Abstract
(E)-3,4-dihydroxystyryl aralkyl sulfones and sulfoxides have been reported as novel multifunctional neuroprotective agents in previous studies, which as phenolic compounds display antioxidative and antineuroinflammatory properties. To further enhance the neuroprotective effects and study structure-activity relationship of the derivatives, we synthesized their acetylated derivatives, (E)-3,4-diacetoxystyryl sulfones and sulfoxides, and examined their neuroprotective effects in vitro models of Parkinson’s disease. The results indicate that (E)-3,4-diacetoxystyryl sulfones and sulfoxides can significantly inhibit kinds of neuron cell injury induced by toxicities, including 6-OHDA, NO, and H2O2. More important, they show higher antineuroinflammatory properties and similar antioxidative properties to corresponding un-acetylated compounds. Thus, we suggest that (E)-3,4-diacetoxystyryl sulfones and sulfoxides may have potential for the treatment of neurodegenerative disorders, especially Parkinson’s disease.
Introduction
Parkinson’s disease (PD) is a neurodegenerative disorder characterized by progressive loss of dopaminergic neurons in the substantia nigra pars compacta, resulting in significant motor and cognitive disabilityCitation1. The single greatest risk factor for the development of PD is advancing age, with associated mitochondrial dysfunction, oxidative stress damage and inflammationCitation2. Currently, 6-hydroxydopamine (6-OHDA), hydrogen peroxide (H2O2) and nitric oxide (NO) models that result in damage to dopaminergic neurons are widely used to generate Parkinson’s disease-like modelsCitation3–5.
In a recent paper, we described the (E)-3,4-dihydroxystyryl aralkyl sulfones and sulfoxides () that displayed multifunctional neuroprotective effects. This study showed that (E)-3,4-dihydroxystyryl aralkyl sulfones and sulfoxides were found to have greater antioxidative effect, stability, and BBB permeability than the lead compound caffeic acid phenethyl ester (CAPE). Thus, (E)-3,4-dihydroxystyryl aralkyl sulfones and sulfoxides were considered as potential multifunctional neuroprotective agentsCitation6.
Studies show that acetylated phenolic compounds exist in naturally occurring compoundsCitation7–9. It has been well reported that acetylated phenolic compounds exert the same or even higher biological activity, such as the inhibition of lipid peroxidation and inhibition of cancer cells growth, compared to the initial phenolic compoundsCitation10. Additionally, acetylation occurs as a modification of proteins inside the cell. An enzyme that transfers acetyl groups from polyphenolic acetates to proteins has been foundCitation11,Citation12. It seems that the acetyl group may also serve as a messenger group. Therefore, the acetyl group may be a structural characteristic that provides significant biological activity. In this study, to further enhance the biological activity and discuss structure-activity relationship, we synthesized acetylated derivatives of (E)-3,4-dihydroxystyryl aralkyl sulfones and sulfoxides, (E)-3,4-diacetoxystyryl sulfone and sulfoxide derivatives, and examined their neuroprotective effects in vitro models of Parkinson's disease.
Materials and methods
Chemistry
Thin-layer chromatography (TLC) was performed on precoated silica gel F254 plates. Detection was by iodine vapor staining and UV light irradiation (UV lamp, model UV-IIB). Column chromatography was carried out with Silica gel H (200–300 mesh or 500 mesh). Melting points were measured on an X4-type apparatus and left uncorrected. 1H NMR and 13C NMR spectra were recorded on a Bruker AVANCE III-400 spectrometer (Beijing, China), Chemical shifts δ in ppm with Me4Si as internal standard, coupling constants J in Hertz. High-resolution mass spectrum (HRMS) was recorded on a Bruker Apex IV FTMS spectrometer (Leipzig, Germany). Pyridine and acetic anhydride were purchased from commercial sources. (E)-3,4-dihydroxystyryl aralkyl sulfones and sulfoxides were synthesized as described previously. Some physical and analytical data for target compounds are shown in .
Table 1. Physical and analytical data for target compounds.
Synthesis of the compounds
General procedure for the synthesis of (E)-3,4-diacetoxystyryl sulfones and sulfoxides (1–16). The (E)-3,4-dihydroxystyryl aralkyl sulfone and sulfoxides (1 mmol) was added to a solution of the pyridine (2.2 mmol) in acetic anhydride (3 mL) and stirred at RT until absence of the (E)-3,4-dihydroxy styrylaralkyl sulfones and sulfoxides (checked by TLC). The reaction mixture was diluted with H2O and extracted with ethyl acetate. The combined organic fractions were washed with brine, dried (Na2SO4), and concentrated under reduced pressure. Purification of the crude residue by column chromatography (petroleum ether: ethyl acetate) afforded the title compound ().
