Abstract
One of the many control mechanisms of serine proteinases is their specific inhibition by protein proteinase inhibitors. An extract of Acacia schweinfurthii was screened for potential serine proteinase inhibition. It was successfully purified to homogeneity by precipitating with 80% (v/v) acetone and sequential chromatographic steps, including ion-exchange, affinity purification and reversed-phase high performance liquid chromatography. Reducing sodium dodecyl sulphate polyacrylamide gel electrophoresis conditions revealed an inhibitor (ASTI) consisting of two polypeptide chains A and B of approximate molecular weights of 16 and 10 kDa, respectively, and under non-reducing conditions, 26 kDa was observed. The inhibitor was shown to inhibit bovine trypsin (Ki of 3.45 nM) at an approximate molar ratio of inhibitor:trypsin (1:1). The A- and B-chains revealed complete sequences of 140 and 40 amino acid residues, respectively. Sequence similarity (70%) was reported between ASTI A-chain and ACTI A-chain (Acacia confusa) using ClustalW. The B-chain produced a 76% sequence similarity between ASTI and Leucaena leucocephala trypsin inhibitor.
Introduction
Proteinases are the enzymes that catalyse the hydrolytic cleavage of specific peptide bonds in their target proteins. These enzymes are widely distributed in nearly all plants, animals and microorganisms and are very important for the maintenance and survival of their host organism and play key roles in many biological processes. The proteolytic events catalysed by these enzymes serve as mediators of signal initiation, transmission and termination in many of the cellular events, such as inflammation, apoptosis, blood clotting and hormone processing pathwaysCitation1.
Despite the fact that these enzymes are indispensable to the cells and organisms that host them, they may be potentially damaging when overexpressed or present in high concentrations. For this reason, the activities of these enzymes need to be strictly regulated and controlled. The synthesis of these enzymes as inactive pre-proteins, and their substrate specificity exert a control on their activities, but it does not fulfil the desired level of regulation, and the fact remains, that cells and organisms require additional means of controlCitation2.
One important control mechanism involves interaction of the active enzymes with proteins that inhibit their activities. These inhibitors form less active or fully inactive complexes with their cognate enzymes. Interest in understanding the physiological significance of proteinase inhibitors (PIs) has increased due to their regulatory action in different processes related to the proteinase-PI balance. The PIs are important tools to achieve a better understanding of fundamental principles of protein interaction and can be used to design new substances for the control of diseases and pathologic processes. PIs have drawn the attention of many researchers due to their potential medical value, for example, human immunodeficiency virus (HIV) PIs and severe acute respiratory syndrome (SARS) coronavirus PIs may be used to combat HIV and SARS virus, respectivelyCitation3.
Most PIs interact with their target proteinases by contact with the active (catalytic) site of the proteinase, resulting in the formation of a stable PI complex that is incapable of enzymatic activityCitation4. With the exception of macroglobulin (720 kDa), which inhibits proteinases of all classes, individual protein inhibitors frequently inhibit proteinases belonging to a single mechanistic class, although some inhibitors inhibit two different classes of enzymesCitation5–7. Of these inhibitors, the most studied class are the inhibitors of serine (Ser) proteinases. These inhibitors are also very unique in that they retain their inhibitory activity after replacement of their reactive site residue (P1) by another residueCitation8.
PIs of plants are proteins that are natural, defence-related proteins, often present in seeds and induced in certain tissues by herbivory or wounding. Thus, their main function is thought to be in plant defence, the regulation of endogenous proteinases, as storage proteins, as well as the prevention of unwanted proteolysisCitation8,Citation9. Plant PIs are shown to also possess anti-insect and anti-fungal activities, and have been reported to inhibit a variety of Ser proteinases, including enzymes of the blood coagulation cascade. They may also be involved in the regulation of programmed cell death in plantsCitation10. Among the seed legumes, the Ser proteinase Bowman–Birk inhibitor and Kunitz trypsin inhibitor (KTI) have been studied extensively. These families differ from each other in size, cysteine content and the number of reactive sites. KTIs are proteins (18--24 kDa), with one or two polypeptide chains and low Cys content, usually with four Cys residues connected by two disulphide bridges and a single reactive siteCitation3.
