Abstract
Adenylyl cyclases, comprise of a large family of enzymes that catalyze synthesis of the cyclic AMP from ATP. The aim of our study was to determine the effect of monovalent ions on both basal, stimulated adenylate cyclase EC 4.6.1.1 (AC) activity and C unit of AC and on GTPase active G-protein in the synaptic membranes of rat brain cortex. The effect of ion concentration from 30 to 200 mM (1 mM MgCl2) showed dose-dependent and significant inhibition of the basal AC activity, stimulated and unstimulated C unit activity. Stimulation of AC with 5 μM GTPγS in the presence of 50–200 mM of tested salts showed inhibitory effect on the AC activity. From our results it could be postulated that the investigated monovalent ions exert inhibitory effect on the AC complex activity by affecting the intermolecular interaction of the activated α subunit of G/F protein and the C unit of AC complex an inhibitory influence of tested monovalent ions on these molecular interaction.
Introduction
The monovalent ions including Na+, K+ and Cl− represent the basic components of all mammalian cells. Distributions of these ions on the cellular level are maintained due to their active transport by the ionic pump. The molecular basis of these pump is Na+,K+-ATPase enzyme system located in the cell membraneCitation1,Citation2. Due to the activity of this enzyme, the intracellular concentrations of Na+ and Cl− ions remain very low compared to the high K+ concentration. On the other hand, the extracellular concentrations of these ions are in the opposite mannerCitation2,Citation3. Moreover, the flux of Na+, K+ and Cl− ions at the membrane level of neurons generate an alteration in the membrane polarity (depolarization or hyperpolarization), which is the key mechanism of the interneural communication in the central nervous system (CNS)Citation4,Citation5. The effect of neurotransmitter signaling in the CNS modulates the permeability and/or the active transport of both monovalent (Na+, K+ and Cl−) and divalent (Ca2+ and Mg2+) ions in both postsynaptic and total neuron membranesCitation4,Citation6. The neurotransmitters can either directly or indirectly affect the permeability of the above-mentioned ions at the neuron membranes as well as the activity of Na+,K+-ATPaseCitation7,Citation8. The adenylate cyclase EC 4.6.1.1 (AC) is transmembrane enzymes, which catalyze conversion of the ATP to cAMPCitation9. Further, cAMP as the intracellular messenger activates protein kinase A (PKA) that initiates an enzyme cascade reactions of phosphorylation within the cellCitation10. The mammalian transmembrane AC consists of C1 and C2 homologous cytoplasmic domainsCitation9. The catalytic inactivity of the AC activity is occurred if either C1 or C2 domain is expressed separately. The activity of AC is gained exclusively by combining both C1 and C2 domains allowing the conversion of ATP to cAMPCitation11,Citation12. Earlier, it was believed that the active AC complex required the presence of two metal ions for its activity. However, the active AC (C1/C2) complex require only Mg2+ ion bound to the C1 domain or to a lesser extend Mn2+ ion bound to C2 domainCitation12–14. The effect of divalent cations, such as Mg2+ and Ca2+ on the AC activity ha been relatively well documentedCitation15–18. However, only a few studies demonstrated the effect of both monovalent cations and anions on the AC activityCitation19–23. In addition, most of the studies investigated the effect of both anions and cations, on the AC activity in the membrane samples of the different peripheral tissues, and opposite results were obtained. Some studies observed only the stimulative effect of both monovalent cations and anions on the AC activity by applying different forms of saltCitation21, while the other studies imposed the same effect exclusively on the monovalent anionsCitation20,Citation24,Citation25. Some authors emphasized stimulative effect of the monovalent anions was directly depended on the presence of Mg2+ ionsCitation20. Replacement of Mg2+ with Mn2+ ions significantly decreased