Abstract
Two series of 1-(3-aminomethyl-4-hydroxyphenyl)-3-pyridinyl-2-propen-1-ones, designed as novel cytotoxins, were synthesized. The compounds had low CC50 values in the micromolar range against HL-60 promyelocytic leukemic cells and HSC-2, HSC-3 and HSC-4 oral squamous cell carcinomas. The CC50 values of these compounds were higher towards non-malignant HGF (gingival fibroblasts), HPC (pulp cells), and HPLF (periodontal ligament fibroblasts) cells, which reveals the tumour-selectivity of these enones. A representative compound 4c caused cleavage of PARP1 in HSC-2 cells but not in HGF cells, which may be a contributing factor to the tumour-selectivity.
Introduction
A major problem facing the human population at the current time is the devastating ravages of cancer. For example, in USA during 2010 at total of 569,490 individuals were expected to die from cancer i.e., more than 1500 people per day while over 1.5 million new cases of cancer are predicted to be diagnosed during 2010Citation1. Some of the major problems of current anticancer drug therapy include their lack of substantially greater toxicity for cancers compared to normal tissues and the emergence of multidrug resistance (MDR). Hence tumour-selective cytotoxins are urgently required whose structures are sufficiently divergent from contemporary anticancer medication so that MDR tumours are sensitive to these compounds.
The principal aim of our laboratory is the discovery of novel cytotoxic and anticancer alkylating agents. Some Mannich ketones as potential cytotoxins have emerged from our researchCitation2,Citation3. Chalcones are α,β-unsaturated ketones, and they are associated with diverse biological activitiesCitation4,Citation5. The cytotoxic activities of this class of compounds has been proposed due to the alkylating potential of α,β-unsaturated ketones towards cellular nucleophiles that are liberated in situ following deaminationCitation6. 4-Hydroxychalcones have demonstrated their effectiveness as cytotoxicCitation7,Citation8, antitumourCitation9, antioxidantCitation9 agents. The antimicrobialCitation10 and mosquito repellentCitation11 activities of o-aminomethyl phenol derivatives (Mannich phenols) are also noteworthy. In view of potential cytotoxicity associated with 4′-hydroxychalcones and Mannich ketones, we were interested in preparing a novel series of Mannich chalcones (2a–f, 4a–e ) in order to explore their effectiveness as potent cytotoxic agents.
Scheme 1. Structure and synthesis of the compounds 1–4. The reagents used in the synthesis are i = NaOH, ii = HCHO/amine.
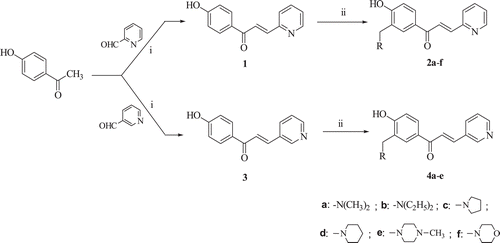
One reason for this pursuit is that conjugated enones react exclusively or preferentially with thiols in contrast to amino or hydroxyl groupsCitation12. Since the latter two groups, but not thiols, are found in nucleic acids, the genotoxic side effects of many anticancer drugs should be avoided. The interest in chalcones is due not only to their antineoplastic properties but also to the absence of their displaying pronounced toxicity in generalCitation13. The thiol-alkylating properties of chalcones will be enhanced by the presence of a strongly electron-withdrawing group in ring B such as a nitro substituent which will increase the electrophilicity of the adjacent olefinic carbon atom (). However nitroaromatics can be reduced to form nitroso and amino metabolites as well as reactive nitro and nitroxyl radicals all of which can induce oxidative stressCitation14. Hence the decision was made to replace aryl ring B of the chalcones by an electron-withdrawing pyridinyl ring.
Further modifications of the chalcone motif were proposed. One objective was to introduce a basic group into ring A. When this substituent is protonated, it will exert an electron-withdrawing effect which will reduce the electron density on the olefinic carbon atom adjacent to the pyridinyl ring. In so doing thiol alkylation will be enhanced and hence cytotoxic potencies may increase. Nitrogen atoms directly attached to aryl rings are weak bases and therefore a methylene spacer between ring A and the basic centre was proposed as indicated in . As the pH falls, so the percentage of the molecules in the ionized form will increase. The Hammett σm value of the dimethylaminomethyl group [CH2N(CH3)2], for example, is 0.00 but on protonation this substituent [CH2NH(CH3)2] is now a strongly electron-withdrawing group with a σm figure of 0.40Citation15. A number of tumours are more acidic than the corresponding normal cellsCitation16 and in these cases electrophilic attack of cellular thiols will increase in acidic tumours and hence selective toxicity to neoplasms may be demonstrated. Various amines were considered in order to observe whether cytotoxic potencies are governed by the nature of the basic group.