E-acetic acid 2-acetoxy-4-[2-(phenylmethanesulfonyl)vinyl]phenyl ester (1): White solid (94%); m.p. 163–164 °C. 1H NMR (400 MHz, CDCl3) δ 7.24–7.41 (m, 9H, ArH, ArCH = CHSO2), 6.66 (d, J = 15.2 Hz, 1H, ArCH = CHSO2), 4.32 (s, 2H, ArCH2SO2), 2.33 (s, 3H, p-ArOCOCH3), 2.32 (s, 3H, m-ArOCOCH3). 13C NMR (100 MHz, CDCl3) δ 167.98, 167.88, 144.36, 143.66, 142.52, 130.93, 129.04, 128.97, 127.88, 126.87, 125.09, 124.28, 123.34, 61.87, 20.62, 20.67. HR-MS (ESI+) m/z: 375.08969 [M + H]+. Found: 375.08979 [M + H]+, 392.11623 [M + NH4]+.
E-acetic acid 2-acetoxy-4-[2-(2-phenylethanesulfonyl)vinyl]phenyl ester (2): White solid (96%); m.p. 99--100 °C. 1H NMR (400 MHz, CDCl3) δ 7.54 (d, J = 15.6 Hz, 1H, ArCH = CHSO2), 7.22–7.36 (m, 8H, ArH,), 6.65 (d, J = 15.6 Hz, 1H, ArCH = CHSO2), 3.35-3.39 (m, 2H, ArCH2CH2SO2), 3.13-3.17 (m, 2H, ArCH2CH2SO2), 2.34 (s, 3H, p-ArOCOCH3), 2.33 (s, 3H, m-ArOCOCH3). 13C NMR (100 MHz, CDCl3) δ 168.01, 167.86, 144.39, 143.06, 142.60, 137.46, 130.91, 128.97, 128.50, 127.07, 127.01, 125.95, 124.25, 123.36, 56.54, 28.89, 20.68, 20.63. HR-MS (ESI+) m/z: 389.10534 [M + H]+. Found: 389.10514 [M + H]+, 406.13183 [M + NH4]+.
E-acetic acid 2-acetoxy-4-[2-(3-phenylpropane-1-sulfonyl)vinyl]phenyl ester (3): White solid (92%); m.p. 76–77 °C. 1H NMR (400 MHz, CDCl3) δ 7.54(d, J = 15.6 Hz, 1H, ArCH = CHSO2), 7.18-7.42 (m, 8H, ArH), 6.75 (d, J = 15.6 Hz, 1H, ArCH = CHSO2), 3.05 (m, 2H, SO2CH2CH2CH2Ar), 2.79 (t, 2H, SO2CH2CH2CH2Ar), 2.34 (s, 3H, p-ArOCOCH3), 2.33 (s, 3H, m-ArOCOCH3), 2.16 (m, 2H, SO2CH2CH2CH2Ar). 13C NMR (100 MHz, CDCl3) δ 168.02, 167.85, 144.39, 143.05, 142.63, 139.80, 130.93, 128.70, 128.48, 127.03, 126.53, 125.81, 124.29, 123.29, 54.31, 34.21, 24.14, 20.67, 20.63. HR-MS (ESI+) m/z: 403.12099 [M + H]+. Found: 403.12080 [M + H]+.
E-acetic acid 2-acetoxy-4-[2-(4-phenylbutane-1-sulfonyl)vinyl]phenyl ester (4): White solid (93%); m.p. 79–80 °C. 1H NMR (400 MHz, CDCl3) δ 7.54 (d, J = 15.6 Hz, 1H, ArCH = CHSO2), 7.16-7.42 (m, 8H, ArH), 6.76 (d, J = 15.6 Hz, 1H, ArCH = CHSO2), 3.08 (m, 2H, SO2CH2CH2CH2CH2Ar), 2.67 (m, 2H, SO2CH2CH2CH2CH2Ar), 2.35 (s, 3H, p-ArOCOCH3), 2.34 (s, 3H, m-ArOCOCH3), 1.77-1.90 (m, 4H, SO2CH2CH2CH2CH2Ar). 13C NMR (100 MHz, CDCl3) δ 168.02, 167.85, 144.38, 143.05, 142.64, 141.21, 130.97, 128.49, 128.36, 127.00, 126.10, 124.29, 123.29, 54.97, 35.31, 30.10, 22.21, 20.67. HR-MS (ESI+) m/z: 417.13664 [M + H]+. Found: 417.13620 [M + H]+, 434.16292 [M + NH4]+.