Since the isolation of soybean trypsin inhibitor (SBTI) by KunitzCitation11, it has been found that PIs are widely distributed in seeds of the Leguminosae. Thus, Acacia schweinfurthii (AS) var schweinfurthii belonging to the family, Leguminosae, and sub family Mimosoideae, indigenous to South Africa, was chosen for this study. Seeds from this plant served as a source of potential inhibitors of Ser proteinases. We report in this paper the purification and characterization of an inhibitor from AS seeds.
Materials and methods
Materials
AS seeds were purchased from Kirstenbosch botanical garden (Cape Town, South Africa). Trypsin, chymotrypsin and trypsin-agarose resin were purchased from Sigma Aldrich (St. Louis, MO), as well as the substrates Z-Gly-Pro-Arg-pNA and N-Suc-Ala-Ala-Pro-Phe-pNA. Toyopearl Super-Q 650 S and Toyo Soda Kogyo octadecylsilica (TSK ODS) column were from Tosoh (Tokyo, Japan). Molecular weight markers were from Optima Scientific (Cape Town, South Africa). All other reagents were of the highest analytical grade available.
Preparation of crude extract
Seeds were milled into a fine powder with a grinder. Twenty grams of the milled seeds were extracted in 200 mL 0.15 M NaCl as described by Joubert et al.Citation12. The proteins were extracted by homogenizing for approximately 10 s. The extract was stirred slowly overnight at 4 °C, followed by centrifugation at 5000 × g for 15 min at 4 °C and the supernatant was kept for further analysis. Proteins were re-extracted from the pellet as described above and the supernatants were combined (crude extract). Proteins in the supernatant were precipitated with 80% (v/v) acetone at 4 °C. Protein precipitates were recovered by centrifugation at 5000 × g for 10 min at 4 °C. The pellets were re-suspended in water and lyophilized for further studies.
Purification by chromatography
Protein from the acetone precipitated material (20 mg) was loaded at 5 mL/h onto an ion exchange chromatography (IEC), Toyopearl Super Q column (29 × 1.6 cm), equilibrated with 0.05M Tris-HCl buffer, pH 7.5. After extensive washing at 40 mL/h, bound material was eluted with a linear NaCl gradient (0–0.6 M) (total volume, 200 mL), followed by 1 M NaCl to remove any bound material left on the column. Fractions (3 mL) were collected and the absorbances at 280 nm were monitored. The peaks that contained inhibitory activity were pooled and a Sephadex G-10 column (64 × 1.6 cm) was used to desalt the fractions, equilibrated with 0.5M ammonium acetate buffer, pH 8. The sample was loaded at 10 mL/h and chromatography was performed at 40 mL/h. Fractions (3 mL) were collected and their absorbance monitored at 280 nm. A trypsin-agarose resin (1.5 mL column) was equilibrated with 10 mM acetic acid, pH 3.2. Protein was applied at 0.5 mL/min and the column was washed with distilled H2O, and eluted with 20 mM HCl, with the absorbance at 280 nm being monitored. Fractions (1 mL) were collected. AS inhibitor was further purified by reversed-phase high performance liquid chromatography (RP-HPLC)Citation13 using a Grace Vydac C-18 RP column. The column was equilibrated with 0.1% trifluoroacetic acid (TFA)/water and eluted using an acetonitrile gradient (0–20%, 5 min; 20–60%, 35 min; 60–100%, 15 min) in 0.1% TFA at a flow rate of 1.0 mL/min. Fractions were collected and freeze dried.
Protein quantification and SDS-PAGE analysis
The protein content of the various fractions was determined by using the bicinchoninic acid (BCA) protein determination assay according to the method of Smith et al.Citation14. The purity and molecular weight (MW) of ASTI were determined using sodium dodecyl sulphate polyacrylamide gel electrophoresis (SDS-PAGE) (15% resolving gel) as described by LaemmliCitation15, under reducing and non-reducing conditions. Peq GOLD Protein-Marker II was used as MW markers (10--200 kDa) and bands were visualized by staining with Coomassie brilliant blue R-250.
