Abstract
Phthalates, used in medical devices (MDs), have been identified as reproductive and developmental toxicants. Their toxicity varies somewhat depending on the specific phthalate and is in part linked to the activation of Peroxisome Proliferating-Activated Receptors (PPARs). So, the use of MDs containing targeted phthalates such as di(2-ethylhexyl) phthalate (DEHP) has been challenged by European directive 2007/47/EC. Therefore, MDs manufacturers were forced to quickly find replacement plasticizers. However, very little toxicological and epidemiological studies are available on human health. So, we proceeded to dock these chemicals in order to identify compounds that are likely to interact with PPARs binding sites. The results obtained are generally very mixed on the harmlessness of these alternatives. Moreover, no data exist on the biological effects of their possible metabolites. As DEHP toxicity resulted mainly from its major metabolites, generalizing the use of these plasticizers without conducting extensive studies on the possible effects on human health of their metabolites seems inconceivable.
Introduction
Phthalates are a class of several different chemicals that have various uses in consumer products such as medical devices (MDs), cosmetics and personal care products, food packaging, children’s toys and childcare articles made of polyvinyl chloride (PVC). These esters of phthalic acid are mainly used as plasticizers in PVC to increase its flexibility, elongation and processability. They have a different hazard classification regarding human health: the high-molecular weight phthalates which are the most widely used plasticizers and the low-molecular weight phthalates which have all been classified as toxic to reproductionCitation1. The most widely used phthalate is di(2-ethylhexyl) phthalate (DEHP). Its excellent performance in the plasticization and processing of PVC explains its wide use in MDs over the past few years. However, phthalates are not chemically bound to the PVC polymer and may leach when the MDs is heated or when the PVC comes into contact with blood, drugs or intravenous fluids.
Human exposure to phthalates occurs through inhalation and ingestion of contaminated air and food as well as from skin contact. Food may become contaminated when it comes in contact with packaging that contains phthalates. For the general population, this may be a major source of exposureCitation1,Citation2. Moreover, very high exposures to phthalates can occur via medical treatment, i.e. via use of MDs containing DEHPCitation3–5.
Children may be exposed to higher concentrations of phthalates from food consumption because they tend to consume more food than adults relative to their body weightCitation1,Citation2,Citation6. An additional exposure route for young children is through mouthing toys containing phthalates. Also, newborns and children in pediatric settings may receive the highest doses from blood transfusions, extracorporeal oxygenation and respiratory therapyCitation1,Citation7. However, it should not be overlooked that neonates and developing fetuses at critical points in their development may be exposed through maternal use of PVC products.
This exposure to phthalates in the general population raises health concerns. Many works have noted health effects such as reproductive abnormalities and developmental effects in animals given doses of phthalates similar to those to which humans are exposedCitation1. Epidemiologic studies have identified a possible association between exposure to phthalates and male reproductive malformation, sperm damage, fertility impairment, female reproductive tract diseases, early puberty in girls, asthma and thyroid effects as well as obesity and Type 2 diabetesCitation1,Citation8. The prevalence of obesity/overweight and Type 2 diabetes has risen dramatically in both wealthy and in poorer countries. The reasons for this sharp increase are not well understood. Many of these illnesses are being reported in children, whereas in the past these diseases were only seen in older individualsCitation8. Interestingly, one environmental factor that is recently receiving attention is the contribution of endocrine disrupting chemicals to the high prevalence of obesity. These compounds can interfere with the normal functions of the endocrine system by affecting the balanced system of glands and hormones that regulate vital body functions (growth, stress response, sex development, gender behavior, ability to reproduce, production and utilization of insulin, and metabolic rate)Citation8. Epidemiology studies confirm that exposure to these chemicals during development is associated with overweight and obesity later in lifeCitation8,Citation9. In fact, endocrine disrupting chemicals interfere with the body’s endocrine system and produce adverse developmental, reproductive, neurological, cardiovascular, metabolic and immune effects in humans. They are capable of mimicking natural hormones and maintain similar modes of action, transport and storage within tissues. The properties of these chemicals, while unintended, make them particularly well suited for activating or antagonizing nuclear hormone receptorsCitation10.