E-acetic acid 2-acetoxy-4-[2-(4-chlorophenylmethanesulfonyl)vinyl]phenyl ester (5): White solid (91%); m.p. 136–137 °C. 1H NMR (400 MHz, CDCl3) δ 7.25–7.41 (m, 8H, ArH, ArCH = CHSO2), 6.67 (d, J = 15.6 Hz, 1H, ArCH = CHSO2), 4.28 (s, 2H, ArCH2SO2), 2.33 (s, 3H, p-ArOCOCH3), 2.32 (s, 3H, m-ArOCOCH3). 13C NMR (100 MHz, CDCl3) δ 167.99, 167.87, 144.50, 144.06, 142.63, 135.30, 132.22, 130.75, 129.22, 126.99,126.34, 124.85, 124.35, 123.39, 61.05, 20.68, 20.63. HR-MS (ESI+) m/z: 409.05071 [M + H]+. Found: 409.05014 [M + H]+, 426.07673 [M + NH4]+.
E-acetic acid 2-acetoxy-4-[2-(4-tertButylphenylmethanesulfonyl)vinyl]phenyl ester (6): White solid (90%); m.p. 146–147 °C. 1H NMR (400 MHz, CDCl3) δ 7.23–7.41 (m, 8H, ArH, ArCH = CHSO2), 6.66 (d, J = 15.2 Hz, 1H, ArCH = CHSO2), 4.28 (s, 2H, ArCH2SO2), 2.31 (s, 6H, 2ArOCOCH3), 2.33 (s,3H, COCH3), 1.31 (s, 9H, (CH3)3CAr). 13C NMR (100 MHz, CDCl3) δ 167.96, 167.89, 152.15, 144.31, 143.52, 142.58, 131.05, 130.77, 130.62, 126.82, 125.95, 125.33, 124.68, 124.26, 123.34, 61.55,34.70, 31.25, 20.68, 20.62. HR-MS (ESI+) m/z: 431.15229 [M + H]+. Found: 431.15244 [M + H]+, 448.17909 [M + NH4]+.
E-acetic acid 2-acetoxy-4-[2-(4-trifluoromethylphenylmethanesulfonyl)vinyl]phenyl ester (7): White solid (92%); m.p. 134–135 °C. 1H NMR (400 MHz, CDCl3) δ 7.26–7.69 (m, 8H, ArH, ArCH = CHSO2), 6.60 (d, J = 15.6 Hz, 1H, ArCH = CHSO2), 4.37 (s, 2H, ArCH2SO2), 2.33 (s, 6H, 2COCH3). 13C NMR (100 MHz, CDCl3) δ 168.01, 167.87, 144.60, 144.40, 142.66, 131.81, 131.36, 130.63, 127.01, 125.93,125.89, 124.74, 124.38, 123.42, 61.30, 20.66, 20.61. HR-MS (ESI+) m/z: 443.07707 [M + H]+. Found: 443.07684 [M + H]+, 460.10333 [M + NH4]+.
E-acetic acid 2-acetoxy-4-[2-(4-methoxyphenylmethanesulfonyl)vinyl]phenyl ester (8): White solid (95%); m.p. 101–102 °C. 1H NMR (400 MHz, CDCl3) δ 7.38 (d, J = 15.6 Hz, 1H, ArCH = CHSO2), 6.91-7.33 (m, 7H, ArH), 6.65 (d, J = 15.6 Hz, 1H, ArCH = CHSO2), 4.27 (s, 2H, ArCH2SO2), 3.83 (s, 3H, CH3OAr), 2.33 (s, 3H, p-ArOCOCH3), 2.32 (s, 3H, m-ArOCOCH3). 13C NMR (100 MHz, CDCl3) δ 167.98, 167.88, 160.18, 144.32, 143.50, 142.58, 132.11, 131.02, 126.86, 125.18, 124.27,123.32, 119.63, 114.42, 61.24,55.33, 20.67, 20.63. HR-MS (ESI+) m/z: 422.12680 [M + NH4]+. Found: 422.12660 [M + NH4]+, 427.08177 [M + Na]+.
