Abstract
One of the most viable options to tackle the growing resistance to the antimalarial drugs is hybrid molecules. It involves combination of different scaffolds in one frame that may lead to compounds with diverse biological profiles. In this context, new hybrids of three different scaffolds viz pyrazole, pyrazoline and thiosemicarbazone moiety were incorporated into one single compound and evaluated for their in vitro schizontocidal activity against the CQ-sensitive 3D7 strain of Plasmodium falciparum. Compounds with significant in vitro antimalarial activity were further evaluated for cytotoxicity against VERO cell lines. The best active compound 48 exhibited an IC50 of 1.13 µM. The in vitro results were further validated by quantitative structure–activity relationship (QSAR).
Introduction
Parasite borne diseases like malaria, sleeping sickness, amoebiasis, dysentery, etc. are rife, predominantly in the developing countries and are responsible for immense distressCitation1. Malaria, caused by P. falciparum, continues to be a grim health concern in Africa, South America and many parts of AsiaCitation2. Curbing this enervating disease has been sternly compromised by the emergence and spread of resistance to a majority of existing antimalarial agentsCitation3. In view of this, there is exigency for the breakthrough of new, affordable and safe antimalarial agentsCitation4.
Pyrazole ring is a biologically active motif, which is of much importance to medicinal chemists owing to its pharmacological diversity. It is also an integral part of a number of well-established commercially available drugs like, zoniporide, celecoxib, pyrazomycin, lonazolac, fezolamine, difenamizole, mepirizole, etc. ().
Taking this as cue, some researchers have also highlighted the antimalarial propensity of this ring. Cunico et al.Citation5 synthesized and evaluated the antimalarial activity of chloroquine–pyrazole analogues (I) and highlighted the critical role of aromatic functionality of pyrazole for activity. Qirante et al.Citation6 evaluated antimalarial activity of ligands and complexes derived from pyrazole (II). Bekhit et al.Citation7 also developed some pyrazole derivatives (III) with antimalarial activity ().
Pyrazolines, the reduced form of pyrazoles, also have several applications in the field of pharmaceutical research. They have a wide range of biological activities such as, antimicrobialCitation8, anticancerCitation9, CNS depressantCitation10, etc. Lately, several researchers have highlighted the antimalarial penchant of pyrazolines. Wanare et al.Citation11 reported pyrazoline derivatives (IV) as growth inhibitors for P. falciparum. Similarly, Acharya et al.Citation12 and Insuasty et al.Citation13 also developed pyrazoline analogues (V–VI) and assessed their antimalarial activity ().
Recently, there has been a great interest in thiosemicarbazones as potential antimalarial agentsCitation14–16. The pioneering work on acetylpyridine thiosemicarbazonesCitation17 (VII) as antimalarials paved way for Pingaew et al.Citation18 to develop benzoyl thiosemicarbazone analogues of isoquinoline (VIII) as antimalarials. Khanye et al.Citation19 also developed gold compounds containing thiosemicarbazone ligands (IX) as antimalarials ().
Currently World Health Organisation recommends the use of two or more agents as the best way to minimize treatment failures and the spread of drug resistanceCitation20. The presence of more than one structurally active moiety in a single molecule may show an enhanced activityCitation21. In the design of new drugs, the development of hybrid molecules through the combination of different scaffolds in one frame may lead to compounds with interesting biological profiles. Therefore, a molecule containing more than one biologically active scaffold, each with different mode of action could be favourable for the treatment of malaria. Therefore, three different scaffolds were selected viz pyrazole, pyrazoline and thiosemicarbazone moiety and incorporated into one single compound. From our ongoing efforts on finding novel hybrid antimalarialsCitation22 we, herein report the design, synthesis and antimalarial activity of novel hybrid molecules based on pyrazole moiety linked to pyrazoline moiety having thiosemicarbazone as a part of pyrazoline moiety (). The synthetic steps involved the formation of pyrazole carbaldehydes through Vilsmeier-Haack reactionCitation23, followed by Claisen-Schmidt condensation of these aldehydes with acetophenones. Resulting chalcones on treatment with thiosemicarbazide gave the title compoundsCitation24,Citation25.
The synthesized compounds were evaluated for in vitro schizontocidal activity against the CQ-sensitive 3D7 strain of P. falciparum using modification of Trager and Jenson methodCitation26 as described by Dua et al.Citation27. The compounds having IC50 less than 5 µg/mL were further evaluated for their cytotoxicity by Mosmann methodCitation28 with certain modifications. Finally, to provide an insight into the relationship existing between the chemical structure and pharmacological activity, QSAR of the synthesised compounds was performed using the PHASE module of SchrödingerCitation29. The generated model gave statistically significant results, with r2 of 0.9992, and the structural features spread across the entire molecule. This further validated our in vitro results.
Methods
Chemistry
All chemicals and solvents were purchased from SD-fine chemical and used without further purification. Melting points were measured by open-end capillary method and are uncorrected. TLC was performed with silica gel 60 F254 (Merck) using methanol: chloroform (1:9) as the solvent system.
Elemental analyses were performed using CHNS Elementar (Vario EL-III). The IR spectra were recorded using a Bruker alpha-T spectrophotometer. 1H-NMR spectra were recorded on a Bruker Avance-400 MHz in DMSO-d6 with tetramethylsilane (TMS) as the internal standard. The 13C-NMR of compounds was recorded on Bruker Avance-100 MHz instrument in DMSO-d6. Chemical shifts (δ) are reported in parts per million, and coupling constants (J) in hertz. High-resolution mass spectral data were obtained on an Agilent 6540 UHD Accurate Mass (Q-ToF) Mass Spectrometer (LTQ XL/LTQ Orbitrap Discovery) coupled to Agilent 1290 series LC system (Agilent PDA detector, autosampler, and thermostat, thermostated column compartment, binary pump).
General procedure for the synthesis of 1-Phenyl-2-(1-phenylethylidene)hydrazines 1–4
GAA (0.024 mole) and phenylhydrazine (0.05 mole) were added to a solution of substituted acetophenone (0.05 mole) in 30 mL methanol. The mixture was refluxed at 80 °C until the completion of reaction. The reaction was monitored through TLC. After completion, the reaction vessel was cooled to 0 °C. The precipitate was filtered, washed with cold methanol and driedCitation30.
General procedure for the synthesis of 1,3-diphenyl-1H-pyrazole-4-carbaldehyde 5–8
POCl3 (0.09 mole) was added drop wise through dropping funnel to previously cooled DMF (0.09 mole) at 0–5 °C and stirred for few minutes. A solution of compounds 1–4 (0.03 mole) in DMF was added drop wise to the reaction mixture. This was then kept at room temperature for few minutes and refluxed at 70–80 °C until the completion of reaction. The reaction was monitored through TLCCitation30.
Following completion, the reaction mixture was cooled to room temperature, quenched with crushed ice and basified with sodium hydroxide to obtain a precipitate. The precipitate was filtered, washed thoroughly with water, dried and recrystallized from ethanol.