The hypothesis that certain environmental pollutants should interfere with various aspects of metabolism seems therefore well establishedCitation11–13.
Indeed, a wide range of substances, both natural and man-made, are thought to cause endocrine disruption: dioxin and dioxin-like compounds, polychlorinated biphenyls and other pesticides, components of plastics such as bisphenol A and phthalatesCitation10.
Phthalates, as endocrine disruptors have been identified as reproductive and developmental toxicants, though their toxicity varies somewhat depending on the specific phthalate structureCitation1. Because of health concerns, as of February 2009 the Consumer Product Safety Improvement Act restricted DEHP and other plasticizers such as benzyl butyl phthalate, and dibutyl phthalate in children’s toys and childcare articles in concentrations exceeding 0.1%Citation14.
The 2008 report by the Scientific Committee on Emerging and Newly-Identified Health Risks (SCENIHR) highlighted DEHP toxicity from several animal and some human studies and also suggested using less-toxic alternativesCitation15. Following these considerations, the use of MDs containing phthalates including DEHP has recently been challenged by European directive 2007/47/EC, effective since March 2010Citation16. Thus, when MDs contain phthalates which are classified as carcinogenic, mutagenic or toxic to reproduction, of category 1 or 2, they must be so-labeled.
Although a number of plasticizers are now restricted from children’s products in the US and European Union, they are unregulated and continue to be used in toys made in many other parts of the world. Accordingly, children continue to be exposed to phthalates by products in current useCitation1.
Toxicology approaches have demonstrated that phthalate plasticizers can directly influence peroxisome proliferator-activated receptors (PPARs) activityCitation8,Citation13,Citation17.
These receptors compose a class of nuclear receptors involved in glucidic and lipidic metabolism. They are divided in three isoforms (PPARα, PPARβ/δ and PPARγ), of which α and γ are of particular interest. The best-characterized functions of PPARs include the role of PPARα in hepatic and muscle fatty acid catabolism, and the opposite but complementary role of PPARγ in adipogenesis and lipid storage. Therefore, metabolic consequences of human phthalate exposure mainly result from the activation of both PPARα and PPARγ, the best-studied receptorsCitation13.
Because human at risk for reproductive toxicity of phthalates are likely to include those exposed occupationally as well as those exposed during medical treatments, an active research for an alternative plasticizer has been initiated. A number of substances have been identified as alternative plasticizers to DEHP such as mellitates, trioctyltrimellitate (TOTM); phthalates, di(2-ethylhexyl) terephthalate (DEHT) and diisononyl phthalate (DINP); adipates, di(2-ethylhexyl) adipate (DEHA); aliphatic esters, 1,2-cyclohexane dicarboxylic acid diiso-nonyl ester (DINCH) and citrates, acetyl tributyl citrate (ATBC) (). All these compounds have been registered by REACH (Registration, Evaluation, Authorisation and Restriction of Chemicals, the EU’s major regulation for chemicals which are used in many everyday products and articles), and authorized in products that traditionally use phthalates, such as toys, childcare articles and MDsCitation1,Citation18.
Figure 1. Chemical structures of di(2-ethylhexyl) phthalate (DEHP) and the selected alternative plasticizers investigated.
