Abstract
Dopaminergic neurons (PC12 cells) were treated with different doses of titanium dioxide nanoparticles (TiO2-NPs), to investigate their effects on α-Synuclein (α-Syn) aggregation and their mechanism of action. Western blotting and immunofluorescent staining were performed. Exposure to TiO2-NPs increased α-Syn expression (p < 0.05) and induced dose-dependent α-Syn aggregation. Pretreatment with N-acetylcysteine partially inhibited α-Syn expression induced by a 200 μg/ml dose of TiO2-NPs. TiO2-NPs reduced the expressions of parkin and ubiquitin C-terminal hydrolase protein, and were associated with oxidative stress in PC12 cells. Dysfunction of the ubiquitin-proteasome system also contributed to α-Syn aggregation. The potentially neurotoxic TiO2-NPs may cause Parkinson's disease.
Introduction
The nigral-striatal dopamine system plays an important role in stabilizing free movement, maintaining muscle tone, and regulating posture. Parkinson's disease can be largely attributed to obvious reduction of dopamine and loss of dopaminergic neurons in the substantia nigra and the striatum. CitationElder et al. (2006) reported that inhalation of manganese oxide (MnO) nanoparticles increased manganese content in the striatum, induced oxidative stress, and caused damage to neuronal tissues. CitationPisanic et al. (2007) reported that exposure of dopaminergic neurons to ferrous oxide (Fe2O3) decreased cell survival and impaired cell differentiation. Our previous data demonstrated that silicon dioxide (SiO2) nanoparticles were easily transferred from the olfactory bulb to the brain (mainly in the striatum and hippocampus), causing striatal damage and dopaminergic toxicity (CitationWu et al. 2011). Accordingly, nanoparticles are potentially neurotoxic. Although common to many neurodegenerative diseases, oxidative stress cannot explain the effects of nanoparticles on the induction of Parkinson's disease, and their mechanisms of action.
The first causative gene identified in familial Parkinson's disease was α-Syn. The Lewy body, a characteristic neuropathological change in Parkinson's disease, mainly comprises α-Syn protein (CitationChartier-Harlin et al. 2004). CitationUversky (2007) verified that α-Syn aggregation played a key role in the pathogenesis and progression of Parkinson's disease. Meanwhile, conformational change of α-Syn can induce neuronal apoptosis. Oxidative stress, as one of the essential pathways to initiate and to promote α-Syn misfolding as well as aggregation (CitationAuluck et al. 2010), plays a crucial role in nanomaterial-induced biological toxicity (CitationNel et al. 2006, CitationPan et al. 2009, CitationLi et al. 2010). Our recent study demonstrated that TiO2-NPs break the dynamic equilibrium between reactive oxygen species (ROS) and anti-oxidative enzyme activity in dopaminergic neurons, attack cell membranes, and induce neuronal apoptosis and cell cycle change (CitationWu et al. 2010). Based on these theories and literatures, we hypothesized that nanoparticles could induce α-Syn protein aggregation through oxidative stress, thus causing damage to dopaminergic neurons and subsequently leading to Parkinson's disease.
The ubiquitin-proteasome system (UPS) is an essential protein degradation pathway in eukaryotic cells, the aberrant function of which might be associated with impaired α-Syn degradation and the onset of Parkinson's disease (CitationEbrahimi-Fakhari et al. 2011). Parkin and ubiquitin C-terminal hydrolase protein (UCH-L1) are key enzymes in UPS, and abnormal UPS functions cause protein accumulation in the brain. For example, disrupted degradation of α-Syn by 26S protease leads to neurotoxicity (CitationPetrucelli and Dawson 2004). However, whether nanoparticles affect the protein degradation function of UPS, and then induce aberrant α-Syn aggregation, remains unknown.
TiO2-NPs have been widely used in cosmetics, antiseptic agents, coating flux, water treatment chemicals, photocatalysts, etc. In this study, we established a co-culture model of TiO2-NPs and dopaminergic neurons, to investigate the effects of TiO2-NPs on α-Syn aggregation, and their mechanism of action, unveiling the role of UPS in α-Syn protein degradation. The results provide significant implications for avoiding risks associated with nanoparticles, improving public health, and promoting the development of nanotechnology.
