Abstract
Carbonic anhydrase IX (CA IX) is selectively expressed in a range of hypoxic tumours and is a validated endogenous hypoxia marker with prognostic significance; hence, CA IX is of great interest as a molecular imaging target in oncology. In this review, we present an overview of the different imaging agents and imaging modalities that have been applied for the in vivo detection of CA IX. The imaging agents reviewed are all entries in the Molecular Imaging and Contrast Agent Database (MICAD) and comprise antibody, antibody fragments and small molecule imaging agents. The effectiveness of these agents for imaging CA IX in vivo gave variable performance; however, a number of agents proved very capable. As molecular imaging has become indispensable in current medical practice we anticipate that the clinical significance of CA IX will see continued development and improvements in imaging agents for targeting this enzyme.
Molecular Imaging and Contrast Agent Database
The Molecular Imaging and Contrast Agent Database (MICAD) is a collection of data comprising molecular imaging and contrast agents that have in vivo animal or human data published in peer-reviewed scientific journalsCitation1,Citation2. MICAD is part of the National Institute of Health (NIH) Common Fund and aims to promote research using molecular imaging probes and to facilitate the translation of preclinical findings to clinical practiceCitation1–3. As of March 2013 there were ∼1400 agents listed in MICAD. Of this, the majority were for positron emission tomography (PET) (41%), followed by single-photon emission computed tomography (SPECT) (29%), optical imaging (14%), magnetic resonance imaging (MRI) (11%), ultrasound (2%), multimodal imaging (1%), X-ray/computer tomography (X-ray/CT) (1%) and photoacoustic imaging (1%).
Cancers are traditionally staged and monitored using invasive methods, for example, biopsies or exploratory surgery, characterizing treatment response by a change in tumour volumeCitation4. These methods are limited, however, as biochemical changes happen much faster than morphological ones. Molecular imaging allows a repeated in vivo measurement of several critical molecular features such that molecular imaging has become indispensable in current medical practiceCitation4. Of the MICAD entries, ten agents target carbonic anhydrase IX (CA IX) (). CA IX is overexpressed in several solid cancer types in hypoxic conditions, while expression in normal tissues is minimal. CA IX is thus considered a marker for tumour hypoxia as it provides differentiation between normal and hypoxic tissue, allowing the opportunity for selective imaging of solid tumours. This article presents the ten CA IX imaging agents in MICAD and outlines their effectiveness for imaging CA IX in vivo.
Table 1. Agents listed in the MICAD that target CA IX.
CA IX as a tumour marker
CA IX is a dimeric zinc metalloenzyme that catalyses the reversible hydration of CO2 to and H+ to play an important role in pH homeostasisCitation24. Each CA IX monomer is comprised of an intracellular domain, a single transmembrane helix domain, an extracellular proteoglycan domain and an extracellular catalytic domainCitation25,Citation26. CA IX is overexpressed in several solid cancer types, including cervical, clear cell renal cell, pancreatic, breast, bladder, head and neck, soft tissue and non-small cell lung carcinomaCitation8,Citation12. In solid tumours, hypoxia inducible factor 1 (HIF-1) regulates a signalling cascade involving ∼100 genes, including CA IXCitation7, to enable adaptive cellular functions to support tumour cell survival, proliferation and metastasis in hypoxiaCitation27. The extracellular pH (pHe) of tumour cells is lower than in normal tissue owing to elevated metabolism leading to increased production and export of acidic metabolites such as lactic acidCitation22. At a molecular level, CA IX overexpression leads to dramatically increased production of
and H+ at the extracellular surface of hypoxic tumour cells. The
is actively transported into the cell to buffer intracellular pH (pHi) while the H+ contributes to acidosis of the extracellular spaceCitation28 to support invasion and metastasisCitation27. The proteoglycan domain makes CA IX unique among CAs, and is proposed to assist CA IX to function efficiently at a more acidic pHCitation25,Citation26.
Solid tumour masses are heterogeneous and show significant variation in oxygen concentrationsCitation18,Citation29. These heterogeneously distributed areas of hypoxia are referred to as the “hypoxic fraction” and arise from the insufficient organization of blood vessels within the solid tumoursCitation30. Hypoxic tumours have a high metastatic potential associated with a more aggressive phenotype, this results in a resistance to radio- and chemotherapy and therefore a poor outcome for the patientCitation4,Citation13,Citation29,Citation31,Citation32. Gray et al.Citation33 reported that tumour cells lacking oxygen were resistant to death via radiation by 2.5- to 3-fold compared to fully oxygenated cellsCitation29,Citation33. These results were obtained separately by other groupsCitation34. Hypoxia has been established as a key feature that can significantly influence tumour behaviour and predict a response to therapyCitation4,Citation29,Citation32. There are several invasive and non-invasive methods available for the detection of tumour hypoxia, but these are not fully reliableCitation4,Citation32. These techniques either yield a variable diagnosis or have functional limitations due to the incomplete penetration of the tumours or resistance to some tumour typesCitation13,Citation18,Citation32. For example, hypoxia can be determined post-mortem in animal models given pimonidazole by intravenous administration 30 min prior to sacrifice. Pimonidazole is a 2-nitroimidazole compound that is reduced in hypoxia, the reduced form can react with and stain intracellular macromolecules in hypoxic regions to provide generic, but selective hypoxia detectionCitation18,Citation35. Co-localization analysis between pimonidazole staining and ex vivo CA IX expression detected by monoclonal antibodies (mAbs) to CA IX has yielded conflicting results, this has been attributed to different levels of oxygen at which pimonidazole is reduced or CA IX overexpressed, respectively; local temporal fluctuations in hypoxia level; or non-hypoxia-related factors that affect CA IX expressionCitation16. For in vivo hypoxia measurements, an invasive oxygen electrode can be used but this is not practical for all cancer types and causes significant patient discomfort. CA IX offers several features that make it a promising clinically relevant candidate for diagnostic imagingCitation29,Citation31. Firstly, the active site domain is extracellular and can be targeted by imaging agents that do not have to transverse the cell membraneCitation29, and secondly, it is expressed in few normal tissues but is overexpressed to high concentrations in hypoxic conditions with a long half-lifeCitation7,Citation31. It has been postulated that while some cell lines may subvert known hypoxia mechanisms and that CA IX is not overexpressed in all cancer cell types, a vast array of human solid tumours typically overexpress CA IXCitation7,Citation31.
Antibody and antibody fragment imaging agents that target CA IX
G250 and cG250 mAbs and antibody fragments, A3 and CC7 mAbs
mAbs are a homogenous population of immunoglobulins (e.g. IgG) directed against a single epitopeCitation36. G250 is a mAb that was isolated >20 years ago and the antigen identified much later as an epitope of CA IXCitation6,Citation37,Citation38. cG250 is a chimeric form of G250 that was developed to be less immunogenic than G250 while retaining the CA IX antigen specificity (Ka = 4 × 109 M−1)Citation6,Citation7,Citation10. Antibody fragments have several advantages over parent antibodies in the IgG format for molecular imaging owing to altered physicochemical properties. This includes more rapid clearance from the blood and normal tissues, reduced residence time, a reduction in the radiation absorbed by the bone marrow, improved tumour penetration – particularly into hypoxic tumour regions, and potentially reduced immunogenicityCitation39. The increased clearance of fragments has several caveats as the absolute tumour uptake may be lower while non-specific uptake by other organs may be higherCitation12,Citation39. In 2009, Neri and colleaguesCitation18 reported the generation and characterization of two high-affinity human mAbs specific to extracellular CA IX, named A3 and CC7. These are the first fully human mAbs with high affinity (nanomolar) for CA IX, importantly they do not inhibit CA IX activity and specificity to CA IX over other forms of CA was also confirmedCitation18. Due to limitations in antibody diffusion within solid tumour massesCitation40–42, A3 and CC7 antibodies in the small immunoprotein (SIP) format were prepared with a lower molecular weight (∼76 kDa) cf. A3 and CC7 when in the IgG format (∼150 kDa). Researchers have undertaken work using these mAbs and mAb fragments conjugated to a range of clinically used radionuclides for imaging CA IX using PET and SPECT ().