General procedure for the synthesis of 3-(1,3-diphenyl-1H-pyrazol-4-yl)-1-phenylprop-2-en-1-one 9–28
Chalcones were synthesised by Claisen Schmidt reactionCitation31. Aldehyde (0.05 mole) and substituted acetophenone (0.05 mole) were dissolved in 35 mL methanol. To this reaction mixture was added sodium hydroxide (0.075 mole), then the mixture was refluxed with stirring for 4–5 h. The reaction was monitored through TLC. After completion, the reaction mixture was poured in ice-cold water, acidified with 4 N HCl and the solid was filtered, dried, and recrystallized from ethanol.
General procedure for the synthesis of 5-(1,3-diphenyl-1H-pyrazol-4-yl)-3-phenyl-4,5-dihydropyrazole-1-carbothioamide 29–48
Equimolar quantities (0.01 mole) of chalcone and thiosemicarbazide were refluxed in presence of sodium hydroxide (0.025 mole) in 25 mL ethanol for 3 h. White solid separation occurs in the reaction vessel itself. After the completion of reaction, the precipitated solid was filtered, washed with water, and driedCitation24,Citation25.
5-(1,3-Diphenyl-1H-pyrazol-4-yl)-3-phenyl-4,5-dihydropyrazole-1-carbothioamide (29)
Yield: 64%; Rf: 0.32; m.p.: 245–246 °C; FT-IR (cm−1): 3413, 3269 (NH2), 1587 (C = N), 1363 (C = S); 1H-NMR (δ ppm, DMSO-d6, 400 MHz): 3.22 (dd, 1H, J = 3.6 & 17.6 Hz), 3.91 (dd, 1H, J = 11.2 & 17.8 Hz), 6.03 (dd, 1H, J = 3.6 & 11.4 Hz), 7.24 (t, 1H, H-4′, J = 7.2 Hz), 7.38–7.49 (m, 8H, H-3,4,5,3′,5′,3″,4″,5″), 7.78 (d, 2H, J = 7.6 Hz, H-2″,6″), 7.83–7.86 (m, 5H, H-2,6,2′,6′ & one proton of NH2), 8.07 (bs, 1H, NH2, D2O exchangeable), 8.13 (s, 1H, pyrazole ring); 13C-NMR (δ ppm, DMSO-d6, 100 MHz): 42.81, 56.16, 118.57, 124.76, 126.29, 126.64, 127.66, 128.37, 128.44, 129.05, 129.95, 130.93, 131.56, 133.53, 139.80, 149.68, 155.24, 176.52; Mass (m/z): 424 (M++1). Anal Calcd. for C25H21N5S: C, 70.90; H, 5.00; N, 16.54; Found: C, 70.92; H, 4.98; N, 16.55.
3-(4-Chlorophenyl)-5-(1,3-diphenyl-1H-pyrazol-4-yl)-4,5-dihydropyrazole-1-carbothioamide (30)
Yield: 60%; Rf: 0.24; m.p.: 252–253 °C; FT-IR (cm−1): 3422, 3254 (NH2), 1582 (C = N), 1364 (C = S); 1H-NMR (δ ppm, DMSO-d6, 400 MHz): 3.21 (dd, 1H, J = 3.6 & 17.8 Hz), 3.88 (dd, 1H, J = 11.6 & 18.0 Hz), 6.02 (dd, 1H, J = 3.6 & 11.2 Hz), 7.23 (t, 1H, H-4′, J = 7.6 Hz), 7.36–7.46 (m, 5H, H-3,4,5,3′,5′), 7.48 (d, 2H, J = 8.4 Hz), H-3″,5″), 7.75 (d, 2H, J = 7.2 Hz, H-2,6), 7.82 (d, 2H, J = 8.0 Hz, H-2′,6′), 7.86 (d, 3H, J = 8.4 Hz, H-2″,6″ & one proton of NH2 merged), 8.09 (bs, 1H, NH2, D2O exchangeable), 8.14 (s, 1H, pyrazole ring); Mass (m/z): 458 (M++1), 459 (M++2). Anal Calcd. for C25H20ClN5S: C, 65.56; H, 4.40; N, 15.29; Found: C, 70.88; H, 4.41; N, 15.30.
5-(1,3-Diphenyl-1H-pyrazol-4-yl)-3-p-tolyl-4,5-dihydropyrazole-1-carbothioamide (31)
Yield: 56%; Rf: 0.46; m.p.; 259–260 °C; FT-IR (cm−1): 3422, 3252 (NH2), 1580 (C = N), 1365 (C = S); 1H-NMR (δ ppm, DMSO-d6, 400 MHz): 2.32 (s, 3H, CH3), 3.19 (dd, 1H, J = 3.6 & 17.8 Hz), 3.88 (dd, 1H, J = 11.2 & 17.6 Hz), 6.02 (dd, 1H, J = 3.6 & 11.2 Hz), 7.23 (d, 2H, J = 8.0 Hz, H-3″,5″), 7.25 (t, 1H, J = 7.6 Hz, H-4′), 7.38–7.49 (m 5H, H-3,4,5,3′,5′), 7.73 (d, 2H, J = 8.4 Hz, H-2,6), 7.78 (d, 3H, J = 7.2 Hz, H-2″,6″ & one proton of NH2 merged), 7.82 (d, 2H, J = 8.0 Hz, H-2′,6′), 8.02 (bs, 1H, NH2, D2O exchangeable), 8.10 (s, 1H, pyrazole ring); Mass (m/z): 438 (M++1). Anal Calcd. for C26H23N5S: C, 71.37; H, 5.30; N, 16.01; Found: C, 71.39; H, 5.29; N, 16.00.
5-(1,3-Diphenyl-1H-pyrazol-4-yl)-3-(4-methoxyphenyl)-4,5-dihydropyrazole-1-carbothioamide (32)
Yield: 48%; Rf: 0.35; m.p.: 242–243 °C; FT-IR (cm−1): 3422, 3251 (NH2), 1586 (C = N), 1359 (C = S); 1H-NMR (δ ppm, DMSO-d6, 400 MHz): 3.19 (dd, 1H, J = 3.2 & 17.8 Hz), 3.78 (s, 3H, OCH3), 3.86 (dd, 1H, J = 11.6 & 17.6 Hz), 6.01 (dd, 1H, J = 3.2 & 11.2 Hz), 6.96 (d, 2H, J = 8.8 Hz, H-3″,5″), 7.24 (t, 1H, J = 7.2 Hz, H-4′), 7.38–7.49 (m, 5H, H-3,4,5,3′,5′), 7.78 (d, 4H, J = 8.4 Hz, H-2,6,2″,6″), 7.83 (d, 2H, J = 8.0 Hz, H-2′,6′), 7.76 (bs, 1H, NH2, D2O exchangeable), 8.00 (bs, 1H, NH2, D2O exchangeable), 8.10 (s, 1H, pyrazole ring); Mass (m/z): 454 (M++1). Anal Calcd. for C26H23N5OS: C, 68.85; H, 5.11; N, 15.44; Found: C, 68.83; H, 5.09; N, 15.42.