An additional substituent to be placed in ring A was a hydroxyl group for two reasons. First, this substituent may assist in hydrogen bonding at a receptor. Second, it is a suitable group for analogue development should this group of compounds prove to be promising lead molecules. For example, if the logP values are too high, the hydroxyl group can be converted into a disodium phosphate ester [OP(O)(ONa)2].
Therefore, the objectives of the present study are: (i) to develop a series of 1-(3-aminomethyl-4-hydroxyphenyl)-3-pyridinyl-prop-2-en-1-ones in order to find if cytotoxicity towards neoplasms was demonstrated and greater toxicity to malignant cells than normal cells is achieved; (ii) to discern any structural features of this series of compounds which govern cytotoxicity and tumour-selective toxicity; (iii) to investigate the possible mode of action of representative compounds by which cytotoxicity and selective tumour toxicity is mediated. Such information will be valuable in affording guidelines for expanding the project.
Materials and methods
Synthesis of the compounds 1–4
Melting points were determined using an Electrothermal 9100 (IA9100, UK) instrument and are uncorrectedCitation1. H NMR (400 MHz) and Citation13C NMR (100 MHz) spectra were obtained using a Varian Mercury Plus spectrometer (Palo Alto, USA). Chemical shifts (δ) are reported in ppm. Mass spectra were undertaken on a HPLC-TOF Waters Micromass LCT Premier XE (Milford, MA, USA) mass spectrometer using an electrospray ion source (ESI).
E-1-(3-(Dimethylaminomethyl-4-hydroxyphenyl)-3-(2-pyridinyl)prop-2-en-1-one (2a)
A solution of paraformaldehyde (0.066 g, 2.2 mmol) and dimethylamine (0.099 g, 2.2 mmol) in acetonitrile (5 ml) was heated under reflux in a microwave oven (80 watts) at 80°C for 5 minutes. A solution of compound 1 (0.50 g, 2.2 mmol) in acetonitrile (20 ml) was added to the reaction mixture and heating in the oven at 80°C continued. The reaction was monitored by TLC and after 7.5 h, the solvent was removed in vacuo and the crude product was chromatographed using a column of silica gel 60 (70–230 mesh) and chloroform: methanol (9:1) as eluant. Evaporation of the solvent afforded 2a as a yellow oil. Yield: 15%Citation1.H NMR (CDCl3) δ 2.34 (s, 6H), 3.73 (s, 2H), 6.87 (d, J = 8.7 Hz, 1H), 7.26 (dd, J = 7.8, 4.6 Hz, 1H), 7.45 (d, J = 7.8 Hz, 1H), 7.72 (dt, J = 7.8, 1.8 Hz, 1H), 7.74 (d, J = 15.3 Hz, 1H), 7.81 (d, J = 1.8 Hz, 1H), 8.00 (dd, J = 8.7, 1.8 Hz, 1H), 8.11 (d, J = 15.3 Hz, 1H), 8.66 (d, J = 4.6 Hz, 1H); 13C NMR (CDCl3) δ 44.6, 62.7, 116.4, 122.0, 124.4, 125.6, 125.7, 129.5, 129.9, 130.9, 137.1, 141.8, 150.3, 153.7, 163.8, 188.7; Mass spectrum: 283.14 (M++1); HRMS (ESI-MS) Calc.: 283.1447 for C17H18N2O2 [M+H+], Found: 283.1445.
The dienones 2b–f and 4a–e were prepared in a similar manner. A solution of compound 3 was used instead of 1 to synthesize 4a–e. The times of heating in the oven and the nature of the eluant used in column chromatography were as follows: 2b: 4h, chloroform: methanol (7:3); 2c: 8h, chloroform: methanol (6:4); 2d: 48h, ethyl acetate: methanol (8:2); 2e: 49h, chloroform: methanol (9:1); 2f: 48h, diisopropyl ether: methanol (7:3). 4a: 72h, chloroform: methanol (8:2); 4b: 72h, chloroform: methanol (8:2); 4c: 40h, chloroform: methanol (95:5); 4d: 84h, ethyl acetate: methanol (9:1); 4e: 40h, chloroform: methanol (95:5).
