Abstract
New and reemerging infectious diseases call for innovative and efficient control strategies of which fast vaccine design and development represent an important element. In emergency situations, when time is limited, identification and use of correlates of protection (COPs) may play a key role as a strategic tool for accelerated vaccine design, testing, and licensure. We propose that general rules for COP-based vaccine design can be extracted from the existing knowledge of protective immune responses against a large spectrum of relevant viral and bacterial pathogens. Herein, we focus on the applicability of this approach by reviewing the established and up-coming COPs for influenza in the context of traditional and a wide array of new vaccine concepts. The lessons learnt from this field may be applied more generally to COP-based accelerated vaccine design for emerging infections.
Introduction
At the global level several factors contribute to a significantly increased risk of appearance and spreading of new and re-emerging infectious diseases. Global warming influences the geographical distribution of important vector organisms for several dangerous pathogensCitation1,Citation2 and the increase in worldwide travel and mobility is an effective driving force for the spread of infectious diseases. The unexpected appearance of Severe Acute Respiratory Syndrome (SARS) in 2002Citation3 and the related Middle East Respiratory Syndrome (MERS) in 2013,Citation4 the pandemic H1N1 influenza virus in 2009Citation5 and the continued threat of H5N1 and H7N9 bird flu,Citation6,Citation7 serve as examples of emerging and re-emerging diseases, that require swift control measures.
The potential threat from emerging and dangerous infections calls for innovative and efficient control strategies in order to increase the general preparedness level. When facing an emergency situation with a new pathogen, time is limited and efforts to accelerate the otherwise time-consuming vaccine development process at all stages are critical. The use of correlates of protection (COPs) may play a key role in speeding up vaccine development from design to licensure in various ways. In this context we define COPs as immune responses that directly can be linked to protection in a causal way, whereas surrogates or co-correlates are understood as responses co-occurring with protection but not necessarily representing a causal mechanism.
In the design phase, vaccine composition and delivery systems may be selected to elicit known or presumed COPs. In the clinical phase, vaccine efficacy measurements performed by monitoring early biomarkers in validated assays instead of measuring protection against clinical disease may provide a faster, but still reliable, outcome. COPs may also be used to bridge preclinical protection studies performed in animal models with clinical studies, or connect early with late phase trials, supporting a faster and more evidence-based progress toward licensure. If it is not feasible to assess clinical efficacy, the size and duration of phase 3 trials can be reduced by using COPs to predict vaccine efficacy.
To be able to apply COP-based vaccine design to new and re-emerging infections for which COPs have not yet been discovered, the establishment of general predictive rules based on the most important classes of pathogens is needed. This can be achieved by systematic extraction of the existing knowledge of protective immune responses against a large spectrum of relevant bacteria and viruses combined with knowledge of the immune mechanisms induced by different vaccine concepts. To demonstrate the applicability of this principle, we have reviewed the current literature on COPs for an infectious disease for which traditional vaccine platforms are being constantly improved and multiple novel vaccine concepts have reached clinical trials: influenza. The 2009 influenza pandemic reminded us that several general aspects of vaccine development are important to improve pandemic preparedness. Among the most important are the needs for universal vaccines comprising conserved antibody and T-cell targets to induce cross-reactive immunity in all age groups, improved adjuvants, and vaccine production platforms which allow fast manufacture. Recently proposed novel COPs for influenza are mostly based on clinical data with sufficient power to support prediction of protection. Importantly, the development of these COPs which goes far beyond the traditional hemagglutination inhibition (HI) assay paves the way for the rational design and faster evaluation of new and promising vaccine concepts. We suggest that the recently obtained knowledge in this field, as summarized herein, is not only important for improved vaccination against influenza, but due to its unique size and scope can also be generalized and applied to accelerate vaccine design and development for a wider range of new and reemerging infections.
Classical Influenza Vaccines and Traditional COPs
Influenza viruses are enveloped viruses that contain a segmented genome of 8 different negative sense single-stranded RNA molecules. The envelope and its 3 integral membrane proteins hemagglutinin (HA), neuraminidase (NA) and the ion-channel M2 overlay a matrix protein (M1), which encloses the virion core. In this core the ribonucleoprotein (RNP) complex, which consists of the viral RNA segments coated with nucleoprotein (NP) and the RNA-dependent RNA polymerases (PB1, PB2, and PA) are found ().
