Abstract
Inhibitory effects of some synthesized dihydroxycoumarin compounds on purified G6PD were investigated. For this purpose, initially human erythrocyte G6PD was purified 7069-fold in a yield of 33.6% by using ammonium sulfate precipitation and affinity chromatography which includes 2′,5′-ADP Sepharose 4B. The purified enzyme showed a single band on sodium dodecyl sulfate-polyacrylamide gel electrophoresis (SDS-PAGE). Enzyme activity was determined spectrophotometrically according to Beutler method at 340 nm. 6,7-Dihydroxy-3-(2-methylphenyl)-2H-chromen-2-one (OPC), 6,7-dihydroxy-3-(3-methylphenyl)-2H-chromen-2-one (MPC) and 6,7-dihydroxy-3-(4-methylphenyl)-2H-chromen-2-one (PPC) were used as dihydroxycoumarin compounds. This study has demonstrated that G6PD activity is very highly sensitive to study coumarin derivatives.
Introduction
Glucose-6-phosphate dehydrogenase (G6PD, EC 1.1.1.49) is the first enzyme of the pentose phosphate pathway (PPP) that converts β-D-glucose-6-phosphate (G6P) to 6-phosphoglucono-δ-lactone with the reduction of NADP+ to NADPHCitation1. PPP is the only source of NADPH in the erythrocytes. The formation of NADPH in erythrocytes has vital importance. The major role of NADPH in erythrocytes is regeneration of reduced glutathione (GSH), which prevents hemoglobin denaturation, preserves the integrity of red blood cell membrane sulfydryl groups and detoxifies hydrogen peroxide and oxygen radicals in and on the red blood cells. G6PD deficiency significantly reduces the production of NADPH and this situation blocks the GSH formation. With the GSH deficiency, free radicals cannot be reduced and causes hemolysis of erythrocytes.
Natural products have been important sources of new pharmacological active agents. Coumarins (2H-chromen-2-ones, 2H-1-benzopyran-2-ones) are among the best known oxygen heterocyclics with an δ-lactone ring and comprise a very large class of compounds found throughout the plant kingdomCitation2. A wide spectrum of biochemical and pharmacological activities are displayed by coumarins and their derivativesCitation3,Citation4. Coumarin derivatives are used widely as anticoagulants (such as warfarin, –OH group is attached at the 4th position) for the treatment of disorders in which there is excessive or undesirable clotting, such as thrombophlebitis, pulmonary embolism and certain cardiac conditions. They are also used as rodenticides due to their ability to cause fatal hemorrhagingCitation5. In recent years, increasing human population leads to the development and variation of a lot of industry such as cosmetics, drugs and food. It is extremely important to investigate the effects of coumarin type compounds on the activity of enzymes which are important for physiological functions, especially in metabolism.
The effect of coumarins on different enzyme activities such as carbonic anhydrase (CA) and paraoxonase (PON) has been investigatedCitation6–9. However, no reports could be found in the literature on the effects of coumarin derivatives on human G6PD enzyme.
Thus, the aim of this study was mainly to design, synthesize and investigate in vitro inhibitory effects of these compounds (OPC, MPC and PPC) that may be in interaction with human body throughout medicinal treatment on purified enzyme.
Materials and methods
2′,5′-ADP-Sepharose 4B was purchased from Pharmacia. NADP, glucose-6-phosphate, protein assay reagents and chemicals for electrophoresis and synthesis were obtained from Sigma (St. Louis, MO) and Aldrich (Germany). The structure of all synthesized dihydroxy coumarin compounds were identified from their IR (Perkin Elmer Spectrum BX II) and 1H-NMR spectra (Bruker GmbH DPX-400). Melting points were measured on an Electrotermal 9200 instrument. 6,7-dihydroxy coumarin compounds, 6,7-dihydroxy-3-(methylphenyl)chromenones, were synthesized according to literatureCitation10.
