Abstract
Taxifolin is a kind of flavanonol, whose biological ability. The objectives of this study were to investigate the antioxidants and antiradical activities of taxifolin by using different in vitro bioanalytical antioxidant methods including DMPD√+, ABTS√+, , and DPPH√-scavenging effects, the total antioxidant influence, reducing capabilities, and Fe2+-chelating activities. Taxifolin demonstrated 81.02% inhibition of linoleic acid emulsion peroxidation at 30 µg/mL concentration. At the same concentration, standard antioxidants including trolox, α-tocopherol, BHT, and BHA exhibited inhibitions of linoleic acid emulsion as 88.57, 73.88, 94.29, and 90.12%, respectively. Also, taxifolin exhibited effective DMPD√+, ABTS√+,
, and DPPH√-scavenging effects, reducing capabilities, and Fe2+-chelating effects. The results obtained from this study clearly showed that taxifolin had marked antioxidant, reducing ability, radical scavenging and metal-chelating activities. Also, this study exhibits a scientific shore for the significant antioxidant activity of taxifolin and its structure-activity insight.
Introduction
Oxidation process is the electrons transfer between two atoms and stands for a required part of aerobic life and our metabolism. Although problems may occur when the electron flow becomes disconnect, generating free radicals and reactive oxygen species (ROS). A free radical can be described as a molecule or molecular fragment including one or more unpaired electronsCitation1. The existence of unpaired electrons usually confers a considerable degree of reactivity upon a free radical. Those radicals derived from molecular oxygen represent the most important class of such species generated in living organismsCitation2. Recently, there has been an intensive interest in the role of ROS in clinical and experimental medicine. ROS includes non-free radical species like ozone (O3), hydrogen peroxide (H2O2), singlet oxygen (1O2), and free radical varieties like hydroxyl radicals (OH√), superoxide anion radicals (), peroxyl radicals (ROO√), hydroperoxyl radicals (HOO√)Citation3,Citation4. Free radicals may be occurring by living cells during pathophysiological and biochemical processes as well as due to environmental pollutants, radiation, chemicals, and toxinsCitation5. ROS can occur in different ways. ROS (i) are generated during irradiation by X-rays, UV light, and γ-rays; (ii) are present as pollutants in the atmosphere; (iii) are products of metal-catalyzed reactions; (iv) are produced by macrophages and neutrophils during inflammation process; and (v) are by-products of mitochondria-catalyzed electron transport reactions and other biochemical mechanismsCitation1.
A normal cell has convenient prooxidant–antioxidant equilibrium. Although this equilibrium can be changed towards the prooxidants when production of ROS is gone up enormously or when ranges of antioxidants are decreased. This stage is entitled as oxidative stressCitation1,Citation6. As our understanding of the free radicals role in human diseases has increased, antioxidants have attracted broader interest because of their role in inhibiting ROS-relayed reactions and their help in protecting the human body against damage by ROSCitation7. Antioxidant is a compound that prevents or retards the oxidation of substrates even if this compound is available in substantially lower concentration than the oxidized substrates. Also, they have features that safeguard the person from free radicals and effects of ROSCitation8,Citation9. In recent years, interest has remarkably increased in identify alternate safe and innate source materials of food antioxidants, and the search for natural antioxidants, particularly of plant basisCitation3,Citation10. Also, restricting the use of synthetic antioxidants has leaded to an incremental interest of natural antioxidant sources. Therefore, there is an increasing way in consumer priorities based on native antioxidants, all them have given driving force to the initiatives to detect native source material of antioxidantsCitation4,Citation11–13.
Vegetables are rich in term of secondary plants metabolites including flavonoids and other pigments. It was reported that growing consumption of vegetable has been widely growing because of theirs. Most of phytochemicals in vegetables such as phenolic acids β-carotene, tocopherols, flavonol, ascorbate, and polyphenols are identified for their biological activity including antioxidant propertiesCitation1.
According to several clinical, epidemiological, and in vitro studies, phenolic compounds reduce the risk of various degenerative diseases including neurodegenerative diseases, cancer, osteoporosis, diabetes, and cardiovascular diseases due to their antioxidant propertiesCitation14,Citation15. Also, phenolic compound had a wide spectrum of biological activitiesCitation16–29.
Flavonoids are a main class of phenolic compounds and secondary plant metabolites generally located in leaves as water-soluble glycosides in vacuoles of plant cellCitation14. They are widely distributed in plants, fulfilling many functions. Flavonoids are not only present in plants as constitutive agents but are also gathered in plant tissues in response to microbial attack. They are the most important plant pigments for different functions including flower coloration, producing yellow or pigmentation in petals designed to attract pollinator animalsCitation30.
Taxifolin (3,5,7,3,4-pentahydroxy flavanone or dihydroquercetin), a flavononol subclass of flavonoids, are abundant in citrus fruits and onion. Taxifolin is interesting potential component of dietary supplements or antioxidant-rich functional foodCitation31.
In this study, we spectrophotometrically determined the antioxidant and antiradical activities of taxifolin using by different bioanalytical methods. Also, another goal of the current study was to explain the structure and antioxidant or antiradical activities of taxifolin.
Materials and methods
Chemicals
Taxifolin, methionine, riboflavin, linoleic acid, butylated hydroxyanisole, butylated hydroxytoluene, N,N-dimethyl-p-phenylenediamine, 2,2′-azino-bis(3-ethylbenzthiazoline-6-sulfonic acid), 2,9-dimethyl-1,10-phenanthroline, nitroblue tetrazolium, 1,1-diphenyl-2-picryl-hydrazyl, 3-(2-pyridyl)-5,6-bis(4-phenyl-sulfonic acid)-1,2,4-triazine, trichloroacetic acid, and α-tocopherol were purchased commercially from Sigma-Aldrich GmbH, Sternheim, Germany. Ammonium thiocyanate was obtained from Merck. All other chemicals used were of analytical grade and attained from either Sigma-Aldrich (Sternheim, Germany) or Merck (Darmstat, Germany).
Total antioxidant activity of taxifolin
For the determination of total antioxidant activity of taxifolin and positive controls, the ferric thiocyanate method (FTM) was usedCitation32,Citation33. This method, in other words inhibition of linoleic acid emulsion (LAE), was described previouslyCitation34–36. The preparation of stock solution and LAE was defined in our previous studiesCitation1,Citation37. The peroxides consisted through peroxidation of linoleic acid will oxidize Fe2+ to Fe3+, which generates a complex with thiocyanate that has a maximum absorbance at 500 nmCitation38. The assay steps were renewed every 5 h up to arriving a high scale. In linoleic acid emulsion, the percent inhibition of lipid peroxidation (ILP) was computed by the following equation:
where AC is the absorbance value of the control reaction, which includes only phosphate buffer and LAE. In the existing solution, the absorbance value of taxifolin and other tested samples is called ASCitation39,Citation40.
