Abstract
Taxifolin, also known as dihydroquercetin, is a flavonoid commonly found in plants. Carbonic anhydrase (CA, EC 4.2.1.1) plays an important role in many critical physiological events including carbon dioxide (CO2)/bicarbonate () respiration and pH regulation. There are 16 known CA isoforms in humans, of which human hCA isoenzymes I and II (hCA I and II) are ubiquitous cytosolic isoforms. In this study, the inhibition properties of taxifolin against the slow cytosolic isoenzyme hCA I, and the ubiquitous and dominant rapid cytosolic isoenzyme hCA II were studied. Taxifolin, as a naturally bioactive flavonoid, has a Ki of 29.2 nM against hCA I, and 24.2 nM against hCA II. For acetylcholinesterase enzyme (AChE) inhibition, Ki parameter of taxifolin was determined to be 16.7 nM. These results clearly show that taxifolin inhibited both CA isoenzymes and AChE at the nM levels.
Introduction
In recent years, natural flavonoids have attracted significant interests in the scientific arena because of their versatility of uses and health-promoting effects. Flavonoids are important compounds that can be found in many plants, including edible fruits and vegetables. Flavonoids act as efficient antioxidants because of their ability to chelate transition metal ions, to scavenge free radicals and to interact with enzymesCitation1–3. It was reported that in vivo and in vitro studies of flavonoids showed that they have a positive impact on many important diseases, such as those affecting cardiovascular systemCitation1,Citation3.
Phenolic compounds contain at last a hydroxyl group (–OH) bonded to aromatic ring and are mildly acidicCitation4–11. Phenolic compounds are classified as simple phenols or polyphenols based on the number of phenol units in the molecule. This chemical class in terms of biological and pharmaceutical is very reactiveCitation12–24.
Taxifolin (dihydroquercetin) is a representative flavonoid compound. It is present in the plants from the Pinus genus and it is also found in citrus fruits and milk thistle seedsCitation25. Taxifolin has many a large area of biological effects, including anti-inflammatory, hepatoprotective effects and antitumor effectsCitation26. More important, taxifolin produces effective antioxidant effects, which contribute to its cardiovascular protective effects. Due to the reducing properties of its hydroxyl groups, taxifolin exhibits antioxidant activity and reacts with free radicalsCitation3.
Researchers have demonstrated that taxifolin decreased the production of lipid radicals in a concentration-dependent manner and reduced the peroxidase activity of the complex of cytochrome c combined with dioleoyl cardiolipin, which is critical for the onset of apoptosis. Taxifolin also ameliorated cerebral ischemia-reperfusion injury by inhibiting oxidative enzymes and reducing the overproduction of ROSCitation27,Citation28. Taxifolin inhibited recombinant human aldose reductase and the accumulation of sorbitol in human erythrocyte in diabetes. Taxifolin also retained the clarity of rat lenses incubated with glucose, suggesting that taxifolin might be effective in preventing osmotic stress in hyperglycemiaCitation29. However, there has been little work on possible beneficial effects of taxifolin for diabetic cardiomyopathy. Caspase enzymes are important factors for modulating the apoptotic cascade. These results have demonstrated that caspase-3 and caspase-9 activities were significantly inhibited by taxifolinCitation30. Additionally, it was shown that taxifolin decreases the angiotensin-converting enzyme activity in the aorta of aging ratsCitation31. Studies have shown the modulatory effects of flavonoids upon multiple enzymes involved in xenobiotic metabolism such as on various cytochrome P450 monooxygenase isoforms and phase II conjugation enzymes and on membrane transport systems including in drug excretionCitation32–35.
Carbonic anhydrase enzymes (CAs) are ubiquitous in all the living organisms. They have crucial physiological and pathological roles such as in fluid balance, calcification, pH regulation, carboxylation reactions, tumorigenicity, bone resorption, the synthesis of and in many other pathophysiological processesCitation36–39. The CA catalyzes the reversible hydration of carbon dioxide (CO2) and water (H2O) to bicarbonate (
) and a proton (H+)Citation40–46.
An enzyme inhibitor is a molecule that engages to an enzyme and decreases its activity. An inhibitor can prevent a substrate from binding the active site of the enzyme, thus hindering catalysis. It is well known that CA inhibitors (CAIs) bind to a catalytic zinc ion (Zn2+) in the active site of CA isoenzymes and block their activityCitation47–52. The clinical use of CAIs had been established as antiglaucoma, and as anticonvulsant agentsCitation48, diureticsCitation42 and antiobesity drugsCitation53–55 in the management of mountain sickness, gastric and duodenal ulcers, neurological disorders or osteoporosisCitation47. Additionally, CAIs have recently been used as hypoxic tumors management agentCitation56,Citation57. The first clinically used heterocyclic and aromatic sulfonamides were clinically used derivatives of acetazolamide (AZA), a known CAICitation58,Citation59. AZA is an inhibitor of CA and used for glaucoma, idiopathic intracranial hypertension, epilepsy and altitude sickness. To regenerate the basic form of CA isoenzyme, a H+ is transferred from the active site to the solvent. This H+ transportation may be supported by active site residues or by present buffers in the reaction medium. The fourth position is occupied by H2O at an acidic pH and is catalytically inactive. At higher pH, a H2O molecule binds to Zn2+ within the CA active site. Then, this proton transfer reaction transfers a H+ to the solvent, leaving an –OH groupCitation47,Citation49,Citation50,Citation52,Citation57.