Amino acid sequence and phylogenetic analysis
Purified, intact ASTI was subjected to reduction, alkylation and RP-HPLCCitation16, allowing the two subunits to be separated. S-carboxamidomethylated (CAM)-ASTI (A-chain) (0.5 mg) was digested separately with endoproteinase Arg-C (5 µg, S/E = 100:1) and Achromobacter protease I (10 µg, S/E = 50:1) in 0.5 mL 0.1 M Tris-HCl buffer (pH 7.6) containing 10 mM CaCl2 at 37 °C for 16 h. CAM-ASTI (A-chain) (0.5 mg) was also digested with Staphylococcus aureus V8 protease (10 µg, S/E = 50:1) in 0.5 mL 20 mM Tris-HCl buffer (pH 8.0) at 37 °C for 16 hCitation17. Each digest was separated by RP-HPLC on a TSK gel ODS 120 T column (5 μm, 4.6 × 250 mm) using a gradient of acetonitrile in 0.1% TFA. The amino acid sequences of intact subunits and peptides were determined by a gas-phase protein sequencer (PPSQ-10; Shimadzu, Japan)Citation17. Sequence similarities between ASTI and other trypsin inhibitors were performed using basic local alignment search tool (BLAST) available on the National Center for Biotechnology Information (NCBI) website (http://blast.ncbi.nlm.nih.gov/). Multiple sequence alignment was obtained using ClustalW2 (http://www.ebi.ac.uk/Tools/msa/clustalw2/), and the phylogenetic tree was viewed using TreeView.
Molecular mass measurements of proteolytic products
The molecular masses of proteolytic products resulting from fragmentation of ASTI were measured by matrix-assisted laser desorption ionization time of flight (MALDI-TOF) mass spectrometry (Voyager–DETM STR, Applied Biosystems, Carlsbad, CA)Citation17.
Trypsin and chymotrypsin inhibition assays
The inhibitory effects of ASTI on bovine trypsin and chymotrypsin were analysed using Z-Gly-Pro-Arg-pNA and N-Suc-Ala-Ala-Pro-Phe-pNA as substrates, respectively. The effect of two substrate concentrations with bovine trypsin, in the presence of varying concentrations of ASTI was studied. The assays were performed according to the method of Smith et al.Citation18 using 50 mM Tris-HCl, pH 8.2, containing 10 mM CaCl2, and the absorbance was recorded at 412 nm. The modes of inhibition and the inhibition constants were calculated using double Dixon and enzyme-inhibitor molar ratio plots. For both assays, ASTI was incubated with the active enzyme for 3 min before the substrate was added.
Results and discussion
Purification of AS inhibitor
The past few decades have seen a growing interest in the identification, purification and characterization of novel PIs from various sources, because of their potent activity in preventing carcinogenesis in a wide range of in vivo and in vitro systems, and their use in developing pest resistance in otherwise susceptible plantsCitation5,Citation19. ASTI was purified to a final homogeneous product via a few chromatographic steps. The acetone fraction of the inhibitor was subjected to IEC (), affinity chromatography () and RP-HPLC (), yielding the active inhibitor, fraction ASb2b (ASTI). The four-step purification procedure of ASTI is shown in . A 7.2-fold purification of the inhibitor was achieved with a specific activity of 1.0 U/mg protein. From the purification table, it can be concluded that the inhibitor constituted 8% of the total trypsin inhibitory activity of the seeds of AS.
Figure 1. (a) Toyopearl Super-Q chromatography of acetone extract of AS (15 mg). The column (29 × 1.6 cm) was equilibrated with 0.05M Tris-HCl buffer, pH 8.0 and a linear NaCl gradient (0–0.6 M) in 0.05 M Tris-HCl buffer was used for elution. Flow rate was 0.6 mL/min. ASa: tubes 5–12, ASb: tubes 110–135 and ASc: tubes 143–155. Approximately 95% inhibition of trypsin was observed for fraction ASb and less than 10% was observed for ASa and ASc. (b) Affinity chromatography of fraction ASb using a trypsin-agarose column (1 mL). The column was equilibrated with 10 mM acetic acid and 20 mM HCl was used for elution. Flow rate was 0.5 mL/min. ASb1: tubes 2–5, ASb2: tubes 8–11. (c) RP-HPLC of fraction ASb2 (500 µg) on a Grace Vydac C-18 RP column. The column was equilibrated with 0.1% (v/v) TFA/water. Elution was performed with an acetonitrile gradient at a flow rate of 1 mL/min.