Fe3+-reducing antioxidant power assay
For the determination of Fe3+-reducing ability of taxifolin, Fe3+(CN−)6–Fe2+(CN−)6 reduction method was usedCitation41,Citation42. In brief, different concentrations of taxifolin (10–30 µg/mL) in 0.75 mL of deionized H2O were added with 1.25 mL of phosphate buffer (0.2 M, pH 6.6) and 1.25 mL of potassium ferricyanide [K3Fe(CN)6] (1%). Then, the solution was incubated at 50 °C during 20 min. After incubation period, 1.25 mL of trichloroacetic acid (TCA) was added (10%). Finally, 0.5 mL of FeCl3 (0.1%) was transferred to this mixture and the absorbance value was enrolled at 700 nm in a spectrophotometerCitation3,Citation10.
Cupric ions (Cu2+)-reducing power-CUPRAC assay
Cupric ions (Cu2+)-reducing power was used as a second reducing method for taxifolin. Cu2+-reducing capability was done according to the method described by Apak et al.Citation43 with slight modificationCitation44. Thus, 0.25 mL of CuCl2 solution (0.01 M), 0.25 mL of ethanolic neocuproine solution (7.5 × 10−3 M) and 0.25 mL of NH4Ac buffer solution (1 M) were transferred to a test tube, which contains a taxifolin at different concentrations (10–30 µg/mL). The total volume was completed with distilled H2O to 2 mL and shaken vigorously. The absorbance of samples was recorded at 450 nm after 30 min.
Ferric ions (Fe2+)-chelating activity
Ferric ions (Fe2+)-binding ability of taxifolin was determined according to the method of Dinis et al.Citation45 with minor modificationCitation46,Citation47. Fe2+ capacity of taxifolin was spectrophotometrically recorded at 562 nm. In brief, to 0.1 mL of FeCl2 (0.6 mM) were added taxifolin at different concentrations (10–20 µg/mL) in methanol (0.4 mL). Then, the reaction was started by the 0.1 mL of ferrozine solution addition (5 mM). The solution was mixed and incubated at room temperature for 10 min. Finally, the absorbance value of the mixture was quantified spectrophotometrically at 562 nm versus blank sampleCitation48.
Superoxide anion radical-scavenging activity
Superoxide radicals ()-scavenging activity of taxifolin was performed in accordance with the methodology of Zhishen et al.Citation49 with slightly adjustmentCitation50.
is produced in methionine, riboflavin, enlightens system, and determined by the come down to NBT to generate blue formazan (NBT2+). All mixtures were prepared in phosphate buffer (0.05 M, pH 7.8). The photo-induced reactions were realized by using the fluorescent lamps (20 W). The mixture was enlightened at 25 °C for 40 min. The photochemically reduced riboflavin produced
, which reduced NBT to NBT2+. The absorbance was spectrophotometrically measured at 560 nmCitation51,Citation52.
DPPH√-scavenging activity
DPPH√ radical-scavenging activity of taxifolin was performed according to the previous studiesCitation53,Citation54. In brief, fresh solution of DPPH· (0.1 mM) was prepared in ethanol. To a solution 1.5 mL of taxifolin in ethanol was added to 0.5 mL of this solution (10–30 µg/mL). These mixtures were mixed vigorously and incubated in dark for 30 min. Finally, the absorbance value was recorded at 517 nm in a spectrophotometerCitation55,Citation56.
ABTS√+-scavenging activity
ABTS√+-scavenging activity of taxifolin was done using the spectroscopic method of Re et al.Citation57. The ABTS radical cation (ABTS√+) was acquired by reacting 7 mM solution of ABTS with 2.45 mM K2S2O8. Prior to assay, the ABTS radical cation solution was diluted with ethanol to an absorbance of 0.750 ± 0.05 at 734 nm. Then, 1 mL of ABTS√+ solution was supplement to 3 mL of taxifolin and control solutions. The extent of decolorization is calculated as percentage reduction of absorbanceCitation58.
DMPD√+-scavenging activity
DMPD radical-scavenging ability of taxifolin was done according to the method described by Fogliano et al.Citation59. DMPD (0.1 M) was prepared by dissolving 0.2 g of DMPD in 10 mL of deionized H2O. Then 1 mL of this solution was supplement to acetate buffer (100 mL, 0.1 M, and pH 5.3). DMPD√+ was obtained by adding 0.2 mL of FeCl3 solution (0.05 M). Different concentrations of taxifolin and positive controls (10–30 μg/mL) were transferred in test tubes. Distilled water was added up to complete 0.5 mL of volume and the absorbance value was measured at 505 nm after ten min.
Percentage of chelating or scavenging effects was computed using the following equation:
where AC is the absorbance value of control and AS is the absorbance value of sampleCitation60.
Statistical analysis
Each experiment was implemented in three times. The acquired data were enrolled as mean ± standard deflection and analyzed by SPSS (version 11.5 for Windows 2000, SPSS Inc., Chicago, IL). One-way analysis of type ANOVA was implemented by procedures. Considerable distinctions between means were identified by Duncan's Multiple Range tests, and p < 0.05 was seen as important and p < 0.01 as very important.
Results and discussion
Polyphenols contain one of the most commonly occurring and ubiquitous groups of plant metabolites and represent an integral part of human dietCitation2. Flavonoids are a class of plant secondary metabolites and the most important group of polyphenols. It was reported that flavonoids are the most common polyphenolic group in the human diet. Recently, interest in phenolic compounds, in general, and flavonoids in particular has increased greatly owing to their antioxidant capacity and their possible beneficial food and pharmaceutical applications in human healthCitation61.
Antioxidant activity, which reflected the ability of plant extracts or pure compounds, inhibits the oxidation processCitation62–64. It was demonstrated that addition of taxifolin inhibits oxidation of linoleic acid emulsion (LAE) by ferric thiocyanate method (FTM). As shown in , taxifolin showed greater antioxidative potency. In the current study, the first used antioxidant method is FTM. These methods determine the quantity of peroxides produced throughout the first steps peroxidation of lipid. Lipid oxidation occurs in food and pharmaceutical and is one of the major anxiety both food and pharmaceutical technologiesCitation65,Citation66. It is in charge of nutritional and pharmaceutical qualities and safeties caused by the formation of secondary, potentially toxic compounds. This situation is very important to human health protection and also economically importantCitation67. Taxifolin demonstrated effective inhibition of lipid peroxidation in the LAE. The impression of 30 μg/mL concentration of taxifolin on the lipid peroxidation of LAE is demonstrated in and . It was identified to be 81.02%. Otherwise, the positive controls like trolox, α-tocopherol, BHT, and BHA demonstrated 88.57, 73.88, 94.29, and 90.12% peroxidation of LAE at the above-mentioned concentration, respectively. During the formation of linoleic acid autoxidation, peroxides occur rapidly. These outcomes expressly showed that taxifolin had the greatest antioxidant activity compared with α-tocopherol (73.88%), but lower than BHA (90.12%), BHT (94.29%), and trolox (88.57%).
Figure 1. Total antioxidant activity of taxifolin and standards like trolox, α-tocopherol, BHT, and BHA at the same concentration (30 μg/mL) assayed by the ferric thiocyanate method in the linoleic acid system. The control value reached a maximum 60 h (BHA, butylated hydroxyanisole; BHT, butylated hydroxytoluene).