Variations to the general procedure are as follows. Paraformaldehyde and the appropriate amine were heated under reflux at 80°C for 0.5h in the synthesis of 2e,f and 4b–e and stirred at room temperature for 0.5h in the case of 4a. In the syntheses of 4d, a solution of 3 in ethanol (not acetonitrile) was added to paraformaldehyde and piperidine dissolved in acetonitrile. The solvent used in the preparation of 2e, 4c and 4e was ethanol and not acetonitrile.
E-1-(3-(Diethylaminomethyl-4-hydroxyphenyl)-3-(2-pyridinyl)prop-2-en-1-one (2b)
Yield: 47%. Yellow oilCitation1.H NMR (CDCl3) δ 1.11 (t, J = 7.1 Hz, 6H), 2.65 (q, J = 7.1 Hz, 4H), 3.85 (s, 2H), 6.84 (d, J = 8.6 Hz, 1H), 7.27 (dd, J = 7.2, 3.9 Hz, 1H), 7.45 (d, J = 7.2 Hz, 1H), 7.72 (d, J = 7.2 Hz, 1H), 7.73 (d, J = 15.4 Hz, 1H), 7.82 (d, J = 1.8 Hz, 1H), 7.99 (dd, J = 8.6, 1.8 Hz, 1H), 8.11 (d, J = 15.4 Hz, 1H), 8.67 (d, J = 3.9 Hz, 1H); 13C NMR (CDCl3) δ 11.3, 46.5, 56.9, 116.4, 122.2, 124.4, 125.6, 125.7, 129.3, 129.9, 130.7, 137.1, 141.7, 150.3, 153.7, 164.3, 188.6; Mass spectrum: 311.17 (M++1); HRMS (ESI-MS) Calc.: 311.1760 for C19H22N2O2 [M+H+], Found: 311.1765.
E-1-[4-Hydroxy-3-(pyrrolidin-1ylmethyl)phenyl]-3-(2-pyridinyl)prop-2-en-1-one (2c)
Yield: 77%. M.p.125-127°CCitation1.H NMR (CDCl3) δ 1.80–1.96 (m, 4H), 2.50–2.80 (m, 4H), 3.90 (s, 2H), 6.86 (d, J = 8.2 Hz, 1H), 7.26 (dd, J = 7.8, 4.1 Hz, 1H), 7.45 (d, J = 7.8 Hz, 1H), 7.72 (d, J = 7.8 Hz, 1H), 7.74 (d, J = 15.3 Hz, 1H), 7.82 (bs, 1H), 7.99 (d, J = 8.2 Hz, 1H), 8.11(d, J = 15.3 Hz, 1H), 8.67 (d, J = 4.1 Hz, 1H); 13C NMR (CDCl3) δ 23.9, 53.7, 58.7, 116.3, 122.6, 124.4, 125.6, 125.7, 129.3, 129.4, 130.8, 137.1, 141.8, 150.3, 153.7, 163.9, 188.7;
Mass spectrum: 309.16 (M++1); HRMS (ESI-MS) Calc.: 309.1603 for C19H20N2O2 [M+H+], Found: 309.1602.
E-1-[4-Hydroxy-3-(piperidin-1-ylmethyl)phenyl]-3-(2-pyridinyl)prop-2-en-1-one (2d)
Yield: 47%. M.p. (diethylether-methanol) 139°CCitation1.H NMR (CDCl3) δ 1.58–1.80 (m, 6H), 2.00–3.00 (m, 4H), 3.75 (s, 2H), 6.85 (d, J = 8.2 Hz, 1H), 7.26 (dd, J = 7.8, 4.6 Hz, 1H), 7.45 (d, J = 7.8 Hz, 1H), 7.73 (d, J = 7.8 Hz, 1H), 7.74 (d, J = 15.1 Hz, 1H), 7.81 (d, J = 1.8 Hz, 1H), 7.99 (dd, J = 8.2, 1.8 Hz, 1H), 8.11 (d, J = 15.1 Hz, 1H), 8.67 (d, J = 4.6 Hz, 1H); 13C NMR (CDCl3) δ 24.0, 26.0, 54.0, 62.0, 116.4, 121.8, 124.4, 125.6, 125.7, 129.4, 130.1, 130.7, 137.1, 141.8, 150.3, 153.7, 163.9, 188.6; Mass spectrum: 323.17 (M++1); HRMS (ESI-MS) Calc.: 323.1760 for C20H22N2O2 [M+H+], Found: 323.1767.