Table 2. PET and SPECT radionuclides combined with antibodies and antibody fragments for imaging CA IX.
Directly radiolabelled CA IX antibodies
With direct radiolabelling the mAb structure remains virtually unchanged and retains high specificity for the target antigen. Direct iodination of mAbs with 131I, 124I and 125I is relatively easy to perform and provides high specific activitiesCitation45. It is typically achieved via the iodogen (1,3,4,6-tetrachloro-3α,6α-diphenyl glycoluril) method, although other methods are availableCitation7,Citation10,Citation46–50. The radioactive iodine is provided via an aqueous solution of NaICitation47. Iodogen, a strong oxidising agent, is used to convert the I− anion to an electrophilic I+ cation, this electrophile then reacts to insert an iodine ortho to the hydroxyl groups of tyrosine residues of the antibody ()Citation48. The iodogen reagent also limits oxidative damage to the protein during radiolabellingCitation47.
Figure 1. Direct iodination of antibodies is typically achieved via the iodogen method to modify a tyrosine residueCitation47,Citation48.
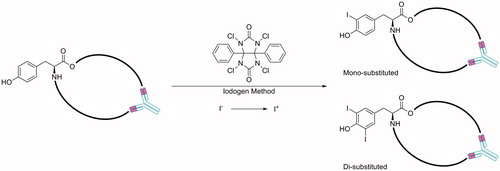
131I has a higher intensity gamma ray when compared to 124I and 125I, it is also widely available, relatively inexpensive and was the first to be established as a radiolabelling agent for cG250Citation44,Citation45,Citation49. 131I was however readily released from the tumour cells following internalization of 131I-cG250 and the abundant high energy gamma photons could affect normal tissues such as the bone marrow, limiting the dose of 131I-cG250 that could be used therapeuticallyCitation49,Citation51. Additionally, the high energy single-photon emission compromises image qualityCitation6. With these drawbacks, research moved to the direct iodination of cG250 using 124I (, entry 1) and 125I (, entry 2)Citation6. 124I, used for PET, has a half-life of ∼4 days, this allows both long term pharmacokinetics to be determined and for centralized production and wide distribution of 124I radiolabelled antibodyCitation6. 125, used for SPECT, I is considered a pure gamma emitter with a low radiation energy and medium range half-life of ∼60 days, whereas 131I is both a beta and gamma emitter with medium range energy and a short range half-life of ∼8 daysCitation52. 125I gives a higher counting efficiency than 131I such that about half the amount of 125I is needed for tracer purposesCitation52.
Renal cell carcinoma (RCC) is the most common malignancy in the kidney and each year in USA ∼39 000 people are diagnosed with and ∼13 000 people die from RCCCitation44. Approximately 80% of RCCs are of the more aggressive clear cell type (ccRCC), of these >95% express the G250/CA IX antigen on the cell surfaceCitation6,Citation7,Citation37,Citation39,Citation44. In RCC, it has been shown that production of CA IX begins after the loss of the tumour suppressor gene VHL (Von Hippel–Lindau protein), whose product is integral to the HIF-1α pathwayCitation7,Citation12,Citation18. This upregulation of CA IX has been determined to be one of the most prominent accessible markers of RCCCitation18. There is debate within the literature regarding the mechanism leading to CA IX overexpression in RCC, irrespective of mechanism, however, the high cell surface production of CA IX renders RCC an excellent model for investigating the properties of CA IX imaging agents.
124I-cG250
Divgi et al.Citation6 carried out the first phase I trial with patients with ccRCC using 124I-cG250 (, entry 1)Citation5 pre-operatively to predict ccRCC, the only human clinical trial in this review. The aim of this research was to determine if PET with 124I-cG250 was able to discern ccRCC from other renal masses. For this clinical trial, 25 patients who were surgical candidates for kidney tumour resection, accrued via one surgeon, were administered with 185 MBq/10 mg of 124I-cG250 over 20 min. PET and CT images were taken 3 h later with positive PET images being indicative of ccRCC. Both PET and CT modalities were used to unequivocally determine tumour location and structure. Out of the 25 patients imaged pre-surgery, 15 gave positive PET images. Following imaging, surgery determined that these 15 patients all had ccRCC. Of the 10 patients with negative PET images there was only one patient surgically determined to have ccRCC.
Animal model studies with 124I-cG250 have been undertaken by Lawrentschuk et al.Citation7 The purpose of this investigation was to identify if there was direct data that linked hypoxia and CA IX expression in RCC. They provided two hypotheses based on the loss of the VHL: (i) that CA IX will reflect hypoxia or (ii) that tumours subvert this pathway with CA IX expression unrelated to hypoxia. SK-RC-52 human RCC xenograft models were established in nude mice. At Day 0, 1, 3, 7 and 10 invasive oxygen electrodes were used to investigate intratumoural hypoxia directly before injection with 5 MBq of 124I-cG250 (25 μg) in 0.2 mL phosphate-buffered saline followed by PET imaging. The PET results showed localization of 124I-cG250 in the tumour as well as the cardiac blood pool. After imaging, the animals were sacrificed and their tumours and organs removed, weighed and biodistribution determined. To investigate CA IX expression, one mouse was taken at 1, 2, 3 and 5 day time points and autoradiography, histology and immunohistochemistry performed. They found no significant correlation between any oxygen parameter and the CA IX expression and concluded that in this model, the level of CA IX expression could not be clearly related to hypoxia with only a weak correlation between hypoxia and the cG250 uptakeCitation7.
125I-mAb
Zhang and colleaguesCitation8 have described a 125I-labelled mAb (125I-mAb) (, entry 2)Citation9 that recognized CA IX without cross-reactivity to other human CAs. A HT-29 xenograft model was established in athymic male mice and an in vivo binding study, biodistribution study and planar imaging were carried out with 125I-mAb. To determine in vivo binding, the mice were injected with 3.70 MBq of 125I-mAb and sacrificed 24 h later. Their blood was collected, the unbound 125I-mAb removed and the activity quantified using a gamma counter. Radioactivity in the blood was high: the radioactivity was 5.5% that of the blood activity at 24 h post-injection (p.i.) which the authors attributed to a small amount of the tracer diffusing into blood cells. For the biodistribution study, 1.295 MBq of 125I-mAb was injected intravenously into mice and the radioactivity of both normal tissues and tumour measured using a gamma counter at a range of time pointsCitation8. Uptake of 125I-mAb by the tumour was significantly higher than for normal tissues, 4.9 ± 1.2 %ID/g (percentage of the injected dose per gram of tissue) at 24 h p.i.Citation8 SPECT imaging, mice were injected with 5.55 MBq of 125I-mAb and after 48 h planar static images (duration 30 s) were acquired using a gamma camera. In parallel, a control study was also undertaken using a 100-fold molar excess of unlabelled mAb injected into the mouse 24 h prior to injection with 125I-mAb. The results showed that the 125I-mAb preferentially concentrated in the HT-29 tumour at 48 h p.i., however, it also accumulated in the thyroid, while in the control study unlabelled mAb blocked accumulation of 125I-mAb in both the tumour and the thyroid. Although the signal to noise ratio was promising, the high levels of radioactivity in the blood make 125I-mAb currently unsuitable for as a diagnostic toolCitation8.