Figure 1. Nomenclature and localization of the major external and internal protein antigens of the influenza virus.
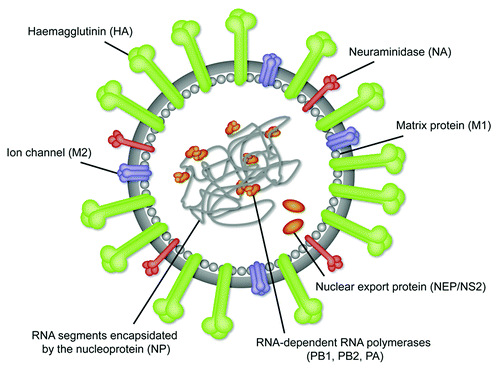
Seasonal influenza is dominated by A and B viruses. Influenza A viruses are divided into subtypes according to the combination of different HA and NA antigens occurring at the surface. Among many possible subtypes of influenza A viruses (17 HA and 9 NA subtypes), H1N1 and H3N2 are the most predominant subtypes currently circulating among humans. Seasonal influenza is associated with the annually occurring sequence variation in HA and NA (antigenic drift), whereas influenza pandemics usually occur as a result of new combinations of HA and NA subtypes (antigenic shift). Due to the continuous antigenic drift of HA (and NA), the composition of virus-derived vaccines against seasonal influenza must be updated on an annual basis to achieve the necessary matching between HA antibody specificity and the prevalent circulating influenza strains.
Two main types of vaccines for seasonal influenza are currently licensed: inactivated influenza vaccines (IIV) mostly for intramuscular administration, and live attenuated influenza vaccines (LAIV) for intranasal delivery. Both vaccine types are usually trivalent comprising 2 A subtypes (currently H1N1 and H3N2) and a single B component, yet quadrivalent vaccines containing an additional B component now also emerge on the market (). Inactivated vaccines may contain whole inactivated virus particles (WIV vaccines), virus disrupted by detergents or solvents (split vaccines) or purified HA and NA (subunit vaccines).
Table 1. Approved seasonal and (pre)pandemic influenza vaccines
Split and subunit vaccines are today the most frequently used products and represent the conventional vaccine concepts for seasonal vaccination. LAIV most often consist of cold adapted and temperature sensitive viral strains, and have been licensed both in US (2003) and Europe (2011). There are indications that LAIV may be more efficacious in young individuals (6 mo to 18 y), whereas no apparent difference in efficacy of LAIV and IIV has been demonstrated in adults (reviewed inCitation8).
While most IIV are non-adjuvanted, a few have been optimized by improved formulations to generate better protection both among the elderlyCitation9 and young children,Citation10 who typically respond poorly to vaccination. Manufacturing of classical influenza vaccines may further vary with respect to virus propagation procedures and inactivation methods. For (pre)pandemic purposes, several IIV and LAIV vaccines have been licensed, some of which are cell based and/or adjuvanted, and widely used in various age groups during the 2009 influenza pandemic. Recently, as a first non-classical influenza vaccine, a recombinant baculovirus expressed trivalent HA0 based vaccine (FluBlok®) was introduced on the market, a technological advance which may speed up mass production of vaccines for novel viral strainsCitation11 ().