E-acetic acid 2-acetoxy-4-[2-(phenylmethanesulfinyl)vinyl]phenyl ester (9): White solid (90%); m.p. 137–138 °C. 1H NMR (400 MHz, CDCl3) δ 7.06–7.40 (m, 9H, ArH, ArCH = CHSO), 6.74 (d, J = 15.6 Hz, 1H, ArCH = CHSO), 4.10 (q, 2H, ArCH2SO), 2.33 (s, 3H, p-ArOCOCH3), 2.32 (s, 3H, m-ArOCOCH3). 13C NMR (100 MHz, CDCl3) δ 168.09, 142.94, 142.42, 135.13, 132.77, 131.16, 130.31, 129.18, 128.91, 128.53, 125.87, 123.97, 122.30, 61.11, 20.68. HR-MS (ESI+) m/z: 359.09477 [M + H]+. Found: 359.09541 [M + H]+.
E-acetic acid 2-acetoxy-4-[2-(2-phenylethanesulfinyl)vinyl]phenyl ester (10): White solid (94%); m.p. 116–117 °C. 1H NMR (400 MHz, CDCl3) δ 7.20–7.36 (m, 9H, ArH, ArCH = CHSO), 6.78 (d, J = 15.2 Hz, 1H, ArCH = CHSO), 2.99-3.19 (m, 4H, ArCH2CH2SO), 2.32–2.36 (m, 6H, 2 ArOCOCH3). 13C NMR (100 MHz, CDCl3) δ 168.11, 168.06, 142.96, 142.46, 138.72, 134.95, 132.72, 131.49, 128.84, 128.63, 126.81, 125.98, 123.99, 122.28, 55.01, 54.97, 27.90, 20.67. HR-MS (ESI+) m/z: 373.11042 [M + H]+. Found: 373.11077 [M + H]+.
E-acetic acid 2-acetoxy-4-[2-(3-phenylpropane-1-sulfinyl)vinyl]phenyl ester (11): White solid (94%); m.p. 110–111 °C. 1H NMR (400 MHz, CDCl3) δ 7.16–7.35 (m, 9H, ArH,), 6.75 (d, J = 15.6 Hz, 1H, ArCH = CHSO), 2.74–2.86 (m, 4H, SOCH2CH2CH2Ar), 2.33 (s, 3H, m-ArOCOCH3), 2.32 (s, 3H, p-ArOCOCH3), 2.12 (m, 2H, SOCH2CH2CH2Ar). 13C NMR (100 MHz, CDCl3) δ 168.14, 168.08, 142.93, 142.45, 140.35, 134.76, 132.74, 131.63, 128.62, 128.52, 126.36, 126.00, 123.98, 122.24, 53.02, 34.63, 23.48, 20.67. HR-MS (ESI+) m/z: 387.12607 [M + H]+. Found: 387.12606 [M + H]+.
E-acetic acid 2-acetoxy-4-[2-(4-phenylbutane-1-sulfinyl)vinyl]phenyl ester (12): Colorless liquid (95%). 1H NMR (400 MHz, CDCl3) δ 7.17–7.37 (m, 9H, ArH, ArCH = CHSO), 6.78 (d, J = 15.6 Hz, 1H, ArCH = CHSO), 2.82 (m, 2H, SOCH2CH2CH2CH2Ar), 2.69 (m, 2H, SOCH2CH2CH2CH2Ar), 2.34 (s, 3H, p-ArOCOCH3), 2.33 (s, 3H, m-ArOCOCH3), 1.77-1.88 (m, 4H, SOCH2CH2CH2CH2Ar). 13C NMR (100 MHz, CDCl3) δ 168.14, 168.07, 142.93, 142.46, 141.55, 134.81, 132.77, 131.71, 128.45, 128.39, 126.00, 123.98, 122.24, 53.88, 35.49, 30.56, 21.63, 20.67. HR-MS (ESI+) m/z: 401.14172 [M + H]+. Found: 401.14104 [M + H]+.
E-acetic acid 2-acetoxy-4-[2-(4-chlorophenylmethanesulfinyl)vinyl]phenyl ester (13): White solid (96%); m.p. 119–120 °C. 1H NMR (400 MHz, CDCl3) δ 7.03–7.37 (m, 8H, ArH, ArCH = CHSO), 6.72 (d, J = 15.6 Hz, 1H, ArCH = CHSO), 4.04 (q, 2H, ArCH2SO), 2.32 (m, 6H, 2ArOCOCH3). 13C NMR (100 MHz, CDCl3) δ 168.09, 143.05, 142.45, 135.54, 135.50, 134.63, 132.58, 131.65, 130.80, 129.05, 127.63, 125.93, 124.03, 122.31, 59.96, 20.67. HR-MS (ESI+) m/z: 393.05580 [M + H]+. Found: 393.05618 [M + H]+.