E-1-[4-Hydroxy-3-(4-methylpiperazin-1-ylmethyl)phenyl]-3-(2-pyridinyl)prop-2-en-1-one (2e)
Yield: 62%. M.p. 119–122°CCitation1.H NMR (CDCl3) δ 2.30 (s, 3H), 2.34–3.00 (m, 8H), 3.80 (s, 2H), 6.87 (d, J = 8.4 Hz, 1H), 7.28 (dd, J = 7.7, 5.0 Hz, 1H), 7.45 (d, J = 7.7 Hz, 1H), 7.72 (d, J = 7.7 Hz, 1H), 7.73 (d, J = 15.2 Hz, 1H), 7.83 (d, J = 2.0 Hz, 1H), 8.00 (dd, J = 8.4, 2.0 Hz, 1H), 8.11 (d, J = 15.2 Hz, 1H), 8.67 (d, J = 5.0 Hz, 1H); 13C NMR (CDCl3) δ 46.1, 52.7, 55.0, 61.3, 116.5, 121.3, 124.5, 125.6, 125.7, 129.7, 130.3, 130.9, 137.1, 141.9, 150.3, 153.6, 163.3, 188.6; Mass spectrum: 338.18 (M++1); HRMS (ESI-MS) Calc.: 338.1869 for C20H23N3O2 [M+H+], Found: 338.1858.
E-1-(4-Hydroxy-3-(morpholin-4-ylmethyl)phenyl)-3-(2-pyridinyl)prop-2-en-1-one (2f)
Yield: 49%. M.p. (diethylether-methanol) 138-139°CCitation1.H NMR (CDCl3) δ 2.40–2.70 (m, 4H), 3.70–3.78 (m, 4H), 3.79 (s, 2H), 6.88 (d, J = 8.4 Hz, 1H), 7.27 (dd, J = 7.6, 4.6 Hz, 1H), 7.45 (d, J = 7.6 Hz, 1H), 7.73 (d, J = 7.6 Hz, 1H), 7.74 (d, J = 15.1 Hz, 1H), 7.83 (d, J = 1.6 Hz, 1H), 8.01 (dd, J = 8.4, 1.6 Hz, 1H), 8.11 (d, J = 15.1 Hz, 1H), 8.67 (d, J = 4.6 Hz, 1H); 13C NMR (CDCl3) δ 53.1, 61.8, 66.9, 116.5, 120.9, 124.5, 125.5, 125.7, 129.9, 130.4, 131.0, 137.1, 142.0, 150.3, 153.6, 163.0, 188.6; Mass spectrum: 325.15 (M++1); HRMS (ESI-MS) Calc.: 325.1552 for C19H20N2O3 [M+H+], Found: 325.1543.
Biological activity
Cytotoxicity evaluation
The compounds in series 1–4 were assayed towards HSC-2, HSC-3, HSC-4, HL-60, HGF, HPC and HPLF cells based on a literature procedureCitation17 with some minor modifications. In brief, cells were cultured in DMEM supplemented with 10% foetal bovine serum (FBS) except the HL-60 cells were cultured in RPMI 1640 medium supplemented with 10% FBS. Varying concentrations of the compound in dimethylsulfoxide were added to the medium and incubated at 37°C for 48h. The viable cell numbers were determined by the MTT method except the viability of HL-60 cells was obtained by cell counting with a hemocytometer after staining with 0.15% trypan blue. The CC50 values were determined from dose-response curves.