the stimulative effect of Cl− on the AC activityCitation20,Citation26. However, only a few studies tested the effects of monovalent ions on the brain, especially on the brain synaptic membranes AC activityCitation7. The stimulative effect of monovalent ions, such as Na+, K+, choline+, and Cl− on the AC activity directly depended on the presence of F− ionsCitation20,Citation21, isoproterenol (with GTP)Citation21,Citation22 or nonhydrolyzing GTP analogs such as Gpp (NH)p and GTPγSCitation20. Furthermore, some studies showed the specific inhibitory role of the monovalent cations, especially Li+ 17 and Na+Citation27 on the AC activityCitation28,Citation29. On the other hand, some monovalent salts including NaCl, KCl, RbCl and CsCl demonstrated dose-dependent stimulative effect on the AC activity in rat liver cell membranes; however it was unclear if the simulative effect was induced either by the cation or by the anion part of the tested salts, but rather it was the consequence of both monovalent ionsCitation30. Eventhough, the monovalent salts did not affected the Km value for ATPCitation20, they only increased the Vmax of AC activityCitation30. However, the most authors gave the advantage to the anions as the active component of the monovalent salts for both stimulatory and inhibitory effects on the AC activityCitation24,Citation25,Citation31,Citation32. Finally, from all mentioned publishing results it is quite difficult to conclude the actual effect of the monovalent ions on the activity of AC complex. Both the inhibitory and the stimulatory effects of the monovalent ions differ in the relation to the type of the prepared membranes, although opposed effects of the monovalent ions were also observed within the same membrane preparationCitation26,Citation33,Citation34.
The aim of this paper was to examine the effect of the monovalent ions, Na+, K+, Rb+, and choline+, in the form of chloride (Cl−) salts (NaCl, KCl, RbCl and choline-Cl), and in the presence of different Mg2+ ion concentrations on the basal and stimulated AC complex activity in the synaptic membranes of the rat brain cortex.
Materials and methods
Experimental animals
Male Wistar rat’s weight 200 ± 10 g was used as the experimental animals. Animals were kept in the temperature-controlled room (25°C). Food and water were ad libitium.
Brain tissue
The decapitation was performed between 8 am and 9 am in the “cold room” (4°C). After decapitation brains were removed from the skulls within 15 s and whole brains were immersed in the cold (4°C) 0.32 M saharose solution (“brain washing”). After 5 min staying in this cold-saharose solution the fore brain cortex were dissected.
Synaptic membranes separation
Separation of the synaptic membranes was performed according to Gurd et al.Citation35. In short, after the homogenization of the brain tissue the synaptosomal fractions from unpurified mitochondria, synaptosomal fraction were separated using the Ficoll’s discontinued gradient. The synaptosomal fraction were washed (0.32 M saharose), lysated (1 mM tris–HCl buffer, pH = 7.4) and used for the separation of the synaptic membranes by fractional centrifugation on the multilayer saharose gradients. The amount of the proteins in the samples was 1 mg/mL. The usual synaptic membrane preparations were complex made of the presynaptic membranes, part of the synaptosomal membranes and part of the postsynaptic membranes which interconnected with the inter synaptic filaments with pre synaptic membranes.
Excluding of calmodulin and Ca2+ ions (“washed membranes”)
The separation of the calmodulin and Ca2+ ions from the synaptic membrane preparations were performed by washing and centrifuging the synaptic membrane preparations for five times in 50 mM tris–HCl pH 7.4 buffer solution containing 0.1 mM EDTA and 0.1 mM EGTA. However, the complete separation of calmodulin, even after multi short washing, could not be done due to its tight bondage to the C unit of the AC complexCitation33. Therefore, small amount of the calmodulin was present in all used synaptic membrane preparations.