Indirectly radiolabelled CA IX antibodies and antibody fragments
Direct radiolabelling of antibodies with most other radionuclides is not possible and instead an indirect approach with a host-linker moiety is needed. In this indirect labelling method, the host coordinates to the radionuclide while the linker allows covalent attachment to the antibody or antibody fragment with minimal disruption to structure. A summary of host-linkers and their method of attachment to the CA IX antibody component are shown in . Brouwers et al.Citation10 (, entry 7) and Hoeben et al.Citation16 (, entry 3) used a desferrioxamine (Df) backbone as host linker for 89Zr incorporation. Carlin et al.Citation12 employed an amide linkage to 1,4,7,10-tetraazacyclododecane-1,4,7,10-tetraacetic acid (DOTA) for 111In incorporation (, entries 4, 5 and 6). Finally, Neri et al.Citation18 conjugate diethylenetriaminepentaacetic acid (DTPA) via a thiourea linker for radiolabelling with 177Lu (, entry 8).
Figure 2. Host-linker moieties that have been employed to indirectly radiolabel antibodies and antibody fragments targeting CA IX.
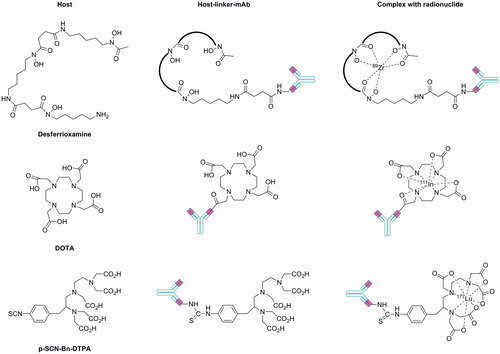
89Zr-Df-cG250
To synthesize 89Zr-Df-cG250 (, entry 3)Citation11, Brouwers et al.Citation10 conjugated the desferrioxamine (as the mesylate salt) host to cG250 via amide bond formation followed by radiolabelling with 165 MBq of 89Zr (). 89Zr was selected as radionuclide because its half-life (3.26 days) is similar to the clearance time of the cG250Citation10. PET imaging with 89Zr-Df-cG250 was used in combination with radioimmunoscintigraphy (RIS) in a SK-RC-52 xenograft rat modelCitation10. Results were compared both to 18F-FDG (18F-fluorodeoxyglucose), the gold standard imaging agent for oncology with PET, and to 111In-DTPA-cG250 for RISCitation10. Eight rats were injected with 20 MBq of 89Zr-Df-cG250 and PET imaging undertaken at 5 min, 24, 48 and 72 h p.i. After imaging, the animals were sacrificed and their organs removed, weighed and the activity of the samples calculated. The PET images showed that 18F-FDG did not accumulate in the tumours, sufficiently for visualization, while at 24 h p.i. 89Zr-Df-cG250 successfully labelled even small (∼100 mg) tumours. The 89Zr-Df-cG250 PET images improved over time with optimal images taken at 72 h p.i.
Figure 3. General synthetic methodology for the production of radiolabelled mAbs using desferrioxamine mesylate (desferal, Df) as the host-linker moietyCitation54.
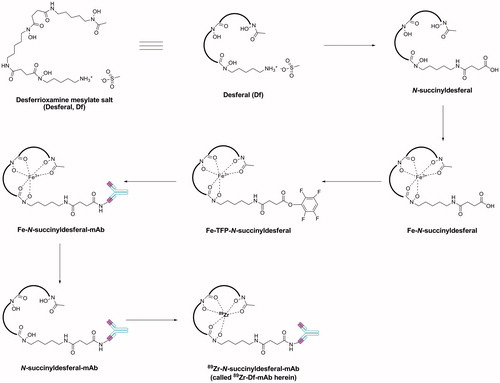
The comparison of biodistribution acquired with 89Zr-Df-cG250 to those acquired with 111In-DTPA-cG250, allowed researchers to conclude that the tumour uptake of 89Zr-Df-cG250 was identical to 111In-DTPA-cG250 (5.0 ± 2.4 and 4.9 ± 2.9%ID/g, respectively) as was the blood levels at the 72 h time point (1.4 ± 0.4 and 1.7 ± 0.7%ID/g, respectively). The uptake of both antibodies was similar in other organs assessed, however, the authors state that in some cases, the PET images produced using 89Zr-Df-cG250 were highly superior to the 111In-Df-cG250 images and therefore this agent may be of value in the planning an monitoring of RCC patientsCitation10. 111In-DTPA-cG250 is not listed as a CA IX targeting agent in MICAD, however, the synthesis and imaging properties in patients with RCC metastases has been reported and showed improved visualization and a higher activity compared with 131I-cG250Citation45. Unlike 131I, 111In is a residualizing radionuclide, that is, one that is retained inside a cell as the radioactive catabolites are trapped in the lysosomesCitation45,Citation53. Based on increasing evidence that cG250 can be internalized by CA IX expressing RCC the use of residualizing radionuclides was suggested as beneficial. Previous studies had shown that labelling cG250 with the residualizing nuclides 88Y and 177Lu had ∼3–4 times the activity of 125I-cG250 at 7 days p.i.Citation45 Due to the similarity between the RIS results for 111In-DTPA-cG250 and 89Zr-Df-cG250 in tumour uptake, blood level and also a similarity amongst other tissues, the authors suggest that 89Zr could be re-classed as a residualizing radionuclideCitation53.
111In-DOTA-cG250, 111In-DOTA-cG250-Fab and 111In-DOTA-cG250-F(ab′)2
To evaluate the potential of cG250 fragments as imaging agents, Carlin et al.Citation12 compared the binding affinity for the CA IX antigen of two low molecular weight fragments of cG250, cG250-Fab and cG250-F(ab′)2, with the parent cG250 (, entries 4, 5 and 6)Citation13–15. The fragments were prepared via enzymatic degradation of the intact cG250 using the hydrolytic enzymes pepsin and papain to give cG250-F(ab′)2 and cG250-Fab, respectivelyCitation12,Citation39. The F(ab′)2 fragment is bivalent (two antigen binding sites), whereas the Fab fragment is monovalent (one antigen binding site)Citation39. cG250, cG250-Fab and cG250-F(ab′)2 were each conjugated to DOTA and then complexed to the residualizing radionuclide 111In ()Citation55. The conjugation occurred in two steps. Firstly, N-hydroxysuccinimide (NHS) and 1-ethyl-3-(3-dimethylaminopropyl)carbodiimide (EDC) are used to synthesize the active monoester of DOTA. Secondly, the DOTA active ester is linked to the mAb or fragment via amide bond formation through a side chain lysine residue on the mAbCitation55. The stoichiometry of incorporated DOTA moieties, binding affinity (Kd) for CA IX expressed by SK-RC-38 cells and the saturation binding data (Bmax) of the mAb and fragments varied ().
Figure 4. Synthetic approach for the production of radiolabelled mAbs and mAb fragments using DOTA as the host-linker moiety.

Table 3. Properties of DOTA-CA IX mAb and mAb fragment conjugatesCitation12.