PARP1 cleavage
The antibody against cleavage PARP1 was purchased from cell Signaling Technology (Beverly, MA, USA) while the anti-actin antibody was obtained from Santa Cruz Biotechnology (Santa Cruz, CA, USA). The HSC-2 and HGF cells were cultured in six well plates for 24h and then incubated with 4c for 24h. The cells were scraped and collected in lysis buffer (20 mM HEPES pH 7.4, 1% Triton-X 100, 150 mM NaCl, 1.5 mM MgCl2, 12.5 mM β-glycerophosphate, 2 mM EGTA, 10 mM NaF, 2 mM DTT, 1 mM Na3PO4, 1 mM PMSF plus 1 × protease inhibitor). Equal amounts of proteins were transferred onto a polyvinyldene difluoride membrane saturated with 5% non-fat dry milk. Membranes were incubated with primary antibody and then with horseradish peroxidase-conjugated secondary antibody of 1 h at room temperature. Membranes were washed with Tris-buffered saline with 0.5% Tween 20 and developed. Secondary antibodies were horseradish peroxidase-conjugated goat antimouse or antirabbit, and reacted with a chemiluminescent substrate system and exposed to X-ray films. A representative compound 4c, was examined for its ability to cause PARP1 cleavage, since it had the highest average SI value towards the four malignant cells among the compounds in series 1–4.
Molecular modelling
The models of the compounds in series 1–4 (Supplementary Figure S1) were built using ChemBio 3D ultra 12.0 and exported to Spartan 2008 for energy minimization using the AM 1 semi-empirical quantum mechanical chemistry method for determining atomic charges and torsion angles. The logP values were calculated using ChemBio3D ultra 12.0. The results were shown at .
Results and Discussion
Condensation between 4-hydroxyacetophenone and the appropriate formylpyridine afforded the unsaturated ketones 1 and 3. These compounds reacted with various amines and formaldehyde producing the corresponding Mannich bases 2a–f and 4a–eCitation1.H NMR spectroscopy revealed that aminomethylation occurred in the ortho position to the aryl hydroxy group and the olefinic double bond assumed the E configuration. Molecular modelling of the compounds in series 1–4 was undertaken in order to determine the atomic charges on the olefinic carbon atom adjacent to the pyridinyl ring, the θ1 and θ2 torsion angles (as indicated in Supplementary Figure S1) and logP values. These results are portrayed in .
The cytotoxicity data are presented in . A representative compound 4c caused cleavage of poly(ADP-ribose)polymerase 1 (PARP1) in HSC-2 but not HGF cells, and it is presented (Supplementary Figure S2).
Table 1. Cytotoxic evaluation of 1, 2a–f, 3 and 4a–e.
Table 2. Determination of some atomic charges and torsion angles of 1–4 and their logP values.
The first question to be addressed is whether the azachalcones 1–4 have antineoplastic properties. The results portrayed in reveal that in general the CC50 values of the compounds are in the low micromolar range towards HL-60, HSC-2, HSC-3 and HSC-4 cells. The potencies of 1-4 towards the tumour cell lines were compared with an alkylating agent, melphalan. Compounds 1, 2a–c, 3, 4c, e were more potent than melphalan towards HSC-3 cells while 1, 2b, c, e, f, 3, 4c–e had lower CC50 values in the HSC-4 bioassay. Furthermore, 1, 3 (HSC-2 screen), 2f, 4a, b, d (HSC-3 test) and 2a, 4a, b (HSC-4 assay) were equipotent with melphalan. The unsaturated ketones 1, 2e and 3 had the lowest average CC50 figures and are clearly lead molecules. In addition to these compounds, 2b, c, 4c, and e also had lower average CC50 values than melphalan. Thus, many of the compounds in series 1–4 possess noteworthy antineoplastic potency.
The second aspect of these compounds to consider is whether they are tumour-specific cytotoxins since tumours are surrounded by different types of normal cells. Selectivity index (SI) figures were generated which are the quotients of the average CC50 values of the non-malignant cells and the CC50 figure of a compound towards a specific cell line. The results in reveal that SI values of greater than 1 were obtained and thus series 1–4 are tumour-specific antineoplastic agents. An average figure of four was arbitrarily chosen as evidence of significant selective toxicity to neoplasms and was displayed by the compounds in series 1–4 except 2a, d–f. The greatest selectivity, which is 6.24, was noted with 4c (). The neoplasms differ in their sensitivity to the compounds, which is possibly caused by their having somewhat distinctive modes of action in each cell line.
Lead compounds should possess both marked cytotoxic potencies and selective toxicity for tumours. In order to identify such molecules, a potency selectivity expression (PSE) was devised which is the product of the reciprocal of the average CC50 values towards HL-60, HSC-2-, HSC-3 and HSC-4 cells (a measure of potency) and the average SI figures towards these cell lines (a determination of tumour-selectivity) expressed as a percentage. The PSE data are given in . Seven compounds have PSE figures greater than 30 namely 1, 2b, c, e, 3, 4c, e and serve as lead molecules for analogue development.