Catalytic unit separation
For the separation of the catalytic unit (C unit) from the AC complex the washed synaptic membranes the modified method according to Bradham and HegazyCitation34 was used. The final protein concentration of 1 mg/mL was made by diluting the isolated synaptic membrane preparation with 50 mM tris–HCl buffer pH 7.4. In order to gain the final concentration of 0.2 M NaCl in the diluted synaptic membrane solutions, the adequate volume of 4 M NaCl solution was added. The samples were incubated at 37°C for 15 min, incubated with 2 mM ditiotreitol (DTT) and 0.5% Lubrol PX for 45 min at 4°C and centrifuged at 100,000×g for 60 min. The supernatant containing the soluble AC protein complex was extracted and used for the gel filtration. The separation of the C unit was performed on 400 mL Sepharose CL-6B colon equilibrated with 50 mM tris–HCl buffer, pH 7.4 containing 0.4% Lubrol PX solution and 2 mM DTT. The determination of the blank and controls were performed by 0.5% blue dextran solution. The separation of the C unit of the AC complex was followed with the separation of the G-proteinCitation34. The small amount of the isolated C unit and the G-protein fractions mutually overlaid, thus the AC activity was determined both in the presence and in the absence of the GTPγS in all secluded fractions. This way, the stimulative effect of the GTPγS on the C unit preparation indicated the presence of G-protein or presence of only α-subunit of G-protein on the isolated C unit. These fractions were eliminated.
Measuring the AC activity
Both the AC and the isolated C unit activity were determined by the amount of synthesised cAMP per time (1 min) in the correlation to 1 mg of proteins in the isolated C unit of the AC complex. The 0.5 mL of incubating medium contained (final concentrations) 50 mM tris–HCl buffer, pH 7.4, 5 mM teophiline and 1 mM DTT. The synaptic complex sample (25 µg proteins) and isolated C unit (10 µg proteins) were pre incubated in the incubation medium (without ATP) for 10 min. To eliminate Ca2+ ions, the sample of the synaptic complex were both pre incubated and post incubated for 5 min with 0.01 and 0.1 mM EGTA, respectively. The reaction started by adding water solution of ATP and after 10 min of incubation the reaction was stopped by placing the test tubes in the boiling water bathroom (100°C) for 3 min. Proteins were extracted from the incubation medium by the centrifugation. The content of the cAMP was determined in the water phase. The boiling effect for stopping the enzyme reaction had no any effect on the cAMP contentCitation36.
Measuring the cAMP
The amount of the synthesized cAMP was measured according to GeislerCitation36. The principle of the method consists of the competitive binding of the label (3H)-cAMP with unlabel (synthesised) cAMP in the incubation medium to the rabbit skeletal muscle cAMP binding protein. The concentration of the label (3H)-cAMP was measured by β-counter. The cAMP binding protein were isolated according to Miyamoto et al.Citation10.
Measuring the GTPase activity of the G-protein
Measuring of the GTPase of the G-protein was done according to Cassel and SelingerCitation37. The incubation medium (0.1 mL) contained the final concentrations of 50 mM imidasole–HCl buffer, pH 7.4; 0.5 mM (App(NH)p); 2 mM β-mercaptoethanol; 0.1 mM (ATP); 3 mM creatine phosphate; 3 units (IU) of creatine phosphokinase; and 0.1 mM EGTA. Synaptic membrane sample was pre incubated for 10 min in the incubation medium contained all components except (γ-32P) GTP as substrate. To exclude Mg2+ ions, synaptic membrane sample were pre incubated for 10 min in 0.1 mM EDTA medium. The reaction was started by adding water solution of (γ-32P) GTP (5–20 mCi/mM) and after 10 min the incubation was stopped by adding 0.5 mL of cold 5% charcoal suspension (Norit-A) in 20 mM Na-phosphate buffer, pH 7.0. Samples were kept 5 min on ice and then centrifuged at 5000 rpm for 15 min to remove Norit-A suspension. Water phase was carefully removed and transferred to the other test tubes in which the content of labeled 32P was measured. The amount of the released 32P from (γ-32P) GTP was measured in β-counter. Since there was a spontaneous release of 32P from (γ-32P) GTP the control sample were included identical incubating medium but with absent of the synaptic membrane sample (no enzyme activity).