Athymic male mice with HT-29 tumours were injected with 1.6 MBq of each 111In-DOTA-cG250, 111In-DOTA-cG250-Fab and 111In-DOTA-cG250-F(ab′)2 for in vivo biodistribution studies. 111In-DOTA-cG250 (tested at 2, 4 and 7 days p.i) showed a high uptake of radioactivity into the HT-29 tumour. At 2 days p.i. uptake (20.1 ± 4.8% ID/g) was similar to that obtained with two non-specific 111In-labelled control antibodies, the specificity of the 111In-DOTA-cG250 was however confirmed over the next 5 days as the tumour uptake increased, while uptake of the control antibodies decreased. Compared to 111In-DOTA-cG250, the radiolabelled fragments were tested at 6 and 24 h p.i due to faster clearance and showed a lower absolute tumour uptake. For F(ab′)2 uptake was 7.6 ± 1.4 and 9.3 ± 2.1%ID/g at 6 and 24 h p.i., respectively, while for Fab uptake was lower again (3.6 ± 1.3 and 3.5 ± 1.7%ID/g at 6 and 24 h p.i., respectively) due to a much faster clearance from circulation. Biodistribution was similar for all three 111In-DOTA-antibodies across other organs. The tumour/blood (T/B) and tumour/muscle (T/M) values were compared for all three agents (). Both the F(ab′)2 and the Fab fragments showed an improvement in the T/B and T/M ratios between 6 and 24 h p.i. with the T/B ratio for the Fab fragment increasing dramatically from 2.8 to 16.6, this ratio is higher than for the 111In-DOTA-cG250 at 7 days p.i. ().
Table 4. T/B and T/M ratios for 111In-DOTA-cG250, 111In-DOTA-cG250-Fab and 111In-DOTA-cG250-F(ab′)2 in mice with HT-29 tumoursCitation12.
To correlate 111In-DOTA-cG250 to regions of perfusion, hypoxia and CA IX expression, autoradiography images were compared to fluorescent images of the same tumour section stained with Hoechst 33342, pimonidazole and a CA IX fluorescent antibody at 2 days p.i. of 111In-DOTA-cG250. The Hoescht stain revealed high perfusion around the rim of the tumour and disorganized perfusion at the centre, the anti-correlation of the endogenous CA IX. The regions of CA IX staining co-localized with pimidazole, showing that the CA IX expression was limited to the hypoxic regions but more importantly for this study, it was shown that although there was some targeting of CA IX with 111In-DOTA-cG250 close to the blood vessels, the uptake of 111In was not directly proportional to the endogenous CA IX. At 4 and 7 days p.i., the correlation of 111In-DOTA-cG250 to CA IX improved.
Similarly, the 111In-DOTA-fragment autoradiography images were compared to the Hoescht, pimidazole and endogenous CA IX fluorescent images at 6 and 24 h p.i. At 6 h p.i. the correlation of 111In-F(ab′)2-cG250 to CA IX is comparable to that of the intact cG250 after 2 days p.i. At 24 h, the 111In matches almost perfectly to the endogenous CA IX image, demonstrating excellent targeting. Additionally, except for the later data points, there is almost an inverse correlation with Hoechst stainingCitation12. Due to the antibody fragments clearing faster into the blood, likewise, diffusion into the tumour is faster, allowing more rapid correlation with CA IX expression. The immunohistochemistry results for the F(ab′)2 and Fab fragments showed a diffuse uptake in the core of the tumour, where the CA IX production is high. The Fab fragment had the fastest blood clearance and from digital analysis of the images, the authors concluded that the Fab fragment was the most rapid and reliable indicator for CA IX expression in hypoxic tumour regions. However, despite this conclusion, due to the lower absolute tumour uptake for both the fragments, and additional problems posed by the non-specific tracer uptake and poor perfusion, the authors recommend using the intact 111In-DOTA-G250 at 7 days p.i. as this gave the most sensitive and accurate detection of CA IXCitation12. The late images of the 111In-DOTA-cG250 were comparable to the early images of the fragments but the higher tumour/non-tumour contrast of 111In-DOTA-cG250 would reduce imaging times.
89Zr-Df-cG250-F(ab′)2
Hoeben et al.Citation16 investigated Df-cG250-F(ab′)2 fragments using 89Zr as the radionuclide. They selected 89Zr as its half-life (3.26 days) matched the pharmacokinetics of the fragment (, entry 7). The labelling chemistry for Df-cG250-F(ab′)2 with 89Zr to generate 89Zr-Df-cG250-F(ab′)2Citation17 is similar to that presented in Citation16. A cohort of BALB nu/nu mice subcutaneously implanted with SCCNij3 cell xenograft tumours, a head and neck tumour model, were used to qualitatively determine the intratumoural distribution of radiolabelled cG250 via autoradiography and compared to 111In-DTPA-cG250. The preparation of 111In-DTPA-cG250 is described by Troost et al.Citation56 It was found that the autoradiography uptake signal of 89Zr-Df-cG250-F(ab′)2 was very similar to that of 111In-DTPA-cG250 with all correlations over two time points p < 0.0001. The uptake of 111In-DTPA-cG250 was much higher than 89Zr-Df-cG250-F(ab′)2 (25.2 ± 6.2 versus 2.68 ± 1.30%ID/g), however, this was expected due to the longer circulatory half-life of IgGCitation16. That being said the 89Zr-Df-cG250-F(ab′)2 showed a faster accumulation in CA IX rich regions at 4 h p.i.
Ten mice were injected with 6.85 ± 0.55 MBq of 89Zr-Df-cG250-F(ab′)2, eight mice (two groups of n = 4) were examined via a small animal PET imaging scanner at 4 and 24 h p.i. At 30 min prior to PET imaging, mice were injected with 80 mg/kg of pimonidazole (hypoxia marker). One minute before sacrifice, mice were injected with Hoechst 33342 (perfusion marker). The tumours were clearly visible in the PET images at both 4 and 24 h p.i. The radioactive uptake of the 89Zr-Df-cG250-F(ab′)2 was compared to the positive area of the tumour stained for CA IX and pimonidazole in tumour sections. The standardized uptake value maximum (SUVmax) of 89Zr-Df-cG250-F(ab′)2 for tumour, muscle and tissue was determined (). The kidneys and liver displayed high uptake of 89Zr-Df-cG250-F(ab′)2, however, this is a common side effect of using F(ab′)2 fragments: it is probable that the fragment can be reabsorbed, which may cause problems for imaging in the future.
Table 5. The SUVmax of 89Zr-Df-cG250-F(ab′)2 and %ID/g of tissue taken from BALB nu/nu mice subcutaneously implanted with SCCNij3 cell xenograft tumours at 4 and 24 h p.i.Citation16.
A significant correlation (p < 0.0001, r = 0.57–0.74) between the CA IX staining and radioactivity uptake was observed at both time pointsCitation16. Similarly, there was a significant correlation (p < 0.0001, r = 0.46–0.68) between pimonidazole (hypoxia) staining and tracer uptake. A strong positive correlation (p = 0.0067, r = 0.93) was also observed between SUVmax on the PET images and the measured tumour uptake found from the analysis of tissue (%ID/g), this correlation is similar to that found by Lawrentschuk et alCitation7. for 124I-cG250 in a SK-RC-52 model. This study concluded that 89Zr-Df-cG250-F(ab′)2 could be useful in determining CA IX-positive hypoxic areas for head and neck carcinomas via demonstrating a significant spatial correlation of 89Zr-Df-cG250-F(ab′)2 and CA IX expression, however, the authors state that further research is required.