3-(4-Aminophenyl)-5-(1,3-diphenyl-1H-pyrazol-4-yl)-4,5-dihydropyrazole-1-carbothioamide (33)
Yield: 54%; Rf: 0.52; m.p.: 249–250 °C; FT-IR (cm−1): 3457, 3276 (NH2), 3391, 3329 (NH2), 1588 (C = N), 1361 (C = S); 1H-NMR (δ ppm, DMSO-d6, 400 MHz): 3.08 (dd, 1H, J = 3.2 & 17.2 Hz), 3.78 (dd, 1H, J = 11.2 & 17.0 Hz), 5.68 (bs, 2H, NH2, D2O exchangeable), 5.98 (dd, 1H, J = 3.2 & 11.0 Hz), 6.52 (d, 2H, J = 7.6 Hz, H-3″,5″), 7.23 (t, 1H, J = 7.6 Hz, H-4′), 7.37–7.54 (m, 8H, H-3,4,5,3′,5′,2″,6″, one proton of NH2), 7.78 (d, 2H, J = 8.0 Hz, H-2,6), 7.81 (d, 3H, J = 8.8 Hz, H-2′,6′& one proton of NH2 merged), 8.02 (s, 1H, pyrazole ring); Mass (m/z): 439 (M++1). Anal Calcd. for C25H22N6S: C, 68.47; H, 5.06; N, 19.16; Found: C, 68.45; H, 5.04; N, 19.14.
5-(3-(4-Chlorophenyl)-1-phenyl-1H-pyrazol-4-yl)-3-phenyl-4,5-dihydropyrazole-1-carbothioamide (34)
Yield: 49%; Rf: 0.28; m.p.: 271–272 °C; FT-IR (cm−1): 3406, 3236 (NH2), 1589 (C = N), 1376 (C = S); 1H-NMR (δ ppm, DMSO-d6, 400 MHz): 3.23 (dd, 1H, J = 3.6 & 17.8 Hz), 3.90 (dd, 1H, J = 11.2 & 17.8 Hz), 6.01 (dd, 1H, J = 3.6 & 11.2 Hz),7.24 (t, 1H, J = 7.2 Hz, H-4′), 7.42–7.45 (m, 5H, H-3″,4″,5″,3′,5′), 7.51 (d, 2H, J = 8.4 Hz, H-3,5), 7.79 (d, 2H, J = 8.4 Hz, H-2′,6′), 7.82–7.85 9m, 5H, H-2,6,2″,6″ & one proton of NH2), 8.09 (bs, 1H, NH2, D2O exchangeable), 8.17 (s, 1H, pyrazole ring); Mass (m/z): 458 (M++1), 459 (M++2). Anal Calcd. for C25H20ClN5S: C, 65.56; H, 4.40; N, 15.29; Found: C, 65.55; H, 4.38; N, 15.30.
3-(4-Chlorophenyl)-5-(3-(4-chlorophenyl)-1-phenyl-1H-pyrazol-4-yl)-4,5-dihydropyrazole-1-carbothioamide (35)
Yield: 52%; Rf: 0.42; m.p.: 260–261 °C; FT-IR (cm−1): 3426, 3264 (NH2), 1577 (C = N), 1358 (C = S); 1H-NMR (δ ppm, DMSO-d6, 400 MHz): 3.22 (dd, 1H, J = 3.6 & 18.0 Hz), 3.87 (dd, 1H, J = 11.6 & 17.6 Hz), 6.00 (dd, 1H, J = 3.6 & 11.4 Hz), 7.25 (t, 1H, J = 7.2 Hz, H-4′), 7.42 (t, 2H, J = 8.0 Hz, H-3′,5′), 7.49–7.51 (d, 2H, J = 8.4 Hz, H-3″,5″), 7.50–7.52 (d, 2H, J = 8.0 Hz, H-3,5), 7.77 (d, 2H, J = 8.4 Hz, H-2′,6′), 7.83 (d, 2H, J = 8.0 Hz, H-2″,6″), 7.85 (d, 2H, J = 8.8 Hz, H-2,6), 7.91 (bs, 1H, NH2, D2O exchangeable), 8.12 (bs, 1H, NH2, D2O exchangeable), 8.19 (s, 1H, pyrazole ring); Mass (m/z): 492 (M++1), 493 (M++2). Anal Calcd. for C25H19Cl2N5S: C, 60.98; H, 3.89; N, 14.22; Found: C, 61.00; H, 3.87; N, 14.23.
5-(3-(4-Chlorophenyl)-1-phenyl-1H-pyrazol-4-yl)-3-p-tolyl-4,5-dihydropyrazole-1-carbothioamide (36)
Yield: 54%; Rf: 0.56; m.p.: 272–273 °C; FT-IR (cm−1): 3428, 3268 (NH2), 1577 (C = N), 1361 (C = S); 1H-NMR (δ ppm, DMSO-d6, 400 MHz): 2.32 (s, 3H, CH3), 3.20 (dd, 1H, J = 3.6 & 17.8 Hz), 3.87 (dd, 1H, J = 11.6 & 17.6 Hz), 5.99 (dd, 1H, J = 3.6 & 11.4 Hz), 7.23–7.28 (m, 3H, H-4′,3″,5″), 7.41 (t, 2H, J = 8.0 Hz, H-3′,5′), 7.51 (d, 2H, J = 8.4 Hz, H-3,5), 7.73 (d, 2H, J = 8.0 Hz, H-2′,6′), 7.79 (d, 3H, J = 8.4 Hz, H-2″,6″ & one proton of NH2 merged), 7.83 (d, 2H, J = 8.0 Hz, H-2,6), 8.05 (bs, 1H, NH2, D2O exchangeable), 8.15 (s, 1H, pyrazole ring); Mass (m/z): 472 (M++1), 473 (M++2). Anal Calcd. for C26H22ClN5S: C, 66.16; H, 4.70; N, 14.84; Found: C, 66.15; H, 4.68; N, 14.85.
5-(3-(4-Chlorophenyl)-1-phenyl-1H-pyrazol-4-yl)-3-(4-methoxyphenyl)-4,5-dihydropyrazole-1-carbothioamide (37)
Yield: 53%; Rf: 0.38; m.p.: 262–263 °C; FT-IR (cm−1): 3426, 3260 (NH2), 1585 (C = N), 1363 (C = S); 1H-NMR (δ ppm, DMSO-d6, 400 MHz): 3.19 (dd, 1H, J = 3.6 & 17.8 Hz), 3.78 (s, 3H, OCH3), 3.86 (dd, 1H, J = 11.6 & 18.0 Hz), 5.98 (dd, 1H, J = 3.6 & 11.4 Hz), 6.97 (d, 2H, J = 8.8 Hz, H-3″,5″), 7.25 (t, 1H, J = 7.6 Hz, H-4′), 7.42 (t, 2H, J = 8.0 Hz, H-3′,5′), 7.51 (d, 2H, J = 8.4 Hz, H-3,5), 7.75 (bs, 1H, NH2), 7.78–7.80 (d, 2H, J = 8.8 Hz, H-2′,6′), 7.79–7.81 (d, 2H, J = 8.4 Hz, H-2″,6″), 7.83 (d, 2H, J = 8.0 Hz, H-2,6), 8.00 (bs, 1H, NH2, D2O exchangeable), 8.14 (s, 1H, pyrazole ring); Mass (m/z): 488 (M++1), 489 (M++2). Anal Calcd. for C26H22ClN5OS: C, 63.99; H, 4.54; N, 14.35; Found: C, 64.00; H, 4.55; N, 14.33.