Alzheimer’s disease (AD) is a neurological disorder in which the patient suffers from memory loss and impaired cognitive abilities. AD is a chronic neurological disorder in which the patient suffers from loss impaired cognitive abilities, deficits in activities of daily living and behavioral disturbancesCitation37,Citation60. According to the cholinergic hypothesis, memory impairment in patients suffering from AD results from decreased levels of the neurotransmitter acetylcholine (ACh) in the cortexCitation39. Acetylcholinesterase (AChE, EC: 3.1.1.7) is a hydrolase that plays a key role in cholinergic transmission through catalyzing the rapid hydrolysis of the neurotransmitter AChCitation61. AChE is a special carboxylic ester hydrolase that hydrolyses the esters of cholineCitation39,Citation60 to produce acetic acid and cholineCitation62.
AChE is found in high concentrations in the brain and in erythrocytesCitation63. AChE is a necessary enzyme for the nervous system. AChE inhibitors (AChEI) are used in the treatment of several neuromuscular diseases, and were studied for treatment of ADCitation39,Citation61,Citation64. The use of AChEI to block the cholinergic degradation of ACh is therefore considered to be a promising approach for the treatment of AD. Natural products might slow the progression of AD by simultaneously protecting neurons from oxidative stress and by acting as an AChEICitation65. Certain organophosphorus and carbamate derivatives are known to be the best inhibitors of AChE catalytic activityCitation66. Carbamate pesticides show their toxicity by irreversibly modifying the catalytic serine residue of AChE, and the inhibiting the AChECitation67. Recently, several studies supported that many active compounds from plant origins, including anthocyanins and terpenoids have AChE inhibition activityCitation68,Citation69. Additionally, some studies demonstrated that grape and blueberry anthocyaninCitation70,Citation71, grape skin anthocyaninCitation72 lycodine-type alkaloids from Lycopodiastrum casuarinoidesCitation73 have neuroprotective roles.
In this study, we identify the potential inhibition profile and associated mechanisms of taxifolin for human CA isoenzymes I and II (hCA I and II) and for AChE which are widely used enzyme in pharmacological industry.
Experimental
Purification of CA isoenzymes
Both hCA isoenzymes were purified by sepharose-4B-l-tyrosine-sulphanilamide affinity chromatographyCitation74–77. For this purpose, the lysate was adjusted with Tris buffer to pH 8.7 and applied to the affinity column. Then, protein content in the eluates was recorded spectrophotometrically at wavelength of 280 nm. SDS–PAGE (sodium dodecyl sulphate-polyacrylamide gel electrophoresis) was performed after purification of the enzymes. The isoenzymes purities were controlled by SDS–PAGE, and a single band was found for each CA isoenzymeCitation78. SDS–PAGE method has been detailed described previouslyCitation79 and was performed using acrylamide in the running (10%) and the stacking gel (3%), with 0.1% SDSCitation80,Citation81.
Determination of CA isoenzymes activities
Both CA isoenzyme activities were performed according to the procedure of Verpoorte et al.Citation82 and as described previously by our groupCitation81,Citation83. The absorbance change was spectrophotometrically reordered at 348 nm during 3 min at room temperature (25 °C). One unit of enzyme activity is expressed as 1 mol/L of released p-nitrophenol per minute at 25 °CCitation84. The protein quantity was spectrophotometrically determined at 595 nm during purification steps by the Bradford methodCitation85. Bovine serum albumin was used as the standard proteinCitation86,Citation87.
CA isoenzymes inhibition assay
The inhibition property of taxifolin against both CA isoenzymes was determined by hydrolysis of p-nitrophenylacetate to p-nitrophenol. The later molecule can be monitored spectrophotometricallyCitation49. The CO2 hydration reaction catalyzed by CA was first observed in the absence of taxifolin; the resulting rates were measured and used as a control for the CA isoenzymes. Also, the same reaction was measured in the presence of taxifolin. The percent inhibition was determined with (%) = [100 − (AS/AC) × 100], where AS is the absorbance of the sample containing taxifolin and AC is the absorbance of the control sample. The activity (%)–[taxifolin] graphs were drawn and the half maximal inhibitory concentration (IC50) values of taxifolin demonstrated >50% inhibition of CA isoenzymes were calculated after suitable dilutions. Ki values for taxifolin were determined for both isoenzymes. For this purpose to determine the Ki values, taxifolin was tested at three different concentrations. Ki is the binding affinity constant of the taxifolin to CA isoenzymes. NPA was used as the substrate at five different concentrations, and Lineweaver–Burk curves were drawnCitation88 in detail as described previouslyCitation89–92.
Acetylcholinesterase inhibition assay
Acetylcholinesterase enzyme inhibition assay was determined on commercially available purified AChE (Product no: C3389-Sigma–Aldrich, St. Louis, MO) from electric gel (Electrophorus electricus) based on the method of Ellman procedureCitation93. Acetyl thiocholine iodide (ATCI) was used as the substrate. Also, 5,5-dithiobis(2-nitrobenzoic) acid (DTNB) was used for the monitoring of AChE activity. Briefly, 150 μl of sodium phosphate buffer (0.1 M, pH 8.0), 10 μl test compound solution and 20 μl of enzyme solution (0.09 units/ml) were mixed and incubated for 15 min at room temperature. Then, 10 μl of DTNB (10 mM) was transferred and the reaction was initiated by the addition of substrate solution (10 μl of ATCI, 14 mM solution). The hydrolysis of the ATCI was measured by the formation of the product, 5-thio-2-nitrobenzoate, which is released by AChE hydrolysis. Absorbance of final solution was spectrophotometrically measured at 412 nm (Beckhman Coulter DU 730) after 10-min incubation. Tacrine, a standard AChE inhibitor, was used as a positive control. The percent of AChE inhibition was calculated as follows:
where AS is the absorbance of the sample containing taxifolin and AC is the absorbance of the control sample.