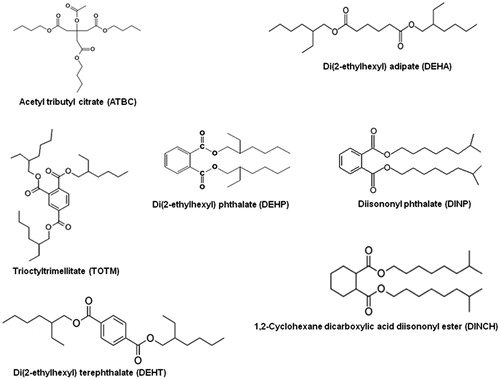
Although many of these alternatives show promising application potential, like DEHP, they are not chemically bound to the polymer and can leach out of products. Nowadays, the risk of exposure to these compounds for patients, particularly in situations classified “at risk”, has not yet been evaluated, because migrations studies, providing sufficient exposure and human toxicity data, have not been performed. Therefore, some investigations are needed such as the molecular interaction between PPARα and PPARγ binding sites. In this study, we proceeded to dock the replacement plasticizers, so-called “alternative to DEHP plasticizers” such as TOTM, DEHT, DEHA, DINP, DINCH and ATBC in order to compare with DEHP and specify the potential interactions of these ligands with PPARα and/or PPARγ.
Materials and methods
The studied compounds were built under the Sybyl 6.9.2 molecular modeling package running on Silicon Graphics Octane 2 workstations, from the standard fragment libraries. Their geometry was optimized until convergence to a 0.001 kcal/mol Å, using the Powell method, with the Tripos force field and partial atomic charges assigned following Gasteiger–Hückel. The dielectric constant was set to four to implicitly render a biological middle. To keep consistent with our previous study of phthalate derivatives, we docked the compounds in the same crystallographic data files of PPARα and γ ligand-binding domains with tesaglitazar (AZ 242), available in the RCSB Protein Data Bank (http://www.pdb.org)Citation19 (PDB ID: 1I7G and 1I7I, respectively)Citation20. The docking process was performed with GOLD suite 5.1Citation21, which behaves rather differently to the 3.0.1 version of our first study and employs refined scoring functions. About 30 conformations were generated for each compound, sorted by the ChemPLP function available in GOLD suite and visually assessed to check the existence of a recurring conformation. If several such clusters of solutions existed, and the larger represented less than half the solutions, the most representative conformation of each cluster was selected as the final docking result. On the contrary, if a cluster achieved at least 15 conformations, the results were inspected to find the most common conformation and evaluate the overall scattering of the whole set of solutions. We felt that this assessment was important as the molecules were thought to be rather poor binders, resulting in instable docking solutions with few well-defined clusters hinting toward a binding mode. However, it should be kept in mind that the crystallographic image of PPARs is a standstill shot of one particular conformation of a very plastic receptor family more able to adapt its conformation to its ligands than rigid body, large domain movement of the enzymes.
Results and discussion
First of all, we checked the consistency of the docking protocol against the previous results obtained for DEHP and TOTMCitation22. For the last, both protocols gave the same fuzzy placement in the binding site of both subtypes of PPAR. TOTM is barely able to be positioned in the pocket due to the length of its side chains, but not as single well-defined binding mode and without any specific polar anchoring. The new docking results were even fuzzier than the previous ones, emphasizing that TOTM was not able to bind to either receptor. DEHP showed a slightly larger cluster of conformations in PPARα, corresponding to a third of the solutions, with its benzene at the very entrance of the pocket (). A similar conformation was also found in the previous study, albeit with a lesser frequencyCitation22. Interestingly, DEHP also forms a number of hydrogen bonds, mostly with the skeletal NH of Ala 333 and the side chain of Thr 279. They were most surely lost due to a too drastic optimization of the geometry of the complex in the previous study, as they appeared very rarely. Here, these hydrogen bonds are constantly kept whereas other less-common interactions appeared with Tyr 334, further toward the outside of the pocket. In the other receptor subtype (PPARγ), a single conformation was found, although with a large dispersion around the center of the conformations cluster. This conformation had also been found in the previous study but was neither the single one nor the largest cluster. It is characterized by the placement of the benzene ring in the intersection of the Y-shaped pocket, away from the entrance, with the side chains occupying the outward funnel and the end of the pocket in front of Tyr 473. No hydrogen bond was formed, therefore showing a poor affinity and probably no activation of PPARγ. The overall solution for both ligands in both receptors appears to be in good agreement between the two docking runs. The differences between the protocols induce a more focused binding mode for the newer docking program version and revealed a set of hydrogen bonds between DEHP and PPARα that were not too infrequent to have been previously detected. We were therefore confident that the docking of the other compounds would give meaningful insights.