Minimal requirements for traditional seasonal influenza vaccines
While annual updating of an already licensed vaccine in many cases does not include immunogenicity testing, introduction of a new seasonal influenza vaccine requires a licensing application which should include satisfactory evidence of immunogenicity and safety as laid down by the regulatory authorities (EMA in Europe and FDA in USA). The humoral responses induced in the required trials are currently evaluated with the traditional “gold standard” COP for influenza: titer of antibodies inhibitory for the major surface antigen hemagglutinin (HA).Citation12 HA is the viral receptor-binding protein, and antibodies that are directed to epitopes located within or in close proximity to the receptor-binding site, can prevent binding of the virus to its receptor on the host cells. It is well documented that the induction of HA specific antibodies is an important COP against infection, provided that these antibodies have the correct strain-specificity.Citation13 Therefore, the induction of HA-antibodies that block receptor-binding is used as a correlate of vaccine efficacy and a current criterion for regulatory approval. Pre- and post-vaccination sera obtained in the registration trials from subjects of different age groups are tested, mostly in the hemagglutination inhibition (HI) assay. In this assay, inhibition of virus-induced agglutination of red blood cells by HA antibodies in serum samples can be measured as the HI titer. Based on studies with seasonal influenza A strains in adults, an HI titer ≥ 40 has been defined as an immunologic correlate corresponding to a 50% reduction of risk of contracting influenza.Citation12,Citation13 For a seasonal vaccine to be registered it must meet, for each tested age group and all viral strains, at least one of 3 defined criteria for immunogenicity as evaluated by the HI assay: the sero-protection rate, the sero-conversion rate, and the mean HI titer increase. Notably, for registration of pandemic vaccines all 3 criteria must be fulfilled.
Limitations of using HI as COP for evaluation of influenza vaccines
Although an HI titer ≥ 40 to a specific influenza strain remains indicative of protection, evidence is accumulating that the relevance of the HI assay may differ substantially between different vaccines and populations tested. First, in the human challenge model it has proved difficult to infect individuals with HI titers as low as ≥8,Citation14 and LAIV show protective efficacy independently of HI titers. Being administered intranasally, LAIV will prime local mucosal immune responses and secretory IgA, not measured in the HI assay, but most likely contributing to protection.Citation15 Second, poor sensitivity of the HI assay has been found for neutralizing antibodies that recognize the conserved HA stalk region, as well as for antibodies specific for avian A virus subtypes H5 and H7, and for B strains, warranting technical modifications.Citation16,Citation17 Third, the use of the adult correlate of protection (HI titer ≥ 40) may not be appropriate when evaluating vaccination of children. As reported by Black et al. a cutoff of 110 was required to predict the conventional 50% clinical protection rate, and to predict an 80% protective level, which would seem more desirable from a public health perspective, a titer of 330 was required.Citation18 This discrepancy may in part relate to the ability of the HI assay to measure both IgM and IgG antibodies, which may lead to an ‘overrating’ of the immune response in naïve children,Citation19 and measuring IgG only may therefore correlate better with protection in pediatric trials. On the other hand, alternative immunological endpoints, such as antibody responses to other viral proteins or cell mediated immune parameters, might better reflect vaccine efficacy in children.Citation19-Citation21 In fact, vaccine-induced T-cell responses have been suggested as a better COP than antibody responses not only in young children,Citation22 but also in adults over 65 y of age.Citation23 The primary goal of vaccination, specifically in the elderly, is to provide protection against clinical disease, rather than sterilizing immunity. Thus, enhanced vaccine-mediated protection against influenza illness in older individuals should probably be assessed by changes in cellular immunity, in addition to standard HI measurements.Citation24
These and other studies have increased the awareness among scientists, vaccine manufacturers and regulatory authorities that new COPs are needed, not only for improved evaluation of traditional influenza vaccines in different target groups, but also for the development of new vaccine concepts.Citation19-Citation21,Citation25,Citation26 Various new seasonal and (pre)pandemic vaccine approaches use novel production- or formulation platforms that do focus on inducing strong(er) HI titers to the immuno-dominant globular head(s) of HA subtypes,Citation27-Citation29 such as FluBlok®.Citation11 For other new vaccine concepts in clinical development, the traditional HI titer may not serve as a relevant COP at all. Dependent on the antigens targeted, such as conserved domains of HA, NA, M2e, or universal T-cell antigens (like NP, PA, PB, or M1), and dependent on their formulation or expression platform,Citation27-Citation39 other COPs should be addressed. These include broadly virus neutralizing activity, antibody dependent cellular cytotoxicity (ADCC), cross-reactive T cell responses, or mucosal secretory IgA (sIgA). As is the case for serological assays,Citation40,Citation41 the application of any cellular biomarkers of protection will require standardized and harmonized assays with inactivated or low pathogenicity viral strains and criteria to compare results between laboratories. Although a wide range of potential COP assays covering both antibody- and cell-mediated immune responses are in the pipeline, they still need further evaluation for clinical performance and methodological validation.