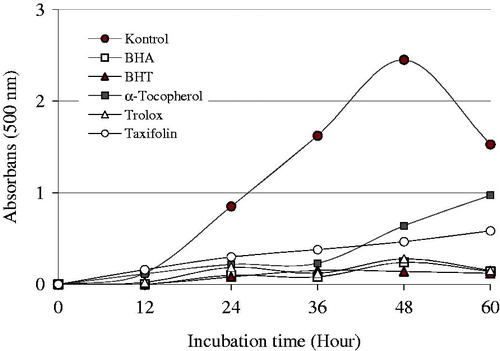
Table 1. Determination of half maximal concentrations (IC50) of taxifolin and standards belonging to Fe2+ chelating, DPPH√, ABTS√+, DMPD√+, and
scavenging assays.
Lipid peroxidation is a free-radical chain reaction, and ROS can quicken this process. The mechanistically approaches of the spectrophotometric analysis of lipid hydroperoxides based on the oxidation of Fe2+ to Fe3+ ions and following chelation of the latter by thiocyanate (SCN−) are thought. The FTM measures the amount of peroxide generated along the first steps of lipid peroxidation. In this test, hydroperoxides produced from linoleic acid supplement to the reaction solution, which has oxidized in air through the experimental period, were immediately evaluated. Thiocyanate and FeCl2 react with one another to generate Fe(SCN−)2 by the agency of hydroperoxidesCitation1,Citation68.
The reducing capability of a bioactive compound can be calculated by means of the direct reduction of Fe[(CN)6]3 to Fe[(CN)6]2Citation69. In this technic, the presence of reductants like taxifolin would result in the reduction of Fe3+ to Fe2+. Addition of free Fe3+ to the reduced product brings about the formation of the intensive Perl's Prussian blue complex, Fe4[Fe(CN−)6]3, which has a strong absorbance at 700 nmCitation70. The Fe3+ reducing assay gets advantage of an electron chain reaction where a ferric salt is utilized as an oxidant. Also, the yellow color of the tested mixture changes to diverse tons of green and blue according as the reducing ability of taxifolin.
As seen in and , taxifolin demonstrated potent Fe3+ reducing capability and these diversities were statistically seen to be considerable important (p < 0.01). The reducing capacity of taxifolin, trolox, α-tocopherol, BHT, and BHA increased constantly when the concentration of sample was increased. Reducing capacity of taxifolin and standard compounds exposed the following order: BHT (3.080, r2: 0.981) ≈ BHA (3.006, r2: 0.979) ≥ Taxifolin (2.847, r2: 0.960) > trolox (1.787, r2: 0.969) > α-tocopherol (1.204, r2: 0.967) at the above-mentioned concentration. The results proved that taxifolin had marked Fe3+-reducing ability.
Figure 2. Reducing power of taxifolin. (A) Fe3+→Fe2+ reductive potential of different concentrations (10–30 μg/mL) of taxifolin (r2: 0.960) and reference antioxidants. (B) Cu2+-reducing ability of different concentrations (10–30 μg/mL) of taxifolin (r2: 0.956) and reference antioxidants. (C) TPTZ-Fe3+→TPTZ-Fe2+ reductive potential of different concentrations (10–30 μg/mL) of taxifolin (r2: 0.993) and reference antioxidants (BHA, butylated hydroxyanisole; BHT, butylated hydroxytoluene).
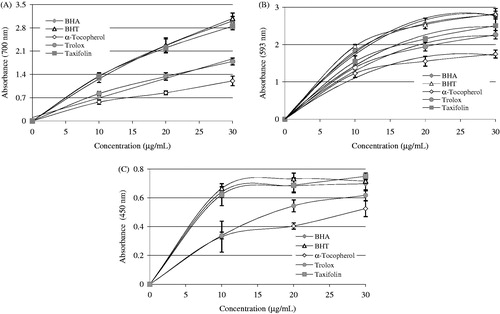
Copper is a vital component for several endogenous antioxidant enzymes. On the other hand, free radicals have been proposed to play a role in the carcinogenesis processCitation71. Hence, the effects of dietary copper levels on the development of cancer have been investigatedCitation72. The CUPRAC method is a simple, rapid, selective, cost-effective, steady, and versatile antioxidant assay useful for a wide variety of polyphenols, as well as for thiols, synthetic antioxidants, and vitamins C and E. Also, this chromogenic redox reaction is conducted at a pH (7.0) and the method allows measuring antioxidants including thiol like glutathione and non-protein thiols. CUPRAC reactions are essentially complete within 30 minCitation43.
Cupric ions (Cu2+) reducing power of 30-μg/mL concentration of taxifolin and standard reducing agents is shown in and . A positive relationship was found between the Cu2+ reducing power and different concentration of the taxifolin (r2: 0.956). It was detected that Cu2+-reducing capacity of taxifolin was addict to concentration (10–30 μg/mL). Cu2+-reducing capability of taxifolin and standard reducing agents at the same concentration (30 μg/mL) showed the following order: taxifolin (0.750 ± 0.24, r2: 0.956) > BHT (0.717 ± 0.17, r2: 0.963) > BHA (0.698 ± 0.49, r2: 0.951) > trolox (0.618 ± 0.39, r2: 0.990) > α-tocopherol (0.525 ± 0.55, r2: 0.968).
The FRAP assay measures the ferric ions (Fe3+)–ferrous ions (Fe2+)-reducing ability of the substance and was initially proposed for the measurement of total antioxidant capacity The Fe3+(CN−)6 reduction method measures the antioxidant effect of any molecule as reducing capability in the reaction. As can seen in and , taxifolin had the most effective reducing capacity using the Fe3+(CN−)6 reduction, Fe3+-TPTZ, and Cu2+-reducing ability when class with the standards including trolox, α-tocopherol, BHT, and BHACitation58. According to results obtained from FRAP assay () reducing power of taxifolin and standard compounds decreased in the following order: BHT (2.826 ± 0.140, r2: 0.987) ≈ BHA (2.811 ± 0.082, r2: 0.996) > taxifolin (2.507 ± 0.136, r2: 0.993) > trolox (2.263 ± 0.114r2: 0.994) > α-tocopherol (1.745 ± 0.108, r2: 0.983). In this method, higher absorbance values indicate higher of reducing ability Fe3+–TPTZ complex. Also, the highest reduction ability was observed for the phenolic BHA. Benzie and Strain reported that the FRAP assay offers a well-known index of antioxidant, or reducing, potential of samples or pure compounds. At low pH values, a Fe3+–TPTZ complex is reduced to the Fe2+–TPTZ, which has maximum absorption at 593 nm with intense blue color of reaction mediumCitation12.