DEHT is the para isomer of DEHP. Due to its linear structure, we felt that it would fare rather badly on both PPAR subtype as it is unable to take the U conformation enabling more conventional ligands to bind to Tyr 464/473 and to occupy one of the other branches of the Y-shaped pocket. However, as DEHP, it should be able to fit in an upright position at the end of the binding site opposite to Tyr 473, quite reminiscent of 2-benzoylamino benzoic acid (PDB entry 1WM0)Citation23. It was clearly the case for PPARα, with no real cluster of conformations (). All the 30 solutions were placed in a similar position at the entrance of the binding site, accommodated in a vertical stance relative to the horizontal inner part of the pocket in front of the residues to which conventional PPAR ligands bind. About seven solutions had one of their side chains outside the receptor and about a third of the solutions formed a hydrogen bond with either the skeleton NH of Ala 333 or the side chain of Tyr 334. This unstable binding mode is most surely a hint of a low affinity. When docked in PPARγ, DEHT had a more closely knitted set of solutions forming a larger cluster encompassing two major placements of the benzene. One is deep in the pocket and very similar to that of DEHP whereas the other is closer to the entrance. But, contrary to our expectations, both binding modes depicted the occupation of the Tyr 473 channel and the entrance of the binding site by the two side chains of DEHT. It is noteworthy that the 11 conformations close to the entry were able to form a hydrogen bond with the skeletal NH of Ser 342, which is the analog of PPARα’s Ala 333. As a conclusion, DEHT seems to be no better PPARα activator than its congener, and behaves even a little bit worse in its interaction with PPARγ, but its binding is helped in slightly less than half of the solutions by a hydrogen bond that may contribute to its affinity.
The open analog of DEHP is DEHA. Due to its more flexible nature, all but three of the solutions were able to form at least one hydrogen bond with PPARα, most notably with Ala 333 (). However, no discernable cluster was found and the 30 solutions are not really in the pocket, but just at the entry, with at most a part of the side chains occupying the outer segment of the pocket. In PPARγ, on the contrary, DEHA is wholly embedded in the pocket, with a side chain in front of Tyr 473 and extending toward the entry funnel. However, there are only six conformations forming hydrogen bonds, all with Ser 342, and no cluster of a well-defined binding mode can be found. On both subtypes, no clear binding mode can be univocally observed due to a lack of stabilizing interactions, but there is a more robust linking pattern with PPARα than with PPARγ. This small size compound is most surely able to fit into the pocket, but it should display a higher affinity for PPARα than γ, although rather modest. The lack of a rigid aromatic core to anchor it by stronger aromatic interactions may explain its behavior and at least partly its probable lack of potency.
DINP bears different ester groups from DEHP, which are longer and have a larger steric requirement at the far end of the chain. This induces more diverse solutions than for DEHP in PPARα. Nonetheless, even if all 30 solutions are positioned in their own way, precluding a cluster of close conformations, a clear trend can be seen. The benzene occupies a position shifted toward the inside of the pocket, compared to DEHP () and all but one solution forms at least a hydrogen bond. Among the interactions observed, the most common involves Thr 279, but a few solutions are able to bind to Thr 279 and Ala 333. The side chains have no preferential orientation, occupying either of the branches of the pocket, and in 12 cases, pointing outside. A certain affinity may result from this rather strong binding. When docked in PPARγ, DINP behaves more like DEHP, with 13 conformations superimposing their aromatic group with that of DEHP. There is a larger dispersion of the other solutions than for DEHP, but again none forms hydrogen bonds with PPARγ. We can therefore suppose a very low affinity, contrary to what happens with PPARα, and most probably no noticeable activation of the receptor.