Chemicals
All chemical used in this study were products of Merck (Darmstadt, Germany), SERVA (Heidelberg, Germany), Boehringer (Mannheim, Germany), Calbiochem (Lucerne, Switzerland) and Sigma (St. Louis, SAD). Radioactive marked 3H-cAMP and (γ-32P) GTP were products of Amersham (Amersham, England).
Results
The effect of the monovalent ions on the basal AC activity
Tested monovalent ions were in the Cl− salt form and in the final concentrations from 1 to 200 mM. Final concentrations of both Mg2+ ions and ATP were 1 mM. Increasing concentration of tested salts up to 10 mM had no significant effect on the AC activity (). However, tested salts in the concentration from 30 to 200 mM show statistically significant and dose-dependent inhibitory effect (). The inhibitory part of the kinetic curve was plotted by Dixon’s graph with average Ki value of 27 mM (). In the presence of 10 and 100 mM MgCl2 (1 mM ATP), increasing concentration of tested salts did not show any effect on the basal AC activity in the rat brain cortex synaptic membranes (data not shown).
Figure 1. The basic adenylate cyclase activity was evaluated in the presence of the increasing concentrations of the monovalent salts choline-Cl, NaCl, KCl and RbCl (1 mM MgCl2; 1 mM ATP). The effect of the increased monovalent ions on the basic adenylate cyclase activity, expressed statistically significant inhibitory effect on the activity of the adenylate cyclase in the concentrations range of the used monovalent salts from 30 to 200 mM. Full dark circle represents Choline-Cl, full white circle represents NaCl, full dark square represents KCl and full white square represents RbCl.
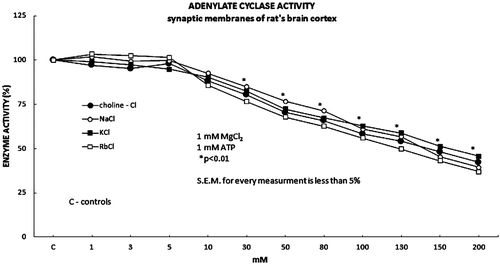
The effect of the monovalent ions on the stimulated AC activity
Neither F− ions (5 mM NaF) nor forskoline (50 μM) showed any effect on the graph curve and Ki value on tested monovalent ions on the AC activity (results not shown). However, the stimulation of the AC complex with 5 μM of GTPγS shifted the initial inhibitory effect of the AC activity to the higher concentration of tested salts (50 mM) ( and ). Furthermore, the increasing concentrations of tested ions (50–200 mM) showed the significant and dose-dependent inhibitory effect on the GTPγS stimulated AC activity (). The average Ki value calculated from the plotted Dixon’s graf was 17 mM, which was significantly lower compared to the average value of Ki = 27 mM for the control (unstimulated) AC activity ().
Figure 3. The adenylate cyclase activity was stimulated with 5 μM GTPτS in the presence of 1 mM MgCl2 and the monovalent ions in forms of their inorganic salts, choline-Cl, NaCl, KCl and RbCl. All tested monovalent ions exhibited significant inhibitory effect on the adenylate cyclase activity when applied in the higher concentrations (50 mM) compared to the control (30 mM). The investigated monovalent ions expressed both the statistically significant and the permanent inhibitory effect on the adenylate cyclase activity in the concentration range from 50 to 200 mM. Full dark circle represents Choline-cl, full white circle represents NaCl, full dark square represents KCl and full white square represents RbCl.