Experimental
Reagents and equipments
TiO2-NPs (anatase, < 25 nm) and penicillin-streptomycin were purchased from Sigma (USA). DMEM, FBS and trypsin were bought from Gibco-Invitrogen (UK). N-acetyl cysteine (NAC) and RIPA cell lysis solution were obtained from Beyotime Institute of Biotechnology. The BCA protein assay kit was purchased from Pierce (USA). The primary antibody for α-Syn and DyLight 488 conjugated goat anti-rabbit antibody were from Abcam (UK). Primary antibodies against parkin, UCH-L1, β-actin and HRP-labeled secondary antibody against rabbit, were bought from Santa Cruz (USA). The ultrasonic vibrator (HK2200) was obtained from Shanghai Kedao Ultrasonic Instrument Co., Ltd. The Ultraclean hood was from Suzhou Antai Airtech Co., Ltd. The CO2 incubator was purchased from Thermo Fisher (Germany). The inverted phase contrast microscope was bought from Olympus (Japan). The SDS-PAGE electrophoresis and membrane transfer apparatus were obtained from Bio-Rad (USA).
Characterization of TiO2-NPs
The particle size and morphology were determined by transmission electron microscopy (TEM). A nanoparticle suspension (1 mg/ml) was prepared by mixing TiO2-NPs, 5% horse serum, 10% serum, and DMEM medium, and sonicated for 30 min at 600 W, 20 kHz. Particle size and zeta potential were measured by the Zetasizer particle size analyzer and potentiometric analyzer, respectively.
Cell culture
PC12, a cell line derived from a pheochromocytoma of the rat adrenal medulla, was purchased from the cell bank of Shanghai Biological Institute. The TPC12 cells were seeded in DMEM medium (5% horse serum, 10% fetal cow serum, 100 U/ml penicillin, and 100 mg/ml streptomycin), and cultured in a 5% CO2 incubator at 37°C, and the culture medium was changed every other day. When reaching 80% confluence, the PC12 cells were subcultured.
Nerve growth factor (NGF) induction: NGF promotes the differentiation of PC12 cells into sympathetic neuron-like cells. Eventually, PC12 cells resemble neurons in physiological and biochemical properties. At 24 h post-subculture, the culture medium was changed into DMEM containing NGF (50 ng/ml NGF, 10% fetal cow serum, 100 U/ml penicillin, and 100 mg/ml streptomycin). The cells were continuously cultured for 7 days, and the culture medium was changed every other day. The cells were observed under an inverted microscope daily.
Co-culture of TiO2-NPs and PC12 cells
After autoclaving (120°C, 2 h), the TiO2-NPs were diluted to 50, 100 and 200 μg/ml with DMEM, and then sonicated for 30 min at 600 W, 20 kHz. The negative control was depleted of nanoparticles. In addition, to explore the role of oxidative stress, we investigated the effect of a 200 μg/mL dose of TiO2-NPs on α-Syn expression in PC12 cells, with or without 20 mM NAC.
Western blotting
After co-culture with different concentrations of TiO2-NPs for 24 h, PC12 cells were digested with 0.25% trypsin, and centrifuged at 1500 rpm for 5 min. After the supernatant was discarded, RIPA cell lysis solution was added to lyse the cells on ice for 30 min. The cell lysates were centrifuged at 12000 rpm, at 4°C for 15 min, and the supernatant was collected and stored at − 80°C. We used the BCA method to measure the protein concentration. After isolation with SDS-PAGE electrophoresis, 30–40 μg of protein was transferred to a PVDF membrane, which was blocked in 5% skimmed milk for 1 h and incubated with α-Syn, parkin, UCH-L1 and β-actin antibodies (prepared in 5% skimmed milk and 1 × TBST) at 4°C, overnight. The membranes were washed 3 times with TBST, and then incubated with the secondary antibody for 1 h at room temperature. Finally, the PVDF membranes were visualized by X-ray.