Ferrozine, which reacts with divalent Fe to form a stable magenta complex species, can create complexes with Fe2+. Fe2+ ions are the most efficient pro-oxidants in pharmacology systems and foodCitation72. In the presence of Fe2+-chelating compounds, Ferrozine–Fe2+ complex formation is broken down, resulting in a decrease in the red color of the Ferrozine–Fe2+ complexCitation58. The data seen from and display that taxifolin has a strong capability to bind Fe2+. It is submitting that its main action as a peroxidation inhibitor may be involved to its Fe2+-linking capacityCitation71. Taxifolin prohibited the formation of the ferrous–ferrozine complex. Taxifolin is able to catch ferrous ion before ferrozine. It can convert Fe2+ ions into insoluble metal complexes or generate sterically hindrance, which can prevent the interactions between metals and lipid intermediatesCitation73. The chemical structure of taxifolin and its three metal binding sites is given in . It may probably chelate three ferrous ions (Fe2+) by the agency of its hydroxyl and carbonyl groups. It was known that biological active molecules with structures including functional groups like C–OH and C=O can bind Fe2+ ions. Additionally, the compounds with molecules containing two or more of the following functional groups: –S–, –O–, –OH, –SH, C=O, –NR2, –COOH, and –H2PO3 in favor of structure–function configuration. In this way, the biological active compounds can easily chelate Fe2+ ionsCitation1,Citation74–76. In our prior study, it was demonstrated that l-Carnitine, which is required for the transport of fatty acids from the cytosol into the mitochondria during the breakdown of lipids, similarly chelated Fe2+ ions thanks to the hydroxyl and carbonyl groups. In a slightly different, it was specified that curcumin, natural phenols that are responsible for the yellow color of turmeric, bounded Fe2+ ions thanks to the hydroxyl and carbonyl groupsCitation49. In a similar vein, l-adrenaline, which used for the treatment of a number of conditions including anaphylaxis, cardiac arrest, and superficial bleeding, bounded Fe2+ ions with hydroxyl and amine groupsCitation12. Taxifolin has the following characteristics: 4′,5′-dihydroxy group in ring B, or 4-keto and 3-hydroxy groups in C ring, or 4′-keto groups in C ring and 5,7-dihydroxy group in A ring have the metal-chelated properties (). In this study, we demonstrated that a taxifolin molecule linked to three ferrous ions (Fe2+) by the agency of hydroxyl and carbonyl groups. Lately, Fiorucci et al. have demonstrated that quercetin, a plant pigment with a molecular structure like or derived from flavone, complexioned metal ions in the same wayCitation77. Their common structural feature is the diphenylpropane moiety, which consists of two aromatic rings linked through three carbon atoms that together generally form an oxygenated heterocyclic. Also, it was reported that different metals have different binding affinities of the flavonoidsCitation78. Thus, for example, iron has the highest binding affinity for the 3-OH group of ring C, then the catechol group ring B, and at the end of 5-OH group of ring A, while the copper ions bind to the first ring catechol group BCitation79.
Figure 3. Comparison of Fe2+-chelating activity of taxifolin (r2: 0.942) and standards like trolox, EDTA, α-tocopherol, BHT, and BHA at the concentrations of 10–20 mg/mL (BHA, butylated hydroxyanisole; BHT, butylated hydroxytoluene).
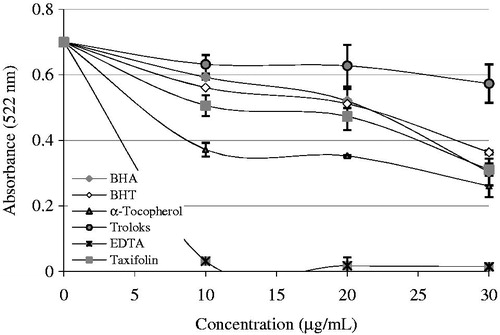
Figure 4. Possible places on taxifolin for chelating the transition metal ions such as Fe2+ in the process of lipid peroxidation.
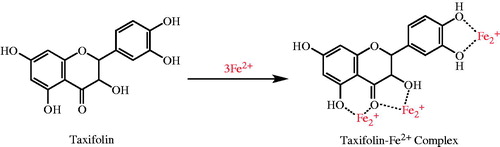
Table 2. Determination of reducing power of taxifolin by K3[Fe(CN)6] reduction and FRAP methods, cupric ions (Cu2+) reduction capacity by Cuprac and FRAP methods*.
In this regard, taxifolin had also effective metal ions-chelating effect. The distinction between taxifolin at different concentrations (10–30 μg/mL) and control value was fixed to be statistically important (p < 0.01, and ). The half maximal inhibitory concentration (IC50) belonging to taxifolin and standard metal chelator compounds including EDTA demonstrated the following order: EDTA (IC50 value: 4.41 μg/mL, r2: 0.940) < α-tocopherol (IC50 value: 19.81 μg/mL, r2: 0.934) < BHA (IC50 value: 30.13 μg/mL, r2: 0.923) < taxifolin (IC50 value: 34.61 μg/mL, r2: 0.945) < BHT (IC50 value: 46.51 μg/mL, r2: 0.945) < trolox (IC50 value: 99.01 μg/mL, r2: 0.918). These results clearly introduce that the Fe2+ ions-chelating effect of taxifolin was higher than trolox and BHT, parallel to BHA, but lower that of α-tocopherol. IC50 represents the concentration of a metal chelator that is required for 50% chelation in vitro. Lower IC50 values indicate higher metal chelation capacity. The results show that taxifolin, being a strong chelating agent, forms highly stable complexes with Fe2+.
DPPH√ becomes a steadier diamagnetic molecule after acceptation of an electron (e−) or hydrogen radical (√H) from an antioxidant compoundCitation67. DPPH radicals scavenging of taxifolin are summarized in and . It is well known that a radical can be stabilizing by the agency of resonance structure of the phenolic compounds. Chemically, it is an ester formed from quinic acid and two units of caffeic acid. Taxifolin has two aromatic rings, which had two phenolic groups (–OH) at the meta- and para- positions with respect to each other. It is this order of arrangement, which is the chief determinant for the strong antioxidant capacity of phenolic compoundsCitation80. It was demonstrated, by studying eight phenolic and analogous compounds, that the antioxidant activity of a molecule increases with increase in the number of hydroxyl groups attached to the aromatic ringCitation81. Also, a positive correlation was further obtained between the number of –OH groups attached to the aromatic ring and the antioxidant activity as well as the antiradical activity of phenolic acidsCitation80,Citation82. Structure–activity research studies have shown that the radical-scavenging efficiency of phenolic compounds is dependent on the presence of –OH groups as well as steric freedomCitation83. Especially, the para-substituted –OH group was found to be highly efficient in radical scavenging. The nature and the position of the substituents with respect to –OH also affect the activity of polyphenols. Previously, the role of –OH groups at the ortho- position in the antioxidant activity of phenolic acids has also been describedCitation84. The extra –OH group in trihydroxy phenolic acid provides greater stability along with higher antioxidant activity. Also, –OH groups not only affect antioxidant ability by intramolecular hydrogen bonding ()Citation84–86, but they also tend to stabilize the antioxidant radical formed. It was reported that the –OH group ortho- to phenol tends to stabilize the radical formed resulting in a lower hydrogen bond dissociation enthalpy and hence an increased antioxidant capacityCitation84. Separation of a hydrogen atom from monophenolic and diphenolic –OH may be readily. As can be seen in , oxygen radicals, which formed in the first level, and supported by the aromatic ring are made profitable. The stability of a radical is measured with possible intermediate stages. The stability of a radical is depending on the number of intermediates. The first radical is stabilized by occurred hiperconjugation. As shown in , depending on the nature of the molecule, more than one mechanism can be written from most stable structure toward the unprofitable radical formation. The arrangement phenolic aromatic markedly influences the antioxidant activity of phenolic acids. Accordingly, several factors such as the number and the position of –OH group, the presence of other functional groups and their position with respect to –OH groups have been shown to affect the antioxidant and antiradical activities. Taxifolin scavenged a DPPH radical on phenolic groups. The reasons of powerful radical-scavenging activity of taxifolin molecule are its conjugation structures and resonance stability of both phenolic rings (). The stabilization of radicals by phenol group of taxifolin is given in . Two radicals are possible by the abstraction of a hydrogen atom from the corresponding orto- or meta- hydroxyl groups from taxifolin.