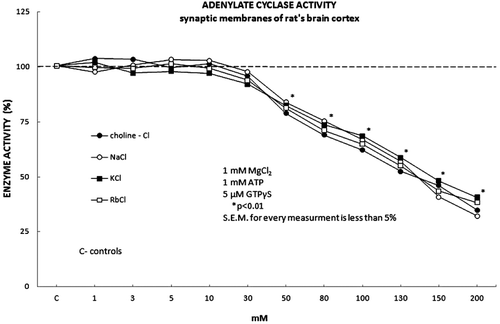
Figure 4. The inhibitory part of the kinetic curve for the adenylate cyclase activity is presented in the form of Dixon’s graph. The adenylate cyclase activity was stimulated with 5 μM GTPτS in the presence of the increasing concentrations of the RbCl. The kinetic activity value Ki for the adenylate cyclase was 17 mM, which is statistically significantly lower compared to the controlled value Ki for the basic kinetic activity of the adenylate cyclase (Ki = 27 mM).
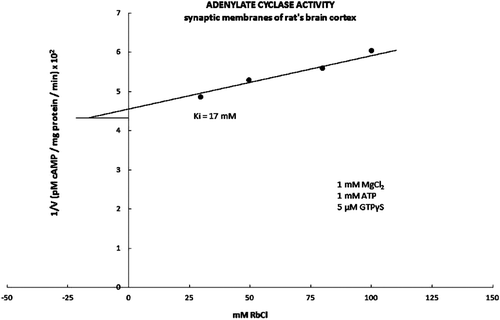
The effect of the monovalent ions on the unstimulated and stimulated C unit activity
Next, we examined the effect of the increasing concentrations of the monovalent ions (choline-Cl, NaCl, KCl, RbCl) on the activity of the isolated C unit of the AC complex (1 mM MgCl2; 1 mM ATP) (). The kinetic curve of the inhibitory effect of all tested monovalent ions on the activity of the isolated C unit of the AC complex was the identical to the inhibitory curve of the same monovalent ions affecting the basic AC activity (1 mM MgCl2; 1 mM ATP) ( and ). The calculated Ki values of the kinetic activity of the isolated C unit in the presence of the increasing concentrations of the monovalent ions were not statistically different among all tested samples, as well as from the Ki values of the basic kinetic AC activity (data not shown). Moreover, the stimulation of the C unit activity by 50 μM forskoline in the presence of Ca2+, calmodulin, and the increasing concentrations of the investigated monovalent ions demonstrated no effect on the inhibitory graphic trend for the kinetic values of C unit activity (1 mM MgCl2; 1 mM ATP; results not shown). The values for Ki did not express any statistically significant difference between the control sample of the C unit and the control sample of the AC complex (data not shown).
The effect of the monovalent ions on the GTPase activity of G-protein
Tested monovalent salts were without any effect on the basal [0.5 μM (γ-32P) GTP; 1 mM MgCl2] and stimulated (0.1 mM l-isoproterenol) GTPase activity of the G-protein of the AC complex in the rat brain cortex synaptic membranes (data not shown).