General procedure for the synthesis of 6,7-dihydroxy-3-(methylphenyl)chromenones
A solution of 2,3,4-trimethoxybenzaldehyde (25.0 mmol) and methylphenylacetonitrile (37.5 mmol) in ethanol (100 mL) was heated to 70 °C. Twenty percent NaOH solution was then added dropwise to the stirred solution until the onset of turbidity. The acrylonitrile was precipitated by cooling the solution to room temperature. The precipitate was filtered, washed with water and dried. Pyridine hydrochloride (100.0 mmol) was added to the crude product and it was heated for 2 h at 180 °C. After cooling, the product was crystallized from water. The precipitated coumarin was washed with water until neutral, dried and recrystallized from ethanol. The yield, melting point and NMR spectra of dried product were determined ().
6,7-Dihydroxy-3-(2-methylphenyl)-2H-chromen-2-one (OPC)
Yield: 76.5%; mp 242 °C; IR (KBr), ν (cm−1): 3464(OH), 1655(C=O), 1297(C–H), 1151(C–O). 1H-NMR (CDCl3 +DMSO), δ (ppm): 2.20 (s, 3H, CH3), 6.83 (s, H, ArH), 6.98 (s, H, ArH), 7.32 (m, 4H, ArH), 7.50 (s, H, cumH), 7.86 (s, 2H, OH).
6,7-Dihydroxy-3-(3-methylphenyl)-2H-chromen-2-one (MPC)
Yield: 76.8%; mp 211 °C; IR (KBr), ν (cm−1): 3172(OH), 1669(C=O), 1258(C–H), 1168(C–O). 1H-NMR (CDCl3 +DMSO), δ (ppm): 2.32 (s, 3H, CH3), 6.75 (s, H, ArH), 6.91 (s, H, ArH), 7.08 (d, H, ArH), 7.21 (d, H, ArH), 7.37 (d, 2H, ArH), 7.70 (s, H, cumH), 7.75 (s, 2H, OH).
6,7-Dihydroxy-3-(4-methylphenyl)-2H-chromen-2-one (PPC)
Yield: 80.5%; mp 257–258 °C; IR (KBr), ν (cm−1): 3151(OH), 1660(C=O), 1273(C–H), 1188(C–O). 1H-NMR (CDCl3 +DMSO), δ (ppm): 2.30 (s, 3H, CH3), 6.75 (s, H, ArH), 6.90 (s, H, ArH), 7.13 (d, 2H, ArH), 7.48 (d, 2H, ArH), 7.67 (s, H, cumH), 7.73 (s, 2H, OH).
Purification of G6PD enzyme from human erythrocytes by affinity chromatography
Appropriate amount of fresh blood samples from human volunteers were collected in the tubes containing EDTA and sample tubes were centrifuged at 3000 × g for 10 min. The plasma and leukocyte coat were removed. The packed erythrocytes were washed with isotonic KCl solution three times, and hemolysate with five volumes of ice-cold water and then centrifuged at 15 000 g for 20 min at 4 °C to remove the ghost and intact cellsCitation11. The hemolysate was subjected to precipitation with ammonium sulfate (30–70%). Ammonium sulfate was slowly added to hemolysate for completely dissolution. This mixture was centrifuged at 15 000 × g for 30 min. The precipitate was dissolved in 50 mM potassium phosphate buffer (pH: 7.0) and then dialyzed at 4 °C in 50 mM potassium acetate/50 mM potassium phosphate buffer (pH: 7.0) at overnight. All of the purification procedures were performed at 4 °C. Two grams of dry 2′,5′-ADP-Sepharose 4B was used for a 10-mL column volume. The gel was washed with 400 mL distilled water to remove foreign bodies and air bubbles of swollen gel were eliminated. The gel was resuspended in 0.1 M potassium acetate/0.1 M potassium phosphate buffer (pH: 6.0) and packed in a column (1 x 10 cm) and equilibrated with the same buffer. The dialyzed enzyme solution was loaded onto the 2′,5′-ADP-Sepharose 4B column was then sequentially washed with 25 mL of 0.1 M potassium acetate/0.1 M potassium phosphate buffer (pH: 6.0) and 25 mL of 0.1 M potassium acetate/0.1 M potassium phosphate buffer (pH: 7.85). The final washing with 0.1 M potassium chloride/0.1 M potassium phosphate buffer (pH: 7.85) was continued until the final absorbance difference became about 0.05 at 280 nm. The enzyme was eluted with a solution of 80 mM potassium phosphate + 80 mM potassium chloride + 0.5 mM NADP+ + 10 mM EDTA (pH: 7.85) and 1 mL fractions were collected. The enzyme activity was measured in the final fractions and the activity-containing tubes were pooledCitation12,Citation13.
