Abstract
Microglial activation has been associated with neurodegenerative diseases by inducing the neuroinflammatory mediators such as nitric oxide (NO), TNF-α and IL-1β. (−)-Nyasol, a norlignan isolated from a medicinal plant Anemarrhena asphodeloides, showed anti-inflammatory potential in lipopolysaccharide (LPS)-activated BV-2 microglial cells. (−)-Nyasol inhibited the production of NO and prostaglandin E2 (PGE2) and also the expression of inducible nitric oxide synthase and cyclooxygenase-2, which are responsible for the respective production of NO and PGE2. It also suppressed the mRNA levels of TNF-α and IL-1β in activated microglial cells. These effects of (−)-nyasol were correlated with the inactivation of p38 MAPK and the suppression of LPS-induced I-κBα degradation. Taken together, these results suggest that (−)-nyasol can be a modulator in neuroinflammatory conditions induced by microglial activation.
Keywords::
Introduction
Activation of microglia has been associated with pathological hallmarks of several neurodegenerative diseases, including Alzheimer’s disease (AD), Parkinson’s disease, stroke and multiple sclerosisCitation1. The activated microglia has been known to be accumulated at the sites of injury or plaques in neurodegenerative central nervous system (CNS)Citation2. Although microglial cells, resident macrophages of the CNS, function as principal immune effector cells responding to any pathological event, massive activation of microglia generates proinflammatory cytokines and proinflammatory molecules. Excessive production of these neurotoxic proinflammatory mediators, such as tumor necrosis factor-α (TNF-α), interleukin-1β (IL-1β), nitric oxide (NO) and prostaglandin E2 (PGE2), plays a pivotal role in aggravating the degenerative processes in the inflamed CNSCitation3. Therefore, the inhibition of the production of proinflammatory molecules through the regulation of microglial activation can be useful therapeutic approach against neurodegenerative disorders.
Anemarrhena asphodeloides Bunge (Liliaceae) has been used as an antipyretic, antiinflammatory, antidiabetic and antidepressant agent in traditional medicine in Korea, China and JapanCitation4. Various constituents have previously been isolated from this plant, including mangiferinCitation5, timosaponinsCitation6 and (−)-nyasol (cis-hinokiresinol)Citation7. In previous report, we have isolated (−)-nyasol as an antagonist of luekotriene B4 receptor from sample plantCitation7. (−)-Nyasol has also been reported to exhibit anti-angiogenic response by reducing the VEGF-induced vessel growth in the mouse corneal neovascularization modelCitation8. Studies using rat basophilic leukemia cells and murine macrophage cells have demonstrated that (−)-nyasol suppresses the production of eicosanoid and NO, respectivelyCitation9. However, the effects of (−)-nyasol on neuroinflammatory response in activated microglia have not yet been studied. Therefore, in this study, we investigated the possibility of (−)-nyasol as a therapeutic potential against neurodegenerative disease associated with microglial activation.
Materials and methods
Test material
(−)-Nyasol (purity >99%) () was isolated as described previouslyCitation7. Briefly, the methanol extracts of crude materials (1 kg) were dispersed in water and extracted with EtOAc. The EtOAc soluble fraction (11 g) was chromatographed on a silica gel (200 g) column eluted with n-hexane-EtOAc (10:1, 5:1 and 1:1, 300 mL each) gradients to give three fractions. The second fraction (0.8 g) was again separated on a silica gel (70 g) column eluted with n-hexane–acetone (5:1) to afford four sub-fractions. The second sub-fraction (0.4 g) was further purified by HPLC (Shimadzu HPLC system with UV monitor at 254 nm; µ-Bondapak C18 column, 10 μm, 10 × 300 mm; eluent 50% aqueous MeOH; flow rate 2.0 mL/min) to afford pure (−)-nyasol as an active principle (120 mg, oil, [α]D 20 = −137.27°, c = 0.173, EtOH). The structure of (−)-nyasol, (Z)-(−)-4,4′-(3-ethenyl-1-propene-1,3-diyl) bisphenol, was identified by spectroscopic analyses (Mass, IR, 1H–1H COSY, DEPT, HMBC) which was in line with the previous dataCitation10. The purified compound was dissolved in dimethyl sulfoxide (DMSO), and the final concentration of DMSO in all experiments was less than 0.1%. All test concentrations of (−)-nyasol showed no significant effect on cell viability.