Discussion
Some previous reports have discussed the possible mechanism of the AC activity stimulation and inhibition by inorganic salts. Here, we examine the effect of the monovalent inorganic salts including NaCl, KCl, RbCl and choline-Cl on both the basic and stimulative activity of the AC complex. The demonstrated inhibitory effect of all investigated monovalent ions strictly depended on the concentrations of the present Mg2+ ion. Only in the presence of 1 mM MgCl2 (1 mM ATP), the increasing concentrations of all investigated monovalent ions exhibited the significantly inhibitory effect by 20–60 % on the basic AC activity, starting from the concentration of 30 mM (). The obtained linearization values for Ki on the Dixon’s graph were not significantly different among all tested samples and the average value for Ki was 27 mM (). However, in the presence of the higher concentrations of Mg2+ ions (10 and 100 mM MgCl2; 1 mM ATP) the investigated monovalent ions used in the concentrations from 1 to 200 mM did not demonstrate any significant effect on the basic AC complex activity (data not shown). Furthermore, the sole removal of Ca2+ (the effect of EGTA), the removal of both calmodulin and Ca2+ ions (the washed membranes), the addition of the activated calmodulin and the stimulation by forskoline in the presence of the increasing concentrations of the monovalent ions did not show any significant effect on the kinetic parameters of the activity of both AC complex and the isolated C unit (1 mM MgCl2; 1 mM ATP) (data not shown). Moreover, the above-mentioned substances affected neither Km nor Ki parameters for ATP. Thus, the obtained results suggested that all investigated monovalent ions in the presence of 1 mM Mg2+ (1 mM ATP) accomplished their inhibitory effect on the activity of both AC complex and the C unit, acting not directly upon the C unit of AC complex, but rather distally from it. Importantly, only the stimulation of the AC complex by 5 μM GTPτS together with the rising concentrations of all tested monovalent ions exhibited the highly significant inhibitory effect on the AC activity. The addition of 5 μM GTPτS to the AC complex required the presence of the higher concentrations of the investigated monovalent ions for the inhibition of the AC activity compared to their inhibitory effect in the absence of GTPτS (). The calculated inhibitory constants for all investigated salts showed no significant difference among all tested samples with the average value of Ki = 17 mM (data not shown). Moreover, the obtained Ki value of the GTPτS stimulated AC activity was significantly lower in comparison to the average Ki value of both basic and stimulated AC and C unit activities that was Ki = 27 mM (data not shown). However, the rising concentrations of the monovalent ions in the presence of 0.5 μM GTPτS did not exhibit any significant effect on the activity GTPase G/F protein of the AC complex (). It is well known that the effect of GTPγS induces the dissociation of α subunit of G/F protein and its interaction with C unit of the AC complexCitation17. Therefore, our results suggest that the investigated monovalent ions exert their inhibitory effect on the AC complex activity by affecting the intermolecular interaction of the activated α subunit of G/F protein and the C unit of AC complex (). The number of studies explored the effect of the monovalent ions on the AC complex activity. Most of these studies have been performed on the membrane models of the peripheral tissues, and used various forms of the anion and the cation monovalent inorganic saltsCitation21,Citation25,Citation30,Citation38. In addition, for the effect of the monovalent salts on the AC activity, some authors attributed exclusively to the cation, while the others to the anion component of the monovalent saltsCitation20,Citation25,Citation30,Citation39 results reveled that all examined monovalent salts (NaCl, KCl, RbCl, choline-Cl) showed the uniform inhibitory effect on the AC activity independently of the different incubation conditions. Therefore, from all the obtained results it was difficult to conclude whether the inhibitory effect of the used monovalent salts aroused from the cation or the anion component of the salts.
From the previous knowledge about the effects of the cation on the activity of the other membrane enzymes, such as Na, K-ATPaseCitation40 and F− anions on the AC complex activityCitation32, it could be presumed that the resulted inhibitory effect of the investigated monovalent salts on both the AC activity and the isolated C unit (1 mM MgCl2; 1 mM ATP) in the synaptic complex of the rats brain cortex occurred due to the anion component of the salts, Cl− ionsCitation20. Thus, it could be suggested that Mg2+ ions present in the high concentrations (10–100 mM MgCl2) mimic the effect of the active α−subunit of G/F protein on the activity of the C unit of the AC complex.
Declaration of interest
The authors report no conflict of interest.
References
- Dempski RE, Friedrich T, Bamberg E. Voltage clamp fluorometry: combining fluorescence and electrophysiological methods to examine the structure-function of the Na(+)/K(+)-ATPase. Biochim Biophys Acta 2009;1787:714–720.
- Gadsby DC, Takeuchi A, Artigas P, Reyes N. Review. Peering into an ATPase ion pump with single-channel recordings. Philos Trans R Soc Lond, B, Biol Sci 2009;364:229–238.
- Katzman R, Pappius HM. (1973). Brain electrolytes and fluid metabolism. Baltimor: Williams and Wilkins Co, 175–189.