G6PD enzyme assay and protein determination
G6PD enzyme activities of synthesized dihydroxy coumarin compounds were measured spectrophotometrically at 340 nm by Beutler’s methodCitation6. One enzyme unit was defined as the enzyme amount that reduces 1 µmole of NADP+ per min under the assay conditions. This method depends on the reduction of NADP+ by G6PD in the presence of G6P. The activity measurements were made by monitoring the increase in absorbance at 340 nm due to the reduction of NADP+ at 25 °C. Quantitative protein determination was performed at 600 nm according to Lowry’s methodCitation14 using bovine serum albumin (BSA) as a standard. Protein samples were fractionated on 12% SDS-PAGE gelCitation15 using a Minigel system. Gel was stained with Coomassie Brilliant Blue R-250, and destained using standard methods to detect protein bands.
Determination of kinetic parameters and in vitro inhibition studies
To obtain Km and Vmax values, various final concentration of G6P (0.06–1.2 mM) and NADP + (0.02–0.3 mM) were used. All kinetic studies were performed at 25 °C and optimum pH (1 M Tris-HCl, pH:8.0). Inhibition experiments were done using G6P as substrate and a group of dihydroxy coumarin derivatives (OPC, MPC and PPC) with different final concentration as possible inhibitors. Kinetic parameters were calculated from Lineweaver–Burk graphs. G6PD activity without a coumarin derivative was accepted as 100% activity. For the coumarin compounds having an inhibition effect, the inhibitor concentration causing up to 50% inhibition (I50 values) were determined by regression analysis graphs drawn by using a Microsoft Excel 2000 computer program. In addition, Ki values of coumarins were calculated on G6PD activity.
Results and discussion
Pentose phosphate metabolic pathway involves the conversion of glucose into pentose sugars, necessary for various biosynthetic reactions. Produced pentose sugars are required as precursors in the biosynthesis of a number of important molecules, such as ATP, CoA, NAD, FAD, RNA and DNA. Pentose phosphate metabolic pathway also provides a form of NADPH which is a reducing power in various biosynthesis reactions.
G6PD is an enzyme which catalyzes the first reaction in the PPPCitation16,Citation17. The activity of G6PD changes depending on nutrition, hormones and especially the concentration of NADPHCitation18. Although there are a lot of research reports about enzyme deficiency in erythrocyte cellsCitation19,Citation20, G6PD deficiency which is concerning to all human biology is yet to be investigatedCitation21.
For the solution to the growing number of health problems, various drugs from plants are produced. But to meet to needs of drugs, a large number of chemical substances synthesized in the laboratories are used as pharmaceutical active ingredient. In this way, the use of drugs obtained from various sources causes the problem of side effects. Many chemicals at relatively low dosages affect the metabolism of biota by altering normal enzyme activity, particularly inhibition of a specific enzymeCitation22. The effects can be dramatic and systematicCitation23.
Coumarins are active components of herbs used for the treatment of various diseasesCitation24. The diverse biological activities of natural and synthetic coumarin derivatives as anticoagulants and antithrombotics are well knownCitation25. Some of the coumarin derivatives are also reported anti-HIV agentsCitation26, lipid lowering agentsCitation27 and antioxidantsCitation28. They have also been shown to inhibit lipid peroxidation and to possess vasorelaxantCitation29, anti-inflammatoryCitation30, and anticarcigonenic activitiesCitation31.
In the literature, inhibition effects of some coumarin derivatives on the various enzymes were investigated but there are not any study related to G6PD enzyme. In a study, two coumarin-based sulfamate drugs (667 COUMATE and STX 118) have been reported to have IC50 values of 25–59 nM for the inhibition of hCA-II activityCitation6. Inhibition of some important enzymes, which play a key role in a metabolic pathway, may lead to pathologic conditions or disorders. These stimulated us to investigate inhibitory effects of a number of dihydroxy coumarin compounds on human erythrocyte G6PD enzyme.