The non-aromatic variant of DINCH can adopt two configurations, produced by the respective position of the side chains relatively to the cycle. The cis configuration displays three major conformations in PPARα, two of which are rather marginal, with four or five solutions, and one having about a third of the conformations (). It is characterized by a conserved hydrogen bond with Ala 333 and the occupation of the part of the pocket in front of Tyr 464. Most of the solutions have their second side chain in the lower part of the other side of the pocket, while a couple point it outside. Its trans congener has a very similar behavior, but with a more fuzzy placement, giving a less closely fitting cluster of 12 solutions (). A finer view shows that in fact, two different clusters can be discerned, with the position of the cyclohexyl group being identical. About eight solutions exhibit one side chain toward Tyr 464 and the other pointing out of the pocket or downward into it as for the cis form, with a conserved hydrogen bond with Ala 333, quite often supplemented by another one with Thr 279 and lost only in one case. About four other solutions show one side chain oriented toward the outside, with the other directed toward Tyr 464 or downward, and loosing the Ala 333 hydrogen bond to a less-conserved Thr 279 or no hydrogen bond at all. Overall, this binding mode is fairly solid, but its rather low frequency does not convey an idea of stability in the interaction. In PPARγ, the cis isomer has two major, but fuzzy, conformations. The position of the molecule is globally unchanged, in particular the esters, but the cyclohexyl ring plane switches sides around the axis of the esters. Whatever the solution, there is no hydrogen bond (), hinting to a very low-predicted affinity, if any, for PPARγ. The trans isomer has a rather well-conserved position of its cycle, with the solutions differing in the placement of their side chains. While 27 solutions have one of them occupying the corridor in front of Tyr 473, the other chain has no preferential conformation and either points outward or occupies the upper part of the tail of the pocket, with all the possibilities in between (). Again, no hydrogen bond has been found and the affinity must be very poor. As a summary, neither the cis nor the trans DINCH has an apparent capacity to bind to any of the tested PPAR subtypes.
The structurally unrelated ATBC has not the shape of a classical PPAR ligand, and is therefore a bit trickier to assess. In PPARα, there is not as single conformation that looks like another, precluding the study of a well-defined cluster. Globally speaking, the central carbon is relatively stable in a narrow sphere of about an Ångström centered in the middle of the Thr 279–Ala 333–Tyr 334 triangle, with the side chains pointing in every direction with no distinct preference. A whole two-thirds of the solutions lie in this position, with the remaining third being placed a bit deeper in the pocket. All form between two and four hydrogen bonds with the usual Ala 333, Thr 279 and Tyr 334, but this pattern is not conserved, as is the placement of the compound, so we doubt it may be a serious proof of a good affinity. Moreover, the position of most of the solutions is rather at the edge of the binding site, with seven solutions only able to reach the Tyr 464 channel and occupying it only partially with one of their short chains (). When docked into PPARγ, ATBC is able to be positioned deeper in the pocket, in a position close to the seven solutions described just above for PPARα. Only five of the solutions have a hydrogen bond, with the skeletal NH of Ser 342. Due to its four-pronged structure, ATBC is able to occupy very fractionally all of the cavities of the binding site, with the three longer chains in the Y-shaped pocket and the methyl group pointing toward the outside. As the PPARs are highly flexible, this may induce a potential for activation, although this aspect is best studied by biological testing than by molecular modeling guessing. It is noteworthy that none of the conformations forming a hydrogen bond are able to occupy all the cavities, somewhat reducing the plausibility of activation of PPARγ as the activating conformation is not bound to the receptor and may well be an artifact of docking resulting from the small size of the compound compared to the pocket.
The highlights of the docking study of the various replacement plasticizers of DEHP can be correlated with the published data about their biological effects. Indeed, these replacement plasticizers have different concerns on environmental and human health perspective because they belong to several different chemical classes. Affinities with respect to PPAR receptors are different from one product to another and therefore different biological activities and especially toxicity may result.