Concomitantly, a diverse landscape of upcoming influenza vaccine approaches has boosted the exploration of new immunological end-points to be used for assessing vaccine immunogenicity and effectiveness of immune responses. In a broader context, this development is not only important for influenza, but the lessons learnt from this field may also pave the way for rational development of vaccines for other diseases based on the same principles. Thus, the putative trendsetting role of influenza can in this regard be attributed to both development of principally new vaccine concepts and a substantial broadening of the repertoire of COPs necessary for efficient evaluation of novel vaccines in general.
Influenza Vaccine Concepts Requiring New COPs
A variety of innovative influenza vaccine approaches in clinical development, that are not solely based on HI assays, broadly target one or more of the following principles of protection: (1) induction of more broadly cross-reactive serum antibodies, (2) cross-reactive cellular immunity, and/or (3) mucosal immune responses (). Potential new influenza COPs relevant for these different vaccine platforms have been identified from preclinical and clinical studies, as reviewed from various perspectives.Citation20,Citation21,Citation25,Citation42,Citation43 Although some immunological biomarkers only can be considered as co-correlates or surrogates, and not true COPs, they may still play a role in evaluating vaccine candidates.Citation44 In children, serum HI titer and nasal IgACitation45 have been described as co-correlates. In addition, non-neutralizing antibody- and T-cell responses have also been reported as co-correlates of protection.Citation46 Nevertheless, despite the complexity of the protective immune response, a good understanding of the underlying mechanisms is fundamental to accelerate the development of any novel influenza vaccine concepts. Importantly, when applied in a broader context of vaccinology, this knowledge may also play an important role in rational development of vaccines against other diseases.
Table 2. Novel influenza vaccine approaches in clinical development categorized according to the primary mode of protection intended to be induced, vaccine type, and antigen targets
Broadly cross-reactive antibody responses to envelope structures as COPs
Antibody responses elicited toward HA primarily bind to the hyper-variable globular head region and only recognize homologous strains within a given virus subtype. This is why the efficacy of conventional seasonal influenza vaccines is mostly limited to circulating strains with a close antigenic match. More broadly reactive antibody responses seem to be directed at less accessible epitopes spanning the interface of properly folded HA trimers, or at the more membrane proximal stalk region of the HA molecule. This stalk region of HA is relatively conserved and is involved in fusion with the endosomal membrane of the host cell. Broadly neutralizing antibodies that bind to this region have been shown to inhibit infection with influenza ACitation47-Citation49 and B strains.Citation50 To target these less variable HA regions more efficiently than conventional virus derived vaccines, several expression platforms delivering full-length HA have entered clinical development. These include recombinant and fusion-proteins, particles (inactivated virions, virosomes, VLPs, BLPs), replicon systems, and DNA vaccines (). Recently, a phase I trial of an intramuscularly given H5 DNA prime, split vaccine boost regimen provided evidence for the induction of anti-stalk antibodies in humans.Citation51 New approaches only targeting the stalk region showed feasibility in preclinical studies, but these concepts have not yet entered clinical development.Citation52-Citation54 Serological assays, such as ELISA and a pseudotype neutralization assayCitation49,Citation55 may be used to assess the induction of HA stalk specific antibodies ().