177Lu-DTPA-A3-SIP and 177Lu-DTPA-CC7-SIP
Radiolabelled A3-SIP and CC7-SIP antibodies were prepared by covalent modification of a side chain lysine residue of the SIP by reaction with p-isothiocyanate benzyl DTPA (p-SCN-Bn-DTPA) to form a thiourea link, followed by coordination of 177Lu radionuclide to the DTPA host ()Citation18,Citation57. Mass spectrometry analysis showed a stoichiometry of ∼1.8 DTPA molecules per antibody moleculeCitation18. The biodistribution of 177Lu-DTPA-A3-SIP and 177Lu-DTPA-CC7-SIP (0.259–0.407 MBq) (, entry 8)Citation19 was evaluated following injection into LS174T colorectal xenograft models (n = 4) and compared to 177Lu-DTPA-SIP-L19 (a clinical stage antibody that targets neo-vasculature structures). The mice were sacrificed 24 h after SIP administration, the organs removed, weighed and the radioactivity counted. Very high kidney and high liver uptake values was observed for both 177Lu-DTPA-A3-SIP and 177Lu-DTPA-CC7-SIP. 177Lu-DTPA-A3-SIP showed a ∼4-fold lower tumour accumulation compared to 177Lu-DTPA-SIP-L19 accumulation (2.4 versus 9.3 %ID/g, respectively), however, had an improved T/B ratio (16.7 versus 5.8, respectively). Biodistribution data for 177Lu-DTPA-CC7-SIP was not presented. This study concluded that simultaneous use of vascular-targeting and hypoxia-targeting antibodies may be desirable to achieve a more homogenous distribution and detection of tumours.
Small molecule imaging agents that target CA IX
Two of the ten agents in MICAD that target CA IX are small molecules.
99mTc-PS
Almost all reported CA small molecule ligands comprise a zinc binding group in their structure of which the primary sulfonamide moiety (−SO2NH2) is the premier example. The primary sulfonamide anion coordinates to the active site Zn2+ of CAs, acting as an “anchor” on to which variable “tail” groups, selected to incorporate desirable properties, can be tetheredCitation54. An important finding by Dubois et al.Citation30 was that the binding of sulfonamide based ligands to cell surface CA IX occurs only under hypoxic conditions. Akurathi et al.Citation20 synthesized a 99mTc-labelled small molecule sulfonamide compound, 99mTc-PS (, entry 9)Citation21, with the aim of imaging CA IX expression to determine regional tumour hypoxia using SPECT. 99mTc is the most widely used radionuclide for diagnostic nuclear medicine (>90%)Citation58. It can be generated at low cost, it has a suitable half-life (6 h) and its gamma ray emission energy is not accompanied by beta emissions, allowing a more precise alignment of imaging detectorsCitation58,Citation59. Additionally, its emission energy is 141 keV, enough to be detected by gamma cameras but low enough to not carry out significant damage to the bodyCitation20,Citation58. 4-(2-Aminoethyl)benzenesulfonamide (AEBS) was chosen as the core structure of 99mTc-PS as it has a high affinity for CA IX (Ki = 33 nM) and the other cancer associated CA (CA XII, Ki = 3.2 nM) but low affinity for off-target cytoplasmic CA I and CA II and membrane-bound CA IV (Ki = 21 μM, 160 and 2.4 μM, respectively). 99mTc requires a chelating agent, so [99mTc(H2O)3(CO)3]+ (prepared using an Isolink™ kit) together with N-2-picolyl-N-acetic acid were used in the synthesis of 99mTc-PS as the [99mTc(H2O)3(CO)3]+ can exchange the three water molecules with the amine, aromatic N-heterocycle and carboxylate donor of N-2-picolyl-N-acetic acid, while the latter is also able to covalently attach to the AEBS small molecule through amide bond formation (). A rhenium analogue of this sulfonamide ligand, Re–PS, was synthesized as a “cold” control compound for 99mTc-PS to enable the indirect study of the affinity of 99mTc-PS for CA I, II, IX and XII under in vitro conditionsCitation20. The in vitro inhibition data for Re–PS was compared to AEBS, it is worthwhile to note that the Ki of Re–PS for CA IX is similar to that for AEBS while it is more potent than AEBS for CA XII.
The stability of 99mTc-PS in whole blood and plasma of mice was analysed at 1 h p.i. In plasma 65% of the radioactivity was in intact 99mTc-PS while 35% was present as a polar radiometabolite. Biodistribution studies with 99mTc-PS were then carried out in mice bearing HT-29 colorectal tumours at 0.5, 1, 2 or 4 h p.i. Accumulation was initially highest in the liver and it was proposed that this may be due to an active transport mechanism on the liver surface. The tracer is moderately hydrophilic resulting in liver clearance, hence at 2 and 4 h p.i. ∼18% ID/g was found in the intestines, indicating clearance to the hepatobiliary pathway. The tracer was also found initially in a significant quantity in the kidneys (36–42 %ID/g at 0.5 and 1 h p.i.), an indication that the tracer may be binding to CA XII or XIV, which are highly expressed on the membrane of the kidneys. Control experiments using AEBS as a blocking agent were used to evaluate this theory and it was concluded that the tracer uptake in the kidneys was not related to interaction with CA XII or XIV. A minimal amount of 99mTc-PS was shown to diffuse into red blood cells (0.1 %ID/g at 1 h p.i.) and is assumed to bind to either red blood cell CA I or CA IICitation20. Disappointingly, the tumour uptake of 99mTc-PS was low at each of the four tested time points (0.1 ± 0.1 %ID/g at 0.5 h then 0.0 ± 0.0 thereafter).
13C-labelled bicarbonate
Gallagher et al.Citation22 designed hyperpolarized H13 as a MRI imaging agent to investigate the redox properties of CA, potentially including CA IX, but not specifically for CA IX (Table 1, entry 10). Hyperpolarized H13
was developed on the basis of dynamic nuclear polarization (DNP), a technique that increases the sensitivity of solution-state magnetic resonance spectroscopy (MRS). By injection of hyperpolarized H13
, their aim was to produce a high resolution pH map of a transverse slice through an animal that may offer a new way of detecting disease and monitoring treatment response. pH changes can indicate inflammation, ischaemia or renal disease amongst other pathological conditions, however, there is currently no clinical tool to measure in vivo pH. Using the Henderson–Hasselbalch equation, the tissue pH can be determined from the measurements of the concentration ratio between H13
and 13CO2, assuming that the pKa of bicarbonate is constant at 6.17Citation23. The replacement of 12C with 13C does not alter the biochemistry of the molecule while it provides a >10 000-fold enhancement of the 13C MRS signals thus allowing changes in pH to be monitored in vivoCitation23.
After validation of the MRI method for pH determination, hyperpolarized H13 (200 μL, 40 mg/kg) was injected into three groups of mice with EL4 murine lymphoma tumours. Group 1 were untreated controls, group 2 were given sodium bicarbonate in the drinking water to increase the tissue pH and group 3 were given an ammonium chloride gavage to decrease the tissue pHCitation22. For comparison, several mice were injected with the pH sensitive molecule 3-aminopropylphosphonate (3-APP) so that 31P spectra could be obtained for comparison. 3-APP is a non-toxic MRS-visible extracellular marker that is relatively unaffected by temperature or ionic strength, the chemical shift frequency of 3-APP is sensitive to pH allowing the determination of pH in vivoCitation60. 13C and 31P MRS imaging at 9.4 T were performedCitation22. The spatial distribution of 13CO2 and H13
in the transverse slice was determined. It was shown that the highest concentration of 13CO2 was in the tumour mass compared to H13
which was highest in the aorta. Although minimal differences were shown in signal intensity between muscle/tumour tissue, a pH map was successfully calculated and a low pH value for the tumour mass was shown, demonstrating that it was possible to image tissue pH noninvasively in vivo. Comparing the untreated mice (tumour pH 6.71), NaHCO3 treated mice (tumour pH 7.02) with NH4Cl (tumour pH 6.47) suggests that the H13
measurement reflects more of the pHe. The authors suggest this could be due to the acquisition of spectra immediately after H13
is injected and that normally there is a higher concentration of bicarbonate in the extracellular environment. A limitation of the method was also highlighted, namely small differences in the rate at which H13
and 13CO2 lose polarization will lead to the calculated pH varying over time. The T1’s were found to be 10.1 ± 2.9 s (n = 9) for H13
and 9.8 ± 2.5 s for 13CO2 (n = 7) with the presence of CA increasing this exchange.