Figure 1. (A) Chemical structure of (−)-nyasol. (B) Effects of (−)-nyasol on lipopolysaccharide (LPS)-induced inflammatory cytokines in BV-2 microglial cells. The cells were stimulated with LPS in absence or presence of (−)-nyasol for 6 h. The levels of IL-1β and TNF-α mRNAs were determined by RT-PCR analysis. GAPDH was used as an internal control. The results shown are the representative of three independent experiments. (C) Effects of (−)-nyasol on LPS-induced nitrite production in BV-2 microglial cells. The amount of nitrite in culture medium was measured by using the Griess reagents, as described in Materials and methods. (D) Effects of (−)-nyasol on LPS-induced PGE2 production in BV-2 microglial cells. PGE2 concentrations were quantified in the culture supernatant by enzyme immunoassay (EIA). The values are expressed as the means ± SD of three individual experiments. *p < 0.01 and **p < 0.001 indicate significant differences from the LPS alone.
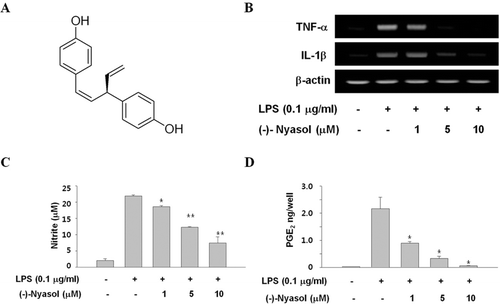
Cells and reagents
Murine microglial cell line (BV-2) was kindly provided by prof. Hee-Sun Kim at Ehwa Womans University, Korea. BV-2 cells were cultured at 37°C in 5% CO2 in Dulbecco’s modified Eagle’s medium (DMEM) supplemented with 10% fetal bovine serum (FBS), 100 units/mL penicillin, and 100 µg/mL streptomycin. Lipopolysaccharide (LPS) derived from E. coli serotype 055:B5 were purchased from Sigma Chemicals Co. (St. Louis, MO, USA). Antibodies against iNOS (BD Biosciences, Franklin Lakes, NJ, USA), COX-2 (Cayman Chemical Company, Ann Arbor, MI, USA), β-actin (Sigma Chemical Co., St. Louis, MO, USA), I-κBα and phospho-I-κBα (Santa Cruz Biotechnologies Inc. Santa Cruz, CA, USA) were used for immunoblot analysis. Antibodies against ERK, phospho-ERK, p38, phospho-p38, JNK and phospho-JNK were from Cell Signaling Technology (Danvers, MA, USA). TRIzol and SuperScript II first-strand cDNA synthesis system were purchased from Invitrogen (Carlsbad, CA, USA).
RNA isolation and reverse transcriptase polymerase chain reaction (RT-PCR) assay
BV-2 cells were treated with 0.1 µg/mL of LPS in the presence or absence of various concentrations of (−)-nyasol. After 6 h treatment, total RNA was isolated using a method of TRIzol extraction according to the manufacturer’s instructions. From each sample, 2 µg of total RNA was then reverse transcribed to single-stranded cDNA by the SuperScript II first-strand cDNA synthesis system. Then PCR analyses were performed on the aliquots of the cDNA preparations to detect IL-1β, TNF-α and GAPDH (as an internal standard) gene expression using a recombinant Taq polymerase. The PCR primer sequences for IL-1β, TNF-α, and GAPDH are as follows: IL-1β, sense 5′-GAGTGTGGATCCCAAGCAAT-3′ and antisense 5′-CTCAGTGCAGGCTATGACCA-3′; and TNF-α, sense 5′-AACTTCGGGGTGATCGGTCC-3′, and antisense 5′-CAAATCGGCTGACGGTGTGGG-3′; GAPDH, sense 5′- TGTGATGGGTGTGAACCACGAG-3′ and antisense 5′-TGCTGTTGAAGTCGCAGGAGAC-3′. The amplified PCR products were electrophoresed on 2% agarose gels and visualized using ethidium bromide staining and UV irradiation.