In this study, G6PD was purified from human erythrocytes by ammonium sulfate precipitation and 2′,5′-ADP-Sepharose 4B affinity chromatography, respectively. Purification factor for ammonium sulfate precipitation and affinity chromatography were 1.2- and 7068.9-fold in a yield of 68.18% and 33.65%, respectively. shows the SDS-PAGE gel for the determination of purity of the enzyme in the study. A high purity was obtained for the enzymes.
Figure 1. SDS-PAGE of purified human erythrocyte G6PD. The enzyme was electrophoresed at pH: 8.3 on a 12% polyacrylamide gel and stained with Coomassie Brilliant Blue R-250. Lanes: 1, molecular weight standards (β-galactosidase, 116 kDa; bovine serum albumin, 66.2 kDa; egg albumin, 45 kDa; lactate dehydrogenase, 35 kDa; Rease Bsp981 (Escherichia coli), 25 kDa; β-lactoglobulin, 18.4 kDa; Lysozyme, 14.4 kDa; 2, purified human erythrocyte G6PD).
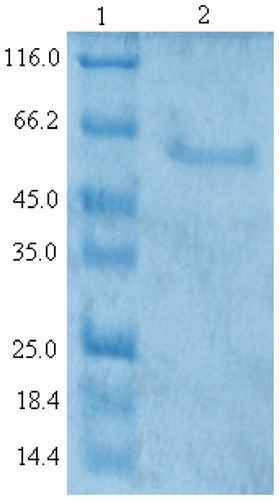
The reaction kinetics of the purified G6PD was determined from Lineweaver–Burk plots using glucose-6-phosphate and NADP+ as substrates with Km values of 0.22 and 0.14 mM and Vmax values of 1.94 and 2.76 U/mg, respectively (). Affinity of the enzyme for NADP+ was considerably higher than for glucose-6-phosphate. The higher G6PD affinity for NADP+ has also been reportedCitation32.
Table 1. Kinetic parameters of human erythrocyte glucose-6-phosphate dehydrogenase.
In the literature, effects of various drugs and chemical substances on the catalytic activity of the G6PD enzyme were investigated. Ki values of these substances are higher than the values calculated for the coumarin derivatives. Ki values of isepamicin sulfate, omepirazol, morphine sulfate, vankomycine, magnesium sulfate, metamizol and granisetron hydrochloride were reported as 1.7 mM, 8.2 mM, 25.9 mM, 2.71 mM, 13.2 mM, 6.3 mM, 4.5 mM, respectivelyCitation33–36.
In this study, for investigation of inhibition effects, IC50 and Ki parameters of coumarin derivatives () for G6PD were determined. IC50 values of OPC, MPC and PPC were 0.305 mM, 0.769 mM and 0.790 mM (, ), and the Ki constants were 1.37 mM, 0.734 mM–0.269 mM, 0.835 mM (, ), respectively.
Figure 3. Lineweaver–Burk graphs in five different substrate (G6P) concentrations sand in two different OPC, MPC and PPC concentrations for determination of Ki.
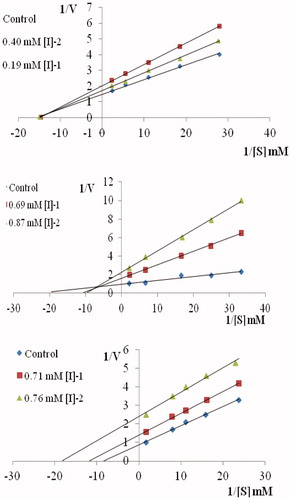
Table 2. Chemical structures of the synthesized coumarins.
Table 3. Inhibition type, Ki and I50 values obtained from regression analysis graphs for G6PD in the presence of coumarin derivatives.