Table 3. Established and emerging COPs for influenza vaccine development
The other major viral envelope protein and component in various novel influenza vaccine approaches is NA. Its function as a sialic acid cleaving enzyme is crucial for efficient release of virions from infected cells, acting in the late stage of the virus replication cycle. Antibodies directed against the enzymatic site can block its function and serum anti-NA antibodies have been associated with resistance to clinical disease.Citation56,Citation57 Hence, unlike antibodies against HA which can prevent infection, NA-specific antibodies limit the release and further spread of viral particles, and thereby mainly prevent serious disease and fatal outcome. Although NA antibodies are considered mostly as subtype specific, they also have cross-reactive potential.Citation58,Citation59 Clinically tested vaccine approaches including NA involve DNA based vaccinesCitation30 and particulate forms, such as virus like particles (VLP)Citation30,Citation60 (). Notably, these platforms can also induce various forms of cellular immunity. The induction of serum NA inhibiting (NI) antibodies is suggested to be an independent predictor of immunity to naturally occurring influenza in the presence of HI antibodies.Citation61 Various non-standardized methods are available to assess anti-NA antibody responses,Citation62-Citation65 including assays that minimize steric hindrance by anti-HA antibodies.Citation57,Citation66,Citation67
The M2 protein is a tetrameric membrane protein that forms an ion channel and plays an important role in the un-coating of the viral genome during the early steps of the virus replication cycle. M2e (the extra cellular domain of M2) is highly conserved in all influenza A subtypes. M2-specific antibodies tend to recognize virus particles poorly, do not neutralize the virus, and antisera against virus contain few M2-specific antibodies. However, M2e is highly expressed in infected cells, and anti-M2e antibodies binding to these cells can induce antibody dependent NK cell mediated killing (ADCC), complement-mediated lysis, or phagocytosis.Citation68,Citation69 As the M2-protein is conserved across influenza A strains, it has been regarded as a basis for the development of universal vaccines.Citation34,Citation70 Despite the fact that neither M2e sero-prevalence after recurrent infections with circulating influenza strains nor vaccination with LAIV or WIV vaccines indicate a strong immunogenicity profile, this vaccine concept is still pushed in the clinical pipelineCitation38,Citation48,Citation71,Citation72 (). Assays to evaluate M2e antibody responsiveness may be based on linear peptides or conformational tetrameric forms of the M2e antigen.Citation73
Besides the traditional HI assay, several other antibody-based assays, as mentioned here, have been demonstrated to play an important role in evaluating new vaccine concepts in the development process (). However, any general assessment of their relative strength and value as potential COPs in clinical trials is complicated, due to the fact that their relevance will be dependent of several factors: vaccine concept to be tested, immune responses to be targeted, and even the population to be vaccinated. Thus, the broad repertoire of COP related assays now available in the influenza field, including both humoral and cellular immune responses, may serve as a necessary menu from which the relevant immune assays should be chosen.
Cross-reactive T cell responses as COPs
In contrast to neutralizing antibodies directed mainly against the highly variable surface antigens (HA and NA) of the influenza virus, cellular immune responses mediated by T cells are mainly directed against epitopes of internal and highly conserved antigens (M, NP, PA, PB). Antibody-mediated immunity mainly acts to prevent viral entry into and release from cells, while T-cell mediated immunity plays an important role in clearance of established infection and reduction of disease severity and mortality. Although the complementary protective role of T-cells has been known for a long time,Citation74 it has recently been highlighted by the demonstration of pre-existing T-cell immunity against the 2009 H1N1 pandemic strain, leading to protection against severe disease in the absence of specific antibody responses.Citation75
Cellular effector functions related to protection can be mediated by 2 conventional T cell subsets. Cytotoxic CD8+ T cells recognize peptide epitopes generated from cytosolic viral proteins by proteasomal degradation and presented by MHC class I molecules on the surface of infected cells. Upon activation, these T cells secrete antiviral cytokines and release perforin and granzymes, of which the latter initiate apoptosis of the infected target cell. In a prospective study low granzyme B levels correlated with the risk of developing influenza disease in older adults.Citation76 More recently levels of pre-existing IFNγ+IL2- CD8+ T cells were shown to correlate with low total symptom score during the 2009 pH1N1 pandemic.Citation77 Importantly, granzyme B levels were also shown to correlate with protection and enhanced cytotoxic activity after influenza