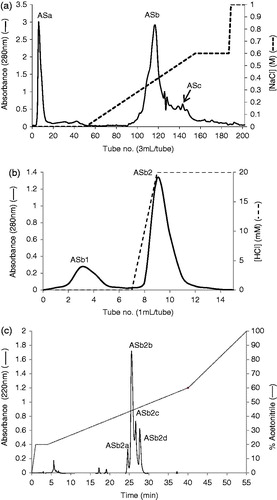
Table 1. Purification table of ASTI from 5 g starting material.
Molecular mass estimation and homogeneity of inhibitor
Electrophoresis was performed under reducing conditions and ASTI was identified as two molecular weight bands of approximately 10 and 16 kDa (). Under non-reducing conditions, one band was visible, indicating an intact protein with a molecular weight of 26 kDa (Figure not shown). It can be concluded from the results that the inhibitor consisted of two subunits, possibly linked by disulfide bonds. An inhibitor from Acacia confusaCitation20 displayed two subunits under reducing SDS-PAGE conditions (15 and 6 kDa) and a single band (21 kDa) under non-reducing conditions. Similar results (i.e. a two subunit inhibitor) have been published for other Acacia species. Kortt and JermynCitation21 purified an inhibitor from Acacia elata seeds and obtained two subunits, 15 kDa for the A-chain and 5 kDa for the B-chain, with a single component under non-reducing SDS-PAGE conditions (20 kDa).
Primary structure
The enzymatic digests of CAM-ASTI (A-chain) with S. aureus V8 protease, endoproteinase Arg-C and Acromobacter protease I were separated by RP-HPLC, yielding E1–E7, R1–R8 and K1–K7 (, respectively). Amino acid sequencing and MALDI-TOF mass spectrometry of the peptide fragments derived from the three enzymatic digestions allowed the determination of the complete amino acid sequence of ASTI, shown in . The sequences of the A- and B-chain revealed 140 and 40 amino acids, respectively. There are five positions showing two amino acid residues, namely positions 52, 62, 67, 70 and 122 (). The two residues at the first four positions are accounted for via the following sequences: E6: TQNGMPAKIWTPPRIGFLS; E7: QQNGMPAKIWSPPRIAFLS; R8: IGFLTPAFYLNIEFQPR; K7: IWSPPRIGFLSPAFYLNIEFQPRDPPSCL; and for position 122: K3: IMPYRDDYK; R2: IKPYR; E5: DRVFGPFKIMPYRDDYKIVYCE. The presence of more than one residue at certain positions in the ASTI A-chain, referred to as a multiplicity of inhibitor molecular forms, may be ascribed to isoforms. Several studies on Ser PIs have confirmed this findingCitation20. The sequences of K5 and R5 showed that endoproteinase Arg-C may also cleave Lys residues. Arg 65 is the amino acid at the P1 position of the reactive site of ASTI (Arg 65-Ile 66), being a characteristic of a trypsin inhibitorCitation22. ClustalW was used to obtain a sequence comparison with other similar inhibitors from plant seeds (). Sequence identity of approximately 70% was calculated between the A-chains of ASTI and ACTICitation22. Comparing the B-chain sequences of ASTI and Leucaena leucocephala trypsin inhibitor (LLTI), a 76% similarity was obtained. Taking into consideration the molecular weight of intact ASTI from SDS-PAGE analysis (26 kDa), the low Cys content (four Cys residues) and the molecule being a two chain structure, ASTI can be considered to be a Kunitz-type inhibitorCitation23. It has similar properties to a complete Kunitz-type trypsin inhibitor alpha chain, which contains only three Cys residues and thus also making it a Kunitz-type inhibitor, as well as 140 amino acid residues of the A-chain of ASTI, a characteristic of Kunitz inhibitors. ACTICitation22 displayed a high sequence identity to the N-terminal sequence of ASTI (84% comparing residues 1–50), and it is also a Kunitz-type inhibitor. Kortt and JermynCitation21 isolated two trypsin inhibitors from A. elata seeds, being homogeneous as judged by gel electrophoresis at pH 4.3 and 8.8, and both inhibitors co-eluted from a Sephadex G-100 column and had the same molecular weight of 25 kDa. It can thus be concluded that properties of ASTI are similar to those of inhibitors from comparable species. The phylogenetic comparison of ASTI with other trypsin inhibitors () showed similarities to other closely related inhibitors, such as ACTI and LLTI. These results were also confirmed when related to the multiple sequence alignments ().