Figure 5. Radical-scavenging activity of taxifolin. (A) DPPH free radical-scavenging activity of different concentrations (10–30 μg/mL) of taxifolin (r2: 0.931) and reference antioxidants. (B) ABTS radical-scavenging activity of different concentrations (10–30 μg/mL) of taxifolin (r2: 0.925) and reference antioxidants. (C) DMPD radical-scavenging activity of different concentrations (10–30 μg/mL) of taxifolin (r2: 0.907) and reference antioxidants. (D) Superoxide anion radical-scavenging activity of different concentrations (10–30 μg/mL) of taxifolin (r2: 0.982) and reference antioxidants (BHA, butylated hydroxyanisole; BHT, butylated hydroxytoluene; DPPH√, 1,1-diphenyl-2-picryl-hydrazyl free radical; ABTS√+, 2,2′-azino-bis(3-ethylbenzthiazoline-6-sulfonic acid; DMPD√+, N,N-dimethyl-p-phenylenediamine radical).
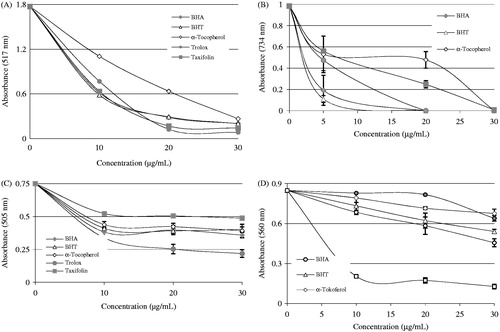
Antiradical potential of taxifolin is evaluated by DPPH√ radicals. DPPH is a dark-colored crystalline powder composed of stable free-radical molecules (DPPH√). It is a stable free radical and converts to a stable diamagnetic molecule by accepting an electron or hydrogen radical. It has an absorbance band at 517 nm, which disappears upon reduction by an antiradical compoundCitation87. It is based on the reduction of a DPPH solution in alcohol in the source of a hydrogen donating antioxidant, owing to the formation of the non-radical form of DPPH-HCitation88. Taxifolin has the ability to convert the steady radical DPPH to the yellow-colored DPPH-H. shows half maximal radical-scavenging concentrations (IC50) of taxifolin and the reference chemical agents like BHA, BHT trolox, and α-tocopherol. IC50 values were found as 77.00 µg/mL for taxifolin (r2: 0.938), 6.93 µg/mL for trolox (r2: 0.945), 13.86 µg/mL for α-tocopherol (r2: 0.973), 86.64 µg/mL for BHT (r2: 0.931), and 86.63 µg/mL for BHA (r2: 0.938). Therefore, DPPH radical-scavenging effects of studied antioxidants increased in the order of trolox > α-tocopherol taxifolin ≥ BHA ≈ BHT ( and ). A lower EC50 value demonstrates a higher DPPH·-scavenging activity.
Another improved technique for the determination of radical scavenging is ABTS√+ scavenging activity. The generation of ABTS√+ defined here includes the directly production of the blue/green ABTS√+ chromophore thanks to the reaction between ABTS and K2S2O8. ABTS•−, the oxidant, was produced by the agency of K2S2O8 oxidation of ABTS2− and the radical cation is calculated spectrophotometrically. This is a direct production of a steady form of radical to create a blue-green ABTS√+ chromophore previous to the reaction with antioxidantsCitation89. ABTS√+ cation can be prepared by running distinct oxidants like permanganate (), chromate (
), and perchlorate (
). In this sense, the oxidizing agent can be called an oxygenation reagent or oxygen-atom transfer agent. Results acquired using K2S2O8 as oxidant show that the occurrence of K2S2O8 increases the ratio of ABTS√+. These radicals were generated in ABTS/K2S2O8 systemCitation1,Citation90.
Efficient radical cation scavenging activity was seen on the tested compounds. As shown in (B), taxifolin is an efficient ABTS√+ scavenger in a concentration-dependent manner (10–30 μg/mL, r2: 0.957). The EC50 value for taxifolin in this analysis was 0.83 μg/mL (r2: 0.937). It is seen that concentration of ABTS√+ (p < 0.01) declines substantially owing to the scavenging capability at all taxifolin concentrations. Moreover, EC50 values for trolox, α-tocopherol, BHT, and BHA were found to be 12.62 μg/mL (r2: 0.986), 1.01 μg/mL (r2: 0.835), 12.30 μg/mL (r2: 0.852), and 12.32 μg/mL (r2: 0.797), respectively. ABTS√+-scavenging efficacy of taxifolin and standards on the ABTS√+ increased in the following order: taxifolin ≥ α-tocopherol >BHA ≈ BHT ≈ trolox ( and ). As well as in DPPH free radical scavenging activity, a lower EC50 value indicates a higher ABTS√+ scavenging activity.
The basic guideline of DMPD√+ scavenging assay is that when it have been used at acidic pH and in convenient oxidant solution, DMPD can form a steady and colored DMPD radical cation. DMPD√+ had a maximum absorbance at 505 nmCitation91. Taxifolin can transfer a hydrogen atom to DMPD√+, quench the color, and produce a solution decoloration. This reaction is rapid and the end point, which is steady, is taken as a measure of the antioxidative competence. Also, this assay shows the talent of radical hydrogen-donors to scavenge the single electron from DMPD√+. Actually, radical cation forms slowly and because of this reason absorbance value increases continuouslyCitation91. On the contrary of the ABTS√+ scavenging method, DMPD√+-scavenging procedure guarantees a very steady end point. This is quite significant when a large-scale screening is necessary. It was shown the main drawback of DMPD√+-scavenging procedure is the fact that its sensitivity and renewability significantly decreased when hydrophobic antioxidants like BHT or α-tocopherol were used. Therefore, these positive controls were not suitable for using in DMPD√+-scavenging assayCitation1,Citation92,Citation93.
The principle of the DMPD assay is that at an acidic pH and in the presence of a suitable oxidant solution DMPD can form a stable and colored radical cation (DMPD√+)Citation93,Citation94. As in the previous both DPPH· and ABTS√+ radical-scavenging methods, taxifolin was an efficient DMPD√+-radical scavenging in a concentration-dependent manner (10–30 μg/mL, r2: 0.951). The EC50 value for taxifolin was found as 231.04 μg/mL (r2: 0.951). This value was found as 173.25 μg/mL (r2: 0.979) for trolox, and 231.01 μg/mL (r2: 0.954) for BHA ( and ). It was reported that hydrophobic antioxidants such as α-tocopherol or BHT did not show activity in this assayCitation95–98. There was an important decline (p < 0.05) in the DMPD√+ concentration owing to the scavenging ability at all taxifolin concentrations. Within different concentrations of taxifolin could not be determined crucial differences in ABTS√+-scavenging potential.