Measurement of nitrite concentration
BV-2 cells were activated with 0.1 µg/mL of LPS in the presence or absence of (−)-nyasol at various concentrations (1–10 µM). After 20 h treatment, supernatant of cell culture media were analyzed for nitrite (NO2−) by the Griess reactionCitation11. Briefly, an equal volume of Griess reagent (1% sulfanilamide/0.1% naphtylethyenediamine dihydrochloride in 2.5% H3PO4) was mixed with cell culture supernatants and color development was assessed at λ = 540 nm with a Versamax microplate reader (Molecular Devices, Sunnyvale, CA, USA). Fresh culture medium was used as the blank in all experiments. The amount of nitrite in the samples was calculated from a sodium nitrite standard curve.
Measurement of prostaglandin E2
The accumulated PGE2 in culture medium was determined by using enzyme immunoassay (EIA) kit from Cayman Chemical (Ann Arbor, MI, USA) according to the manufacturer’s instruction. A standard curve was prepared simultaneously with PGE2 standard ranging from 0.06 to 6 ng/mL.
Protein extraction and immunoblot
To prepare whole-cell lysis extracts, BV-2 cells were treated with 0.1 µg/mL of LPS in the presence or absence of various concentrations of (−)-nyasol. Following 20 h treatment, cells were harvested, washed twice with ice-cold phosphate buffered saline (PBS) (pH = 7.4), and gently lysed with cell lysis buffer (Cell Signaling Technology, Danvers, MA, USA). Cell lysates were then centrifuged at 10,000g for 20 min at 4°C. Supernatants were collected and protein concentrations were determined using BCA protein assay kit.
Cytosol and nuclear extraction
BV-2 cells were cultured in 6-well plates and then treated with 5 and 10 µM (−)-nyasol for 30 min prior to activation with 0.1 µg/mL of LPS. Following 15 min treatment, cells were washed twice with ice-cold PBS (pH = 7.4), and harvested using NE-PER nuclear and cytoplasmic extraction reagents according to the manufacturer’s instructions (Pierce Biotechnology, Rockford, IL, USA).
Statistical analysis
Data were presented as mean ± SD and p values were determined using the unpaired Student’s t-test. The difference was considered to be statistically significant when p values are less than 0.05.
Results and discussion
Traditional remedies derived from medicinal plants have been considered as safe and readily available even though the manner of actions is mostly still unclear. Thus, revealing the molecular mechanism of natural products for their chemical and biological activities might be a good strategy for the development of new therapeutic agents. In the course of searching plant-derived anti-neuroinflammatory compounds, (−)-nyasol () from Anemarrhena asphodeloides was found to exhibit a potent antiinflammatory effect in BV-2 murine microglia model. In present study, we investigated the effect of (−)-nyasol on neuroinflammation-related microglial functions.
During neuroinflammatory response, the activation of microglia contributes to host damage by excessive releasing of various proinflammatory cytokines such as IL-1β and TNF-α. TNF-α induces neuronal death when presented to neurons as part of a glial derived inflammatory conditionCitation12. IL-1β plays an important role as a direct mediator of neuroimmune interactions in neurodegenerative conditionCitation13. Thus, inhibition of proinflammatory cytokine production can give a key mechanism in the regulation of neuroinflammation involved in degenerative disorders. To assess whether (−)-nyasol controls the production of proinflammatory cytokines during inflammation, we investigated the effect of (−)-nyasol on the LPS induced expression of IL-1β and TNF-α mRNA in BV-2 microglial cells. Cells were treated with 1, 5 and 10 µM of (−)-nyasol in the presence of LPS (0.1 µg/mL) for 6 h. (−)-Nyasol attenuated the expression of IL-1β and TNF-α mRNA dose-dependently in LPS-stimulated microglial cells, whereas the IL-1β and TNF-α mRNA levels were markedly up-regulated by LPS treatment ().