Results and discussion
Carbonic anhydrases catalysis the crucial pathophysiological processes that are connected with CO2/ transport and homeostasis, biosynthetic reactions including gluconeogenesis, ureagenesis and lipogenesis, respiration, calcification, tumorigenicity, electrolyte secretion in a variety of tissues and organs, and bone resorptionCitation94. Phenolic compounds are a class of chemicals containing of a –OH bonded directly to an aromatic hydrocarbon group and are categorized either as simple phenols or as polyphenols depending on the number of phenol units in the moleculeCitation24. Recently, a lot of phenolic acid, phenols and phenolic derivatives were investigated in detail as inhibitors of the Zn2+-containing CA isoenzymes by our groupCitation95–99. It was reported all CA isoforms are inhibited by three different mechanisms: (i) inhibition by coordination of the inhibitor to the Zn2+ located in the active site of CA isoenzymes, thereby replacing the Zn2+-bound H2O/–OH, which leads to a tetrahedral geometry for Zn2+. This geometry can also arise by the addition of an inhibitor to the metal coordination sphere when the Zn2+ has trigonal bipyramidal geometryCitation1. (ii) Inhibition by anchoring of the inhibitor to the Zn2+-bound solvent molecule, i.e. an H2O/–OH. Phenolic compounds and polyamine molecules can bind to CA in this way, as shown schematically for phenol or (iii) inhibition by inhibitor occlusion of the active site or activator-binding site of CACitation100–102. Thus, by binding in a non-classical way to CAs, phenols and their derivatives provide interesting leads for identifying novel types of CAIs. Taxifolin has two classical structural characteristics in one molecule: phenols and ketone.
It has been reported that phenols act as an inhibitors of the Zn2+-containing CA isoenzymesCitation103. Phenol binds to CA in a diverse manner when compared to the classic sulfonamide inhibitors. Sulfonamides coordinate to the Zn2+ ion in the CA active site by replacing the fourth non-protein ligand, which is typically an H2O molecule or a –OH ion. By binding in a non-classical way to CAs, phenols and their derivatives constitute interesting leads for identifying novel types of CAIsCitation94,Citation100,Citation102. In the present study, we report the inhibition profiles of taxifolin against the slower cytosolic isoform, hCA I and the more rapid isoenzyme hCA II. Taxifolin showed effective inhibition of both isoforms ().
Table 1. The inhibition profiles of taxifolin on purified hCA I and II from human erythrocytes by sepharose-4B-l-tyrosine-sulfanilamide affinity chromatography and inhibition of AChE purified from electric gel (Electrophorus electricus).
To describe inhibitory effects, researchers often list an IC50 value; however, a more suitable measure is the Ki constant. Ki values were calculated from Lineweaver–Burk graphs (, and ), and both the Ki and IC50 parameters of the taxifolin were determined in this study. The Lineweaver–Burk plot is a graphical representation of the Lineweaver–Burk equation of enzyme kineticsCitation104. The plot provides a useful graphical method for analysis of the Michaelis–Menten equation:
Figure 1. Determination of the half maximal inhibitory concentration (IC50) (A) and inhibition constant (Ki) values (B) of taxifolin for human erythrocyte carbonic anhydrase I isoenzyme (hCA I) by using a Lineweaver–Burk graph.
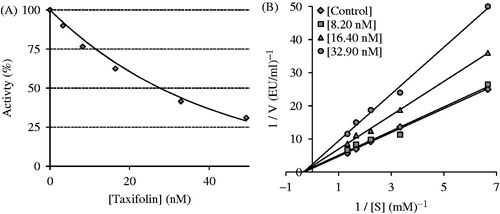
Figure 2. Determination of the half maximal inhibitory concentration (IC50) (A) and inhibition constant (Ki) values (B) of taxifolin for human erythrocyte carbonic anhydrase II isoenzyme (hCA II) by using a Lineweaver–Burk graph.
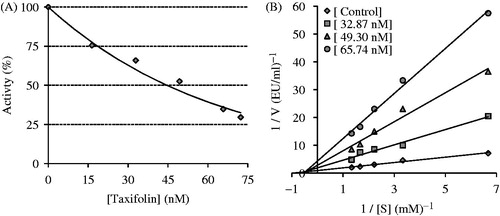
Taking the reciprocal gives
where V is the reaction velocity, Km is the Michaelis–Menten constant, Vmax is the maximum reaction velocity and [S] is the substrate concentration.
As shown in and , the IC50 and Ki values were found for taxifolin against both CA isoenzymes. For the cytosolic isoenzyme hCA I, taxifolin had an IC50 value of 27.72 nM and a Ki value of 29.20 ± 2.85 nM (). On the other hand, AZA is a hCA I that is used for the medical treatment of glaucoma, altitude sickness, epileptic seizure, idiopathic intracranial hypertension, central sleep apnoea, cystinuria, periodic paralysis and dural ectasia. AZA was extensively used as positive control for both CA isoenzymes. In the present study, AZA demonstrated IC50 values of 315.52 and 123.53 nM against hCA I and II, respectively. On the other hand, its Ki values for both isoenzymes were found as 184.34 and 61.12 nM, respectively. These results clearly show that taxifolin had more CA isoenzyme inhibitor effects than that of AZA.