E-acetic acid 2-acetoxy-4-[2-(4-tertButylphenylmethanesulfinyl)vinyl]phenyl ester (14): White solid (97%); m.p. 85–86 °C. 1H NMR (400 MHz, CDCl3) δ 7.20–7.42 (m, 7H, ArH), 7.08 (d, J = 15.6 Hz, 1H, ArCH = CHSO), 6.77 (d, J = 15.6 Hz, 1H, ArCH = CHSO), 4.08 (q, 2H, ArCH2SO), 2.32 (s, 6H, ArOCH3), 1.33 (s, 9H, (CH3)3Ar). 13C NMR (100 MHz, CDCl3) δ 168.09, 151.59, 142.91, 142.40, 135.01, 132.84, 131.35, 130.03, 126.11, 125.91, 125.87, 123.95, 122.30, 60.83, 34.66, 31.27, 20.68, 20.65. HR-MS (ESI+) m/z: 415.15737 [M + H]+. Found: 415.15809 [M + H]+.
E-acetic acid 2-acetoxy-4-[2-(4-trifluoromethylphenylmethanesulfinyl)vinyl]phenyl ester (15): White solid (93%); m.p. 116–117 °C. 1H NMR (400 MHz, CDCl3) δ 7.05–7.67 (m, 8H, ArH, ArCH = CHSO), 6.74 (d, J = 15.6 Hz, 1H, ArCH = CHSO), 4.12 (q, 2H, ArCH2SO), 2.33 (s, 6H, 2ArOCOCH3). 13C NMR (100 MHz, CDCl3) δ 215.25, 168.04, 143.15, 142.49, 135.84, 132.45, 130.73, 130.69, 125.91, 125.75, 125.72, 125.68, 124.05, 122.32, 60.16, 20.65. HR-MS (ESI+) m/z: 427.08216 [M + H]+. Found: 427.08312 [M + H]+.
E-acetic acid 2-acetoxy-4-[2-(4-methoxyphenylmethanesulfinyl)vinyl]phenyl ester (16): White solid (95%); m.p. 106–107 °C. 1H NMR (400 MHz, CDCl3) δ 6.90–7.30 (m, 8H, ArH, ArCH = CHSO), 6.74 (d, J = 15.6 Hz, 1H, ArCH = CHSO), 4.07 (q, 2H, ArCH2SO), 3.82 (m, 3H, ArOCH3), 2.32 (s, 3H, p-ArOCOCH3), 2.31 (s, 3H, m-ArOCOCH3). 13C NMR (100 MHz, CDCl3) δ 168.10, 159.80, 142.90, 142.41, 135.03, 132.83, 131.52, 131.24, 125.87, 123.96, 122.28, 120.95, 114.34, 60.39, 55.31, 20.67. HR-MS (ESI+) m/z: 389.10534 [M + H]+. Found: 389.10534 [M + H]+, 411.08778 [M + Na]+.
Pharmacology
Inhibiting 6-OHDA and H2O2 -induced cell injury in PC12 cells
The PC12 cells were purchased from the Shanghai Institute of Cell Biology, Chinese Academy of Sciences. Fetal bovine serum, Donor equine serum, trypsin-EDTA, penicillin streptomycin were obtained from Invitrogen (Carlsbad, CA). MTT and DMSO were acquired from AMRESCO (Solon, OH). High-glucose DMEM was obtained from Thermo Scientific (Waltham, MA). 6-OHDA and H2O2 were purchased from Sigma (St. Louis, MO). PC12 cells were maintained in high-glucose DMEM supplemented with 10% fetal bovine serum, 5% horse serum, 100 U mL−1 Penicillin and 100 U mL−1 Streptomycin in a humidified atmosphere of 5% CO2 at 37 °C. PC12 cells were plated in 96-well microplates at a density of 4 × 105 cells mL−1 (100 μL per well). After 24 h of incubation to allow for cell attachment, the PC12 cells were preincubated with samples for 3 h, which were dissolved in DMSO and diluted with medium to the final concentrations. Afterwards, 20 μL of 6-OHDA (diluted with medium to a final concentration of 400 μM) or H2O2 (diluted with medium to a final concentration of 500 μM) solution was added. After 48 h and 5 h, respectively, the cell viability was measured with MTT assay. The absorbance was determined at 570 nm using a microplate reader. Compared with the vehicle control, the viability of cells treated with drugs is calculated by the following formula: OD(drug-treated)/OD(normal cells) × 100%.
