mL−1) showed a dose-dependent increase in the GST activity of 3.2 times higher activity than the control cells (5.8 nmol/mL/min/mL).
Oxidative stress-induced ROS production
Oxidative stress-induced ROS production detected with DCFH-DA was significantly decreased by TC-2 extract in a dose-dependent manner. Based on this particular experiment, the TC-2 extract in 10–100 µg mL−1concentration range reduced oxidative stress in C2C12 cells in a dose-dependent manner (). The generation of ROS is increased in both types of diabetes and the onset of diabetes is closely associated with oxidative stress. Abnormally high levels of ROS and simultaneous decline of antioxidant defense systems can lead to the damage of cellular organelles and enzymes, increased lipid peroxidation and development of complications of diabetes mellitus. In the present experiment, no evidence of any increase or decrease in 2,7-dichlorofluorescein fluorescence was observed in cells incubated with extracts alone.
Figure 7. Evaluation of oxidative stress in C2C12 cell lines by flow cytometry. Blank – cells without any treatment; control – cells treated with hydrogen peroxide; A – cells treated with hydrogen peroxide and ascorbic acid (25 µg mL−1); others are cells treated with hydrogen peroxide and different concentrations (10, 20, 40, 80 and 100 µg mL−1) of TC-2 extract. Values are represented as mean value ± SD (n = 3).
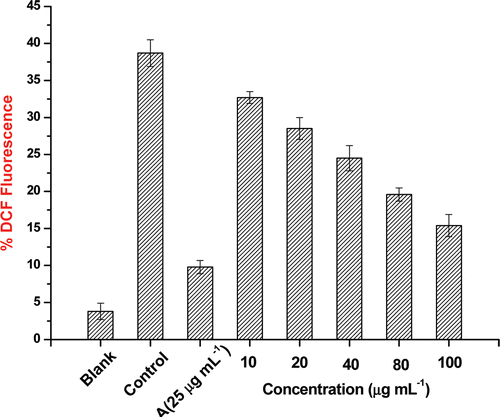
In diabetic condition due to hyperglycemia, there is excess generation of ROS. Overproduction and insufficient removal of ROS result in vascular dysfunction, damage to cellular proteins, membrane lipids and nucleic acids. The present study revealed that the T. chebula extract (TC-2) has a very high in vitro radical scavenging activity and which further confirmed by the cell line studies. This indicates that the extracts effectively reduces the ROS levels and thus helps in the management of diabetes.
Conclusion
The ethyl acetate (TC-2), methanol (TC-3) and methanol–water (70:30) (TC-4) extracts of T. chebula showed inhibitory potential against the key enzyme (α-glucosidase) linked to type 2 diabetes and also strong antioxidant activity. Out of the three extracts, the TC-2 fraction showed very low IC50 value for α-glucosidase inhibition. This may be attributed to its high hydrolysable tannin content comprising mainly chebulagic and chebulinic acids.
Acknowledgement
The authors are thankful to the Director, National Institute for Interdisciplinary Science and Technology for providing all the facilities and CSIR for the financial support to carry out the investigations.
Declaration of interest
The authors report no declarations of interest.
References
- Laube H., Acarbose: An Update of Its Therapeutic Use in Diabetes Treatment. Clin Drug Invest 2002;22:141–156.
- Wilson RL. Free radicals and tissue damage, mechanistic evidence from radiation studies. Biochemical Mechanisms of Liver Injury. 1998:123–224.
- Lollinger J. Free Radicals and Food Additives.; London: Taylor and Francis, 1981:121.
- Baynes JW. Perspectives in diabetes role of oxidative stress in development of complications in diabetes. Diabetes 1991;40:405–412.
- Collier A, Wilson R, Bradley H, Thomson JA, Small M. Free radical activity in type 2 diabetes. Diabet Med 1990;7:27–30.
- Logani MK, Davies RE. Lipid oxidation: biologic effects and antioxidants–a review. Lipids 1980;15:485–495.
- Edwards MV. Vegetable tanning materials of India IV. Myrabalans. Dehra Dun India Forest Leaflet 1945;75:19–25.
- Lin TC, Nonaka GI, Nishioka I, Ho FC. Tannins and related compounds. CII. Structures of terchebulin, an ellagitannin having a novel tetraphenylcarboxylic acid (terchebulic acid) moiety, and biogenetically related tannins from Terminalia chebula RETZ. Chem Pharm Bull 1990;38:3004–3008.