As seen in , for the physiologically predominant CA II, taxifolin had an IC50 value of 43.31 nM and a Ki value of 24.15 ± 6.45 nM. Many studies have shown that the inhibition of CA II is brought about by an inhibitor’s ability to bind to the catalytic Zn2+ in the CA active site and mimic the tetrahedral transition stateCitation47,Citation49,Citation50.
There are important differences in inhibition between the two isoenzymes. The main difference is found in the active site architectures of the two hCA isoenzymes and is due to the presence of more histidine residues in the CA I isoformCitation47. In addition to the Zn2+ binding ligands (His 94, His 96 and His 119) discussed in the introduction, the His 64 residue of CA I play an important role in catalysis. Another difference between the two isozymes is that CA II contains a histidine cluster consisting of the following residues: His 64, His 4, His 3, His 10, His 15 and His 17 which are absent in CA I. Hence, these two isozymes exhibit different affinities for the inhibitors. In general, CA II has a higher affinity for the inhibitor than CA ICitation47.
hCA I is highly abundant in red blood cells and is found in many tissues but its precise physiological function is unknown. CA I is associated with cerebral and retinal edema; thus, the inhibition of CA I may be a valuable tool for fighting these conditions. The physiologically predominant cytosolic isoform hCA II is ubiquitous, and it is associated with several diseases including epilepsy, edema, glaucoma and altitude sicknessCitation56,Citation57,Citation105.
AZA is a well-known example of a clinically established CAICitation106,Citation107 and in recent years we have reported its strong inhibition of both human cytosolic CA I and II. CAI effects are also exhibited by a wide spectrum of phenolic compounds including melatoninCitation40, morphineCitation41, vitamin ECitation74, CAPECitation84, antioxidant phenolsCitation100, phenolic acidsCitation98, natural product polyphenols and phenolic acidsCitation96, natural phenolic compoundsCitation51,Citation92,Citation95, antioxidant polyphenol productsCitation92,Citation95 (3,4-dihydroxyphenyl)(2,3,4-trihydroxyphenyl)methanone and its derivativesCitation52, natural and synthetic bromophenolsCitation57,Citation91,Citation108, novel sulfonamide derivatives of aminoindanes and anilinesCitation44, novel phenolic sulfamidesCitation108, novel phenolic benzylamine derivativesCitation45, sulfonamide derivativesCitation60 and novel sulfamide analogues of dopamine-related compoundsCitation38, new benzotropone derivativesCitation50, guaiacol and catechol derivativesCitation55, capsaicinCitation109, hydroquinoneCitation110 and brominated diphenylmethanone and its derivativesCitation46, novel sulfamides and sulfonamides incorporating a tetralin scaffoldCitation39. These extensive studies indicate the importance of CA I and II isoenzyme inhibitors.
AChE was very strongly inhibited by taxifolin (). Taxifolin had an IC50 value of 30.1 nM and a Ki value of 16.7 ± 3.9 nM (). On the other hand, donepezil hydrochloride, which is used to the treatment of mild-to-moderate AD and various other memory impairments, had been shown to lower AChE inhibition activity (IC50: 55 nM)Citation106. Donepezil hydrochloride contains N-benzylpiperidine and an indanone moiety that shows longer and more selective action.
Conclusions
Taxifolin demonstrated unique inhibition profiles against both CA isoforms I and II. These results demonstrated that in the light of the high homology between these two CAs, they exhibit similar activity. Taxifolin was first identified as a potent CAI because phenolic compounds with aromatic rings have previously been identified as inhibitors of CA. In this study, nanomolar levels of Ki and IC50 values were observed for taxifolin. We show that taxifolin is a selective inhibitor for both cytosolic CA isoenzymes. These results clearly indicate the potential for use of bioactive taxifolin to identifying more CAIs and for eventually targeting additional isoforms. Additionally, taxifolin had effective AChE inhibition properties and it can be a good candidate for the treatment of mild-to-moderate AD and various other memory impairments.
Declaration of interest
The authors declare no conflict of interest. I.G. and S.H.A. would like to extend his sincere appreciation to the Deanship of Scientific Research at King Saud University for its funding of this research through the Research Group Project No. RGP-VPP-254.