Suppression LPS induced NO production in BV2 cells
BV2 cells were purchased from Institute of Basic Medicine, Chinese Academy of Medical Sciences. LPS was purchased from Sigma (St. Louis, MO). BV2 microglial cells were maintained in high-glucose DMEM supplemented with 5% fetal bovine serum, 100 U mL−1 Penicillin and 100 U mL−1 Streptomycin in a humidified atmosphere of 5% CO2 at 37 °C. BV2 microglial cells were plated in 96-well microplates at a density of 4 × 105 cells mL−1 (100 μL per well). After 24 h of incubation to allow for cell attachment, the BV2 microglial cells were preincubated with samples for 3 h, which were dissolved in DMSO and diluted with medium to the final concentrations. Afterwards, 20 μL of LPS solution (diluted with medium to a final concentration of 100 nM) was added. After 24 h, the NO assay kit was used to perform nitrite assays. The NO assay kit was gained from Applygen (Beijing, China). The culture media was mixed with an equal volume of reagent of the NO assay kit in 96-well plates. The absorbance was determined at 540 nm using a microplate reader. The release amount of NO was calculated by the offered linear equation of the NO assay kit. The percentage inhibition of nitric oxide was calculated from the following formula: [(RL − R0 − Rc)/(RL − R0)] × 100%, where RL is the release amount of only LPS treated group, R0 is the release amount of normal control and Rc is the release amount of the test compound and LPS treated group.
Results and discussion
Chemistry
In this study, the synthetic route of (E)-3,4-diacetoxystyryl sulfone and sulfoxide derivatives is shown in Scheme 1. (E)-3,4-dihydroxystyryl aralkyl sulfones and sulfoxides as starting materials were synthesized from aralkyl sulfonylacetic acids or aralkyl sulfinylacetic acids with 3,4-dihydroxy benzaldehyde as described previouslyCitation6. They were acetylated by acetic anhydride under the catalysis of pyridine followed by chromatographic purification to afford the corresponding (E)-3,4-diacetoxystyryl sulfones (1–8) and (E)-3,4-diacetoxystyryl sulfoxides (9–16). Reaction was carried out at room temperature, and the derivatives were obtained after column chromatography in good yields (approximately 95%). All compounds showed a single spot on TLC and had spectral data in accord with their anticipated structure.
Pharmacology
Inhibiting 6-OHDA-induced cell injury in PC12 cells
As a dopaminergic system neurotoxicant, 6-OHDA has been shown to cause key pathophysiological changes of PD, including mitochondrial dysfunction and oxidative stress. When 6-OHDA is oxidized in dopaminergic neurons, it produces reactive oxygen species leading to redox imbalance, which activate various signaling molecules in dopaminergic neuronsCitation13. Thus, 6-OHDA model was used to test the neuroprotective effects of target compounds. The protective effect can be evaluated by the cell viability using MTT assay. The cell viabilities attributable to the protective efficiency of tested compounds (1–16) against 6-OHDA at 40 μM are listed in . The results show that almost all of the tested compounds, particularly 2 and 7, significantly inhibit 6-OHDA-induced cell injury. The protective effects of compound 7 at 10 and 40 μM are shown in . The electron-withdrawing chloro- (5 and 13) and trifluoromethyl- substituted (7 and 15) compounds exhibit higher effects than unsubstituted (1 and 9) and electron-donating tert-butyl- (6 and 14) and methoxyl- substituted (8 and 16) compounds. Comparing these results with previous reported dataCitation6 indicated that, introducing two acetyl groups leads to negligible improvement in inhibition effects of 6-OHDA-induced cell injury.
Figure 3. Protective effect of compound 7 on 6-OHDA-induced toxicity. PC12 cells in 6-well plates were pretreated by the tested compound for 3 h. Then cells were treated with 500 μM 6-OHDA for 12 h. Compare untreated control cultures (a) with those treated with 6-OHDA (b) and 6-OHDA plus compound 7 (10 μM; (c)) and (40 μM; (d)). Compound 7 significantly attenuated 6-OHDA-induced toxicity as revealed by the cell states.