3-(4-Aminophenyl)-5-(3-(4-chlorophenyl)-1-phenyl-1H-pyrazol-4-yl)-4,5-dihydropyrazole-1-carbothioamide (38)
Yield: 56%; Rf: 0.33; m.p.: 252–253 °C; FT-IR (cm−1): 3455, 3273 (NH2), 3391, 3323 (NH2), 1590 (C = N), 1360 (C = S); 1H-NMR (δ ppm, DMSO-d6, 400 MHz): 3.09 (dd, 1H, J = 2.4 & 17.6 Hz), 3.78 (dd, 1H, J = 10.8 & 17.4 Hz), 5.70 (bs, 2H, NH2, D2O exchangeable), 5.96 (dd, 1H, J = 2.8 & 10.8 Hz), 6.52 (d, 2H, J = 8.0 Hz, H-3″,5″), 7.25 (t, 1H, J = 7.2 Hz, H-4′), 7.42 (t, 2H, J = 7.6 Hz, H-3′,5′), 7.49–7.54 (m, 5H, H-3,5,2″,6″ and proton of NH2), 7.80–7.84 (m, 4H, H-2,6,2′,6′), 7.87 (bs, 1H, NH2), 8.07 (s, 1H, pyrazole ring); 13C-NMR (δ ppm, DMSO-d6, 100 MHz): 42.22, 55.09, 113.12, 117.68, 118.11, 124.52, 125.82, 126.27, 128.61, 128.74, 129.48, 129.52, 131.86, 132.65, 139.16, 147.92, 151.35, 155.58, 174.82. Mass (m/z): 473 (M++1), 474 (M++1). Anal Calcd. for C25H21ClN6S: C, 63.48; H, 4.48; N, 17.77; Found: C, 63.49; H, 4.46; N, 17.79.
3-Phenyl-5-(1-phenyl-3-p-tolyl-1H-pyrazol-4-yl)-4,5-dihydropyrazole-1-carbothioamide (39)
Yield: 60%; Rf: 0.55; m.p.: 242–243 °C; FT-IR (cm−1): 3407, 3267 (NH2), 1591 (C = N), 1368 (C = S); 1H-NMR (δ ppm, DMSO-d6, 400 MHz): 2.34 (s, 3H, CH3), 3.19 (dd, 1H, J = 3.6 & 17.8 Hz), 3.89 (dd, 1H, J = 11.2 & 17.8 Hz), 6.03 (dd, 1H, J = 3.6 & 11.2 Hz), 7.23 (t, 1H, J = 7.6 Hz, H-4′), 7.26 (d, 2H, J = 7.6 Hz, H-3,5), 7.41–7.45 (m, 5H, H-3′,5′,3″,4″,5″), 7.66 (d, 2H, J = 8.0 Hz, H-2″,6″), 7.81–7.85 (m,5H, H-2,6,2′,6′ & one proton of NH2 merged), 8.08 (bs, 1H, NH2, D2O exchangeable), 8.10 (s, 1H, pyrazole ring); Mass (m/z): 438 (M++1). Anal Calcd. for C26H23N5S: C, 71.37; H, 5.30; N, 16.01; Found: C, 71.35; H, 5.29; N, 16.00.
3-(4-Chlorophenyl)-5-(1-phenyl-3-p-tolyl-1H-pyrazol-4-yl)-4,5-dihydropyrazole-1-carbothioamide (40)
Yield: 60%; Rf: 0.35; m.p.: 269–270 °C; FT-IR (cm−1): 3418, 3260 (NH2), 1572 (C = N), 1361 (C = S); 1H-NMR (δ ppm, DMSO-d6, 400 MHz): 2.32 (s, 3H, CH3), 3.21 (dd, 1H, J = 4.0 & 11.2 Hz), 3.88 (dd, 1H, J = 10.8 & 17.0 Hz), 6.01 (dd, 1H, J = 4.0 & 11.2 Hz), 7.22 (t, 1H, J = 7.6 Hz, H-4′), 7.24 (d, 2H, J = 8.4 Hz, H-3,5), 7.41 (t, 2H, J = 8.0 Hz, H-3′,5′), 7.46 (d, 2H, J = 8.8 Hz, H-3″,5″), 7.80 (d, 2H, J = 8.8 Hz, H-2′,6′), 7.83–7.85 (m, 5H, H-2″,6″, 2,6 and one proton of NH2 merged), 8.01 (bs, 1H, NH2), 8.06 (s, 1H, pyrazole ring); Mass (m/z): 472 (M++1), 473 (M++2). Anal Calcd. for C26H22ClN5S: C, 66.16; H, 4.70; N, 14.84; Found: C, 66.15; H, 4.68; N, 14.86.
5-(1-Phenyl-3-p-tolyl-1H-pyrazol-4-yl)-3-p-tolyl-4,5-dihydropyrazole-1-carbothioamide (41)
Yield: 52%; Rf: 0.30; m.p.: 259–260 °C; FT-IR (cm−1): 3428, 3266 (NH2), 1578 (C = N), 1363 (C = S); 1H-NMR (δ ppm, DMSO-d6, 400 MHz): 2.27 (s, 3H, CH3), 2.32 (s, 3H, CH3), 3.16 (dd, 1H, J = 4.0 & 11.2 Hz), 3.86 (dd, 1H, J = 10.8 & 17.0 Hz), 6.01 (dd, 1H, J = 4.0 & 11.2 Hz), 7.22 (d, 2H, J = 8.4 Hz, H-3″,5″), 7.26 (d, 2H, J = 7.6 Hz, H-3,5), 7.22–7.28 (1H, H-4′ merged), 7.41 (t, 2H, J = 8.0 Hz, H-3′,5′), 7.65 (d, 2H, J = 7.6 Hz, H-2,6), 7.73 (d, 2H, J = 7.6 Hz, H-2″,6″), 7.79 (bs, 1H, NH2), 7.81(d, 2H, J = 8.0 Hz, H-2′,6′), 8.04 (bs, 1H, NH2),8.08 (s, 1H, pyrazole ring); Mass (m/z): 452 (M++1). Anal Calcd. for C27H25N5S: C, 71.81; H, 5.58; N, 15.51; Found: C, 71.80; H, 5.60; N, 15.50.