Based on superoxide anion radical-scavenging () activity results, it was found that
scavenging activity of taxifolin is more than that of BHA, BHT, α-tocopherol, and trolox. As seen in and , the IC50 value of
radical scavenging of taxifolin was found to be 9.91 µg/mL (r2: 0.943). Conversely, IC50 values of BHA, BHT, trolox, and α-tocopherol were found as 34.65 µg/mL (r2: 0.995), 69.31 µg/mL (r2: 0.994), 93.01 µg/mL (r2: 0.991), and 46.51 µg/mL (r2: 0.947), respectively. When the EC50 value is lower,
-scavenging activity increases. These resultants demonstrated that when compared with
-scavenging activity, in general, chemical agents' taxifolin had the highest activity, and these distinctions were found to be statistically important.
Conclusion
Phenolic structures comprise a diverse group of molecules classified as secondary metabolites in plants that have a large range of structures and functions. Taxifolin is effective polyphenolic compounds and a food and nutritional supplements. A greater number of potential clinical applications have been reported. Taxifolin was found to be a powerful antioxidant and antiradical activities in different in vitro bioassays when compared with standard antioxidant compounds. As discussed above, taxifolin can be used for minimizing or preventing lipid oxidation in food or pharmaceutical products, delaying the formation of toxic oxidation products, maintaining nutritional quality, and prolonging the shelf life of food or pharmaceutical materials.
Declaration of interest
The authors declare no conflict of interest. S. H. Alwasel would like to extend his sincere appreciation to the Research Chairs Program at King Saud University for funding this research.
References
- Gülçin İ. Antioxidant activity of food constituents – an overview. Arch Toxicol 2012;86:345–91
- Valko M, Rhodes CJ, Moncol J, et al. Free radicals, metals and antioxidants in oxidative stress-induced cancer. Chem Biol Interact 2006:160;1–40
- Gülçin İ. Antioxidant and antiradical activities of l-Carnitine. Life Sci 2006;78:803–11
- Bursal E, Gülçin İ. Polyphenol contents and in vitro antioxidant activities of lyophilized aqueous extract of kiwifruit (Actinidia deliciosa). Food Res Int 2011;44:1482–9
- Elmastaş M, Gülçin İ, Beydemir Ş, et al. A study on the in vitro antioxidant activity of juniper (Juniperus communis L.) seeds extracts. Anal Lett 2006;39:47–65
- Köksal E, Gülçin İ. Antioxidant activity of cauliflower (Brassica oleracea L.). Turk J Agric For 2008;32:65–78
- Kancheva VD, Kasaikina OT. Bio-antioxidants – a chemical base of their antioxidant activity and beneficial effect on human health. Curr Med Chem 2013;20:4784–805
- Gülçin İ, Tel AZ, Kirecci E. Antioxidant, antimicrobial, antifungal and antiradical activities of Cyclotrichium niveum (Boiss.) Manden and Scheng. Int J Food Propert 2008;11:450–71
- Gülçin İ, Elias R, Gepdiremen A, et al. A comparative study on the antioxidant activity of fringe tree (Chionanthus virginicus L.) extracts. Afr J Biotechnol 2007;6:410–18
- Gülçin İ. Antioxidant activity of caffeic acid (3,4-dihydroxycinnamic acid). Toxicology 2006;217:213–20
- Gülçin İ. In vitro prooxidant effect of caffeine. J Enzyme Inhib Med Chem 2008;23:149–52
- Gülçin İ. Antioxidant activity of l-adrenaline: an activity–structure insight. Chem Biol Interact 2009;179:71–80
- Gülçin İ. Antioxidant activity of eugenol – a structure and activity relationship study. J Med Food 2011;14:975–85
- Bimpilas A, Tsimogiannis D, Balta-Brouma K, et al. Evolution of phenolic compounds and metal content of wine during alcoholic fermentation and storage. Food Chem 2015;178:164–71
- Gülçin İ, Beydemir S. Phenolic compounds as antioxidants: carbonic anhydrase isoenzymes inhibitors. Mini Rev Med Chem 2013;13:408–30
- ArasHisar Ş, Hisar O, Beydemir Ş, et al. Effect of vitamin E on carbonic anhydrase enzyme activity in rainbow trout (Oncorhynchus mykiss) erythrocytes in vitro and in vivo. Acta Vet Hung 2004;52:413–22
- Çoban TA, Beydemir Ş, Gülçin İ, Ekinci D. Morphine inhibits erythrocyte carbonic anhydrase in vitro and in vivo. Biol Pharm Bull 2007;30:2257–61
- Gülçin İ, Beydemir Ş, Çoban TA, Ekinci D. The inhibitory effect of dantrolene sodium and propofol on 6-phosphogluconate dehydrogenase from rat erythrocyte. Fresen Environ Bull 2008;17:1283–7
- Şişecioğlu M, Çankaya M, Gülçin İ, Özdemir M. The Inhibitory effect of propofol on lactoperoxidase. Protein Peptide Lett 2009;16:46–9
- Şişecioğlu M, Çankaya M, Gülçin İ, Özdemir M. Interactions of melatonin and serotonin to lactoperoxidase enzyme. J Enzyme Inhib Med Chem 2010;25:779–83
- Öztürk Sarıkaya SB, Gülçin İ, Supuran CT. Carbonic anhydrase inhibitors. Inhibition of human erythrocyte isozymes I and II with a series of phenolic acids. Chem Biol Drug Des 2010;75:515–20
- Innocenti A, Öztürk Sarıkaya SB, et al. Carbonic anhydrase inhibitors. Inhibition of mammalian isoforms I-XIV with a series of natural product polyphenols and phenolic acids. Bioorg Med Chem 2010;18:2159–64
- Şişecioğlu M, Gülçin İ, Çankaya M, et al. The effects of norepinephrine on lactoperoxidase enzyme (LPO). Sci Res Essays 2010;5:1351–6
- Şentürk M, Gülçin İ, Beydemir Ş, et al. In vitro inhibition of human carbonic anhydrase I and II isozymes with natural phenolic compounds. Chem Biol Drug Des 2011;77:494–9
- Öztürk Sarıkaya SB, Topal F, Şentürk M, et al. In vitro inhibition of α-carbonic anhydrase isozymes by some phenolic compounds. Bioorg Med Chem Lett 2011;21:4259–62
- Şişecioğlu M, Gülçin İ, Çankaya M, Özdemir H. The inhibitory effects of l-adrenaline on lactoperoxidase enzyme (LPO) purified from buffalo milk. Int J Food Propert 2012;15:1182–9
- Topal M, Gülçin İ. Rosmarinic acid: a potent carbonic anhydrase isoenzymes inhibitor. Turk J Chem 2014;38:894–902
- Scozzafava A, Passaponti M, Supuran CT, Gülçin İ, Carbonic anhydrase inhibitors: guaiacol and catechol derivatives effectively inhibit certain human carbonic anhydrase isoenzymes (hCA I, II, IX, and XII). J Enzyme Inhib Med Chem 2015. [Epub ahead of print]. doi:10.3109/14756366.2014.956310