Immunofluorescent staining
The PC12 cells were collected and suspended into solutions with appropriate concentrations. The cell solutions were mounted onto lysine-coated slides, and allowed to stand for 10 min. The slides were fixed in 4% paraformaldehyde, then washed and incubated in 0.3% Triton X-100/PBS, for 20 min at room temperature. After blocking with 3% BSA for 20 min, the slides were incubated with rabbit anti-rat α-Syn (1:500) at 4°C, overnight. PBS was taken as the negative control. The slides were washed and incubated with DyLight 488 labeled goat anti-rabbit antibody (1:100) at 37°C for 1 h, cover-slipped with 50% glycerol, and checked under a fluorescent microscope.
Data analysis
Data were presented as mean ± SD. Statistical analysis was performed by SPSS 12.0, with one-way ANOVA comparing the differences between groups. A value of p < 0.05 was considered statistically significant.
Results
Morphology and particle size of TiO2-NPs
The TEM images showed the TiO2-NPs as spherical, even-sized particles with an average diameter of ∼ 20 nm (). These nanoparticles were well suspended, without obvious aggregation. In the DMEM medium, theTiO2-NPs were negatively charged, showing apparent aggregation and diverse particle sizes ().
Table I. Average aggregation size and zeta potential in a solution of TiO2–NPs.
TiO2-NP-induced α-Syn expression in PC12 cells
The results of western blotting showed that after treatment with different doses of TiO2-NPs for 24 h, the cytoplasmic level of α-Syn expression was significantly increased (p < 0.05) in a dose-dependent manner ( and ). Pretreatment with NAC partially inhibited the α-Syn expression induced by 200 μg/ml TiO2-NPs ().
Figure 2. The result of western blotting, showing the effect of TiO2-NPs on α-Syn expression in PC12 cells.
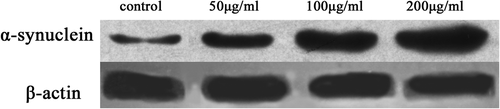
Figure 3. The result of western blotting, showing the α-Syn expression in PC12 cells after treatment with TiO2-NPs and NAC.
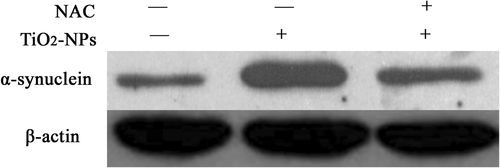
Table II. The result of western blotting, showing the effect of TiO2-NPs on α-Syn in PC12 cells (grey value, %).
TiO2-NP-induced α-Syn aggregation in PC12 cells
The results of immunofluorescent staining showed that more α-Syn was subjected to aggregation in PC12 cells after treatment with TiO2-NPs for 24 h (). Moreover, this effect was dose-dependent.
Effect of TiO2-NPs on expression of UCH-L1 and parkin in PC12 cells
After treatment with TiO2-NPs, the expressions of UCH-L1 and parkin were decreased dose-dependently, compared with those of the control ( and ).
Figure 5. The result of western blotting, showing the expression of parkin and UCH-L1 after treatment with TiO2-NPs.
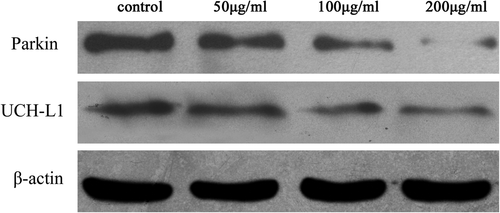
Table III. Parkin and UCH-L1 expression levels after TiO2-NPs treatment in PC12 cells (grey value, %).
Discussion
Parkinson's disease is a neurodegenerative disease, caused by the loss of dopaminergic neurons in the substantia nigra, accompanied by fibrillar inclusion and Lewy bodies in remnant neurons and other brainstem nuclei as the main pathological changes. The aggregation of α-Syn plays an important role in the formation of Lewy bodies (CitationTrojanowski and Li 1998). In this study, we used different doses of TiO2-NPs (50, 100 and 200 μg/ml) to treat dopaminergic neurons (PC12 cells) for 24 h, and then detected α-Syn expression and aggregation, by western blotting and immunofluorescent staining, respectively. The level of expression of α-Syn was related to the concentration of TiO2-NPs, in a dose-dependent manner. With increasing TiO2-NPs, more α-Syn was expressed, accompanied by cytoplasmic α-Syn aggregation. Hence, TiO2-NPs can induce α-Syn aggregation in neurons, and neurotoxicity thereafter.