Properties of antibodies and small molecules as imaging agents
Although antibodies provide excellent specificity towards their antigen, there are several factors that make tumour targeting with antibodies difficult. Solid tumours are heterogeneous masses and a common limitation of antibody-based imaging agents is slow or incomplete tissue distribution, especially to areas with poor vasculatureCitation31 as the drugs cannot completely penetrate the tissueCitation61. The contributing factors to this include molecular size (IgG ∼ 150 KDa), the antigen barrier and the high tumour interstitial pressure. Additionally, slow plasma clearance maintains high drug levels which complicate imaging and therapyCitation61 and present a high radiation dose risk to the bone marrowCitation13. Rudnick and Adams have discussed in depth the interaction between the affinity, avidity and efficacy of antibodies in tumour targetingCitation62. Although not the focus of this article, the critical parameters they specify that affect a binding site are: antigen density, mAb internalization and metabolism and the mAb binding affinityCitation62.
Antibody fragments may provide advantages over the intact antibodies. Their reduced molecular size (e.g. cG250-F(ab′)2 fragments ∼100 kDa compared to intact cG250 ∼150 kDa) may aid in tissue penetration and their reduced clearance time from the blood can result in higher tumour uptake compared to normal tissues. However, fragments may have increased renal uptake as they are reabsorbed by the renal tubular cells and may lead to radiation-induced nephrotoxicityCitation16,Citation39. Similarly, scFv (single-chain variable) fragments of ∼25 kDa may have excellent tumour penetration but their rapid clearance from the blood may be advantageous or disadvantageous. Other disadvantages include that they are monovalent and there is a possibility of aggregationCitation63,Citation64. SIPs consist of two scFv fragments held via a hinge, making them bivalent, and their size of ∼80 kDa means that they have a slower clearance timeCitation63,Citation64. They have an increased total affinity and have reduced immunogenicityCitation63,Citation64. However, they have no effector functions and can be difficult to generate as the synthesis involves several stepsCitation18,Citation63.
Aromatic sulfonamides are the classical small molecule CA inhibitors that bind to the active site of CACitation65. The CA active sites are extremely tolerant to diverse and variable structures appended to the aromatic sulfonamide moietyCitation66. It is possible to vary considerably the size, shape, charge, polarity, hydrophobicity etc. and still have nanomolar CA inhibition. This tolerance allows finetuning of the biopharmaceutical and toxicological properties of the inhibitor via structural manipulationsCitation66. Additionally, small molecules may be advantageous in their cost and ease of manufacture as well as their improved ADME (adsorption, distribution, metabolism and excretion) and DMPK (drug metabolism and pharmacokinetics) properties when compared to antibodiesCitation66. However, sensitivity, degradation, toxicity and clearance may all be considerably problematic with these compounds as well as specificity for CA IX over other CAs. To overcome these challenges the small molecule has to be designed very carefully to take the limitations into account.
Imaging via PET is the most common imaging modality used with the agents described here. PET is advantageous in that it allows the absolute quantitation of tracer uptake unlike determination of uptake via histological sections which is highly subjectiveCitation12. As well as allowing the possibility of serial imaging, it is possible to image the whole tumour volume, instead of biopsy or tissue specimensCitation12. PET displays a much higher sensitivity than SPECT (∼2–3 times the order of magnitude). Using SPECT, however, has the potential to increase the observational time window due to the longer half-life of the emitters usedCitation67. MRI has superior soft tissue contrast resolution, multiplanar image acquisition and functional imaging capabilitiesCitation68. Of note is that only one of these agents has been designed for MRI. With the growth of MRI in hospitals and clinics, it is likely that more agents will be developed to use this modality which provides high resolution and superior soft tissue contrast. However, the ultimate deciding factor in the choice of imaging modality is the biological considerations of the systemCitation67. Rahmim and ZaidiCitation67 warn that in the biological context, generalized comparisons between modalities are not appropriate and instead they need to be performed on a case-by-case basis so that the correct imaging modality is selected.
The development of agents for in vivo imaging of CA IX extends well beyond those agents listed in MICAD. Recent examples include small molecule 18F labelled PET agentsCitation69, and an extensive comparison of 124I-cG250 with 89Zr-Df-cG250 in murine models with CA IX expressing ccRCC zenografts (SK-RC-52 or NU-12) using PETCitation70. The biodistribution results demonstrated very high tumour uptake. In the NU-12 tumour model uptake of the two agents was similar (48.7 ± 15.2%ID/g versus 32.0 ± 22.9%ID/g, respectively, p = 0.257), while in the SK-RC-52 tumour model the uptake of 89Zr-Df-cG250 was significantly higher than 124I-cG250 (114.7 ± 25.2%ID/g versus 38.2 ± 18.3%ID/g, respectively, p = 0.029). This high uptake gave excellent PET image contrast, for example small intraperitoneal lesions (7 mm3) were visualized with 89Zr-Df-cG250 and were readily discriminated from the liver and spleen. The results from this study suggest that PET in RCC patients may be more sensitive using 89Zr-Df-cG250 instead of 124I-cG250Citation70.
Conclusions
Of the MICAD entries, ten agents target CA IX, an antigen overexpressed in several solid cancer types in hypoxic conditions with expression in normal tissues is minimal. CA IX is a challenging diagnostic target for non-invasive molecular imaging, especially given the similarities in the active site for all 12 catalytically active human CAs. It is however an extremely important target as the detection and monitoring of hypoxic tumours can inform clinical decisions surrounding cancer therapy. Continued development of CA IX imaging agents will ideally balance patient safety, good tumour penetration and accurate localization of hypoxia. The ten MICAD agents described herein, highlight the scope and remaining opportunity for development of improved agents targeting CA IX imaging in oncology.
Declaration of interest
The Australian Research Council (Grant Nos DP110100071 and FF110100185 to S.-A.P.), Griffith University (Scholarship to D.S.) and the (Australian) National Imaging Facility provided financial support.
The authors report no conflicts of interest. The authors alone are responsible for the content and writing of this article.