References
- Rice-Evans CA, Miller NJ, Paganga G. Structure antioxitant activity relationships of flavanoids and phenotic acids. Free Radical Biol Med 1996;20;933–56
- Gülçin İ. Antioxidant activity of food constituents – an overview. Arch Toxicol 2012;86:345–91
- Makarova K, Lastawska K, Wagner D, Wawer I. ESR study of spin trapping in Fenton media in the presence of taxifolin. J Mol Struct 2014;1067:27–36
- Gülçin İ, Daştan A. Synthesis of dimeric phenol derivatives and determination of in vitro antioxidant and radical scavenging activities. J Enzyme Inhib Med Chem 2007;22:685–95
- Ak T, Gülçin İ. Antioxidant and radical scavenging properties of curcumin. Chem Biol Interact 2008;174:27–37
- Gülçin İ, Büyükokuroğlu ME, Oktay M, Küfrevioğlu Öİ. On the in vitro antioxidant properties of melatonin. J Pineal Res 2002;33:167–71
- Gülçin İ, Büyükokuroğlu ME, Küfrevioğlu Öİ. Metal chelating and hydrogen peroxide scavenging effects of melatonin. J Pineal Res 2003;34:278–81
- Gülçin I, Beydemir S, Buyukokuroglu ME. In vitro and in vivo effects of dantrolene on carbonic anhydrase enzyme activities. Biol Pharm Bull 2004;27:613–16
- Gülçin İ, Beydemir Ş, Alici HA, et al. In vitro antioxidant properties of morphine. Pharmacol Res 2004;49:59–66
- Gülçin İ, Berashvili D, Gepdiremen A. Antiradical and antioxidant activity of total anthocyanins from Perilla pankinensis decne. J Ethnopharmacol 2005;101:287–93
- Gülçin İ, Elias R, Gepdiremen A, Boyer L. Antioxidant activity of lignans from fringe tree (Chionanthus virginicus L.). Eur Food Res Technol 2006;223:759–67
- Göçer H, Gülçin İ. Caffeic acid phenethyl ester (CAPE): correlation of structure and antioxidant properties. Int J Food Sci Nutr 2011;62:821–5
- Gülçin İ, Beydemir Ş, Çoban TA, Ekinci D. The inhibitory effect of dantrolene sodium and propofol on 6-phosphogluconate dehydrogenase from rat erythrocyte. Fresen Environ Bull 2008;17:1283–7
- Gülçin İ, Elias R, Gepdiremen A, et al. Antioxidant secoiridoids from fringe tree (Chionanthus virginicus L.). Wood Sci Technol 2009;43:195–212
- Şişecioğlu M, Çankaya M, Gülçin İ, Özdemir M. The Inhibitory effect of propofol on lactoperoxidase. Protein Peptide Lett 2009;16:46–9
- Gülçin İ. Antioxidant activity of caffeic acid (3,4-dihydroxycinnamic acid). Toxicology 2006;217:213–20
- Gülçin İ. Antioxidant and antiradical activities of L-carnitine. Life Sci 2006;78:803–11
- Şişecioğlu M, Çankaya M, Gülçin İ, Özdemir M. Interactions of melatonin and serotonin to lactoperoxidase enzyme. J Enzyme Inhib Med Chem 2010;25:779–83
- Gülçin İ. Comparison of in vitro antioxidant and antiradical activities of L-tyrosine and L-Dopa. Amino Acids 2007;32:431–8
- Gülçin İ. Measurement of antioxidant ability of melatonin and serotonin by the DMPD and CUPRAC methods as trolox equivalent. J Enzyme Inhib Med Chem 2008;23:871–6
- Şişecioğlu M, Gülçin İ, Çankaya M, Özdemir H. The inhibitory effects of L-adrenaline on lactoperoxidase enzyme (LPO) purified from buffalo milk. Int J Food Propert 2012;15:1182–9
- Gülçin İ. Antioxidant activity of L-adrenaline: an activity-structure insight. Chem Biol Interact 2009;179:71–80
- Gülçin İ. Antioxidant properties of resveratrol: a structure-activity insight. Innov Food Sci Emerg 2010;11:210–18
- Gülçin İ. Antioxidant activity of eugenol – a structure and activity relationship study. J Med Food 2011;14:975–85
- Kim NC, Graf TN, Sparacino CM, et al. Complete isolation and characterization of silybins and isosilybins from milk thistle (Silybum marianum). Org Biomol Chem 2003;1:1684–9
- Weidmann AE. Dihydroquercetin: more than just an impurity? Eur J Pharmacol 2012;684:19–26
- Vladimirov YA, Proskurnina EV, Demin EM, et al. Dihydroquercetin (taxifolin) and other flavonoids as inhibitors of free radical formation at key stages of apoptosis. Biochemistry (Mosc) 2009;74:301–7
- Wang YH, Wang WY, Chang CC, et al. Taxifolin ameliorates cerebral ischemia-reperfusion injury in rats through its anti-oxidative effect and modulation of NF-kappa B activation. J Biomed Sci 2006;13:127–41
- Haraguchi H, Ohmi I, Fukuda A, et al. Inhibition of aldose reductase and sorbitol accumulation by astilbin and taxifolin dihydroflavonols in Engelhardtia chrysolepis. Biosci Biotechnol Biochem 1997;61:651–4
- Sun X, Chen RC, Yang ZH, et al. Taxifolin prevents diabetic cardiomyopathy in vivo and in vitro by inhibition of oxidative stress and cell apoptosis. Food Chem Toxicol 2014;63:221–32
- Arutyunyan TV, Korystova AF, Kublik LN, et al. Effects of taxifolin on the activity of angiotensin-converting enzyme and reactive oxygen and nitrogen species in the aorta of aging rats and rats treated with the nitric oxide synthase inhibitor and dexamethasone. Age 2013;35:2089–97