Nitric oxide (NO) has been considered as a representative proinflammatory mediator of microglial activationCitation14. Prolonged microglial activation leads to an overproduction of proinflammatory mediators and subsequently contributes to neurodegenerative disorders. Therefore, we investigated whether (−)-nyasol affects the production of NO in LPS-stimulated microlial cells. The nitrite, a stable metabolite of NO produced by inducible nitric oxide (iNOS), was measured as the indicator of NO production in culture medium. (−)-Nyasol significantly inhibited the LPS-induced NO production in a dose-dependent manner, whereas LPS treatment dramatically increased the concentrations of NO ().
PGE2, another key inflammatory mediator, is produced by cyclooxygenase (COX)Citation15. COX enzyme has two isoforms of constitutive form (COX-1) and inducible form (COX-2). COX-1 is believed to have housekeeping function, while COX-2 is induced by proinflammatory mediators resulting in the stimulation of inflammatory responses. However, the contribution of these enzymes in prostanoid formation varies depending on the stimuli and cell type. In the brain, both COX-1 and COX-2 are constitutively expressed. In physiological conditions, COX-1 is mainly expressed in microglia and perivascular cellsCitation16,Citation17 and COX-2 is found in mostly in hippocampal and cortical glutamatergic neuronsCitation18. Recently, some reports have shown that COX-1 plays an important role in neuroinflammation and COX-2 is necessary to switch off CNS inflammation. This changes the classical view of COX-1 as the isoform of homeostasis and COX-2 as the major proinflammatory isoformCitation19,Citation20. As for the expression of COX expression in inflammatory model of microglia, different results have been reported. Cavello et al.Citation21 had described an increase of COX-1 and 2 levels in LPS- stimulated N13 microglial cells. On the other hand, there was no difference in COX-1 levels in LPS or hypoxia conditioned rodent microglia and BV-2 microglia cell line, whereas the expression of COX-2 was strongly inducedCitation22,Citation23. In addition, Kim et al.Citation24 showed that expression of mRNA and protein of COX-2 increased but the expression of constitutive COX-1 was not altered in LPS-induced BV-2 cells. Therefore, an increase of LPS induced PGE2 is mirrored by the up-regulation of COX-2 protein that was barely detectible in non-activated BV-2 cells. Taking the above factors into consideration, the present study was designed to evaluate the effect of (−)-nyasol against LPS-stimulated BV-2 microglial cells. To assess the inhibitory effect of (−)-nyasol on LPS-induced PGE2 production, we quantified PGE2 concentrations in the culture supernatant by EIA. (−)-Nyasol significantly inhibited the LPS-induced production of PGE2 in a dose-dependent manner; whereas LPS treatment dramatically increased the concentrations of PGE2 (). These results indicate that (−)-nyasol suppressed LPS-induced production of excessive inflammatory mediators, and could be helpful for the treatment of neuroinflammation associated with microglial activation.
Next, we examined the suppressive effect of (−)-nyasol on the expression of the proinflammatory enzymes iNOS and COX-2 which produce NO and PGE2 as key mediators of inflammation, respectively. As shown in , the mRNA levels of iNOS and COX-2 were suppressed by (−)-nyasol in LPS-stimulated BV-2 microglia. The iNOS and COX-2 protein levels were attenuated by the treatment of (−)-nyasol in a concentration-dependent manner, whereas the iNOS and COX-2 protein levels were markedly up-regulated by LPS treatment (). These results suggest that (−)-nyasol inhibits the production of proinflammatory NO and PGE2 by the down-regulation of iNOS and COX-2 expression in stimulated microglia.
Figure 2. (A) The effects of (−)-nyasol on LPS-induced iNOS and COX-2 mRNA levels in BV-2 microglial cells. The mRNA expressions of iNOS and COX-2 were examined by RT-PCR. Cells were treated with lipopolysaccharide (LPS) (0.1 µg/mL) in the presence or absence of (−)-nyasol for 6 h. (B) The iNOS and COX-2 protein levels were determined by Western blot analysis. Cells were treated with LPS (0.1 µg/mL) in the presence or absence of (−)-nyasol for for 20 h. The results shown are the representative of three independent experiments.