References
- Molecular Imaging and Contrast Agent Database (MICAD). About MICAD. Bethesda (MD): National Center for Biotechnology Information, NLM, NIH; 2004--2013. Available from: http://micad.nih.gov
- Chopra A, Shan L, Eckelman W, et al. Molecular Imaging and Contrast Agent Database (MICAD): evolution and progress. Mol Imaging Biol 2012;14:4–13
- MICAD. MICAD Overview Powerpoint Presentation 2004–2013
- Michalski M, Chen X. Molecular imaging in cancer treatment. Eur J Nucl Med Mol Imaging 2012;38:358–77
- Leung K. 124I-Chimeric monoclonal antibody G250. Molecular Imaging and Contrast Agent Database (MICAD). Bethesda (MD): National Center for Biotechnology Information, NLM, NIH; 2004--2013. [last updated: 20 May 2010]. Available from: http://www.ncbi.nlm.nih.gov/books/NBK43528/
- Divgi C, Pandit-Taskar N, Jungbluth A, et al. Preoperative characterisation of clear-cell renal carcinoma using iodine-124-labelled antibody chimeric G250 (124I-cG250) and PET in patients with renal masses: a phase I trial. Lancet Oncol 2007;8:304–10
- Lawrentschuk N, Lee F, Jones G, et al. Investigation of hypoxia and carbonic anhydrase IX expression in a renal cell carcinoma xenograft model with oxygen tension measurements and 124I-cG250 PET/CT. Urol Oncol 2011;29:411–20
- Li J, Shi L, Wang C, et al. Preliminary biological evaluation of 125I-labeled anti-carbonic anhydrase IX monoclonal antibody in the mice bearing HT-29 tumours. Nucl Med Commun 2011;32:1190–3
- Shan L. 125I-Labeled mouse anti-human carbonic anhydrase IX monoclonal antibody Molecular Imaging and Contrast Agent Database (MICAD). Bethesda (MD): National Center for Biotechnology Information, NLM, NIH; 2004--2013. [last updated 30 May 2012]. Available from: http://www.ncbi.nlm.nih.gov/books/NBK97273/
- Brouwers A, Verel I, Van Eerd J, et al. PET radioimmunoscintigraphy of renal cell cancer using 89Zr-labeled cG250 monoclonal antibody in nude rats. Cancer Biother Radiopharm 2004;19:155–63
- Leung K. 89Zr-N-succinyldesferal-chimeric monoclonal antibody cG250 Molecular Imaging and Contrast Agent Database (MICAD). Bethesda (MD): National Center for Biotechnology Information, NLM, NIH; 2004--2013. [last updated 27 May 2010; cited March 2013]. Available from: http://www.ncbi.nlm.nih.gov/books/NBK43525/
- Carlin S, Khan N, Ku T, et al. Molecular targeting of carbonic anhydrase IX in mice with hypoxic HT29 colorectal tumor xenografts. PLoS One 2010;5:e10857, 1--9
- Chopra A. [111In]-Labeled chimeric monoclonal antibody cG250 directed against carbonic anhydrase IX Molecular Imaging and Contrast Agent Databasr (MICAD). Bethesda (MD): National Centre for Biotechnology Information, NLM, NIH; 2004--2013. [last updated 2 September 2010]. Available from: http://www.ncbi.nlm.nih.gov/books/NBK45632/
- Chopra A. [111In]-Labeled monovalent Fab fragment of chimeric monoclonal antibody cG250 directed against carbonic anhydrase IX Molecular Imaging and Contrast Agent Database (MICAD). Bethesda (MD): National Center for Biotechnology Information, NLM, NIH; 2004--2013. [last updated 2 September 2010]. Available from: http://www.ncbi.nlm.nih.gov/books/NBK45632/
- Chopra A. [111In]-Labeled divalent Fab fragment of chimeric monoclonal antibody cG250 directed against carbonic anhydrase IX Molecular Imaging and Contrast Agent Database (MICAD). Bethesda (MD): National Center for Biotechnology Information, NLM, NIH; 2004--2013. [last updated 2 September 2010]. Available from: http://www.ncbi.nlm.nih.gov/books/NBK45634/
- Hoeben B, Kaanders J, Franssen G, et al. PET of hypoxia with 89Zr-labeled cG250-F(ab′)2 in head and neck tumors. J Nucl Med 2010;51:1076–83
- Chopra A. 89Zr-Labeled N-suc-desferrioxamine-conjugated anti-carbonic anhydrase IX chimeric monoclonal antibody cG250-F(ab′)2 fragments Molecular Imaging and Contrast Agent Database (MICAD). Bethesda (MD): National Center for Biotechnology Information, NLM, NIH; 2004–2013. [last updated 14 October 2010]. Available from: http://www.ncbi.nlm.nih.gov/books/NBK47332/
- Ahlskog J, Schliemann C, Marlind J, et al. Human monoclonal antibodies targeting carbonic anhydrase IX for the molecular imaging of hypoxic regions in solid tumours. Brit J Cancer 2009;101:645–57
- Leung K. 177Lu-Benzyl-diethylenetriamine pentaacetic acid-anti-carbonic anhydrase IX small immunoprotein A3 Molecular Imaging and Contrast Agent Database (MICAD). Bethesda (MD): National Center for Biotechnology Information, NLM, NIH; 2004–2013. [last updated 12 January 2010]. Available from: http://www.ncbi.nlm.nih.gov/books/NBK23180/
- Akurathi V, Dubois L, Lieuwes N, et al. Synthesis and biological evaluation of a 99mTc-labelled sulfonamide conjugate for in vivo visualization of carbonic anhydrase IX expression in tumor hypoxia. Nucl Med Biol 2010;37:557–64
- Chopra A. [99mTc](CO)3N-(pyridin-2-yl-methyl)-N[2-(4-sulfamoylphenyl)-ethyl]aminoethyl acetate Molecular Imaging and Contrast Agent Database (MICAD). Bethesda (MD): National Center for Biotechnology Information, NLM, NIH; 2004–2013. [last updated 28 October 2010]. Available from: http://www.ncbi.nlm.nih.gov/books/NBK47642/
- Gallagher F, Kettunen M, Day S, et al. Magnetic resonance imaging of pH in vivo using hyperpolarized 13C-labelled bicarbonate. Nat Lett 2008;453:940–4
- Shan L. Hyperpolarized 13C-labeled bicarbonate (H13) for in vivo pH measurement with 13C magnetic resonance spectroscopy Molecular Imaging and Contrast Agent Database (MICAD). Bethesda (MD): National Center for Biotechnology Information, NLM, NIH; 2004–2013. [last updated 12 April 2010]. Available from: http://www.ncbi.nlm.nih.gov/books/NBK32324/
- Neri D, Supuran C. Interfering with pH regulation in tumours as a therapeutic strategy. Nat Rev Drug Discov 2011;10:767–77
- Innocenti A, Pastoreková S, Pastorek J, et al. The proteoglycan region of the tumor-associated carbonic anhydrase isoform IX acts as anintrinsic buffer optimizing CO2 hydration at acidic pH values characteristic of solid tumors. Bioorg Med Chem Lett 2009;19:5825–8
- De Simone G, Supuran C. Carbonic anhydrase IX: biochemical and crystallographic characterization of a novel antitumor target. Biochim Biophys Acta 2010;1804:404–9
- Chiche J, Ilc K, Laferriere J, et al. Hypoxia-inducible carbonic anhydrase IX and XII promote tumor cell growth by counteracting acidosis through the regulation of the intracellular pH. Cancer Res 2009;69:358–68
- McDonald P, Winum J, Supuran C, Dedhat S. Recent developments in targeting carbonic anhydrase IX for cancer therapeutics. Oncotarget 2012;3:84–97
- Apte S, Chin F, Graves E. Molecular imaging of hypoxia: strategies for probe design and application. Curr Org Synth 2011;8:593–603
- Dubois L, Lieuwes N, Maresca A, et al. Imaging of CA IX with fluorescent labelled sulphonamides distinguishes hypoxic and (re)-oxygenated cells in a xenograft tumour model. Radiother Oncol 2009;92:423–8