3-(4-Methoxyphenyl)-5-(1-phenyl-3-p-tolyl-1H-pyrazol-4-yl)-4,5-dihydropyrazole-1-carbothioamide (42)
Yield: 55%; Rf: 0.27; m.p.: 236–237 °C; FT-IR (cm−1): 3423, 3249 (NH2), 1586 (C = N), 1365 (C = S); 1H-NMR (δ ppm, DMSO-d6, 400 MHz): 2.29 (s, 3H, CH3), 3.75 (s, 3H, OCH3), 3.21 (dd, 1H, J = 4.0 & 11.2 Hz), 3.86 (dd, 1H, J = 10.8 & 17.0 Hz), 6.03 (dd, 1H, J = 4.0 & 11.2 Hz), 6.94 (d, 2H, J = 8.8 Hz, 3″,5″), 7.21 (t, 1H, J = 7.6 Hz, H-4′), 7.26 (d, 2H, J = 7.6 Hz, H-3,5), 7.41 (t, 2H, J = 8.0 Hz, H-3′,5′), 7.72 (d, 2H, J = 7.6 Hz, H-2,6), 7.75 (bs, 1H, NH2), 7.77 (d, 2H, J = 7.6 Hz, H-2″,6″), 7.80 (d, 2H, J = 8.0 Hz, H-2′,6′), 7.88 (bs, 1H, NH2), 8.03 (s, 1H, pyrazole ring); Mass (m/z): 468 (M++1). Anal Calcd. for C27H25N5OS: C, 69.35; H, 5.39; N, 14.98; Found: C, 69.33; H, 5.41; N, 15.00.
3-(4-Aminophenyl)-5-(1-phenyl-3-p-tolyl-1H-pyrazol-4-yl)-4,5-dihydropyrazole-1-carbothioamide (43)
Yield: 48%; Rf: 0.59; m.p.: 241–242 °C; FT-IR (cm−1): 3449, 3271 (NH2), 3382, 3317, (NH2), 1591 (C = N), 1359 (C = S); 1H-NMR (δ ppm, DMSO-d6, 400 MHz): 2.35 (s, 3H, CH3), 3.04 (dd, 1H, J = 3.2 & 17.6 Hz), 3.76 (dd, 1H, J = 10.8 & 17.4 Hz), 5.68 (bs, 2H, NH2, D2O exchangeable), 5.97 (dd, 1H, J = 3.6 & 11.2 Hz), 6.51 (d, 2H, J = 8.8 Hz, H-3″,5″), 7.22 (t, 1H, J = 7.6 Hz, H-4′), 7.26 (d, 2H, J = 8.4 Hz, H-3,5), 7.40 (t, 2H, J = 8.0 Hz, H-3′,5′), 7.48 (d, 2H, J = 8.8 Hz, H-2″,6″), 7.54 (bs, 1H, NH2), 7.66 (d, 2H, J = 8.0 Hz, H-2,6), 7.80 (d, 2H, J = 7.6 Hz, H-2′,6′), 7.84 (bs, 1H, NH2), 7.98 (s, 1H, pyrazole ring); Mass (m/z): 453 (M++1). Anal Calcd. for C26H24N6S: C, 69.00; H, 5.35; N, 18.57; Found: C, 68.98; H, 5.33; N, 18.55.
5-(3-(4-Methoxyphenyl)-1-phenyl-1H-pyrazol-4-yl)-3-phenyl-4,5-dihydropyrazole-1-carbothioamide (44)
Yield: 50%; Rf: 0.49; m.p.: 255–256 °C; FT-IR (cm−1): 3423, 3268 (NH2), 1592 (C = N), 1365 (C = S); 1H-NMR (δ ppm, DMSO-d6, 400 MHz): 3.79 (s, 3H, OCH3), 3.19 (dd, 1H, J = 4.0 & 11.2 Hz), 3.86 (dd, 1H, J = 10.8 & 17.0 Hz), 6.04 (dd, 1H, J = 4.0 & 11.2 Hz), 7.03 (d, 2H, J = 8.4 Hz, H-3,5), 7.23 (t, 1H, J = 7.2 Hz, H-4′), 7.40–7.45 (m, 5H, H-3′,5′,3″,4″,5″), 7.73–7.77 (m, 4H, H-2,6,2″,6″), 7.80–7.82 (m, 3H, H-2′,6′ and one proton of NH2 merged), 7.98 (bs, 1H, NH2), 8.09 (s, 1H, pyrazole ring); Mass (m/z): 454 (M++1). Anal Calcd. for C26H23N5OS: C, 68.85; H, 5.11; N, 15.44; Found: C, 68.83; H, 5.09; N, 15.46.
3-(4-Chlorophenyl)-5-(3-(4-methoxyphenyl)-1-phenyl-1H-pyrazol-4-yl)-4,5-dihydropyrazole-1-carbothioamide (45)
Yield: 54%; Rf: 0.38; m.p.: 232–233 °C; FT-IR (cm−1): 3428, 3265 (NH2), 1594 (C = N), 1373 (C = S); 1H-NMR (δ ppm, DMSO-d6, 400 MHz): 3.18 (dd, 1H, J = 3.2 & 17.6 Hz), 3.77 (s, 3H, OCH3), 3.85 (dd, 1H, J = 11.6 & 17.8 Hz), 6.00 (dd, 1H, J = 3.6 & 11.2 Hz), 6.99 (d, 2H, J = 8.0 Hz, H-3,5), 7.22 (m, 3H, H-3″,5″,4′), 7.40 (t, 2H, J = 7.2 Hz, H-3′,5′), 7.69 (d, 2H, J = 8.0 Hz, H-2,6), 7.72 (d, 2H, J = 8.0 Hz, H-2′,6′), 7.78 (bs, 1H, NH2), 7.80 (d, 2H, J = 8.0 Hz, H-2″,6″), 8.02 (bs, 1H, NH2), 8.06 (s, 1H, pyrazole ring); Mass (m/z): 488 (M++1), 489(M++2). Anal Calcd. for C26H22ClN5OS: C, 63.99; H, 4.54; N, 14.35; Found: C, 63.98; H, 4.55; N, 14.30.
5-(3-(4-Methoxyphenyl)-1-phenyl-1H-pyrazol-4-yl)-3-p-tolyl-4,5-dihydropyrazole-1-carbothioamide (46)
Yield: 60%; Rf: 0.31; m.p.: 245–246 °C; FT-IR (cm−1): 3433, 3273 (NH2), 1581 (C = N), 1358 (C = S); 1H-NMR (δ ppm, DMSO-d6, 400 MHz): 2.31 (s, 3H, CH3), 3.15 (dd, 1H, J = 3.2 & 17.0 Hz), 3.78 (s, 3H, OCH3), 3.84 (dd, 1H, J = 11.2 & 17.2 Hz), 6.00 (dd, 1H, J = 3.2 & 11.4 Hz), 7.00 (d, 2H, J = 8.4 Hz, H-3,5), 7.22 (3H, H-4′,3″,5″), 7.40 (t, 2H, J = 7.6 Hz, H-3′,5′), 7.69 (d, 2H, J = 8.8 Hz, H-2,6), 7.72 (d, 2H, J = 8.4 Hz, H-2″,6″), 7.80 (d, 2H, J = 8.0 Hz, H-2′,6′), 7.79 (bs, 1H, NH2), 8.02 (bs, 1H, NH2),8.06 (s, 1H, pyrazole ring); Mass (m/z): 468 (M++1). Anal Calcd. for C27H25N5OS: C, 69.35; H, 5.39; N, 14.98; Found: C, 69.36; H, 5.40; N, 14.96.