- Arabaci B, Gülçin İ, Alwasel S, Capsaicin: A potent inhibitor of carbonic anhydrase isoenzymes. Molecules 2015;19:10103–14
- Harborne JB, Williams CA. Advances in flavonoid research since 1992. Phytochemistry 2000;55:481–504
- Rice Evans CA, Miller NJ, Paganga G. Structure–antioxidant activity relationships of flavonoids and phenolic acids. Free Radical Biol Med 1996;20:933–56
- Gülçin İ, Alici HA, Cesur M. Determination of in vitro antioxidant and radical scavenging activities of propofol. Chem Pharm Bull 2005;53:281–5
- Gülçin İ, Mshvildadze V, Gepdiremen A, Elias R. Antioxidant activity of saponins isolated from ivy: α-hederin, hederasaponin-C, hederacolchiside-E and hederacolchiside F. Planta Med 2004;70:561–3
- Gülçin İ, Büyükokuroğlu ME, Oktay M, Küfrevioğlu Öİ. On the in vitro antioxidant properties of melatonin. J Pineal Res 2002;33:167–71
- Gülçin İ. Comparison of in vitro antioxidant and antiradical activities of l-tyrosine and l-Dopa. Amino Acids 2007;32:431–8
- Gülçin İ. Antioxidant properties of resveratrol: a structure–activity insight. Innov Food Sci Emerg 2010;11:210–18
- Gülçin İ, Elias R, Gepdiremen A, Boyer L. Antioxidant activity of lignans from fringe tree (Chionanthus virginicus L.). Eur Food Res Technol 2006;223:759–67
- Gülçin İ, Daştan A. Synthesis of dimeric phenol derivatives and determination of in vitro antioxidant and radical scavenging activities. J Enzyme Inhib Med Chem 2007;22:685–95
- Oyaizu M. Studies on products of browning reaction: antioxidative activities of products of browning reaction prepared from glucosamine. Jpn J Nutr 1986;44:307–315
- Elmastas M, Gülçin İ, Işıldak Ö, et al. Antioxidant capacity of bay (Laurus nobilis L.) leaves extracts. J Iran Chem Soc 2006;3:258–66
- Gülçin İ, Elmastaş M, Aboul-Enein HY. Determination of antioxidant and radical scavenging activity of basil (Ocimum basilicum) assayed by different methodologies. Phytother Res 2007;21:354–61
- Bursal E, Köksal E, Gülçin İ, et al. Antioxidant activity and polyphenol content of cherry stem (Cerasus avium L.) determined by LC-MS/MS. Food Res Int 2013;51:66–74
- Apak R, Güçlü K, Özyürek M, Karademir SE. A novel total antioxidant capacity index for dietary polyphenols, vitamin C and E, using their cupric ion reducing capability in the presence of neocuproine: The CUPRAC method. J Agric Food Chem 2004;52:7970–81
- Gülçin İ, Topal F, Çakmakçi R, et al. Pomological features, nutritional quality, polyphenol content analysis and antioxidant properties of domesticated and three wild ecotype forms of raspberries (Rubus idaeus L.). J Food Sci 2011;76:C585–93
- Dinis TCP, Madeira VMC, Almeida LM. Action of phenolic derivatives (acetaminophen, salicylate, and 5-aminosalicylate) as inhibitors of membrane lipid peroxidation and as peroxyl radical scavengers. Arch Biochem Biophys 1994;315:161–9
- Gülçin İ, Şat İG, Beydemir Ş, et al. Comparison of antioxidant activity of clove (Eugenia caryophylata Thunb) buds and lavender (Lavandula stoechas L.). Food Chem 2004;87:393–400
- Çakmakçı S, Topdaş EF, Kalın P, et al. Antioxidant capacity and functionality of oleaster (Elaeagnus angustifolia L.) flour and crust in a new kind of fruity ice cream. Int J Food Sci Technol 2015;50:472–81
- Sehitoglu MH, Han H, Kalin P, et al. Pistachio (Pistacia vera L.) Gum: a potent inhibitor of reactive oxygen species. J Enzyme Inhib Med Chem 2015;30:264–9
- Zhishen J, Mengcheng T, Jianming W. The determination of flavonoid contents in mulberry and their scavenging effects on superoxide radicals. Food Chem 1999;64:555–9
- Gülçin İ, Beydemir S, Sat İG, Kuvrevioglu Öİ. Evaluation of antioxidant activity of cornelian cherry (Cornus mas L.). Acta Aliment Hung 2005;34:193–202
- Gülçin İ, Elias R, Gepdiremen A, et al. Antioxidant secoiridoids from fringe tree (Chionanthus virginicus L.). Wood Sci Technol 2009;43:195–212
- Gülçin İ, Mshvildadze V, Gepdiremen A, Elias R. Antioxidant activity of a triterpenoid glycoside isolated from the berries of Hedera colchica: 3-O-(β-d-glucopyranosyl)-hederagenin. Phytother Res 2006;20:130–4
- Ak T, Gülçin İ. Antioxidant and radical scavenging properties of curcumin. Chem Biol Interact 2008;174:27–37
- Gülçin İ, Mshvildadze V, Gepdiremen A, Elias R. Screening of antioxidant and antiradical activity of monodesmosides and crude extract from Leontice smirnowii Tuber. Phytomedicine 2006;13:343–51
- Gülçin İ. The antioxidant and radical scavenging activities of black pepper (Piper nigrum) seeds. Int J Food Sci Nut 2005;56:491–9
- Gülçin İ, Berashvili D, Gepdiremen A. Antiradical and antioxidant activity of total anthocyanins from Perilla pankinensis decne. J Ethnopharmacol 2005;101:287–93
- Re R, Pellegrini N, Proteggente A, et al. Antioxidant activity applying an improved ABTS radical cation decolorization assay. Free Radical Biol Med 1999;26:1231–7
- Gülçin İ, Bursal E, Şehitoğlu HM, et al. Polyphenol contents and antioxidant activity of lyophilized aqueous extract of propolis from Erzurum, Turkey. Food Chem Toxicol 2010;48:2227–38
- Fogliano V, Verde V, Randazzo G, Ritieni A. Method for measuring antioxidant activity and its application to monitoring the antioxidant capacity of wines. J Agric Food Chem 1999;47:1035–40
- Gülçin İ. Measurement of antioxidant ability of melatonin and serotonin by the DMPD and CUPRAC methods as trolox equivalent. J Enzyme Inhib Med Chem 2008;23:871–6
- Schroeter H, Boyd C, Spencer JPE, et al. MAPK signaling in neurodegeneration: influences of flavonoids and of nitric oxide. Neurobiol Aging 2002;23:861–80
- Gülçin İ, Oktay M, Kireçci E, Küfrevioğlu Öİ. Screening of antioxidant and antimicrobial activities of anise (Pimpinella anisum L.) seed extracts. Food Chem 2003;83:371–82
- Gülçin İ, Büyükokuroğlu ME, Oktay M, Küfrevioğlu Öİ. On the in vitro antioxidant properties of melatonin. J Pineal Res 2002;33:167–71