- Bao B, Groves K, Zhang J, et al. In vivo imaging and quantification of carbonic anhydrase IX expression as an endogenous biomarker of tumor hypoxia. PLoS One 2012;7:e50860, 1–12
- Mees G, Dierckx R, Vangestel C, Van de Wiele C. Molecular imaging of hypoxia with radiolabelled agents. Eur J Nucl Med Mol Imaging 2009;36:1674–86
- Gray L, Conger A, Ebert M, et al. The concentration of oxygen dissolved in tissues at the time of irradiation as a factor of radiotherapy. Brit J Radiol 1953;26:638–48
- Read J. Mode of action of x-ray doses given with different oxygen concentrations. Br J Radiol 1952;25:336–38
- Varai M, Calkins-Adams D, Rinker L, et al. Pimonidazole: a novel hypoxia marker for complementary study of tumor hypoxia and cell proliferation in cervical carcinoma. Gynecol Oncol 1998;71:270–7
- Boenisch T. Chapter 1: Antibodies. In: Kumar GL, Rudbeck L, eds. Immunohistochemical staining methods. 5th ed. Dako Education Gude [Internet]. Carpinteria (CA): Dako North America; 2009:1--9. Available from: http://www.dako.com/08002_ihc_staining_methods_5ed.pdf [last accessed 25 Oct 2013]
- Oosterwijk-Wakka J, Boerman O, Mulders P, Oosterwijk E. Monoclonal antibody G250 recognizing carbonic anhydrase IX in renal cell carcinoma: biological and clinical studies. In: Bukowski RM, Figlin RA, Motzer RJ, eds. Renal cell carcinoma: molecular targets and clinical applications, 2nd ed. New York: Humana Press; 2009:231--47
- Pastorek J, Pastoreková S, Callebut I, et al. Cloning and characterization of MN, a human, tumor-associated protein with a domain homologous to carbonic anhydrase and a putative helix-loop-helix DNA binding segment. Oncogene 1994;9:2788–888
- Brouwers A, Mulders P, Oosterwijk E, et al. Pharmacokinetics and tumour targeting of 131I-labeled F(ab′)2 fragments of the chimeric monoclonal antibody G250: preclincial and clinical pilot studies. Cancer Biother Radiopharm 2004;19:466–77
- Adams G, Weiner L. Monoclonal antibody therapy of cancer. Nat Biotechnol 2005;23:1147–57
- Dennis M, Jin H, Dugger D, et al. Imaging tumors with an albumin-binding Fab, a novel tumor-targeting agent. Cancer Res 2007;67:254–61
- Emir E, Bander N, Finstad C, et al. Predicting response to radioimmunotherapy from the tumor microenvironment of colorectal carcinomas. Cancer Res 2007;67:11896–905
- Sonzogni A. NuDat 2.6 database [Internet]. New York: National Nuclear Data Center, Brookhavan National Laboratory; 2013. Available from: http://www.nndc.bnl.gov/nudat2/ [last accessed 17 Aug 2013]
- Stillebroer AB, Oosterwijk E, Oyen WJG, et al. Radiolabeled antibodies in renal cell carcinoma. Cancer Imaging 2007;7:179--88
- Brouwers A, Buijs W, Oosterwijk E, et al. Targeting of metastatic renal cell carcinoma with the chimeric monoclonal antibody G250 labeled with 131I or 111In. Clin Cancer Res 2003;9:3953–60
- Mather S, Ward B. High efficiency iodination of monoclonal antibodies for radiotherapy. J Nucl Med 1987;28:1034–6
- Bailey G. The iodogen method for radiolabeling proteins. The protein protocols handbook. 1st ed. 1996:673–4
- Salacinski P, McLean C, Sykes J, et al. Iodination of proteins, glycoproteins, and peptides using a solid-phase oxidizing agent, 1,3,4,6-tetrachloro-3a,6a-diphenyl glycoluril (iodogen). Anal Biochem 1981;117:136–46
- Brouwers A, van Eerd J, Frielink C, et al. Optimization of radioimmunotherapy of renal cell carcinoma: labeling of monoclonal antibody cG250 with 131I, 90Y,177Lu, or 186Re. J Nucl Med 2004;45:327–37
- Yuanfang L, Chuanchu W. Radiolabeling of monoclonal antibodies with metal chelates. Pure Appl Chem 1991;63:427–63
- Roselli M, Schlom J, Gansow O, et al. Comparative biodistributions of yttrium- and indium-labeled monoclonal antibody B72.3 in athymic mice bearing human colon carcinoma xenografts. J Nucl Med 1989;30:672–82
- Riccabona G. I125 in the clinical evaluation of thyroid disease. N Engl J Med 1965;273:126–30
- Stein R, Govindan S, Hayes M, et al. Advantage of a residualizing iodine radiolabel in the therapy of a colon cancer xenograft targeted with an anticarcinoembryonic antigen monoclonal antibody. Clin Cancer Res 2005;11:2727–34
- Verel I, Visser G, Boellaard R, et al. 89Zr immuno-PET: comprehensive procedures for the production of 89Zr-labeled monoclonal antobodies. J Nucl Med 2003;44:1271–81
- Smith-Jones P, Vallabahajosula S, Goldsmith S, et al. In vitro characterization of radiolabeled monoclonal antibodies specific for the extracellular domain of prostate-specific membrane antigen. Cancer Res 2000;60:5237–43
- Troost E, Bussink J, Kaanders J, et al. Comparison of different methods of CAIX quantification in relation to hypoxia in three human head and neck tumor lines. Radiother Oncol 2005;76:194–9
- Brechbiel M, Gansow O, Atcher R, et al. Synthesis of 1-(p-isothiocyanatobenzyl) dericvatives of DTPA and EDTA. Antibody labeling and tumor-imaging studies. Inorg Chem 1986;25:2772–81
- Dilworth JR, Parrot SJ. The biomedical chemistry of technetium and rhenium. Chem Soc Rev 1998;27:43–55
- Nave C. Technetium-99m. Georgia, USA: Georgia State University. [cited 20 August 2013]. Available from: http://hyperphysics.phy-astr.gsu.edu/hbase/nuclear/technetium.html
- Gillies RJ, Liu Z, Bhujwalla Z. 31P-MRS measurements of extracellular pH of tumors using 3-aminopropylphosphonate. Am J Physiol. 1994;267:195–203
- Schmidt M, Wittrup K. A modeling analysis of the effects of molecular size and binding affinity on tumour targeting. Mol Cancer Ther 2009;8:2861–71
- Rudnick S, Adams G. Affinity and avidity in antibody based tumor targeting. Cancer Biother Radiopharm 2009;24:155–61
- Ayriss J, Kuan C, Boulton S, et al. Molecular targets for antibody-mediated immunitherapy of malignant glioma. In: Van Meir EG, ed. CNS cancer: models, markers, prognostic factors, targets and therapeutic approaches. Chap. 36. New York: Humana Press; 2009:865--98
- Occhino M, Raffaghello L, Burrone O, et al. Generation and characterization of dimeric small immunoproteins specific for neuroblastoma associated antigen GD2. Int J Mol Med 2004;14:383–8
- Supuran C. Carbonic anhydrases: novel therapeutic applications for inhibitors and activators. Nature Rev Drug Disc 2008;7:168–81
- Poulsen S. Carbonic anhydrases inhibition as a cancer therapy: a review of patent literature, 2007–2009. Expert Opin Ther Patants 2010;20:795–806
- Rahmim A, Zaidi H. PET versus SPECT: strengths, limitations and challenges. Nucl Med Commun 2008;29:193–207
- Torgian D, Zaidi H, Kwee T, et al. PET/MR imaging: technical aspects and potential clinical applications. Radiology 2013;267:26–44
- Pan J, Lau J, Mesak F, et al. Synthesis and evaluation of 18F-labeled carbonic anhydrase IX inhibitors for imaging with positron emission tomography. J Enzyme Inhib Med Chem 2013. [Epub ahead of print]. doi: 10.3109/14756366.2013.773994
- Stillebroer AB, Franssen GM, Mulders PFA, et al. ImmunoPET imaging of renal cell carcinoma with 124I- and 89Zr-labeled anti-CAIX monoclonal antibody cG250 in mice. Cancer Biother Radiopharm 2013;28:510–15