Figure 3. (a) Separation of peptides generated by digestion of ASTI A-chain with S. aureus V8 protease. Peptides were separated by RP-HPLC on a TSK gel ODS 120 T column using a gradient of acetonitrile in 0.1% TFA. Flow rate was 1.0 mL/min. (b) Separation of peptides generated by digestion of ASTI A-chain with endoproteinase Arg-C. Peptides were separated by RP-HPLC on a TSK gel ODS 120 T column using a gradient of acetonitrile in 0.1% TFA. Flow rate was 1.0 mL/min. (c) Separation of peptides generated by digestion of ASTI A-chain with Achromobacter protease I. Peptides were separated by RP-HPLC on a TSK gel ODS 120 T column using a gradient of acetonitrile in 0.1% TFA. Flow rate was 1.0 mL/min.
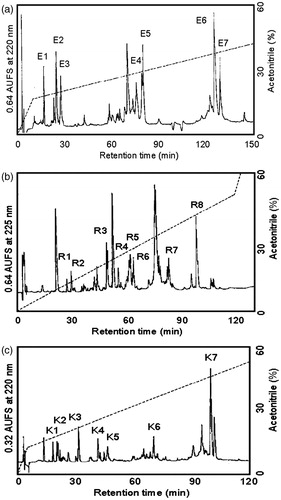
Figure 4. Amino acid sequence of the A- and B-chain of ASTI, deduced from protease digestions, RP-HPLC and sequence analysis. (E), S. aureus V8 protease digest of A-chain; (K), Achromobacter protease 1 digest of A-chain and (R), endoproteinase Arg-C digest of A-chain.
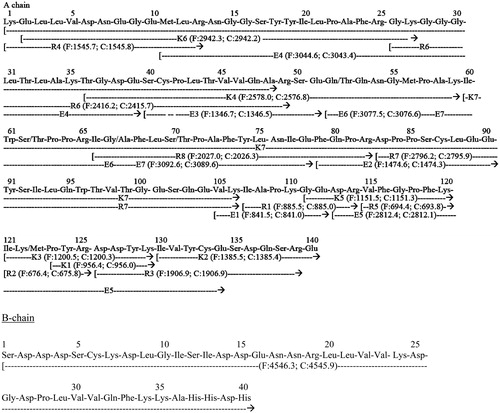
Figure 5. (a) Alignment between the sequence of the A-chain of ASTI with other comparative plant seed inhibitor sequences obtained from ClustalW search. Comparative sequences are obtained with Kunitz-type trypsin inhibitor A chain, ACTI-A (A. confusa), gi|299509|; Kunitz-type trypsin inhibitor A-chain, LLTI-A (L. leucocephala), gi|18202442|; trypsin inhibitor A-chain, BVTI-A (Bauhinia variegata), gi|15082208| and KTI A-chain, KTI-A gi|162138868|. (*), Identical sequences; (:), similarities of three or four amino acids. (b) Alignment between the sequence of the B-chain of ASTI with other comparative plant seed inhibitor sequences obtained from ClustalW search. Comparative sequences are obtained with Kunitz-type trypsin inhibitor B-chain, ACTI-B (A. confusa), gi|299508|; SBTI and Kunitz-type trypsin inhibitor B-chain, LLTI-B (L. leucocephala), gi|18202443|. (*), Identical sequences; (:), similarities of three or four amino acids.
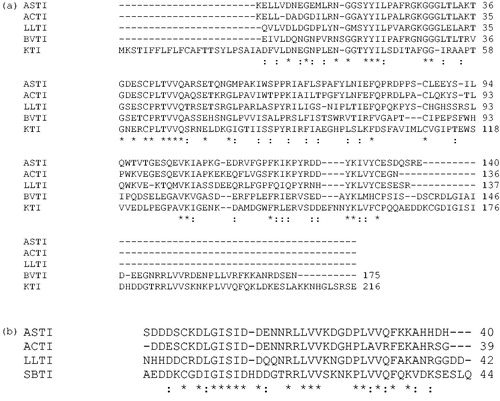
Figure 6. A phylogenetic tree analysis of ASTI with other inhibitors. Protein sequences were obtained from http://blast.ncbi.nlm.nih.gov/ by a BLAST search of ASTI. Accession numbers for the sequences used are as follows: trypsin isoinhibitor (Adenanthera pavonina), APTI, gi|225058|; LLTI, gi|18202442|; Prosopis juliflora trypsin inhibitor, PJTI, gi|417176|; ACTI, gi|299509|; Tamarindus indica trypsin inhibitor, TITI, gi|308756025|; BVTI, gi|32363181|; Bauhinia ungulate Factor X inhibitor, BUXI, gi|32363179|; Psophocarpus tetragonolobus trypsin inhibitor, PTTI, gi|86450987 and chymotrypsin inhibitor (Erythrina variegate), gi|265716|. The scale bar shows a branch length of 0.1 (i.e. a 10% difference in amino acids).