- Middleton E Jr, Kandaswami C, Theoharides TC. The effects of plant flavonoids on mammalian cells: implications for inflammation, heart disease, and cancer. Pharmacol Rev 2000;52:673–51
- Cermak R, Wolffram S. The potential of flavonoids to influence drug metabolism and pharmacokinetics by local gastrointestinal mechanisms. Curr Drug Metab 2006;7:729–44
- Egert S, Rimbach G. Which sources of flavonoids: complex diets or dietary supplements? Adv Nutr 2011;2:8–14
- Boušová I, Hájek J, Dršata J, Skálová L. Naturally occurring flavonoids as inhibitors of purified cytosolic glutathione S-transferase. Xenobiotica 2012;42:872–9
- Supuran CT, Scozzafava A. Carbonic anhydrases as targets for medicinal chemistry. Bioorg Med Chem 2007;15:4336–50
- Zolnowska B, Slawinskia J, Pogorzelska A, et al. Carbonic anhydrase inhibitors. Synthesis, and molecular structure of novel series N-substituted N′-(2-arylmethylthio-4-chloro-5-methyl benzenesulfonyl) guanidines and their inhibition of human cytosolic isozymes I and II and the transmembrane tumor-associated isozymes IX and XII. Eur J Med Chem 2014;71:135–47
- Aksu K, Nar M, Tanc M, et al. The synthesis of sulfamide analogues of dopamine related compounds and their carbonic anhydrase inhibitory properties. Bioorg Med Chem 2013;21:2925–31
- Akincioglu A, Topal M, Gülçin I, Goksu S. Novel sulfamides and sulfonamides incorporating tetralin scaffold as carbonic anhydrase and acetylcholine esterase inhibitors. Arch Pharm 2014;347:68–76
- Beydemir S, Gülçin I. Effect of melatonin on carbonic anhydrase from human erythrocyte in vitro and from rat erythrocyte in vivo. J Enzyme Inhib Med Chem 2004;19:193–7
- Coban TA, Beydemir S, Gülçin I, Ekinci D. Morphine inhibits erythrocyte carbonic anhydrase in vitro and in vivo. Biol Pharm Bull 2007;30:2257–61
- Supuran CT. Carbonic anhydrases: novel therapeutic applications for inhibitors and activators. Nat Rev Drug Discov 2008;7:168–81
- Akbaba Y, Akincioglu A, Gocer H, et al. Carbonic anhydrase inhibitory properties of novel sulfonamide derivatives of aminoindanes and aminotetralins. J Enzyme Inhib Med Chem 2014;29:35–42
- Akbaba Y, Bastem E, Topal F, et al. Synthesis and carbonic anhydrase inhibitory effects of novel sulfamides derived from 1-aminoindanes and anilines. Arch Pharm 2014;347:950–7
- Cetinkaya Y, Gocer H, Goksu S, Gülçin I. Synthesis and carbonic anhydrase isoenzymes inhibitory effects of novel benzylamine derivatives. J Enzyme Inhib Med Chem 2014;29:168–74
- Çetinkaya Y, Göçer H, Gülçin İ, Menzek A. Synthesis and carbonic anhydrase isoenzymes inhibitory effects of brominated diphenylmethanone and its derivatives. Arch Pharm 2014;347:354–9
- Supuran CT, Scozzafava A. Carbonic anhydrase inhibitors and their therapeutic potential. Expert Opin Ther Pat 2000;10:575–600
- Mincione F, Scozzafava A, Supuran CT. In drug design of zinc-enzyme inhibitors: functional, structural, and disease applications. Hoboken: Wiley; 2009:139
- Supuran CT. Carbonic anhydrase inhibitors. Bioorg Med Chem Lett 2010;20:3467–74
- Güney M, Coskun A, Topal F, et al. Oxidation of cyanobenzocycloheptatrienes: synthesis, photooxygenation reaction and carbonic anhydrase isoenzymes inhibition properties of some new benzotropone derivatives. Bioorg Med Chem 2014;22:3537–43
- Gülçin I, Beydemir Ş. Phenolic compounds as antioxidants: carbonic anhydrase isoenzymes inhibitors. Mini Rev Med Chem 2013;13:408–30
- Nar M, Cetinkaya Y, Gulcin I, Menzek A. (3,4-Dihydroxyphenyl)(2,3,4-trihydroxyphenyl)methanone and its derivatives as carbonic anhydrase isoenzymes inhibitors. J Enzyme Inhib Med Chem 2013;28:402–6
- De Simone G, Di Fiore A, Supuran CT. Are carbonic anhydrase inhibitors suitable for obtaining antiobesity drugs? Curr Pharm Des 2008;14:655–60
- Göksu S, Naderi A, Akbaba Y, et al. Carbonic anhydrase inhibitory properties of novel benzylsulfamides using molecular modeling and experimental studies. Bioorg Chem 2014;56:75–82
- Scozzafava A, Passaponti M, Supuran CT, Gülçin İ, Carbonic anhydrase inhibitors: guaiacol and catechol derivatives effectively inhibit certain human carbonic anhydrase isoenzymes (hCA I, II, IX, and XII). J Enzyme Inhib Med Chem 2015; DOI:10.3109/14756366.2014.956310
- Sethi KK, Verma SM, Tanc M, et al. Carbonic anhydrase inhibitors: synthesis and inhibition of the human carbonic anhydrase isoforms I, II, IX and XII with benzene sulfonamides incorporating 4- and 3-nitrophthalimide moieties. Bioorg Med Chem 2014;22:1586–95
- Boztaş M, Çetinkaya Y, Topal M, et al. Synthesis and carbonic anhydrase isoenzymes I, II, IX, and XII inhibitory effects of dimethoxy-bromophenol derivatives incorporating cyclopropane moieties. J Med Chem 2015;58:640–50