3-(4-Methoxyphenyl)-5-(3-(4-methoxyphenyl)-1-phenyl-1H-pyrazol-4-yl)-4,5-dihydropyrazole-1-carbothioamide (47)
Yield: 61%; Rf: 0.43; m.p.: 234–235 °C; FT-IR (cm−1): 3424, 3250 (NH2), 1587 (C = N), 1365 (C = S); 1H-NMR (δ ppm, DMSO-d6, 400 MHz): 3.15 (dd, 1H, J = 3.2 & 17.6 Hz), 3.78 (s, 3H, OCH3), 3.79 (s, 3H, OCH3), 3.83 (dd, 1H, J = 11.2 & 17.8 Hz), 5.99 (dd, 1H, J = 3.2 & 11.2 Hz), 6.96 (d, 2H, J = 8.4 Hz, H-3,5), 7.01 (d, 2H, J = 8.8 Hz, H-3″,5″), 7.22 (t, 1H, J = 7.2 Hz, H-4′), 7.40 (t, 2H, J = 8.0 Hz, H-3′,5′), 7.70 (d, 2H, J = 8.4 Hz, H-2′,6′), 7.75 (bs, 1H, NH2), 7.77 (d, 2H, J = 8.8 Hz, H-2″,6″), 7.80 (d, 2H, J = 8.4 Hz, H-2,6), 7.99 (bs, 1H, NH2), 8.06 (s, 1H, pyrazole ring); 13C-NMR (δ ppm, DMSO-d6, 100 MHz): 42.78, 55.63, 55.86, 56.09, 114.49, 114.54, 118.46, 124.02, 124.40, 125.96, 126.07, 126.47, 129.38, 129.62, 129.93, 139.84, 149.51, 155.19, 159.57, 161.63, 176.14; Mass (m/z): 484 (M++1). Anal Calcd. for C27H25N5O2S: C, 67.06; H, 5.21; N, 14.48; Found: C, 67.04; H, 5.20; N, 14.50.
3-(4-Aminophenyl)-5-(3-(4-methoxyphenyl)-1-phenyl-1H-pyrazol-4-yl)-4,5-dihydropyrazole-1-carbothioamide (48)
Yield: 52%; Rf: 0.40; m.p.: 255–256 °C; FT-IR (cm−1): 3483, 3204 (NH2), 3418, 3308 (NH2), 1565 (C = N), 1347 (C = S); 1H-NMR (δ ppm, DMSO-d6, 400 MHz): 3.05 (dd, 1H, J = 3.2 & 17.6 Hz), 3.75 (dd, 1H, J = 11.2 & 17.4 Hz), 3.79 (s, 3H, OCH3), 5.68 (bs, 2H, NH2, D2O exchangeable), 5.96 (dd, 1H, J = 3.2 & 10.8 Hz), 6.51 (d, 2H, J = 8.4 Hz, H-3″,5″), 7.01 (d, 2H, J = 8.8 Hz, H-3,5), 7.22 (t, 1H, J = 7.2 Hz, H-4′), 7.40 (t, 2H, J = H-3′,5′), 7.48 (d, 2H, J = 8.4 Hz, H-2″,6″), 7.54 (bs, 1H, NH2), 7.70 (d, 2H, J = 8.8 Hz, H-2,6), 7.79 (d, 2H, J = 8.0 Hz, H-2′,6′), 7.81 (bs, 1H, NH2), 7.97 (s, 1H, pyrazole ring); Mass (m/z): 469 (M++1). Anal Calcd. for C26H24N6OS: C, 66.64; H, 5.16; N, 17.94; Found: C, 66.66; H, 5.13; N, 17.95.
Pharmacology
In vitro antimalarial activity
In vitro drug sensitivity of the synthesized compounds was assessed using Trager and JensenCitation26 method as described by Dua et al.Citation27. Chloroquine sensitive 3D7 P. falciparum strains were used for the study. Culture was maintained in A+ erythrocytes using RPMI 1640 medium supplemented with AB+ human serum (10%), sodium bicarbonate (0.2%), HEPES buffer (25 mM) and gentamycin (50 µg mL−1). The culture was treated with different drug concentrations. After 72 h of incubation, blood smears were prepared and stained with JSB I and JSB II. Percentage maturation of schizonts against control was determined. Chloroquine was used as the standard reference. The inhibitory concentration value which kills 50% of the parasites (IC50) was considered for anti-plasmodial activity. IC50 values were determined using HN-NonLin V1.1.
Compounds with IC50 less than 5 µM were further tested for cytotoxicity study to find out the selectivity index (SI) of the synthesised compounds.
Cytotoxicity assay
Cytotoxicity of the compounds was carried out with Vero cell line using Mosmann methodCitation28 with certain modifications. The cells were incubated with different dilutions of selected compounds for 72 h using MTT as reagent for the detection of cytotoxicity. Fifty percent cytotoxic concentration (CC50) values represent the concentration of compound required to kill 50% of the fibroblast cells. The SI was calculated using the formula
QSAR
In order to get better insights into the structural requirements of the title compounds to act as antimalarials QSAR of the compounds was performed using PHASE module of SchrödingerCitation29. The structures were drawn on maestro interface. The IC50 of the compounds were converted to their molar values and subsequently calculated to free energy-related terms, i.e. pIC50 (). Activity threshold was set to 4.75 to discriminate between active and inactive ligands. The standardized structures were then subjected to conformational search by OPLS 2005 force field using the confogen module of the Schrödinger software. After generating the conformers, various pharmacophoric features were defined.
Table 1. IC50 and SI values of the synthesised compounds.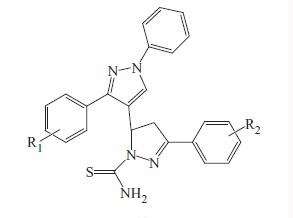
Active analogue approach was used to identify common pharmacophore hypotheses (CPHs). Common pharmacophores were selected from the conformations of the set of active ligands using a tree-based partitioning technique, which groups together similar pharmacophores according to their intersite distances. After the generation of pharmacophores their scoring, clustering and ranking was done. The purpose of scoring was to identify the suitable hypothesis, which can explain the overall aspects of the structural requirements of the receptor site. Highest scoring hypothesis was subsequently used for the development of 3D QSAR models. For the present study, ADHRR.9 hypothesis, containing one H-acceptor, one H-donor, an hydrophobic core and two aromatic ring systems, was selected (). This hypothesis was chosen as it had structural features spread across the entire molecule.
The 3D-QSAR models were generated by combining the generated hypothesis with their schizontocidal activity data, to identify the overall aspects of molecular requirements which govern the biological activity. For generating the 3D-QSAR model, the dataset was divided into training set (70%) and test set (30%) by using random selection criteria. The training set molecules were further taken to generate 3D-QSAR models using an atom based QSAR method with a grid spacing of 1.0 Å. QSAR models were validated by predicting the activities of the test set. A three component (PLS factor) model was found to be the best statistical parameter to obtain an optimum model.