vaccination.Citation23,Citation78-Citation80 CD4+ T cells, on the other hand, recognize viral epitopes that have been processed in the endo-lysosomal pathway of antigen presenting cells and presented by MHC class II molecules. These cells act not only by orchestrating the responsiveness of CD8+ T cells and B cells by secreting Th1 (IFNγ, TNFα), Th2 (IL-4, IL5, IL13), Th17 (IL-17), Tfh (IL21), or regulatory (IL-10, TGF-β) cytokines,Citation81 but also by directly killing infected cells.Citation82 Pre-existing influenza-specific CD4+ T cells were recently found to correlate with decreased viral shedding and reduced severity of illness following experimental influenza infection of humans.Citation83 In addition, a decline in the INF-γ/IL-10 ratio produced by PBMC stimulated in vitro with influenza virus was shown to be associated with increased risk for influenza disease in the elderly.Citation23 Induction of CD4+ T cell immunity may correlate with the serological response to influenza vaccination: the CD4+ Th1 response after primary H5N1 vaccination predicted protective antibody levels after booster doses,Citation84,Citation85 and frequencies of IFN-γ and IL-17 producing CD4+ T cells correlate with HI responses in younger adults after seasonal vaccination.Citation86 While influenza specificT cell responses seem particularly relevant as COPsCitation22,Citation23,Citation78 and indeed can contribute to cross-protective immunity,Citation87 tight regulation is needed to avoid immuno-pathological effects after vaccination as described for natural infection.Citation88-Citation90
Of the most widely used seasonal influenza vaccines, split and subunit vaccines elicit CD4+ T cells specific for epitopes from HA and NA subtypes, while LAIV can also induce CD4+ and CD8+ T cell responses to epitopes from internal proteins. Multiple vaccine candidates using liver vectors or particulate formulations are in clinical development specifically designed to induce cross-reactive T cell immunity against internal antigens or their epitopesCitation91-Citation96 (). For these and several other novel platforms that deliver viral antigens into the MHC class I or II processing pathway, either as recombinant live attenuated virus, recombinant synthetic polypeptide, DNA, α-virus, or as formulated particles (), standardized CD8+ and CD4+ T cell assays are needed to assess vaccine effectiveness and to compare results between different laboratories ().
Granzyme B is a key mediator of cytolytic activity of CD8+ T cells and an assay based on its enzymatic activity (serine protease) has been developedCitation79 and recently standardized and validated by several laboratories.Citation97 This assay warrants application to evaluating protective T-cell immunity in clinical trials. To probe the different functional cytokines released by CD4+ T-helper cells that support the induction of cellular immune responses (INF-γ, TNF-α, and IL2), promote antibody responses (IL4, IL5, IL13), or play a regulatory role (TGF-β, IL10), various assays are available. Multicolor flow cytometry has the advantage of combining the characterization of cell surface markers and intracellular production of multiple cytokines at the single cell level. Based on this approach various subsets of multifunctional T cells have recently been proposed as predictors of protection.Citation77,Citation81,Citation83 Besides standardized ELISA assays, it is also possible to measure a wide range of secreted Th1 and Th2 cytokines in multiplexed assays following standardized and validated formats.Citation97 Based on the established correlation between the INF-γ /IL10 balance and protection against disease,Citation23 such assays should represent efficient tools for evaluating vaccines in combination with assays for cytotoxic effector functions (granzyme B assay). The relevance of INF-γ has been further supported by the finding that the frequency of virus specific INF-γ secreting cells could be correlated with protection against disease in a vaccination study of children by using an ELISPOT assay.Citation22
The relative strength of the T cell based COPs discussed here is difficult to evaluate, since they are proposed on the basis of clinical studies with different design performed in various age groups, and only some of them are supported with data from animal models. Nevertheless, the evidence for CD8+ T cell mediated cytotoxicity as a valid COP is strong, due to a large number of human and animal studies employing different experimental settings and technical assays to demonstrate the relevance of this effector function.Citation23,Citation43,Citation74,Citation76,Citation79,Citation98 Among the cytokine based COPs suggested, there are reasons to consider INF-γ positive T cells as a relevant correlate based on preclinical and clinical studies involving both controlled vaccine efficacy trials and population based observational studies.Citation22,Citation23,Citation77 Although the recently reported protective role of T cell subsets expressing defined combinations of cytokines and surface markers seems promising,Citation77,Citation81,Citation83 the experimental evidence and relative strength for such assays as COPs, so far may be assessed as somewhat weaker.