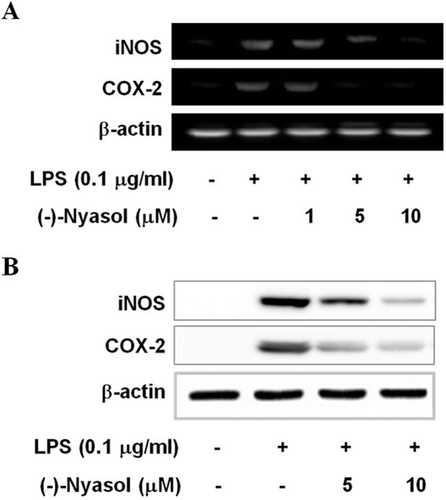
Nuclear factor-κB (NF-κB) is localized in cytoplasm as inactive dimer composed of p65 and p50 subunits and bound to the inhibitory protein I-κBα in resting microgliaCitation25. Microglial activation evokes I-κB kinase complex-induced phosphorylation of I-κBα, which leads to its degradation and subsequent translocation of NF-κB to the nucleus. The nuclear NF-κB activates the transcription of target genes, such as TNF-α, IL-1β, iNOS and COX-2, leading to the induction of their mRNA expressionCitation26. To elucidate the action mechanism of (−)-nyasol for the inhibitory consequence of inflammatory responses in activated microglia, we observed the effects of (−)-nyasol on the LPS-induced I-κBα degradation and NF-κB translocation to the nucleus. As shown in , the phosphorylation levels of I-κBα were increased in LPS-stimulated microglia, whereas (−)-nyasol treatment decreased the levels of I-κBα phosphorylation. In addition, I-κBα levels were markedly decreased with LPS treatment compared to the unstimulated control group, suggesting the LPS-induced degradation of I-κBα. (−)-Nyasol treatment reversed I-κBα levels in a concentration dependent manner. Furthermore, we also examined the effects of (−)-nyasol on the nuclear translocation of the p65 subunit of NF-κB. The p65 subunit was found in the nuclei of LPS-stimulated BV-2 microglia through the nuclear translocation of NF-κB from cytosol. (−)-Nyasol treatment markedly decreased the LPS-induced nuclear translocation of p65 subunit (). PARP and β-actin were used as internal controls. Taken together, these observations indicate that inhibitory effects of (−)-nyasol on I-κBα degradation and NF-κB nuclear translocation are responsible for the inhibitory production of proinflammatory mediators and cytokines in LPS-stimulated BV-2 microglial cells.
Figure 3. Effect of (−)-nyasol on lipopolysaccharide (LPS)-induced I-κBα degradation and p65 translocation to the nucleus in BV-2 microglial cells. Cells were pretreated with (−)-nyasol for 30 min prior to stimulation of LPS. After treatment with LPS for an additional 15 min, cytosolic I-κBα and nuclear p65 were analyzed by Western blot. Images are the representative of three independent experiments that shows similar results.
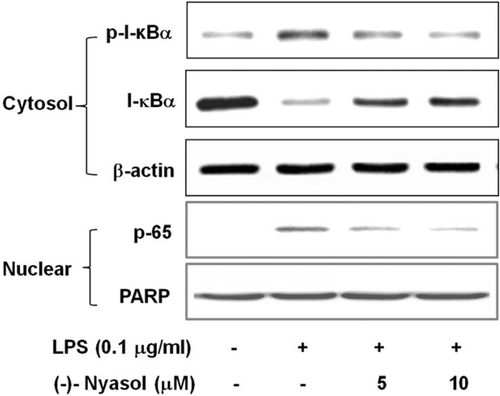
MAP kinases such as ERK, p38 and JNK are upstream signaling molecules in inflammatory reactions and mediate transcriptional changes of gene expression during the microglial activation processesCitation27. Several studies demonstrated that MAP kinases play a critical role in the overproduction of proinflammatory mediators and cytokines such as NO, PGE2, TNF-α and IL-1β by activated BV-2 microglial cellsCitation28. In addition, MAP kinases play an important role in the regulation of NF-κB activityCitation29. To determine the probable involvement of (−)-nyasol in the inhibitory action of inflammatory response, we examined the effect of (−)-nyasol on LPS-stimulated phosphorylation of MAP kinases in BV-2 microglial cells. Cells were pretreated with (−)-nyasol at the indicated concentrations for 30 min and then stimulated with LPS (0.1 µg/mL) for 15 min. The active phosphorylated forms of ERK, p38 and JNK was increased by LPS treatment. By the treatment of (−)-nyasol, the LPS-induced phosphorylation of p38 MAP kinase was reduced in a dose-dependent manner, whereas phosphorylations of ERK and JNK were not affected. Non-phosphorylated ERK, p38 and JNK were not changed by LPS and/or (−)-nyasol (). These results indicate that the suppression of p38 MAP kinase phosphorylation may contribute to the inhibitory effect of (−)-nyasol on inflammatory responses in LPS-stimulated BV-2 microglial cells.