Thereby, lesser toxicities are reported for TOTM, in particular a weaker hepatotoxicity than DEHP. It did not induce developmental effects in rats following gavage treatment during gestation. This is probably due to its inability to fit into the binding sites of the PPARs receptorsCitation22. However, a single-generation study found no effects on reproductive function, but did report decreased spermatocyte and spermatid counts in males treated by gavage at high doseCitation18.
DEHT, the para-isomer structure of DEHP undergoes a weak conversion to its primary metabolite, leading to a lower toxicity than DEHP. No reproductive effects based on fertility, gender ratios, liver litter size or postnatal survival were observed in animal studiesCitation18. Our results are in broad agreement with these observations since DEHT has a very weak affinity with respect to PPARα and so, seems to be no better PPARα activator than its congener DEHP.
Recent studies reported a possible peroxisome proliferation with DEHA that was similar to that of DEHP, which is a rodent-specific effect of questionable relevance to humansCitation24. A cancer bioassay in mice was positive, showing induction of liver tumors in both males and females. It has been hypothesized that the observed mouse liver tumors are a result of peroxisome proliferationCitation18. These effects with DEHA were already highlighted with DEHP whose probable mechanism was the activation of PPARα. In our study, DEHA has a slight affinity for PPARα with respect to DEHP because of the lack of the aromatic cycle. Therefore, the peroxisome proliferation effect of DEHA seems to be justified. In such cases, we should also consider the doses used. In addition, others studies reported that DEHA produced developmental/reproductive effects, including ovarian follicle atresia and prolonged estrus cycle in female rats, increased postnatal mortality in rat pups exposed perinatallyCitation18.
The toxicological aspect of DINP in rodents seems to be similar to that of DEHP. This has led to restrictions on its use in children’s articles that can be placed in the mouth. It now must not exceed 0.1% by mass of the plasticized materialCitation25. Our docking study shows that DINP has affinities for PPARα-like DEHP. On the whole, DINP cannot be claimed as valid alternative to DEHP since its toxicity profile would likely follow similar pathways and its use is forbidden in other PVC applications.
DINCH is the non-aromatic analog to DINP. It can adopt two configurations: cis and trans. In our docking study, neither the cis nor the trans DINCH has an apparent capacity to bind to any of the tested PPARs subtypes sites. This explains why its toxicological effects appear different from those of DEHP and DINP. Indeed, DINCH is neither a reproductive toxicant nor an endocrine disruptor. However, it was found to cause renal toxicity and thyroid hyperplasia in ratsCitation26, probably through other mechanisms not involving PPARs.
Despite its low toxicity compared to DEHP in animal models, in particular reduced growth, increased liver and possibly kidney weight, the plasticizer ATBC is a matter of concern because of its easier leachability from the PVCCitation18,Citation27. Moreover, it is suggested that ATBC-containing products should be used cautiously because they may alter metabolism of endogenous steroid hormones as well as administered drugsCitation28. However, the compound has not a capacity to bind to any of the tested PPARs subtypes. The toxicological effects, weak as they are, could pass through other mechanisms that need to be elucidated.
Major differences exist between these plasticizers in terms of physicochemical properties and toxicities. Information on disposition and metabolism can explain the great differences in the biological effects of these compounds. For example, DEHP toxicity has been clearly identified as resulting mainly from its major metabolite, the monoester MEHPCitation27. Except for DINP that follows the same rules as DEHP, all the other compounds require extensive metabolic study in order to identify and study their major metabolites. A better understanding of the human metabolism and excretion kinetics of these plasticizers is crucial for identifying metabolites whose harmlessness could be compromised. Indeed, the distribution of the replacement plasticizers is not homogeneous in MDs, as indicated by some studiesCitation15,Citation29. For this reason, in situations classified “at risk”, bio-monitoring studies will allow assessing the levels of these chemicals and/or metabolites in fluids like blood, urine or expired air in order to predict the potential risk of human exposure as well as health risk.