I50 values showed the same trend for MPC and PPC and higher than OPC. These results show that MPC and PPC are weak inhibitors on G6PD enzyme than OPC. OPC, MPC and PPC compounds inhibited G6PD enzyme activity in a noncompetitive, mixed and uncompetitive manner respectively (). Differences in chemical structures of coumarins also lead to different types of inhibition. As shown in the , the chemical structures of coumarin derivates are similar to each other. The only difference is due to the different positions of methyl groups on phenyl ring. Methyl group is located in the 2nd position in OPC, 3rd position in MPC and 4th position in PPC. This situation may be because OPC connects to the enzyme from a different region than the active region. The presence of methyl group in position 3 instead of 2 influences the mechanism of enzyme action. Therefore, MPC can be connected to the enzyme in both active and inactive region. Methyl group is located in 4th position in the structure of PPC because it only binds to the enzyme when the enzyme–substrate complex occurs. Our results indicate that studied coumarin derivatives have more potent inhibitory effect on the G6PD enzyme.
Declaration of interest
The authors report no conflicts of interest. The authors alone are responsible for the content and writing of this article.
The financial support of Scientific Research Council of Balikesir University (Project No: 2009/20) is gratefully acknowledgement.
References
- Pilz RB, Wills RC, Boss GR. The influence of ribose 5-phosphate availability on purine synthesis of cultured human lymphoblasts and mitogen-stimulated lymphocytes. J Biol Chem 1984;259:2927--35
- Murray RD, Mendez J, Brown SA. The natural coumarins: occurrence, chemistry and biochemistry. London, UK: John Wiley & Sons; 1982
- Khan KM, Saify ZS, Khan MZ, et al. Synthesis of coumarin derivatives with cytotoxic, antibacterial and antifungal activity. J Enzyme Inhib Med Chem 2004;19:373--9
- Rehman SU, Chohan ZH, Gulnaz F, Supuran CT. In-vitro antibacterial, antifungal and cytotoxic activities of some coumarins and their metal complexes. J Enzyme Inhib Med Chem 2005;20:333--40
- Budzisz E. Synthesis, reactions and biological activity of phosphorus-containing derivatives of chromone and coumarin. Phosphorus Sulfur Silicon 2004;179:2131--47
- Leese MP, Leblond B, Smith A, et al. 2-substituted estradiol bis-sulfamates, multitargeted antitumor agents: synthesis, in vitro SAR, protein crystallography, and in vivo activity. J Med Chem 2006;49:7683--96
- Maresca A, Temperini C, Vu H, et al. Non-zinc mediated inhibition of carbonic anhydrases: coumarins are a new class of suicide inhibitors. J Am Chem Soc 2009;131:3057–62
- Maresca A, Temperini C, Pochet L, et al. Deciphering the mechanism of carbonic anhydrase inhibition with coumarins and thiocoumarins. J Med Chem 2010;53:335–44
- Erzengin M, Basaran I, Cakir U, et al. In vitro inhibition effect of some dihydroxy coumarin compounds on purified human serum paraxonase 1 (PON1). Appl Biochem Biotechnol 2012;168:1540–8
- Basaran I, Sinan S, Cakir U, et al. In vitro inhibition of cytosolic carbonic anhydrases I and II by some new dihydroxycoumarin compounds. J Enzyme Inhib Med Chem 2007;23:32--6
- Beutler E. Red cell metabolism: a manual of biochemical methods. 1st ed. New York: Grune & Stratton, Inc; 1971:92–4
- Ninfali P, Orsenigo T, Barociani L, Rapa S. Rapid purification of glucose-6-phosphate dehydrogenase from mammal’s erythrocyte. Prep Biochem 1990;20:297--309
- Şentürk M, Ceyhun SB, Erdoğan O, Küfrevioğlu İÖ. In vitro and in vivo effects of some pesticides on glucose-6-phosphate dehydrogenase enzyme activity from rainbow trout (oncorhynchus mykiss) erythrocytes. Pesticide Biochem Physiol 2009;95:95--9