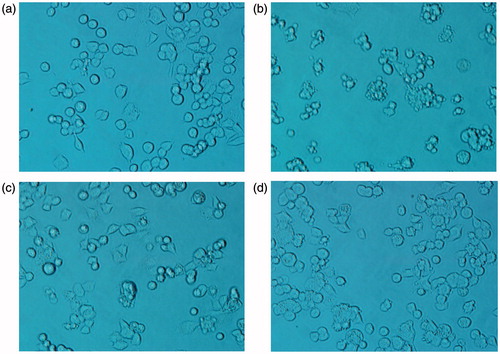
Table 2. Protective effect of 1–16 on 6-OHDA-induced toxicity of PC12 at 40 μM.
Inhibiting H2O2-induced cell injury in PC12 cells
Studies suggest that excessive production of ROS can lead to neuronal apoptosisCitation14, so removing or preventing free radical formation is beneficial for treating PD. The effects of inhibiting free radical of 1–16 were evaluated on H2O2-induced apoptosis in PC12 cells. The cell viabilities attributable to the protective efficiency of tested compounds against H2O2 at 5 μM are listed in . From the results, almost all of target compounds, particularly 3, 6 and 11, significantly inhibit H2O2-induced cell injury. The protective effects of compound 3 at 2, 4 and 8 μM are shown in , which presenting dose dependence. And the chloro (5 and 13), tert-butyl (6 and 14), and trifluoromethyl (7 and 15) substituted compounds display more effective activities compared to unsubstituted (1 and 9) and methoxyl substituted (8 and 16) compounds. Compared these results with previous reported data, it is showed that introducing two acetyl groups don’t result in improvement in inhibition effects of H2O2-induced cell injury.
Figure 4. Compound 3 at 2, 4 and 8 μM inhibit H2O2-induced cell injury. PC12 cells in 96-well plates were pretreated by the tested compound for 3 h. Then the cells were treated with 500 μM H2O2 for 5 h. Cell viability was determined by the MTT assay. The viability of untreated cells as control is defined as 100%.
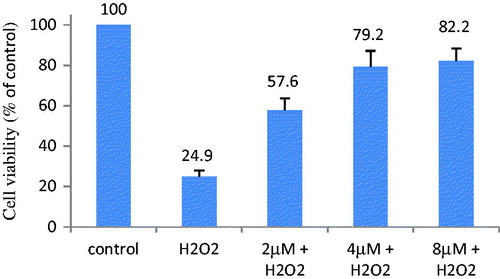
Table 3. Inhibition effects of H2O2-induced cell injury of 1–16 in PC12 cells at 5 μM.
Suppression of LPS-induced NO production in BV2 microglial cells
The free radical nitric oxide (NO) as a main member of neuroinflammatory cytokine is produced by activated microglia cells. Overproduction of NO can cause chronic neuroinflammation and promote neuronal injury, eventually leading to neuronal deathCitation15,Citation16. Therefore, suppression of NO production is a useful strategy for treating PD. In this study, by means of the Griess assayCitation17, we examined the NO production inhibition effect of 1–16. The results are listed in . From the results, most of tested compounds significantly inhibit NO production. It seems that tert-butyl (6 and 14), chloro (5 and 13), trifluoromethyl (7 and 15) and methoxyl (8 and 16) substituted compounds exhibit the more effective activities compared to unsubstituted compounds (1 and 9). Excitingly, compared to un-acetylated compounds, majority of acetylated derivatives reveal higher NO production inhibition effects. Especially compounds 6 (IC50 = 6.3 ± 0.2 µM) and 7 (IC50 = 7.9 ± 0.2 µM) exhibit 2-fold higher activities than the corresponding un-acetylated compounds. In addition, the selected concentrations of tested compounds used in our experiment do not lead to any significant cytotoxicity. Therefore, to a certain extent, tested compounds exhibit relatively low toxicity to neural cells.
Table 4. Suppression effects of LPS induced NO production of 1–16 in BV2 microglial cells.