Figure 4. Effect of (−)-nyasol on lipopolysaccharide (LPS)-induced activation of mitogen-activated protein kinases (MAPKs) in BV-2 microglial cells. Cells were pretreated with (−)-nyasol for 30 min prior to stimulation of LPS. After treatment with LPS for an additional 15 min, proteins were extracted and analyzed for the levels of phosphorylated extracellular signal-regulated protein kinase (ERK), p38 and c-Jun N-terminal kinases (JNK) by Western blot. The results shown are the representative of three independent experiments.
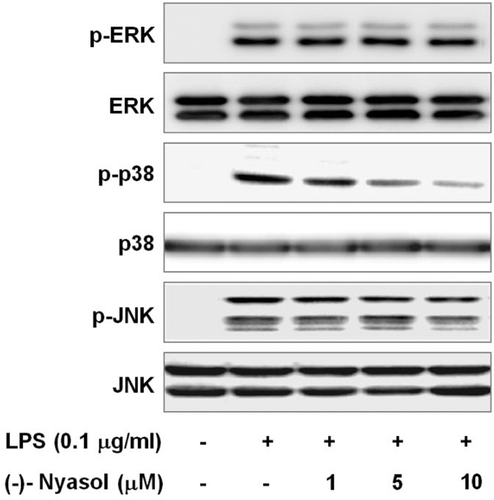
Lim et al.Citation9 reported that (−)-nyasol inhibited the iNOS mediated NO production and COX-2 mediated PGE2 production in LPS-treated RAW 264.7 cells, a mouse macrophage-like cell line. But, they observed the no effects of (−)-nyasol on the expression of iNOS and COX-2 at 100 µM concentration. In present study, we demonstrated that the expression levels of iNOS and COX-2 were inhibited via the suppression of NF-κB activation at 10 µM of (−)-nyasol in BV-2 microglia.
It has been reported that same compound may have inconsistent biological effects due to the different concentrations, cell lines and the experimental procedures. For instance, CYL-4d inhibits the production of NO more effectively in microglia than in macrophagesCitation30, whereas the other compound, such as naringenin, shows more effective inhibition of iNOS and COX-2 expression in macrophages than in microgliaCitation31. Resident microglia and blood-derived monocytes/macrophages may exhibit differential sensitivities in response to tested compound. Here, it seems reasonable to assume that the present findings may be of biological significance concerning the disclosed molecular action and the beneficial effects of (−)-nyasol in neuroinflammatory experimental studies in vitro.
In conclusion, (−)-nyasol, isolated from Anemarrhena asphodeloides, demonstrated the anti-inflammatory potential in LPS-activated BV-2 microglial cells. (−)-Nyasol suppressed the expression of TNF-α and IL-1β mRNA and the production of inflammatory mediators such as nitric oxide and PGE2. It reduced mRNA and protein levels of iNOS and COX-2 by the suppression of LPS-induced I-κBα degradation and also repressed the activation of p38 MAP kinase. The data above suggest that (-)-nyasol might have therapeutic potential for neuroinflammatory diseases.