- Lowry OH, Rosebrough NJ, Farr AL, Randall RJ. Protein measurement with the Folin phenol reagent. J Biol Chem 1951;193:265--75
- Laemmli UK. Cleavage of structural proteins during the assembly of the head of bacteriophage T4. Nature 1970;227:680--5
- Mehta A, Mason PJ, Vulliamy TJ. Glucose-6-phosphate dehydrogenase deficiency. Best Pract Res Clin Haematol 2000;13:21--38
- Shannon WN, Gover S, Lam MVS, Adams MJ. Human glucose-6-phosphate dehydrogenase: the crystal structure reveals a structural NADP+ molecule and provides insights into enzyme deficiency. Structure 2000;8:293--303
- Ayala A, Fabregat I, Machado A. The role of NADPH in the regulation of glucose-6-phosphate and 6-phosphogluconate dehydrogenases in rat adipose tissue. Mol Cell Biochem 1991;105:7--13
- Valentine WN, Tanaka KR, Paglia DE. Hemolytic anemias and erythrocyte enzymopathies. Ann Intern Med 1985;103:245--57
- Beutler E. Glucose-6-phosphat dehydrogenase deficiency and other enzyme abnormalities. In: Beutler E, Lichtman MA, Coller BS, Kipps TJ, eds. Williams hemotology. NewYork: McGraw-Hill Company; 1995:564
- Beutler E. G6PD deficiency. Blood 1994;84:3613--36
- Hochster RM, Kates M, Quastel, JH. Metabolic inhibitors. New York: Academic Press; 1972:3:71, 1973:4:66
- Christensen GM, Olson D, Riedel B. Chemical effects on the activity of eight enzymes: a review and a discussion relevant to environmental monitoring. Environ Res 1982;29:247--55
- Okamoto T, Kobayashi T, Yoshida S. Chemical aspects of coumarin compounds for the prevention of hepatocellular carcinomas. Curr Med Chem-Anti-Cancer Agents 2005;5:47--51
- Murray RDH, Mendez J, Brown SA. Natural coumarins: occurrence, chemistry and biochemistry. New York: Wiley; 1982
- Spino C, Dodier M, Sotheeswaran S. Anti-HIV coumarins from Calophyllum seed oil. Bioorg Med Chem Lett 1998;8:3475--8
- Madhavan GR, Balraju V, Mallesham B, et al. Novel coumarin derivatives of heterocyclic compounds as lipid-lowering agents. Bioorg Med Chem Lett 2003;13:2547--51
- Kontogiorgis C, Hadjipavlou-Litina D. Biological evaluation of several coumarin derivatives designed as possible anti-inflammatory/antioxidant agents. J Enzyme Inhib Med Chem 2003;18:63--9
- Hoult JRS, Paya M. Pharmacological and biochemical actions of simple coumarins: natural products with therapeutic potential [review]. Gen Pharmac 1996;27:713--22
- Khan MY, Sharma P. Synthesis of new α-pyronoflavones and related products. Indian J Chem 1993;32B:817--21
- Kempen I, Papapostolou D, Thierry N, et al. 3-Bromophenyl 6-acetoxymethyl-2-oxo-2H-1-benzopyran-3-carboxylate inhibits cancer cell invasion in vitro and tumour growth in vivo. Br J Cancer 2003;88:1111--18
- Özmen İ, Çiftçi M, Küfrevioğlu Öİ, Çürük MA. Investigation of the mutation points and effects of some drugs on glucose-6-phosphate dehydrogenase-deficient people in the Erzurum region. J Enzyme Inhib Med Chem 2004;19:45–50
- Özmen İ, Küfrevioğlu Öİ, Gul M. Effects of some antibiotics on activity of glucose-6-phosphate dehydrogenase from human erythrocytes in vitro and effect of isepamicin sulfate on activities of antioxidant enzymes in rat erythrocytes. Drug Chem Toxicol 2005;28:443--52
- Özdemir H, Çiftçi M. In vitro effects of some drugs on human red blood cell glucose-6-phosphate dehydrogenase enzyme activity. J Enzyme Inhib Med Chem 2006;21:75--80
- Çiftçi M, Özmen İ, Büyükokuroğlu ME, et al. Effects of metamizol and magnesium sulfate on enzyme activity of glucose 6-phosphate dehydrogenase from human erythrocyte in vitro and rat erythrocyte in vivo. Clin Biochem 2001;34:297--302
- Özmen İ, Küfrevioğlu Öİ. Effect of antiemetic drugs on glucose 6-phosphate dehydrogenase and some antioxidant enzymes. Pharmacol Res 2004;50:499--504