Conclusion
To find more potent neuroprotective agents and study structure–activity relationship of the derivatives of (E)-3,4-dihydroxystyryl aralkyl sulfones and sulfoxides, in this work, we continue our study on their acetylated derivatives, (E)-3,4-diacetoxystyryl sulfones and sulfoxides. The neuroprotective effects of acetylated derivatives, including antioxidative and antineuroinflammatory properties, were examined by in vitro PD models. The biological results show that tested compounds display potent effects against various kinds of toxicities, including 6-OHDA, H2O2 and NO. The structure-activity relationship of (E)-3,4-diacetoxystyryl sulfone and sulfoxide derivatives is basically consistent with corresponding un-acetylated derivatives. The electron-withdrawing group chloro and trifluoromethyl substituted compounds show more effective neuroprotective effects compared with unsubstituted and electron-donating group methoxyl substituted compounds. Additionally, on the basis of the activity comparison of acetylated and un-acetylated compounds, we find that acetylated compounds show higher antineuroinflammatory properties and similar antioxidative properties to un-acetylated compounds. As a whole, introducing acetyl groups leads to improvement in neuropretective effects. Therefore, (E)-3,4-diacetoxystyryl sulfone and sulfoxide derivatives can be considered as lead candidates in the treatment of neurodegenerative disorders, especially PD.
Acknowledgements
We gratefully acknowledge the National Natural Science Foundation of China (Grants 20972011, 21042009, and 21172014) and Ministry of Science and Technology of China (Grant 2009ZX09301-010).
Declaration of interest
The authors report no conflicts of interest. The authors alone are responsible for the content and writing of this article.
References
- Dauer W, Przedborski S. Parkinson's disease: mechanisms and models. Neuron 2003;39:889–909
- Lin M, Beal M. Mitochondrial dysfunction and oxidative stress in neurodegenerative diseases. Nature 2006;443:787–95
- Jeon BS, Jackson-Lewis V, Burke RE. 6-Hydroxydopamine lesion of the rat substantia nigra: time course and morphology of cell death. Neurodegeneration 1995;4:131–7
- Sanders LH, McCoy J, Hu X, et al. Mitochondrial DNA damage: molecular marker of vulnerable nigral neurons in Parkinson's disease. Neurobiol Dis 2014;70:214–23
- Wu WY, Wu YY, Huang H, et al. Biochanin A attenuates LPS-induced pro-inflammatory responses and inhibits the activation of the MAPK pathway in BV2 microglial cells. Int J Mol Med 2015;35:391–8
- Ning X, Guo Y, Wang X, et al. Design, synthesis, and biological evaluation of (e)-3,4-dihydroxystyryl aralkyl sulfones and sulfoxides as novel multifunctional neuroprotective agents. J Med Chem 2014;57:4302–12
- Shang X, Wang Y, Li C, et al. Acetylated flavonol diglucosides from Meconopsis quintuplinerVia. Phytochemistry 2006;67:511–15
- Gabrieli C, Kokkalou E. A new acetylated glucoside of luteolin and two flavone glucosides from LaVandula stoechas ssp.stoechas. Pharmazie 2003;58:426–7
- Torres P, Poveda A, Jimenez-Barbero J, et al. Regioselective lipase-catalyzed synthesis of 3-o-acyl derivatives of resveratrol and study of their antioxidant properties. J Agric Food Chem 2010;58:807–13
- Fragopoulou E, Nomikos T, Karantonis HC, et al. Biological activity of acetylated phenolic compounds. J Agric Food Chem 2007;55:80–9
- Vane JR. Inhibition of prostaglandin synthesis as a mechanism of action for aspirin-like drugs. Nat New Biol 1971;231:232–5
- Khurana P, Kumari R, Vohra P, et al. Acetoxy drug: protein transacetylase catalyzed activation of human platelet nitric oxide synthase by polyphenolic peracetates. Bioorg Med Chem 2006;14:575–83
- Asaithambi A, Ay M, Jin H, et al. Protein kinase D1 (PKD1) phosphorylation promotes dopaminergic neuronal survival during 6-OHDA-induced oxidative stress. PLoS One 2014;9:e96947
- Sultana R, Newman S, Mohmmad-Abdul H, et al. Protective effect of the xanthate, D609, on Alzheimer’s amyloid β-peptide (1–42)-induced oxidative stress in primary neuronal cells. Free Radical Res 2004;38:449–58
- Singh S, Swarnkar S, Goswami P, Nath C. Astrocytes and microglia: responses to neuropathological conditions. Int J Neurosci 2011;121:589–97
- Pehar M, Vargas MR, Cassina P, et al. Complexity of astrocyte-motor neuron interactions in amyotrophic lateral sclerosis. Neurodegener Dis 2005;2:139–46
- Redondo M, Zarruk JG, Ceballos P, et al. Neuroprotective efficacy of quinazoline type phosphodiesterase 7 inhibitors in cellular cultures and experimental stroke model. Eur J Med Chem 2012;47:175–85