Investigations were also undertaken with a view to determine structural features which influence potency. In the first place, evaluations were conducted to find the optimal amino group(s) in 2a–f and 4a–e. In order to make these comparisons, a scoring system was employed whereby the most potent compound in series 2 was assigned a rating of 6, the compound with the next highest potency awarded 5 points and so forth. Standard deviations were taken into consideration, the scores for equipotent compounds were divided equally and a total of 21 points (Σ 6, 5, 4, 3, 2, 1) were awarded for 2a–f in each bioassay. In the case of 4a–e, 15 points (Σ 5, 4, 3, 2, 1) were allocated. Using this approach, the order of potencies (the scores are in parentheses) for series 2 were as follows, namely 2e (22.5) >2c (18.5) >2b (14.5) >2f (13.5) >2a (11) >2d (4). For series 4, the order of potencies were 4c, e (17) >4d (13.5) >4a (8) >4b (4.5). Thus in both series 2 and 4, the 4-methyl-1-piperazinyl and 1-pyrolidinyl groups are present in the most potent compounds. This can be a useful observation to consider analogue development.
A further issue requiring resolution was whether greater cytotoxic potency was going to be found in the 2-pyridinyl or 3-pyridinyl series of compounds having the same amino group. The 2-pyridinyl compounds had greater potencies than the analogues in series 4 in half (10/20) of the comparisons made. In six cases, equipotencies were observed while 4d was invariably more potent than 2d (4/20). It is of interest to note that 1 had lower CC50 values than 3 in the HL-60 and HSC-3 tests, while equipotency was observed in the other two bioassays. In general, therefore lower CC50 values were found in the 2-pyridinyl series 2 than with the structural isomers 4.
The average selectivity index figures reveal that each of the compounds in series 4 had a higher value than the analogues in series 2, which have the same amino substituent. The average SI figure of 4a–e was 5.31 in contrast to 3.90 for 2a–e. In addition, the average SI values of 1 and 3 were 4.35 and 5.28, respectively. Hence, the conclusion to be drawn is that in terms of demonstrating greater toxicity to neoplasms than normal cells, the presence of a 3-pyridinyl ring is preferable to the 2-pyridinyl isostere. The three compounds with the highest average SI figures are 3, 4c, d and are therefore lead molecules. One may also note that the SI values generated are dependent on the neoplastic cell line. For example, as indicated in , the average SI figures of the compounds in series 1–4 in 5.95 in the HL-60 screen which is more than double the value observed in the HSC-2 bioassay.
In regard to the PSE figures, the data in reveals that the average PSE values for 2a–e and 4a–e were similar being 24.9 and 28.0, respectively. The PSE data for 1 and 3 were also virtually identical. This observation confirms the earlier conclusions that the presence of a 2-pyridinyl group enhances potency, while the 3-pyridinyl analogues display greater toxicity to tumourous tissues than non-malignant cells.
Molecular modelling of the compounds in series 1–4 was undertaken with a view to discern correlations between various physicochemical characteristics of the molecules and the bioactivity observed with special reference to the general observations that potencies were greater in 2a–e than 4a–e, whereas the SI values were higher in series 4. Thus the atomic charges on the β-carbon atom were obtained. In addition, the torsion angles θ1 and θ2 between the aryl and pyridinyl rings with the adjacent unsaturated groups as illustrated in supplementary Figure S1 were generated. Furthermore the logP values of the compounds in series 1–4 were determined. These results are presented in .
Cellular thiols would be expected to react with α,β-unsaturated ketones at the β-carbon atomCitation6. Hence if the rate and extent of thiol alkylation is an important contributor to cytotoxicity, the charge densities on the β-olefinic carbon atom will influence potency considerably. The average atomic charges of 2a–e and 4a–e were −0.293 and −0.047, respectively. In terms of potency, this result is counterintuitive as one would have predicted that CC50 values would diminish as the fractional negative charge on the β-carbon atoms was lowered thereby enhancing nucleophilic attack by thiols. However, selective toxicity, which is greater in series 4 than 2, may be associated with the smaller negative charge on the β-carbon atom.