Results and discussion
Chemistry
Synthetic pathway which leads to the synthesis of title compounds (29–48), is outlined in . The synthesis involves four-step reaction. In the first step, various substituted acetophenones were reacted with phenylhydrazine to yield corresponding hydrazine derivatives (1–4). The pyrazole aldehydes (5–8), an intermediate required for chalcone (9–28) formation, were obtained by using Vilsmeier Haack formylation reactionCitation23. The key chalcone intermediates (9–28) were then synthesized through Claisen-Schmidt condensation of acetophenone derivatives in alcoholic solution containing sodium hydroxideCitation31. For preparation of pyrazoline, a variety of methods have been applied, among which refluxing of chalcone and thiosemicarbazide in methanol using alkaline condition (sodium hydroxide; 25 mmol) was found to be the best method with greater yields and shorter reaction timeCitation24,Citation25. The purity of the new synthesized compounds was checked by TLC. The structures of title compounds were determined on the basis of elemental analysis and various spectroscopic methods. Elemental analysis values were within the range of ± 0.4% of the theoretical value. In IR spectra, absorptions were observed at 1347–1376 cm−1 (C = S), 1572–1594 cm−1 (C = N) and 3204–3276 cm−1 and 3406–3483 cm−1 (NH2). In 1H-NMR spectra, the three protons HA, HM and HX of pyrazoline ring appeared as doublets of doublets ().
Scheme 1. Reagents and conditions: (i) Phenyl hydrazine, GAA, methanol, reflux; (ii) DMF, POCL3; (iii) Aromatic ketone, NaOH, methanol, reflux; (iv) Thiosemicarbazide, ethanol, reflux.
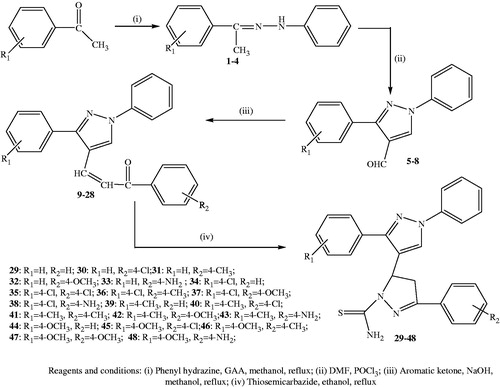
The HA proton (δ 3.26–3.30) appeared as doublet of doublet due to cisoid coupling to the HX proton and geminally to HM proton. The HM proton, deshielded to some extent by aryl group (δ 3.80–3.86), was coupled transoid to HX proton and geminally to HA proton. The third, HX proton (δ 5.63–5.68) was more deshielded due to effect of aryl group and electronegative nitrogen and was coupled transoid to the HM proton and cisoid to the HA proton. The two protons of CSNH2 appeared at different chemical shift values (δ 7.76–7.82 and 8.04–8.06) due to anisotropic effect exerted by thiocarbomyl group.
Pharmacology
In vitro antimalarial activity of the synthesized compounds was assessed using Trager and JensenCitation26 method as modified by Dua et al.Citation27 against the CQ-sensitive 3D7 strain of P. falciparum ().
Keeping all the aromatic features constant (), the compounds bear H, Cl, CH3 and OCH3 on the one of the phenyl ring (B) attached to the pyrazole ring and H, Cl, CH3, OCH3 and NH2 on the phenyl ring (C) attached to pyrazoline ring. The in vitro studies indicate that compounds with amino substitution on ring C gave the best inhibitory profiles, as shown by compounds 33, 38, 43 and 48 with IC50 values of 3.22, 2.09, 1.53 and 1.13 µM, respectively. The most active compound, i.e. 48 bears amino at one end and methoxy group at the other. Compounds 37, 42 and 47 having methoxy substitution on ring C also showed good activity with IC50 values of 7.86, 6.14 and 4.20 µM. The replacement of the methoxy by a methyl group and chloro atom decreases the antimalarial activity. The compounds with chloro substitution at both ring B and C elicit the least in vitro schizontocidal activity ().
The compounds 33, 38, 43, 47 and 48 with IC50-value less than 5 µM were further tested for cytotoxicity study on VERO cells using Mosmann methodCitation28 to find out their selectivity. The compounds showed SI ranging from 12 to 40. The most active compound 48 with IC50-value 1.13 µM also showed the highest SI amongst the tested compounds indicating that compounds belonging to this prototype would be less toxic ().
Following conclusions can be made on the basis of in vitro results:
Substitution with electron releasing group showed good activity; however, electropositive group with hydrogen bonding ability were found to be most active.
The replacement of electropositive group by electronegative group decreases the activity.
The least activity is elicited by compound with electronegative substitution at both rings B and C.
The compounds having in vitro antimalarial activity also showed less cytotoxicity.
QSAR
By taking into account the antimalarial activity of our synthesized compounds, a systemic pharmacophore modelling was performed and 3D-QSAR model was generated by PHASE module of Schrödinger, to support the assumption of flexible substituents responsible for better inhibition. Out of the different pharmacophore hypotheses, ADHRR.9 was selected. Active alignment of the data set is shown in .
The statistical parameters of the 3D-QSAR analysis are summarized in .
Table 2. Statistical parameters of the best model.
The model expresses 99% correlation (r2 = 0.9992). Observed and predicted activities show a close agreement with the regression line as indicated by the fitness graph (). This further validated the significance of the model. Validity of the model was also expressed by a determination coefficient on the test set (q2) of 0.79.The results of QSAR characteristics are given in .
Figure 6. Electron withdrawing, Hydrogen bond donor and hydrophobic characteristics of the hypothesis ADHRR.9.
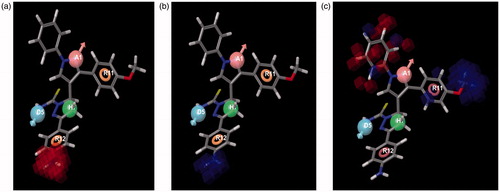
The 3D-QSAR results covering the electron withdrawing, hydrogen bond donor (HBD) and hydrophobic characteristics were generated. The hypothesis ADHRR.9 includes one hydrogen bond acceptor, one HBD, one hydrophobic core and two aromatic ring features. The 3D-QSAR model is in agreement with the in vitro results. The blue cubes of the 3D pharmacophore regions refer to ligand regions in which the specific feature is vital for activity, while the red cubes indicate that the presence of that particular feature will reduce the activity.
represents the electron withdrawing characteristics of the data set. The QSAR shows that presence of electron withdrawing groups at position-4 of Ring C reduces the activity. Further, substantiating the in vitro results, which show that compounds 30, 35 and 40 having chloro substitution at this position have poor inhibitory profiles.
represents the HBD characteristics of the data set. HBD at position-4 of Ring C improves the activity, as is evident from compounds 33, 38, 43 and 48. In the series, these compounds have the best inhibitory profiles in the data set.
represents the hydrophobic characteristics of the data set. It demonstrates that the presence of hydrophobic substitutions at position-4 of Ring B increases the inhibitory profiles of the compounds. For instance, compounds with methoxy substitution at this position among all series are better inhibitors.
Conclusion
In conclusion, 20 compounds were synthesised, characterized and tested against 3D7 strain of P. falciparum. The active compounds had an IC50 value in the range of 1.13–4.20 µM. The compounds with IC50 less than 5 µM were further evaluated for their cytotoxicity assay. The most active compound, 48 shows an IC50 of 1.13 µM (0.546 µg/mL) with reduced cytotoxicity. In order to support the systemic pharmacophore model, a predictive 3D-QSAR model was generated, which further substantiated the in vitro antimalarial results.