Oxidative stress predominantly controls the initiation/progression of α-Syn misfolding and aggregation (CitationAuluck et al. 2010), and in neurons, it allows the nitration modification of α-Syn and further induces its aggregation (CitationReynolds et al. 2008). Besides, oxidative stress promotes the generation of an active dopamine metabolite, dopamine quinine, which can covalently bind α-Syn, stabilize its primary fibrillar structure, and inhibit fibrillar aggregation, thus enhancing the neurotoxic effects induced by TiO2-NPs (CitationHastings 2009). Meanwhile, nanomaterial-induced biological toxicity is mainly attributed to oxidative stress (CitationNel et al. 2006, CitationPan et al. 2009, CitationLi et al. 2010). Moreover, treatment with TiO2-NPs can increase the amount of ROS in PC12 cells, and induce cell apoptosis through mitochondria (CitationNel et al. 2006, CitationPan et al. 2009, CitationLi et al. 2010), implying that oxidative stress is involved in TiO2-NP-induced neurotoxicity (CitationWu et al. 2010). In this study, after pretreatment with the anti-oxidant NAC, α-Syn was significantly reduced in PC12 cells, indicating that oxidative stress was able to mediate theTiO2-NP-induced α-Syn expression and aggregation.
UPS is an essential cellular protein degradation pathway responsible for clearing and degrading misfolded, mutated and oxidative stress-damaged proteins. Aberrant UPS function might be closely associated with neurodegenerative diseases, such as Parkinson's disease and Alzheimer's disease (CitationEbrahimi-Fakhari et al. 2011). The main component of Lewy bodies is the α-Syn aggregate, in addition to ubiquitin, protease subunit, molecular chaperone, neurofilament, and ubiquitinated proteins. Thus, disruption of UPS function might be involved in Lewy body formation. In UPS, proteins are target-degraded, mainly by the 26S proteasome, which is an ATP-dependent process (CitationShang and Taylor 2011). TiO2-NPs may induce mitochondrial damage and dysfunction, reduce ATP synthesis, and increase production of ROS, thus disturbing the normal function of hydrolase in UPS (CitationWu et al. 2010). Hence, it is of great significance to analyze whether TiO2-NP-induced α-Syn aggregation in PC12 cells is associated with UPS dysfunction.
Parkin encodes an important E3 ligase in UPS, the mutation of which affects the ubiquitination of its substrate, impairs protein degradation, and causes α-Syn aggregation in neurons (CitationRakovic et al. 2011). UCH-L1 is one of the most abundant proteins in the brain, accounting for about 2% of all soluble proteins. It is also an essential UPS enzyme, mainly hydrolyzing the C-terminal of ubiquitinated protein and recycling the ubiquitin. Point mutation of UCH-L1 causes familial Parkinson's disease and α-Syn aggregation (CitationEsteve-Rudd et al. 2010). In this study, after 24 h of treatment with TiO2-NPs, the levels of expression of both parkin and UCH-L1 decreased, as evidenced by western blotting. The results implied that TiO2-NPs caused damages to the UPS and protein degradation pathway in the PC12 cells, thus inducing cytoplasmic α-Syn increase and aberrant aggregation.
Conclusions
In summary, treatment with TiO2-NPs induces the expression and aggregation of α-Syn, a causative gene for Parkinson's disease, in PC12 cells. This process is associated with oxidative stress. Meanwhile, UPS dysfunction might promote α-Syn aggregation. The findings suggest that exposure to TiO2-NPs is potentially neurotoxic, which may result in Parkinson's disease.
Declaration of interest
The authors report no declarations of interest. The authors alone are responsible for the content and writing of the paper.