- Gülçin İ, Büyükokuroğlu ME, Oktay M, Küfrevioğlu Öİ. Antioxidant and analgesic activities of turpentine of Pinus nigra Arn. Subsp. pallsiana (Lamb.) Holmboe. J Ethnopharmacol 2003;86:51–8
- Gülçin İ, Küfrevioğlu Öİ, Oktay M, Büyükokuroğlu ME. Antioxidant, antimicrobial, antiulcer and analgesic activities of nettle (Urtica dioica L.). J Ethnopharmacol 2004;90:205–15
- Oktay M, Gülçin İ, Küfrevioğlu Öİ. Determination of in vitro antioxidant activity of fennel (Foeniculum vulgare) seed extracts. Lebens Wissen Technol 2003;36:263–71
- Yanishlieva NV, Marinova E, Pokorny J. Natural antioxidants from herbs and spices. Eur J Lipid Sci Technol 2006;108:776–93
- Min DB, Boff JM. Chemistry and reaction of singlet oxygen in foods. Comp Rev Food Sci F 2002;1:58–72
- Gülçin İ, Elias R, Gepdiremen A, et al. Antioxidant activity of bisbenzylisoquinoline alkaloids from Stephania rotunda: Cepharanthine and fangchinoline. J Enzyme Inhib Med Chem 2010;25:44–53
- Gülçin İ, Kirecci E, Akkemik E, et al. Antioxidant and antimicrobial activities of an aquatic plant: Duckweed (Lemna minor L.). Turk J Biol 2010;34:175–88
- Gülçin İ, Topal F, Oztürk Sarikaya SB, et al. Polyphenol contents and antioxidant properties of medlar (Mespilus germanica L.). Rec Nat Prod 2011;5:158–75
- Balaydın HT, Gülçin İ, Menzek A, et al. Synthesis and antioxidant properties of diphenylmethane derivative bromophenols including a natural product. J Enzyme Inhib Med Chem 2010;25:685–95
- Talaz O, Gülçin İ, Göksu S, Saracoglu, N. Antioxidant activity of 5,10-dihydroindeno[1,2-b]indoles containing substituents on dihydroindeno part. Bioorg Med Chem 2009;17:6583–9
- Halliwell B, Murcia MA, Chirico S, Aruoma OI. Free radicals and antioxidants in food and in vivo: what they do and how they work. Crit Rev Food Sci Nut 1995;35:7–20
- Rodrigo R, Rivera G. Renal damage mediated by oxidative stress: a hypothesis of protective effects of red wine. Free Radical Biol Med 2002;33:409–22
- Göçer H, Akıncıoğlu A, Öztaşkın N, et al. Synthesis, antioxidant and antiacetylcholinesterase activities of sulfonamide derivatives of dopamine related compounds. Arch Pharm 2013;346:783–92
- Fiorucci SB, Golebiowski J, Cabrol-Bass D, Antonczak S. DFT study of quercetin activated forms involved in antiradical, antioxidant, and prooxidant biological processes. J Agric Food Chem 2007; 55:903–11
- Miller CA, Rice-Evans NJ, Paganga G. Structure–antioxidant activity relationships of flavonoids and phenolic acids. Free Radical Biol Med 1996;20:933–56
- Cotelle N. Role of flavonoids in oxidative stress. Cur Top Med Chem 2001;1:569–90
- Sroka Z, Cisowski W. Hydrogen peroxide scavenging, antioxidant and anti-radical activity of some phenolic acids. Food Chem Toxicol 2003;41:753–8
- Galato D, Ckless K, Susin MF, et al. Antioxidant capacity of phenolic and related compounds: correlation among electrochemical, visible spectroscopy methods and structure–antioxidant activity. Redox Rep 2001;6:243–50
- Palafox-Carlos H, Gil-Chávez J, Sotelo-Mundo PR, et al. Antioxidant interactions between major phenolic compounds found in ‘ataulfo’ mango pulp: chlorogenic, gallic, protocatechuic and vanillic acids. Molecules 2012;17:12657–64
- Lu Z, Nie G, Belton PS, et al. Structure–activity relationship analysis of antioxidant ability and neuroprotective effect of gallic acid derivatives. Neurochem Int 2006;48:263–74
- Wright JS, Johnson ER, DiLabio GA. Predicting the activity of phenolic antioxidants: theoretical method, analysis of substituent effects, and application to major families of antioxidants. J Am Chem Soc 2001;123:1173–83
- Nenadis N, Zhang HY, Tsimidou MZ, et al. Structure–antioxidant activity relationship of ferulic acid derivatives: effect of carbon side chain characteristic groups. J Agric Food Chem 2003;51:1874–9
- Siquet C, Paiva-Martins F, Lima JLFC, et al. Antioxidant profile of dihydroxy- and trihydroxyphenolic acids – a structure–activity relationship study. Free Radical Res 2006;40:433–42
- Bursal E, Köksal E, Gülçin İ, et al. Antioxidant activity and polyphenol content of cherry stem (Cerasus avium L.) determined by LC-MS/MS. Food Res Int 2013;51:66–74
- Şerbetçi Tohma H, Gülçin İ. Antioxidant and radical scavenging activity of aerial parts and roots of Turkish liquorice (Glycyrrhiza glabra L.). Int J Food Propert 2010;13:657–71
- Matthäus B. Antioxidant activity of extracts obtained from residues of different oilseeds. J Agric Food Chem 2002;50:3444–52
- Gülçin İ, Beydemir S, Topal F, et al. Apoptotic, antioxidant and antiradical effects of majdine and isomajdine from Vinca herbacea Waldst. and kit. J Enzyme Inhib Med Chem 2012;27:587–94
- Gülçin İ, Elmastaş M, Aboul-Enein HY. Antioxidant activity of clove oil – a powerful antioxidant source. Arab J Chem 2012;5:489–99
- Halliwell B. Antioxidants and human disease: a general introduction. Nut Rev 1997;55:S44–9
- Göçer H, Gülçin İ. Caffeic acid phenethyl ester (CAPE): correlation of structure and antioxidant properties. Int J Food Sci Nutr 2011;62:821–5
- Çetinkaya Y, Göçer H, Menzek A, Gülçin İ. Synthesis and antioxidant properties of (3,4-dihydroxyphenyl)(2,3,4-trihydroxyphenyl)methanone and its derivatives. Arch Pharm 2012;345:323–34
- Köksal E, Gülçin İ, Öztürk Sarıkaya SB, Bursal E. On the in vitro antioxidant activity of silymarine. J Enzyme Inhib Med Chem 2009;24:395–405
- Gülçin İ, Beydemir S, Topal F, et al. Apoptotic, antioxidant and antiradical effects of majdine and isomajdine from Vinca herbacea Waldst. and kit. J Enzyme Inhib Med Chem 2012;27:587–94
- Aksu K, Topal F, Gülçin I, et al. Acetylcholinesterase inhibitory and antioxidant activities of novel symmetric sulfamides derived from phenethylamines. Arch Pharm 2015;348:446–55
- Oztaskın N, Çetinkaya Y, Taslimi P, et al. Antioxidant and acetylcholinesterase inhibition properties of novel bromo-phenol derivatives. Bioorg Chem 2015;60:49–57