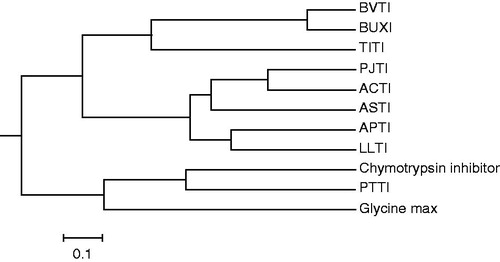
Inhibition of serine proteinases
For ASTI a Ki of 3.45 × 10−9 M was obtained with bovine trypsin (). The Ki value obtained for ASTI can be compared with that of a trypsin inhibitor purified from Entada scandens seeds (Ki of 4.9 × 10−9 M)Citation3, indicating a very strong and potent inhibitor. The Ki value of SBTI interacting with bovine trypsin is in the range of 10−9 MCitation24, similar to a Ki of 3.5 × 10−9 M obtained (result not shown). The results suggest a high affinity of ASTI, as well as SBTI, for trypsin. The molar ratio of ASTI: enzyme showed a value of 0.8:1 for near complete inhibition of trypsin (result not shown). The Ki value reported for ACTI was 0.294 × 10−9 MCitation20. The latter result indicates that ACTI has a stronger binding affinity than ASTI.
Figure 7. Double–Dixon plot to determine the inhibition constant (Ki) of ASTI interacting with bovine trypsin. Substrate concentrations of 25 and 5 mM were used. Bovine trypsin concentration was 1 nM. 25 mM (♦) (R2 = 0.913) and 5 mM (R2 = 0.920) (▴).
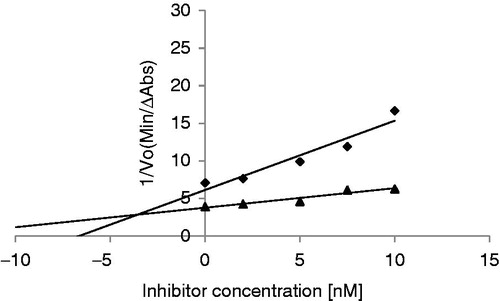
Chymotrypsin inhibition was determined for ASTI and at an enzyme: inhibitor molar ratio of 1:1 weak/insignificant inhibition was observed (result not shown). The latter result was also observed with inhibitors purified from seeds from Mimosoideae families, where inhibitors of trypsin were found to also weakly inhibit chymotrypsinCitation21 and with inhibitors purified from A. elata seedsCitation21, A. confusa seedsCitation20 and E. scandens seedsCitation3. However, recently Lam and NgCitation25 purified a 70 kDa chymotrypsin inhibitor from A. confusa seeds that had no trypsin inhibitory activity and was highly potent in inhibiting HIV-1 reverse transcriptase.
Conclusion
The results revealed that we purified a new PI from seeds of the Leguminosae, and in terms of the physicochemical and kinetic properties, ASTI can be considered a Kunitz-type trypsin inhibitor. In ongoing experiments the effect of ASTI on the blood coagulation system is studied.
Declaration of interest
This work was supported by the National Research Foundation (South Africa), the Hungarian Science Foundation (Hungary), the FAPESP (09/53766-9) (Brazil) and the Nelson Mandela Metropolitan University.