This study was supported by grants from the Natural Science Foundation of China (No. 31200756) and Science and Technology Research Program for Colleges and Universities in Shandong Province, China (No. J12LK54).
References
- Auluck PK, Caraveo G, Lindquist S. 2010. α-Synuclein: membrane interactions and toxicity in Parkinson's disease. Annu Rev Cell Dev Biol. 26:211–233.
- Chartier-Harlin MC, Kachergus J, Roumier C, Mouroux V, Douay X, Lincoln S, et al. 2004. α-Synuclein locus duplication as a cause of familial Parkinson's disease. Lancet. 364:1167–1169.
- Ebrahimi-Fakhari D, Cantuti-Castelvetri I, Fan Z, Rockenstein E, Masliah E, Hyman BT, et al. 2011. Distinct roles in vivo for the ubiquitin–proteasome system and the autophagy–lysosomal pathway in the degradation of α-synuclein. J Neurosci. 31: 14508–14520.
- Elder A, Gelein R, Silva V, Feikert T, Opanashuk L, Carter J, et al. 2006. Translocation of inhaled ultrafine manganese oxide particles to the central nervous system. Environ Health Perspect. 114: 1172–1178.
- Esteve-Rudd, J, Campello, L, Herrero, MT, Cuenca, N, & Martín-Nieto, J. 2010. Expression in the mammalian retina of parkin and UCH-L1, two components of the ubiquitin-proteasome system. Brain Res. 1352:70–82.
- Hastings TG. 2009. The role of dopamine oxidation in mitochondrial dysfunction: implications for Parkinson's disease. J Bioenerg Biomembr. 41:469–472.
- Li JJ, Hartono D, Ong CN, Bay BH, Yung LY. 2010. Autophagy and oxidative stress associated with gold nanoparticles. Biomaterials. 31:5996–6003.
- Nel A, Xia T, Mädler L, Li N. 2006. Toxic potential of materials at the nanolevel. Science. 311:622–627.
- Pan Y, Leifert A, Ruau D, Neuss S, Bornemann J, Schmid G, et al. 2009. Gold nanoparticles of diameter 1.4 nm trigger necrosis by oxidative stress and mitochondrial damage. Small. 5:2067–2076.
- Petrucelli L, Dawson TM. 2004. Mechanism of neurodegenerative disease: role of the ubiquitin proteasome system. Ann Med. 36:315–320.
- Pisanic TR II, Blackwell JD, Shubayev VI, Fiñones RR, Jin S. 2007. Nanotoxicity of iron oxide nanoparticle internalization in growing neurons. Biomaterials. 28:2572–2581.
- Rakovic A, Grünewald A, Kottwitz J, Brüggemann N, Pramstaller PP, Lohmann K, Klein C. 2011. Mutations in PINK1 and Parkin impair ubiquitination of Mitofusins in human fibroblasts. PLoS One. 6:e16746.
- Reynolds AD, Glanzer JG, Kadiu I, Ricardo-Dukelow M, Chaudhuri A, et al. 2008. Nitrated alpha‐synuclein‐activated microglial profiling for Parkinson's disease. J Neurochem. 104: 1504–1525.
- Shang F, Taylor A. 2011. Ubiquitin–proteasome pathway and cellular responses to oxidative stress. Free Radic Biol Med. 51: 5–16.
- Trojanowski JQ, Lee VM. 1998. Aggregation of neurofilament and α-synuclein proteins in Lewy bodies: implications for the pathogenesis of Parkinson disease and Lewy body dementia. Arch Neurol. 55:151–152.
- Uversky VN. 2007. α-Synuclein Aggregation and Parkinson's Disease. Protein Misfolding, Aggregation, and Conformational Diseases. Springer US, 61–110.
- Wu J, Sun J, Xue Y. 2010. Involvement of JNK and P53 activation in G2/M cell cycle arrest and apoptosis induced by titanium dioxide nanoparticles in neuron cells. Toxicol Lett. 199:269–276.
- Wu J, Wang C, Sun J, Xue Y. 2011. Neurotoxicity of silica nanoparticles: brain localization and dopaminergic neurons damage pathways. ACS Nano. 5:4476–4489.