Several studies have revealed that the torsion angles between an aryl or heterocyclic ring and an adjacent unsaturated group are correlated with biological potenciesCitation18. Hence the decision was made to determine the θ1 and θ2 torsion angles which are indicated in supplementary Figure S1. One may note that in most cases the tilt of the aryl and pyridinyl rings in 1–4 are in opposite directions, i.e., the θ1 and θ2 values are in either clockwise (+) or anticlockwise (−) orientations. Insertion of aminomethyl groups into the meta position of the aryl rings in 1 and 3 leading to the meta position series 2 and 4 had a minimal effect on the θ1 values. Examination of the θ2 figures shows that the point of attachment of the pyridinyl ring to the β-carbon atom had a marked effect on the θ2 torsion angles. The average θ2 values of 2a–e and 4a–e were 33.4° and 10.6°, respectively, which may contribute to the variation in potency and selectivity between series 2 and 4. Thus in the future, replacement of the heterocyclic ring by an aryl ring possessing substituents in one or two ortho positions (creating high θ2 values) as well as placing the same groups in the meta and para locations (low θ2 figures) should be undertaken to explore this hypothesis.
The logP values of the compounds in series 1–4 are presented in . They are in the range of 1.5–3.0 and thus within one of the guidelines for compounds which are well absorbed after oral dosingCitation19. The logP values were higher in 2a–e than the analogues in series 4 which had the same basic group. In developing this project, compounds with a wider range of hydrophobicity should be prepared and subjected to bioevaluation. The results will shed some light on whether an increase in logP values will enhance cytotoxic potencies, while reduction in hydrophobicity will lead to compounds with increased selective toxicity to tumours.
Many cytotoxins exert their bioactivity by inducing apoptosisCitation20, but single-stranded DNA breaks can be repaired by poly(ADP-ribose)polymerase 1 (PARP1)Citation21. The compounds, which cleave PARP1 (and hence reduce the extent of repair of DNA damage) to a greater extent in neoplasms compared with normal cells, may be useful agents in cancer chemotherapy. A representative compound 4c, which had the highest average SI value towards the four malignant cells among the compounds in series 1–4, was examined for its ability to cause PARP1 cleavage in HSC-2 and HGF cells. Supplementary Figure S2 reveals that some cleavage of PARP1 occurs only in HSC-2 cells not in HGF cells after 24 h at 25 µM. Hence, a possible factor contributing to the tumour-selectivity of 4c (and maybe to other compounds in series 1–4) is the ability to inhibit DNA repair preferentially in malignant cells.
Conclusions
This study revealed that various 1-(3-aminomethyl-4-hydroxyphenyl)-3-pyridinyl-2-propen-1-ones possess not only noteworthy potencies towards several neoplasms but demonstrate a greater toxicity to tumours than various non-malignant cells. In general, the compounds in series 2 are more potent than the analogues in series 4. On the other hand, greater SI values are found among 4a–e. Using HSC-2 cells, a representative compound 4c cleaved PARP1 but at the same concentration this effect was not noted in HGF cells. Various physicochemical parameters obtained from molecular modelling enabled some guidelines for amplification of the project to be made.
Supplementary Material
Download PDF (94.2 KB)Acknowledgments
This study was supported by the Research Foundation of Ataturk University (Project Number: 2009/317, 2010/166) Erzurum, Turkey.
Declaration of interest
The authors report no conflict of interest and are responsible for the contents and writing of the paper.
References
- American Cancer Society, Cancer Facts and Figures 2010, American Cancer Society, Atlanta, 2010. Cancer Facts and Figures 2010, American Cancer SocietyM, Atlanta, Georgia, 2010, http://www.cancer.org/acs/groups/content/@nho/documents/document/acspc-024113.pdf, accessed on June 21, 2012.
- Gul HI, Gul M, Erciyas E. Toxicity of some bis Mannich bases and corresponding piperidinols in the brine shrimp (Artemia salina) bioassay. J Appl Toxicol 2003;23:53–57.
- Gul M, Gul HI, Das U, Hanninen O. Biological evaluation and structure-activity relationships of bis-(3-aryl-3-oxo-propyl)-methylamine hydrochlorides and 4-aryl-3-arylcarbonyl-1-methyl-4-piperidinol hydrochlorides as potential cytotoxic agents and their alkylating ability towards cellular glutathione in human leukemic T cells. Arzneimittelforschung 2005;55:332–337.