Mucosal immune responses as COPs
The principal advantage of mucosal immunization is the induction of local immune responses at the site of infection, in addition to systemic immunity. As recently reviewed,Citation99 various immune mechanisms at the mucosal surface of the human respiratory tract may act to prevent or clear influenza infection. It is well established that secretory IgA (sIgA) released at mucosal surfaces, as well as plasma derived IgG can control viral infection in the respiratory tract, but sIgA has a greater potential to cross-protect against heterologous influenza strains.Citation100,Citation101 Mucosal IgA is capable of neutralizing virus once inside mucosal epithelial cells.Citation102 Local intracellular virus may also be cleared by cytolysis of infected cells by intra-epithelial cytotoxic CD8+ T cells, and vaccine induced NK cell mediated mechanisms have also been implicated in viral clearance. The superiority of LAIV over IIV noted in various meta-analyses of vaccine efficacy in different study populations (not only limited to children), is partly attributed to their differential capacity to induce mucosal antibodies in the respiratory tract.Citation103-Citation105 IIV are injected intramuscularly and induce good serum antibody responses but no mucosal antibodies, whereas intranasally administered LAIV efficiently prime both virus-specific mucosal IgA and serum IgG responses.Citation106 Mucosal immune mechanisms are mediated by the mucosa-associated-lymphoid tissue (MALT), a system of anatomically separate but functionally connected mucosal lymphoid sites, such as the nasopharynx-associated lymphoid tissue (NALT) and the gut-associated lymphoid tissue (GALT).Citation107,Citation108 Successful priming at mucosal surfaces requires an appropriate type of vaccine antigen (pathogen derived complex particles), and in case of subunit antigens a mucosal adjuvant system is usually also necessary.Citation109 Vaccine platforms most successful in activating mucosal immunity are either attenuated pathogens (like LAIV), replicating vectors administered to mucosal tissues, or vaccines that mimick mucosal pathogens in particle size, innate properties and the ability to target mucosal dendritic cells (DC).Citation108,Citation110 Delivery routes that are effective at inducing mucosal immune responses in combination with novel influenza vaccine platforms include intranasal,Citation30,Citation60,Citation110-Citation113 oral,Citation95,Citation114 sublingual,Citation115-Citation117 and intradermalCitation118 administration. Some of these concepts are already in clinical development ().
An increasing body of evidence suggests that IgA responses contribute to protection against influenza, and this parameter should be further evaluated as a valid COP, at least for mucosal vaccines. At present measurement of sIgA in mucosal secretions such as nasal lavages is not standardized, neither with regard to clinical sampling nor assay methodology.Citation42 Current methods are mostly based on IgA ELISA,Citation107,Citation119 and the results were found to correlate to HI and VN titers in nasal lavageCitation120 but not in serum. In we have illustrated the broadening of protective immune responses to influenza by highlighting some vaccine concepts in development compared with traditional split or subunit vaccination.
Figure 2. Connectograms showing both type and strength of a spectrum of immune responses induced by principally different influenza vaccine concepts: (A) Classical split or subunit vaccines mostly inducing vaccine specific HA and NA antibodies, (B) Vaccines specifically targeting a more broad antibody response with potential for cross-protection, (C) Vaccines specifically targeting conserved antigens for cross-reactive T cell responses, and (D) LAIV covering a broad range of both humoral and cellular immune responses.