- Franchi M, Vullo D, Gallori E, et al. Carbonic anhydrase inhibitors: inhibition of human and murine mitochondrial isozymes V with anions. Bioorg Med Chem Lett 2003;13:2857–61
- Taslimi P, Gulcin İ, Ozgeris B, et al. The human carbonic anhydrase isoenzymes I and II (hCA I and II) inhibition effects of trimethoxyindane derivatives. J Enzyme Inhib Med Chem 2015; DOI:10.3109/14756366.2015.1014476
- Göçer H, Akıncıoğlu A, Öztaşkın N, et al. Synthesis, antioxidant and antiacetylcholinesterase activities of sulfonamide derivatives of dopamine related compounds. Arch Pharm 2013;346:783–92
- Gocer H, Akıncıoğlu A, Goksu S, et al. Carbonic anhydrase and acetylcholine esterase inhibitory effects of carbamates and sulfamoylcarbamates. J Enzyme Inhib Med Chem 2015;30:316–20
- Fulton MH, Key PB. Acetylcholinesterase inhibition in estuarine fish and invertebrates as an indicator of organophosphorus insecticide exposure and effects. Environ Toxicol Chem 2001;20:37–45
- Allam AR, Sridhar GR, Das UN. Elevated butrylcholinesterase and acetylcholinesterase may predict the development of type 2 diabetes mellitus and Alzheimer’s disease. Med Hypotheses 2007;69:1272–6
- Greenblatt HM, Dvir H, Silman I, Sussman JL. Acetylcholinesterase: a multifaceted target for structure-based drug design of anticholinesterase agents for the treatment of Alzheimer’s disease. J Mol Neurosci 2003;20:369–83
- Costa P, Gonccalves S, Valentao P, et al. Accumulation of phenolic compounds in in vitro cultures and wild plants of Lavandula viridis L′Hér and their antioxidant and anti-cholinesterase potential. Food Chem Toxicol 2013;57:69–74
- Hobbiger F. The inhibition of acetylcholinesterase by organophosphorus compounds and its reversal. Proc R Soc Med 1961;54:403–5
- Massoulie J, Pezzementi L, Bon S, et al. Molecular and cellular biology of cholinesterases. Prog Neurobiol 1993;41:31–91
- Ryu HW, Curtis-Long MJ, Jung S, et al. Anticholinesterase potential of flavonols from paper mulberry (Broussonetia papyrifera) and their kinetic studies. Food Chem 2012;132:1244–50
- Szwajgier D. Anticholinesterase activities of selected polyphenols – a short report. Pol J Food Nutr Sci 2014;64:59–64
- Krikorian R, Shidler MD, Nash TA, et al. Blueberry supplementation improves memory in older adults. J Agric Food Chem 2010;58:3996–4000
- Gutierres JM, Carvalho FB, Schetinger MR, et al. Anthocyanins restore behavioral and biochemical changes caused by streptozotocin-induced sporadic dementia of Alzheimer’s type. Life Sci 2013;96:7–17
- Pervin M, Hasnat MdA, Lee YM, et al. Antioxidant activity and acetylcholinesterase inhibition of grape skin anthocyanin (GSA). Molecules 2014;19:9403–18
- Zhang DB, Chen JJ, Song QY, et al. Lycodine-type alkaloids from Lycopodiastrum casuarinoides and their acetylcholinesterase inhibitory activity. Molecules 2014;19:9999–10010
- Aras Hisar S, Hisar O, Beydemir S, et al. Effect of vitamin E on carbonic anhydrase enzyme activity in rainbow trout (Oncorhynchus mykiss) erythrocytes in vitro and in vivo. Acta Vet Hung 2004;52:413–22
- Coban TA, Beydemir S, Gülçin I, Ekinci D. The inhibitory effect of ethanol on carbonic anhydrase isoenzymes: in vivo and in vitro studies. J Enzyme Inhib Med Chem 2008;23:266–70
- Senturk M, Gülçin I, Beydemir S, et al. In vitro inhibition of human carbonic anhydrase I and II isozymes with natural phenolic compounds. Chem Biol Drug Des 2011;77:494–9
- Atasaver A, Özdemir H, Gülçin İ, Küfrevioğlu Öİ. One-step purification of lactoperoxidase from bovine milk by affinity chromatography. Food Chem 2013;136:864–70
- Laemmli DK. Cleavage of structural proteins during the assembly of the head of bacteriophage T4. Nature 1970;227:680–3
- Gülçin I, Kufrevioglu OI, Oktay M. Purification and characterization of polyphenol oxidase from nettle (Urtica dioica L.) and inhibition effects of some chemicals on the enzyme activity. J Enzyme Inhib Med Chem 2005;20:297–302
- Beydemir S, Gülçin I, Hisar O, et al. Effect of melatonin on glucose-6-phospate dehydrogenase from rainbow trout (Oncorhynchus mykiss) erythrocytes in vitro and in vivo. J Appl Anim Res 2005;28:65–8
- Hisar O, Beydemir S, Gülçin I, et al. The effect of melatonin hormone on carbonic anhydrase enzyme activity in rainbow trout (Oncorhynchus mykiss) erythrocytes in vitro and in vivo. Turk J Vet Anim Sci 2005;29:841–5
- Verpoorte JA, Mehta S, Edsall JT. Esterase activities of human carbonic anhydrases B and C. J Biol Chem 1967;242:4221–9
- Coban TA, Beydemir S, Gülçin I, et al. Sildenafil is a strong activator of mammalian carbonic anhydrase isoforms I–XIV. Bioorg Med Chem 2009;17:5791–5