Supplementary material available online
Supplemental Material.pdf
Download PDF (24.7 MB)Acknowledgements
We are thankful to Jamia Hamdard, for providing research laboratory and instrumentation facilities (NMR, mass and IR) at the institute.
Declaration of interest
The authors report no declarations of interest. Authors are thankful to University Grants Commission, Government of India, New Delhi, for financial support.
References
- Tomar V, Bhattacharjee G, Kamaluddin, et al. Synthesis of new chalcone derivatives containing acridinyl moiety with potential antimalarial activity. Eur J Med Chem 2010;45:745–51
- Mane UR, Li H, Huang J, et al. Pyrido[1,2-a]pyrimidin-4-ones as antiplasmodial falcipain-2 inhibitors. Bioorg Med Chem 2012;20:6296–304
- Cochin SD, Paunescu E, Maes L, et al. Synthesis and antimalarial activity of new analogues of amodiaquine. Eur J Med Chem 2008;43:252–60
- Hans RH, Guantai EM, Lategan C, et al. Synthesis, antimalarial and antitubercular activity of acetylenic chalcones. Bioorg Med Chem Lett 2010;20:942–4
- Cunico W, Cechinel CA, Bonacorso HG, et al. Antimalarial activity of 4-(5-trifluoromethyl-1H-pyrazol-1-yl)-chloroquine analogues. Bioorg Med Chem Lett 2006;16:649–53
- Qirante J, Ruiz D, Gonzalez A, et al. Platinum(II) and palladium(II) complexes with (N,N′) and (C,N,N′)-ligands derived from pyrazole as anticancer and antimalarial agents: Synthesis, characterization and in vitro activities. J Inorg Biochem 2011;105:1720–8
- Bekhit AA, Hymete A, Asfaw H, Bekhit AEDA. Synthesis and biological evaluation of some pyrazole derivatives as anti-malarial agents. Archiv der Pharmazie 2012;345:147–54
- Wahab BFA, Latif EA, Mohamed HA, Awad GEA. Design and synthesis of new 4-pyrazolin-3-yl-1,2,3-triazoles and 1,2,3-triazol-4-yl-pyrazolin-1-ylthiazoles as potential antimicrobial agents. Eur J Med Chem 2012;52:263–8
- Bashir R, Ovais S, Yaseen S, et al. Synthesis of some new 1,3,5-trisubstituted pyrazolines bearing benzene sulfonamide as anticancer and anti-inflammatory agents. Bioorg Med Chem Lett 2011;21:4301–5
- Das N, Dash B, Dhanawat M, Shrivastava SK. Design, synthesis, preliminary pharmacological evaluation, and docking studies of pyrazoline derivatives. Chem Papers 2012;66:67–74
- Wanare G, Aher R, Kawathekar N, et al. Synthesis of novel α-pyranochalcones and pyrazoline derivatives as Plasmodium falciparum growth inhibitors. Bioorg Med Chem Lett 2010;20:4675–8
- Acharya BN, Saraswat D, Tiwari M, et al. Synthesis and antimalarial evaluation of 1, 3, 5-trisubstituted pyrazolines. Eur J Med Chem 2010;45:430–8
- Insuasty B, Montoya A, Becerra D, et al. Synthesis of novel analogs of 2-pyrazoline obtained from [(7-chloroquinolin-4-yl)amino]chalcones and hydrazine as potential antitumor and antimalarial agents. Eur J Med Chem 2013;67:252–62
- Chellan P, Nasser S, Vivas L, et al. Cyclopalladated complexes containing tridentate thiosemicarbazone ligands of biological significance: Synthesis, structure and antimalarial activity. J Organomet Chem 2010;695:2225–32
- Chellan P, Shunmoogam-Gounden S, Hendricks DT, et al. Synthesis, structure and in-vitro biological screening of palladium(II) complexes of functionalised salicylaldimine thiosemicarbazones as antimalarial and anticancer agents. Eur J Inorg Chem 2010;2010:3520–8
- Khanye SD, Smith GS, Lategan C, et al. Synthesis and in vitro evaluation of gold(I) thiosemicarbazone complexes for antimalarial activity. J Inorg Biochem 2010;104:1079–83
- Klayman DL, Bartosevich JF, Griffin TS, et al. 2-Acetylpyridine thiosemicarbazones. 1. A new class of potential antimalarial agents. J Med Chem 1979;22:855–62
- Pingaew R, Prachayasittikul S, Ruchirawat S. Synthesis, cytotoxic and antimalarial activities of benzoyl thiosemicarbazone analogs of isoquinoline and related compounds. Molecules 2010;15:988–96
- Khanye SD, Wan B, Franzblau SG, et al. Synthesis and in-vitro antimalarial and antitubercular activity of gold(III) complexes containing thiosemicarbazone ligands. J Organomet Chem 2011;696:3392–6
- WHO Guidelines for the Treatment of Malaria. 2nd ed. Geneva: WHO Press, World Health Organisation; 2010
- Capela R, Cabal GG, Rosenthal PJ, et al. Design and evaluation of primaquine-artemisinin hybrids as a multistage antimalarial strategy. Antimicrob Agents Chemother 2011;55:4698–706
- Marella A, Akhtar M, Shaquiquzzaman M, et al. Synthesis and in-vitro antimalarial evaluation of pyrazoline: A new antimalarial scaffold. J Pharm Res 2014;8:77–80
- Smith MB, March J. Aromatic substitution, electrophilic. In: March's advanced organic chemistry reactions, mechanisms, and structure. 6th ed. Hoboken (NJ): John Wiley & Sons, Inc.; 2007:724–5
- Desai NC, Joshi VV, Rajpara KM, et al. Facile synthesis of novel fluorine containing pyrazole based thiazole derivatives and evaluation of antimicrobial activity. J Fluorine Chem 2012;142:67–78
- Budakoti A, Abid M, Azam A. Syntheses, characterization and in-vitro antiamoebic activity of new Pd(II) complexes with 1-N-substituted thiocarbamoyl-3,5-diphenyl-2-pyrazoline derivatives. Eur J Med Chem 2007;42:544–51
- Trager W, Jensen JB. Human malaria parasites in continuous culture. Science 1976;193:673–7
- Dua VK, Sinha SN, Biswas S, et al. Isolation and antimalarial activity of peroxydisulfate oxidation products of primaquine. Bioorg Med Chem Lett 2002;12:3587–9
- Mosmann TJ. Rapid colorimetric assay for cellular growth and survival: application to proliferation and cytotoxicity assays. J Immunol Methods 1983;65:55–63
- Phase, version 3.5, Schrodinger. New York (NY): LLC; 2013
- Vora JJ, Vasava SB, Parmar KC, et al. Synthesis, spectral and microbial studies of some novel schiff base derivatives of 4-Methylpyridin-2-amine. Eur J Chem 2009;6:1205–10
- Kohler EP, Chadwell HM. Benzalacetophenone (Chalcone). Org Syntheses 1941;1:78