- Krnjevic K. Chemical nature of synaptic transmission in vertebrates. Physiol Rev 1974;54:418–540.
- Rigoard P, Buffenoir-Billet K, Giot JP, d’Houtaud S, Delmotte A, Lapierre F. [Anatomic bases of surgical approaches to the nerves of the lower limb: tips for young surgeons]. Neurochirurgie 2009;55:375–383.
- Phillis JW. Na+/K(+)-ATPase as an effector of synaptic transmission. Neurochem Int 1992;20:19–22.
- Abashidze S, Jariashvili T, Kometiani Z. The effect of EGTA and Ca++ in regulation of the brain Na/K-ATPase by noradrenaline. BMC Biochem 2001;2:8.
- Stojanovic T, Djuricic BM, Mrsulja BB. The effect of physostigmine on (Na+ + K+)-ATPase activity in different rat brain regions. Experientia 1980;36:1348–1350.
- Strittmatter S, Neer EJ. Properties of the separated catalytic and regulatory units of brain adenylate cyclase. Proc Natl Acad Sci USA 1980;77:6344–6348.
- Miyamoto E, Kuo JF, Greengard P. Cyclic nucleotide-dependent protein kinases. 3. Purification and properties of adenosine 3′,5′-monophosphate-dependent protein kinase from bovine brain. J Biol Chem 1969;244:6395–6402.
- Sadana R, Dessauer CW. Physiological roles for G protein-regulated adenylyl cyclase isoforms: insights from knockout and overexpression studies. Neurosignals 2009;17:5–22.
- Mitterauer T, Hohenegger M, Tang WJ, Nanoff C, Freissmuth M. The C2 catalytic domain of adenylyl cyclase contains the second metal ion (Mn2+) binding site. Biochemistry 1998;37:16183–16191.
- Hu B, Nakata H, Gu C, De Beer T, Cooper DM. A critical interplay between Ca2+ inhibition and activation by Mg2+ of AC5 revealed by mutants and chimeric constructs. J Biol Chem 2002;277:33139–33147.
- Tesmer JJ, Sunahara RK, Gilman AG, Sprang SR. Crystal structure of the catalytic domains of adenylyl cyclase in a complex with Gsalpha.GTPgammaS. Science 1997;278:1907–1916.
- Chen K, Wang JJ, Yung WH, Chan YS, Chow BK. Excitatory effect of histamine on neuronal activity of rat globus pallidus by activation of H2 receptors in vitro. Neurosci Res 2005;53:288–297.
- Willoughby D, Cooper DM. Organization and Ca2+ regulation of adenylyl cyclases in cAMP microdomains. Physiol Rev 2007;87:965–1010.
- Willoughby D, Cooper DM. Ca2+ stimulation of adenylyl cyclase generates dynamic oscillations in cyclic AMP. J Cell Sci 2006;119:828–836.
- Yovell Y, Kandel ER, Dudai Y, Abrams TW. A quantitative study of the Ca2+/calmodulin sensitivity of adenylyl cyclase in Aplysia, Drosophila, and rat. J Neurochem 1992;59:1736–1744.
- Cooper DM, Schell MJ, Thorn P, Irvine RF. Regulation of adenylyl cyclase by membrane potential. J Biol Chem 1998;273:27703–27707.
- Deterre P, Gozlan H, Bockaert J. GTP-dependent anion-sensitive adenylate cyclase in snail ganglia potentiation of neurotransmitter effects. J Biol Chem 1983;258:1467–1473.
- Leclerc L, Harding SE, Harris P. The effect of anions and cations on cardiac adenylate cyclase: interactions with isoprenaline and GTP. Biochem Biophys Res Commun 1984;118:561–566.
- Watson EL, Jacobson KL, Singh JC. Monovalent ion enhancement of beta-adrenergic-stimulated adenylate cyclase activity in mouse parotid gland. Biochem Pharmacol 1989;38:1069–1074.