- Lin TC, Hsu FL. Tannins and related compounds from Terminalia arborea. Chin Pharm J 1996;48:167–175.
- Saleem A, Husheem M, Härkönen P, Pihlaja K. Inhibition of cancer cell growth by crude extract and the phenolics of Terminalia chebula retz. fruit. J Ethnopharmacol 2002;81:327–336.
- Sabu MC, Kuttan R. Anti-diabetic activity of medicinal plants and its relationship with their antioxidant property. J Ethnopharmacol 2002;81:155–160.
- Malekzadeh F, Ehsanifar H, Shahamat M, Levin M, Colwell RR. Antibacterial activity of black myrobalan (Terminalia chebula Retz) against Helicobacter pylori. Int J Antimicrob Agents 2001;18:85–88.
- Dutta BK, Rahman I, Das TK. Antifungal activity of Indian plant extracts. Mycoses 1998;41:535–536.
- Ahn MJ, Kim CY, Lee JS, Kim TG, Kim SH, Lee CK et al. Inhibition of HIV-1 integrase by galloyl glucoses from Terminalia chebula and flavonol glycoside gallates from Euphorbia pekinensis. Planta Med 2002;68:457–459.
- Badmaev V, Nowakowski M. Protection of epithelial cells against influenza A virus by a plant derived biological response modifier Ledretan-96. Phytother Res 2000;14:245–249.
- Kaur S, Grover IS, Singh M, Kaur S. Antimutagenicity of hydrolyzable tannins from Terminalia chebula in Salmonella typhimurium. Mutat Res 1998;419:169–179.
- Kaur S, Arora S, Kaur K, Kumar S. The in vitro antimutagenic activity of Triphala–an Indian herbal drug. Food Chem Toxicol 2002;40:527–534.
- Singleton VL, Rossi JA. Colorimetry of total phenolics with phosphomolybdic-phosphotungstic acid reagents. Am J Enol Vitic. 1965;16:144–158.
- Suresh Babu K, Tiwari AK, Srinivas PV, Ali AZ, China Raju B, Rao JM. Yeast and mammalian alpha-glucosidase inhibitory constituents from Himalayan rhubarb Rheum emodi Wall.ex Meisson. Bioorg Med Chem Lett 2004;14:3841–3845.
- Choi CW, Kim SC, Hwang SS, Choi BK, Ahn HJ, Lee MY, et al. Antioxidant activity and free radical scavenging capacity between Korean medicinal plants and flavonoids by assay-guided comparison. Plant Sci 2002;163:1161–1168.
- Re R, Pellegrini N, Proteggente A, Pannala A, Yang M, Rice-Evans C. Antioxidant activity applying an improved ABTS radical cation decolorization assay. Free Radic Biol Med 1999;26:1231–1237.
- Liu F, Ooi VE, Chang ST. Free radical scavenging activities of mushroom polysaccharide extracts. Life Sci 1997;60:763–771.
- Rook GA, Steele J, Umar S, Dockrell HM. A simple method for the solubilisation of reduced NBT, and its use as a colorimetric assay for activation of human macrophages by gamma-interferon. J Immunol Methods 1985;82:161–167.
- Habig WH, Pabst MJ, Jakoby WB. Glutathione S-transferases. The first enzymatic step in mercapturic acid formation. J Biol Chem 1974;249:7130–7139.
- Wang H, Joseph JA. Quantifying cellular oxidative stress by dichlorofluorescein assay using microplate reader. Free Radic Biol Med 1999;27:612–616.
- Gunawan-Puteri MDPT, Kawabata J. Novel α-glucosidase inhibitors from Macaranga tanarius leaves. Food Chem 123:384–389.
- Toda M, Kawabata J, Kasai T. Inhibitory effects of ellagi- and gallotannins on rat intestinal alpha-glucosidase complexes. Biosci Biotechnol Biochem 2001;65:542–547.
- Anam K, Widharna RM, Kusrini D. α-glucosidase inhibitor activity of Terminalia species. Int J Pharmacol. 2009;5:277–280.
- Gao H, Huang YN, Gao B, Kawabata J. Chebulagic acid is a potent alpha-glucosidase inhibitor. Biosci Biotechnol Biochem 2008;72:601–603.
- Toeller M. alpha-Glucosidase inhibitors in diabetes: efficacy in NIDDM subjects. Eur J Clin Invest 1994;24 Suppl 3:31–35.