Acknowledgement
This work was supported by Sookmyung Women’s University Research Grants in 2011 and by MRC program (2011- 0030699) through the National Research Foundation of Korea (NRF) funded by Korea Government (MEST).
Declaration of interest
The authors report no declarations of interest. The authors alone are responsible for the content and writing of the paper.
References
- González-Scarano F, Baltuch G. Microglia as mediators of inflammatory and degenerative diseases. Annu Rev Neurosci 1999;22:219–240.
- Rock RB, Gekker G, Hu S, Sheng WS, Cheeran M, Lokensgard JR, Peterson PK. Role of microglia in central nervous system infections. Clin Microbiol Rev 2004;17:942–64.
- Block ML, Hong JS. Microglia and inflammation-mediated neurodegeneration: multiple triggers with a common mechanism. Prog Neurobiol 2005;76:77–98.
- Kawasaki T, Yamauchi T. Saponins of timo (Anemarrhenae rhizoma). II. Structure of timosaponin A-III. Chem Pharm Bull 1963;11:1221–1224.
- Zhou T, Zhu Z, Wang C, Fan G, Peng J, Chai Y et al. On-line purity monitoring in high-speed counter-current chromatography: application of HSCCC-HPLC-DAD for the preparation of 5-HMF, neomangiferin and mangiferin from Anemarrhena asphodeloides Bunge. J Pharm Biomed Anal 2007;44:96–100.
- Saito S, Nagase S, Ichinose K. New steroidal saponins from the rhizomes of Anemarrhena asphodeloides Bunge (Liliaceae). Chem Pharm Bull 1994;42:2342–2345.
- Lee HJ, Ryu JH. Hinokiresinol: a novel inhibitor of LTB4 binding to the human neutrophils. Planta Med 1999;65:391.
- Jeong SJ, Higuchi R, Ono M, Kuwano M, Kim YC, Miyamoto T. cis-Hinokiresinol a norlignan from Anemarrhena asphodeloides, inhibits angiogenic response in vitro and in vivo. Biol Pharm Bull 2003;26:1721–1724.
- Lim H, Nam JW, Seo EK, Kim YS, Kim HP. (-)-Nyasol (cis-hinokiresinol), a norneolignan from the rhizomes of Anemarrhena asphodeloides, is a broad spectrum inhibitor of eicosanoid and nitric oxide production. Arch Pharm Res 2009;32:1509–1514.
- Bae G, Yu JR, Lee J, Chang J, Seo EK. Identification of nyasol and structurally related compounds as the active principles from Anemarrhena asphodeloides against respiratory syncytial virus (RSV). Chem Biodivers 2007;4:2231–2235.
- Green LC, Wagner DA, Glogowski J, Skipper PL, Wishnok JS, Tannenbaum SR. Analysis of nitrate, nitrite, and [15N]nitrate in biological fluids. Anal Biochem 1982;126:131–138.
- Wen W, Sanelli T, Ge W, Strong W, Strong MJ. Activated microglial supernatant induced motor neuron cytotoxicity is associated with upregulation of the TNFR1 receptor. Neurosci Res 2006;55:87–95.
- Rothwell N, Allan S, Toulmond S. The role of interleukin 1 in acute neurodegeneration and stroke: pathophysiological and therapeutic implications. J Clin Invest 1997;100:2648–2652.
- Hill KE, Zollinger LV, Watt HE, Carlson NG, Rose JW. Inducible nitric oxide synthase in chronic active multiple sclerosis plaques: distribution, cellular expression and association with myelin damage. J Neuroimmunol 2004;151:171–179.
- Kawano T, Anrather J, Zhou P, Park L, Wang G, Frys KA, Kunz A, Cho S, Orio M, Iadecola C. Prostaglandin E2 EP1 receptors: downstream effectors of COX-2 neurotoxicity. Nat Med 2006;12:225–229.
- Hoozemans JJM, Rozemuller AJM, Janssen I, De Groot CJA, Veerhuis R, Eikelenboom P. Cyclooxygenase expression in microglia and neurons in Alzheimer’s disease and control brain. Acta Neurophathol 2001;101:2–8.