- Katsori AM, Hadjipavlou-Litina D. Chalcones in cancer: understanding their role in terms of QSAR. Curr Med Chem 2009;16:1062–1081.
- Dimmock JR, Elias DW, Beazely MA, Kandepu NM. Bioactivities of chalcones. Curr Med Chem 1999;6:1125–1149.
- Gordon PN, Johnston JD, English AR. Beta-aminoketones as anti-infective agents. In: Hobby GL, ed. Antimicrobial Agents and Chemotherapy. Bethesda: American Society for Microbiology, 1965;165–167.
- Dimmock JR, Kandepu NM, Hetherington M, Quail JW, Pugazhenthi U, Sudom AM et al. Cytotoxic activities of Mannich bases of chalcones and related compounds. J Med Chem 1998;41:1014–1026.
- Gul HI, Cizmecioglu M, Zencir S, Gul M, Canturk P, Atalay M et al. Cytotoxic activity of 4′-hydroxychalcone derivatives against Jurkat cells and their effects on mammalian DNA topoisomerase I. J Enzyme Inhib Med Chem 2009;24:804–807.
- Calliste CA, Le Bail JC, Trouillas P, Pouget C, Habrioux G, Chulia AJ et al. Chalcones: structural requirements for antioxidant, estrogenic and antiproliferative activities. Anticancer Res 2001;21:3949–3956.
- Molodykh ZV, Kudryavtseva LA, Shagidullina RA, Shtanova LV, Teitelbaum AB, Zotova AM, Ryzhkina IS, Kudrina MA, Anisimova NN, Ivanov BE. . Antimicrobial Activity of Ortho-Aminomethylphenols and Their Derivatives. Khimiko-Farmatsevticheskii Zhurnal 1987;21:182–186.
- Kovalenko LG, Viktorov-Nabokov OV, Ruban EM, Skrynik EM, Denisova ZA. [Repellent properties of Mannich bases and cresol and phenol derivatives for Aedes aegypti L. mosquitoes and the flea Xenopsylla cheopis Roths]. Med Parazitol (Mosk) 1983;52:46–48.
- Mutus B, Wagner JD, Talpas CJ, Dimmock JR, Phillips OA, Reid RS. 1-p-Chlorophenyl-4,4-dimethyl-5-diethylamino-1-penten-3-one hydrobromide, a sulfhydryl-specific compound which reacts irreversibly with protein thiols but reversibly with small molecular weight thiols. Anal Biochem 1989;177:237–243.
- Pati HN, Das U, Sakagami H, Kawase M, Chu Q, Wang Q et al. 1,3-Diaryl-2-propenones and 2-benzylidene-1,3-indandiones: a quest for compounds displaying greater toxicity to neoplasms than normal cells. Arch Pharm (Weinheim) 2010;343:535–541.
- Kerns EH, Di L.. Drug-like properties: concepts, structure design and methods: from ADME to toxicity optimization. Oxford: Academic Press, 2008, p. 220.
- Perrin DD, Dempsey B, Serjeant EP.. pKa Prediction for Organic Acids and Bases. London: Chapman and Hall, 1981, p. 116.
- Wike-Hooley JL, Haveman J, Reinhold HS. The relevance of tumour pH to the treatment of malignant disease. Radiother Oncol 1984;2:343–366.
- Motohashi N, Wakabayashi H, Kurihara T, Fukushima H, Yamada T, Kawase M et al. Biological activity of barbados cherry (acerola fruits, fruit of Malpighia emarginata DC) extracts and fractions. Phytother Res 2004;18:212–223.
- Pandeya SN, Dimmock JR. An Introduction to Drug Design. New Delhi: New Age International (P) Limited, 1997, pp.72–74.
- Lipinski CA, Lombardo F, Dominy BW, Feeney PJ. Experimental and computational approaches to estimate solubility and permeability in drug discovery and development settings. Adv Drug Del Rev 1997;23:3–25.
- Tsurusawa M, Saeki K, Fujimoto T. Differential induction of apoptosis on human lymphoblastic leukemia Nalm-6 and Molt-4 cells by various antitumor drugs. Int J Hematol 1997;66:79–88.
- Malanga M, Althaus FR. The role of poly(ADP-ribose) in the DNA damage signaling network. Biochem Cell Biol 2005;83:354–364.