The possibility of using materials that do not require the use of plasticizers is undoubtedly an appealing approach to overcoming plasticized PVC concerns in medical applications. The polymer will need to be flexible, biocompatible and inert during the sterilization process. Therefore, polymers considered to be suitable alternatives to PVC include the following: ethylene vinyl acetate (EVA), silicones, polyurethanes and polyolefins; the most common types of polyolefins being polyethylene and polypropyleneCitation30. Although most of the selected materials perfectly matched the performance of PVC, their high cost is still a major limit to their exploitation. The safety profile of these polymers would also need to be tested properly since the toxicity data on humans, especially regarding their long-term effects, are lacking.
Conclusion
Phthalates are classified as endocrine-disrupting chemicals and have been linked to adverse health effects, particularly in relation to early life exposures. The most widely used phthalate is DEHP. The widespread population exposure to these products has raised substantial concern due to their potential detrimental health effects. Their toxicity varies somewhat depending on the specific phthalate structure and is in part linked to the activation of the PPARs. All the approaches converge to limit the harmful effects of these plasticizers either by improving their safety or by preventing their release into physiological fluids. As a response, MDs manufacturers were forced to quickly find replacement plasticizers, so-called “alternative to DEHP plasticizers”.
However, the safety profiles and leaching aptitude of these compounds are questions to be resolved, because the potential health effects in MDs have not been fully assessed and would be worthy of further investigation in the light of their applications. Our docking study highlights the need for a more comprehensive toxicological evaluation of the biological effects of these alternatives as well as their main metabolites.
Declaration of interest
The authors report no conflicts of interest. The authors alone are responsible for the content and writing of this article.
References
- Lowell Center for Sustainable Production. Phthalates and their Alternatives: Health and Environmental Concerns (2011). Available from: http://www.sustainableproduction.org/publ.alternatives.php [last accessed Sept 2014]
- Wargo J, Cullen MR, Taylor HS. Plastics that may be harmful to children and reproductive health. Environment & Human Health, Inc. (EHHI); 2008. Available from: www.ehhi.org/reports/plastics/ehhi_plastics_report_2008.pdf [last accessed Aug 2014]
- Heudorf U, Mersch-Sundermann V, Angerer J. Phthalates: toxicology and exposure. Int J Hyg Env Health 2007;210:623–34
- Bagel S, Decaudin B, Neuville S, et al. Phthalates in medical devices for parenteral and enteral nutrition. Nutr Clin Met 2011;25:15–23
- Kambia N, Dine T, Gressier B, et al. Strong variability of di(2-ethylhexyl)phthalate (DEHP) plasmatic rate in infants and children undergoing 12-hour cyclic parenteral nutrition. J Parenter Enteral Nutr 2013;37:229–35
- Mankidy R, Wiseman S, Ma H, Giesy JP. Biological impact of phthalates. Toxicol Lett 2013;217:50–8
- Scientific Committee on Emerging and Newly Identified Health Risks (SCENIHR). Preliminary Report on the Safety of Medical Devices Containing DEHP Plasticized PVC or Other Plasticizers on Neonates and Other Groups Possibly at Risk. European Commission Health and Consumer Protection Directorate-General 2007. Available from: http://ec.europa.eu/health/ph_risk/committees/04_scenihr/docs/scenihr_o_008.pdf [last accessed July 2014]
- Newbold RR. Impact of environmental endocrine disrupting chemicals on the development of obesity. Hormones 2010;9:206–17
- Goncharov A, Haase RF, Santiago-Rivera A, et al. High serum PCBs are associated with elevation of serum lipids and cardiovascular disease in a native American population. Environ Res 2008;106:226–39