- García-Bueno B, Serrats J, Sawchenko PE. Cerebrovascular cyclooxygenase-1 expression, regulation, and role in hypothalamic-pituitary-adrenal axis activation by inflammatory stimuli. J Neurosci 2009;29:12970–12981.
- Wang H, Hitron IM, Iadecola C, Pickel VM. Synaptic and vascular associations of neurons containing cyclooxygenase-2 and nitric oxide synthase in rat somatosensory cortex. Cereb Cortex 2005;15:1250–1260.
- Choi SH, Langenbach R, Bosetti F. Genetic deletion or pharmacological inhibition of cyclooxygenase-1 attenuate lipopolysaccharide-induced inflammatory response and brain injury. FASEB J 2008;22:1491–1501.
- Choi SH, Aid S, Bosetti F. The distinct roles of cyclooxygenase-1 and -2 in neuroinflammation: implications for translational research. Trends Pharmacol Sci 2009;30:174–181.
- Calvello R, Panaro MA, Carbone ML, Cianciulli A, Perrone MG, Vitale P, Malerba P, Scilimati A. Novel selective COX-1 inhibitors suppress neuroinflammatory mediators in LPS-stimulated N13 microglial cells. Pharmacol Res 2012;65:137–148.
- Li P, Lu J, Kaur C, Sivakumar V, Tan KL, Ling EA. Expression of cyclooxygenase-1/-2, microsomal prostaglandin-E synthase-1 and E-prostanoid receptor 2 and regulation of inflammatory mediators by PGE2 in the amoeboid microglia in hypoxic postnatal rats and murine BV-2 cells. Neuroscience 2009;164:948–962.
- Font-Nieves M, Sans-Fons MG, Gorina R, Bonfill-Teixidor E, Salas-Pérdomo A, Marquez-Kisinousky L, Santalucia T, Planas AM. Induction of COX-2 enzyme and down-regulation of COX-1 expression by lipopolysaccharide (LPS) control prostaglandin E2 production in astrocytes. J Biol Chem 2012;287:6454–6468.
- Kim IS, Ko HM, Koppula S, Kim BW, Choi DK. Protective effect of Chrysanthemum indicum Linne against 1-methyl-4-phenylpridinium ion and lipopolysaccharide-induced cytotoxicity in cellular model of Parkinson’s disease. Food Chem Toxicol 2011;49:963–973.
- Karin M, Ben-Neriah Y. Phosphorylation meets ubiquitination: the control of NF-κ B activity. Annu Rev Immunol 2000;18:621–663.
- Camandola S, Mattson MP. NF-kappa B as a therapeutic target in neurodegenerative diseases. Expert Opin Ther Targets 2007;11:123–132.
- Koistinaho M, Koistinaho J. Role of p38 and p44/42 mitogen-activated protein kinases in microglia. Glia 2002;40:175–183.
- Ock J, Kim S, Yi KY, Kim NJ, Han HS, Cho JY, Suk K. A novel anti-neuroinflammatory pyridylimidazole compound KR-31360. Biochem Pharmacol 2010;79:596–609.
- Surh YJ, Chun KS, Cha HH, Han SS, Keum YS, Park KK, Lee SS. Molecular mechanisms underlying chemopreventive activities of anti-inflammatory phytochemicals: down-regulation of COX-2 and iNOS through suppression of NF-kappa B activation. Mutat Res 2001;480–481:243–268.
- Lin MW, Tsao LT, Chang LC, Chen YL, Huang LJ, Kuo SC, Tzeng CC, Lee MR, Wang JP. Inhibition of lipopolysaccharide-stimulated NO production by a novel synthetic compound CYL-4d in RAW 264.7 macrophages involving the blockade of MEK4/JNK/AP-1 pathway. Biochem Pharmacol 2007;73:1796–1806.
- Chao CL, Weng CS, Chang NC, Lin JS, Kao ST, Ho FM. Naringenin more effectively inhibits inducible nitric oxide synthase and cyclooxygenase-2 expression in macrophages than in microglia. Nutr Res 2010;30:858–864.