- Yuji O, Tsukasa K, Shigehiro M, Itaru Y. Effects of lithium on the β-adrenergic receptor adenylate cyclase system in rat cerebral cortical Membranes, Japan. J Pharmacol 1991;55:407–414.
- Seifert R. Monovalent anions differentially modulate coupling of the beta2-adrenoceptor to G(s)alpha splice variants. J Pharmacol Exp Ther 2001;298:840–847.
- Svoboda M, Robberecht P, Christophe J. Deactivation of persistently activated pancreatic adenylate cyclase. Evidence of uncoupling of hormone receptors and enzyme effector in the persistently activated state, and of the presence of two guanyl nucleotide regulatory sites. FEBS Lett 1978;92:351–356.
- Bockaert J, Cantau B, Sebben-Perez M. Hormonal inhibition of adenylate cyclase. A crucial role for Mg2+. Mol Pharmacol 1984;26:180–186.
- Steer ML, Wood A. Inhibitory effects of sodium and other monovalent cations on human platelet adenylate cyclase. J Biol Chem 1981;256:9990–9993.
- Blume AJ, Boone G. Effective coupling of opiate receptors to adenylate cyclase: Requirement for nucleoside triphosphates and monovalent cations. Fed Proc 1979;38:628.
- Mørk A, Geisler A. Mode of action of lithium on the catalytic unit of adenylate cyclase from rat brain. Pharmacol Toxicol 1987;60:241–248.
- Katz MS, Kelly TM, Piñeyro MA, Gregerman RI. Anions and cations as stimulators of liver adenylate cyclase. Biochim Biophys Acta 1980;632:11–25.
- Litvin TN, Kamenetsky M, Zarifyan A, Buck J, Levin LR. Kinetic properties of “soluble” adenylyl cyclase. Synergism between calcium and bicarbonate. J Biol Chem 2003;278:15922–15926.
- Toyoshige M, Basi NS, Rebois RV. Chloride effects on Gs subunit dissociation. Fluoroaluminate binding to Gs does not cause subunit dissociation in the absence of chloride ion. J Biol Chem 1996;271:8791–8795.
- Brostrom CO, Brostrom MA, Wolff DJ. Calcium-dependent adenylate cyclase from rat cerebral cortex. Reversible activation by sodium fluoride. J Biol Chem 1977;252:5677–5685.
- Bradham LS, Hegazy MG. Separation of the catalytic and stimulatory regulatory subunits of rat brain adenylate cyclase. J Cyclic Nucleotide Protein Phosphor Res 1983;9:331–340.
- Gurd JW, Jones LR, Mahler HR, Moore WJ. Isolation and partial characterization of rat brain synaptic plasma membranes. J Neurochem 1974;22:281–290.
- Geisler A, Klysner R, Thams P, Christensen S. A simple and inexpensive protein binding assay for cyclic AMP in biological materials. Acta Pharmacol Toxicol (Copenh) 1977;40:365–368.
- Limbird LE, Connolly TM, Sweatt JD, Uderman HD. Human platelet alpha 2-adrenergic receptors: effect of Na+ on interaction with the adenylate cyclase system and on epinephrine-stimulated platelet secretion. Adv Cyclic Nucleotide Protein Phosphorylation Res 1985;19:235–242.
- Jakobs KH, Minuth M, Aktories K. Sodium regulation of hormone-sensitive adenylate cyclase. J Recept Res 1984;4:443–458.
- Pedemonte CH, Beaugé L. Effects of ATP and monovalent cations on Mg2+ inhibition of (Na,K)-ATPase. Arch Biochem Biophys 1986;244:596–606.
- Cassel D, Levkovitz H, Selinger Z. The regulatory GTPase cycle of turkey erythrocyte adenylate cyclase. J Cyclic Nucleotide Res 1977;3:393–406.