- Gocer H, Gülçin I. Caffeic acid phenethyl ester (CAPE): a potent carbonic anhydrase isoenzymes inhibitor. Int J Acad Res 2013;5:150–5
- Bradford MM. A rapid and sensitive method for the quantitation of microgram quantities of protein utilizing the principle of protein-dye binding. Anal Biochem 1976;72:248–51
- Koksal E, Gülçin İ. Purification and characterization of peroxidase from cauliflower (Brassica oleracea L.) buds. Protein Peptide Lett 2008;15:320–6
- Senturk M, Gülçin I, Ciftci M, Kufrevioglu OI. Dantrolene inhibits human erythrocyte glutathione reductase. Biol Pharm Bull 2008;31:2036–9
- Lineweaver H, Burk D. The determination of enzyme dissociation constants. J Am Chem Soc 1934;56:658–66
- Yıldırım A, Atmaca U, Keskin A, et al. N-Acylsulfonamides strongly inhibit human carbonic anhydrase isoenzymes I and II. Bioorg Med Chem 2015; DOI:10.1016/j.bmc.2014.12.054
- Cetinkaya Y, Gocer H, Menzek A, Gülçin İ. Synthesis and antioxidant properties of (3,4-dihydroxyphenyl)(2,3,4-trihydroxyphenyl)methanone and its derivatives. Arch Pharm 2012;345:323–34
- Akbaba Y, Balaydın HT, Menzek A, et al. Synthesis and biological evaluation of novel bromophenol derivatives as carbonic anhydrase inhibitors. Arch Pharm 2013;346:447–54
- Ozturk Sarikaya SB, Topal F, Senturk M, et al. In vitro inhibition of α-carbonic anhydrase isozymes by some phenolic compounds. Bioorg Med Chem Lett 2011;21:4259–62
- Ellman GL, Courtney KD, Andres V, Featherston RM. A new and rapid colorimetric determination of acetylcholinesterase activity. Biochem Pharmacol 1961;7:88–95
- Topal M, Gülçin İ. Rosmarinic acid: a potent carbonic anhydrase isoenzymes inhibitor. Turk J Chem 2014;38:894–902
- Innocenti A, Gülçin İ, Scozzafava A, Supuran CT. Carbonic anhydrase inhibitors. Antioxidant polyphenol natural products effectively inhibit mammalian isoforms I-XV. Bioorg Med Chem Lett 2010;20:5050–3
- Innocenti A, Ozturk Sarıkaya SB, Gülçin I, Supuran CT. Carbonic anhydrase inhibitors. Inhibition of mammalian isoforms I-XIV with a series of natural product polyphenols and phenolic acids. Bioorg Med Chem 2010;18:2159–64
- Innocenti A, Vullo D, Scozzafava A, Supuran CT. Carbonic anhydrase inhibitors. Interactions of phenols with the 12 catalytically active mammalian isoforms (CA I-XIV). Bioorg Med Chem Lett 2008;18:1583–7
- Ozturk Sarikaya SB, Gülçin I, Supuran CT. Carbonic anhydrase inhibitors. Inhibition of human erythrocyte isozymes I and II with a series of phenolic acids. Chem Biol Drug Des 2010;75:515–20
- Khoddami A, Wilkes MA, Roberts TH. Techniques for analysis of plant phenolic compounds. Molecules 2013;18:2328–75
- Nair SK, Ludwig PA, Christianson DW. Phenol as a carbonic anhydrase inhibitor. J Am Chem Soc 1994;116:3659–60
- Innocenti A, Vullo D, Scozzafava A, Supuran CT. Carbonic anhydrase inhibitors: inhibition of mammalian isoforms I–XIV with a series of substituted phenols including paracetamol and salicylic acid. Bioorg Med Chem 2008;16:7424–8
- Davis RA, Innocenti A, Poulsen SA, Supuran CT. Carbonic anhydrase inhibitors. Identification of selective inhibitors of the human mitochondrial isozymes VA and VB over the cytosolic isozymes I and II from a natural product-based phenolic library. Bioorg Med Chem 2010;18:14–18
- Hisar O, Beydemir S, Gülçin I, et al. Effect of low molecular weight plasma inhibitors of rainbow trout (Oncorhyncytes mykiss) on human erythrocytes carbonic anhydrase-II isozyme activity in vitro and rat erythrocytes in vivo. J Enzyme Inhib Med Chem 2005;20:35–9
- Akbaba Y, Türkeş C, Polat L, et al. Synthesis and paroxonase activities of novel bromophenols. J Enzyme Inhib Med Chem 2013;28:1073–9
- Akincioglu A, Akbaba Y, Gocer H, et al. Novel sulfamides as potential carbonic anhydrase isoenzymes inhibitors. Bioorg Med Chem 2013;21:1379–85
- Ogilvie JM, Ohlemiller KK, Shah GN, et al. Carbonic anhydrase XIV deficiency produces a functional defect in the retinal light response. Proc Natl Acad Sci USA 2007;104:8514–19
- Avvaru BS, Busby SA, Chalmers MJ, et al. Apo-human carbonic anhydrase II revisited: implications of the loss of a metal in protein structure, stability, and solvent network. Biochemistry 2009;48:7365–72
- Balaydin HT, Gülçin I, Menzek A, et al. Synthesis and antioxidant properties of diphenylmethane derivative bromophenols including a natural product. J Enzyme Inhib Med Chem 2010;25:685–95
- Arabaci B, Gülçin I, Alwasel S. Capsaicin: a potent inhibitor of carbonic anhydrase isoenzymes. Molecules 2014;19:10103–14
- Scozzafava A, Kalın P, Supuran CT, et al. The impact of hydroquinone on acetylcholine esterase and certain human carbonic anhydrase isoenzymes (hCA I, II, IX and XII). J Enzyme Inhib Med Chem 2015; DOI: 10.3109/14756366.2014.999236