References
- Ivanov D, Emonet C, Foata F, et al. A serpin from the gut bacterium Bifidobacterium longum inhibits eukaryotic elastase-like serine proteases. J Biol Chem 2006;281:17246–52
- Habib H, Fazili KM. Plant protease inhibitors: a defense strategy in plants. Biotech Mol Biol Rev 2007;2:68–85
- Lingaraju MH, Gowda LR. A Kunitz trypsin inhibitor of Entada scandens seeds: another member with single disulfide bridge. Biochim Biophys Acta 2008;1784:850–5
- Birk Y. Plant protease inhibitors – significance in nutrition, plant protection, cancer prevention and genetic engineering. Berlin: Springer Publishers; 2003
- Oliva MLV, Silva MCC, Sallai RC, et al. A novel subclassification for Kunitz proteinase inhibitors from leguminous seeds. Biochim 2010;92:1667–73
- De Oliveira C, Navarro-Xavier RA, Anjos-Vallota EA, et al. Effect of plant neutrophil elastase inhibitor on leucocyte migration, adhesion and cytokine release in inflammatory conditions. Br J Pharmacol 2010;161:899–910
- Oliva ML, Sampaio UM. Bauhinia Kunitz-type proteinase inhibitors: structural characteristics and biological properties. Biol Chem 2008;389:1007–13
- Laskowsky MJ, Kato I. Protein inhibitors of proteinases. Ann Rev Biochem 1980;49:593–626
- Konarev AV, Griffin J, Konechnaya GY, Shewry PR. The distribution of serine protease inhibitors in seeds of the Astridae. Phytochemistry 2004;65:3003–20
- Ee KY, Zhao J, Rehman A, Agbool S. Characterisation of trypsin and α-chymotrypsin inhibitors in Australian wattle seed (Acacia victoriae Bentham). Food Chem 2008;107:337–43
- Kunitz M. Crystalline soybean trypsin inhibitor. J Gen Physiol 1947;30:291–310
- Joubert FJ, Merrifield EH, Dowdle EBD. The reactive sites of proteinase inhibitors from Erythrina seeds. Int J Biochem 1987;19:601–6
- Paiva PMG, Oliva MLV, Fritz H, et al. Purification and primary structure determination of two Bowman-Birk type trypsin isoinhibitors from Cratylia mollis seeds. Phytochemistry 2006;67:545–52
- Smith PK, Krohn RI, Hermanson GT, et al. Measurement of protein using bicinchoninic acid. Anal Biochem 1985;150:76–85
- Laemmli UK. Cleavage of structural proteins during the assembly of the head of baceriophage T4. Nature 1970;227:680–5
- Kabuto S, Ogawa T, Muramoto K, et al. The amino acid sequence of pancreatic alpha-amylase from the ostrich, Struthio camelus. Comp Biochem Physiol Part B 2000;127:481–90
- Terada T, Watanabe Y, Tateno H, et al. Structural characterization of a rhamnose-binding glycoprotein (lectin) from Spanish mackerel (Scomberomorous niphonius) eggs. Biochim Biophys Acta 2007;1770:617–29
- Smith N, Naudé RJ, Oelofsen W. Kinetic and thermodynamic characterization of ostrich (Struthio camelus) trypsins and chymotrypsins. Int J Biochem 1993;25:367–72
- Schuler TH, Poppy GM, Kerry BR, Denholm I. Insect-resistant transgenic plants. Trends Biotechnol 1998;16:168–75
- Lin J-Y, Chu S-C, Wu H-C, Hsieh Y-S. Trypsin inhibitor from the seeds of Acacia confusa. J Biochem 1991;110:879–83
- Kortt AA, Jermyn MA. Acacia proteinase inhibitors – purification and properties of the trypsin inhibitors from Acacia elata seeds. Eur J Biochem 1981;115:551–7
- Wu H-C, Lin J-Y. The complete amino acid sequence of a Kunitz family trypsin inhibitor from seeds of Acacia confusa. J Biochem 1993;113:258–63
- Richardson M. The proteinase inhibitors of plants and micro-organisms. Phytochemistry 1977;16:159–69
- Oliva MLV, Oliva V, Roberto CS, et al. Bauhinia serine proteinase inhibitors: effect on factor X, factor XII and plasma kallikrein. lmmunopharmacology 1996;32:85–7
- Lam SK, Ng TB. A dimeric high-molecular-weight chymotrypsin inhibitor with antitumor and HIV-1 reverse transcriptase inhibitory activities from seeds of Acacia confusa. Phytomedicine 2010;17:621–5