- Schug TT, Janesick A, Blumberg B, Heindel JJ. Endocrine disrupting chemicals and disease susceptibility. J Steroid Biochem Mol Biol 2011;127:204–15
- Newbold RR, Padilla-Banks E, Jefferson WN, Heindel JJ. Effects of endocrine disruptors on obesity. Int J Androl 2008;31:201–8
- Tremblay A, Chaput JP. About unsuspected potential determinants of obesity. Appl Physiol Nutr Metab 2008;33:791–6
- Desvergne B, Feige JN, Casals-Casas C. PPAR-mediated activity of phthalates: a link to the obesity epidemic? Mol Cell Endocrinol 2009;304:43–8
- U.S. Environmental Protection Agency. Phthalates Action Plan (Revised 03/14/2012). Available from: http://www.epa.gov/oppt/existingchemicals/pubs/actionplans/phthalates_ actionplan_revised_2012-03-14.pdf [last accessed Jul 2014]
- Genay S, Luciani C, Décaudin B, et al. Experimental study on infusion devices containing polyvinyl chloride: to what extent are they di(2-ethylhexyl)phthalate-free? Int J Pharm 2011;412:47–51
- Directive, 2007/47/EC of the European parliament and of the Council of 5 September 2007 amending Council Directive 90/385/EEC on the approximation of the laws of the Member States relating to active implantable medical devices, Council Directive 93/42/EEC concerning medical devices and Directive 98/8/EC concerning the placing of biocidal products on the market (OJEU 21.09.2007)
- Barrett JR. To each his own: DEHP yields species-specific metabolic phenotypes. Environ Health Perspect 2010;118:A81
- Babich MA. Review of exposure and toxicity data for phthalate substitutes (2010). Available from: http://www.cpsc.gov/PageFiles/126546/phthalsub.pdf [last accessed Jul 2014]
- Berman HM, Westbrook J, Feng Z, et al. The protein data bank. Nucleic Acids Res 2000;28:235–42
- Cronet P, Petersen JFW, Folner R, et al. Structure of the PPARalpha and -gamma ligand binding domain in complex with AZ 242; ligand selectivity and agonist activation in the PPAR family. Structure 2001;9:699–706
- Jones G, Willett P, Glen RC, et al. Development and validation of a genetic algorithm for flexible docking. J Mol Biol 1997;267:727–48
- Kambia N, Renault N, Dilly S, et al. Molecular modelling of phthalates-PPARs interactions. J Enzyme Inhib Med Chem 2008;23:611–16
- Ostberg T, Svensson S, Selén G, et al. A new class of peroxisome proliferator-activated receptor agonists with a novel binding epitope shows antidiabetic effects. J Biol Chem 2004;279:41124–30
- Ito Y, Nakamura T, Yanagiba Y, et al. Plasticizers may activate human hepatic Peroxisome Proliferator-Activated Receptor a less than that of a mouse but may activate constitutive androstane receptor in liver. PPAR Res 2012;2012:201284
- European Union, Directive 2005/84/EC of the European Parliament and of the Council of 14 December 2005 amending for the 22nd time Council Directive 76/769/EEC on the approximation of the Laws, Regulations and Administrative Provisions of the Member States relating to restrictions on the marketing and use of certain dangerous substances and preparations (phthalates in toys and childcare articles), 2005
- Janssen PJCM, Bremmer HJ. Risk assessment non-phthalate plasticizers in toys. 2009. Available from: https://www.vwa.nl/txmpub/files/?p_file_id=2000547.pdf [last accessed Jul 2014]
- Bernard L, Décaudin B, Lecoeur M, et al. Analytical methods for the determination of DEHP plasticizer alternatives present in medical devices: a review. Talanta 2014;129:39–54
- Takeshita A, Igarashi-Migitaka J, Nishiyama K, et al. Acetyl tributyl citrate, the most widely used phthalate substitute plasticizer, induces cytochrome p450 3a through steroid and xenobiotic receptor. Toxicol Sci 2011;123:460–70
- Gimeno P, Thomas S, Bousquet C, et al. Identification and quantification of 14 phthalates and 5 non-phthalate plasticizers in PVC medical devices by GC-MS. J Chromatogr B Analyt Technol Biomed Life Sci 2014;949–950:99–108
- Chiellini F, Ferri M, Morelli A, et al. Perspectives on alternatives to phthalate plasticized poly(vinyl chloride) in medical devices applications. Prog Polym Sci 2013;38:1067–88