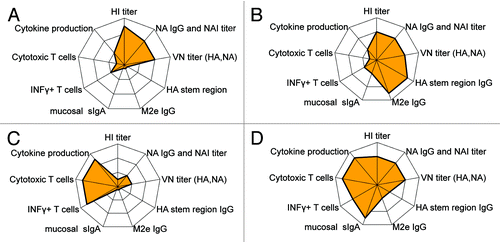
Systems Vaccinology: A New Approach for Discovery and Application of COPs in Vaccine Development
Systems vaccinology represents an entirely different approach which is already showing promise for establishing as yet unknown COPs for several diseases.Citation121-Citation124 Developments in transcriptomics, proteomics, and bioinformatics have made it possible to perform genome-wide and global analysis of host responses after infection and vaccination, allowing for discovery of new and relevant correlates or surrogates of protection. Systems vaccinology aims to identify both innate and adaptive immune signatures able to predict the immunogenicity and protective capacity of vaccines, as well as identification of potentially novel mechanisms of immune regulation. For instance, the outcome of LAIV or IIV vaccination in man with respect to the humoral response could be predicted based on the expression of a defined set of genes only 3 d after vaccination.Citation121 Such systems biology approaches could be useful, not only to shorten clinical testing but also to assess the quality of animal models and identify animal vs. human correlates. A substantial portion of the recently obtained knowledge from systems vaccinology is based on influenza work. We therefore claim that the rapidly moving influenza field strongly contributes to improved understanding of novel biomarkers for protection that may be generally applied to accelerate vaccine development processes.
Animal Models and Bridging to Human COPs
The successful use of animal models for human vaccine development largely lies in the ability to extrapolate virus and vaccine induced immune response, virus behavior and virus induced clinical disease and pathology from animals to humans. A number of small animal models exist for preclinical evaluation of influenza vaccines of which the 2 mostly used model systems are mice and ferrets.Citation125
To be able to measure COPs, the availability of a relevant challenge model remains crucial. To this end, observations and analyses made in infected humans and clinical challenge trialsCitation83 should guide the choice of animal model and outline the parameters to be monitored and measured in a preclinical study. For influenza infection, these parameters include influenza like illness, pathology, mortality, virus susceptibility, replication kinetics and organ distribution, as well as virus transmission. Despite the widespread use of mice, the clinical disease and pathology seen in the mouse influenza model do not correlate well with observations in humans.Citation125-Citation127 Moreover, mice are not susceptible to most human seasonal strains, which require adaptation, and the outcome of challenge studies performed in mice should therefore be treated with caution. On the other hand, ferrets develop fever, nasal discharge, suffer from weight loss and develop lung pathology and thus more closely resemble infection in humans. Ferrets also exhibit the same subtype-dependent severity of disease which can vary from a common cold to a deadly disease.Citation125-Citation128 Moreover, ferrets are susceptible to human and avian influenza strains, largely show the same tissue distribution of infection, and are able to spread the virus through aerosol transmission. This means the ferret is a suitable model to study the effect of vaccines on influenza virus transmission which is particularly important for universal vaccines that most likely will not provide neutralizing protection. Additional factors that should be considered when setting up a challenge model are the route of infectionCitation129 and the infectious dose.Citation130 Intranasal administration of H5N1 influenza results in a severe CNS disease and intratracheal administration in severe pneumoniaCitation129 of which only the latter has been described in humans.Citation131,Citation132
The immune system that most closely resembles humans is that of non-human primates. Although this model is used for some influenza studies it is not the model of widespread choice, due to ethical and economic reasons.Citation125,Citation128 Preclinical research is therefore based on models that to a lesser extent represent the human immune system, but still may be good predictors. Antibody responses can be measured equally well in mice and ferrets by means of ELISA (IgG and IgA), HI, NI, and virus neutralization. The mouse model, however, offers greater opportunities for measuring cellular immune responses. The availability of inbred, knockout and transgenic mouse strains and the feasibility of adoptive transfer and T-cell depletion experiments greatly enhance the investigation of COPs. Such experiments have demonstrated a role for CD8+ T cells in viral clearance and contributed to establishment of immune correlates in mice. Furthermore, single epitope vaccination and flow cytometric analysis of epitope specific INF-γ producing CD8+ T cells has even revealed the contribution of a single T-cell clone to virus control.Citation98 In addition, the availability of HLA-transgenic mice increases the relevance of this research for humans.Citation133,Citation134 These types of experiments are not feasible in ferrets since they are outbred animals, the nature of their MHC is unknown, and cellular reagents are scarce. Nevertheless, some cellular reagents, developed for other species, do show cross-reactivity with some of the CD markers and cytokines in ferrets, which allows the detection of CD3, CD8, and INF-γ.Citation135,Citation136 In addition, INF-γ ELISPOT assays have been developed but are not yet commercially available.Citation137 Moreover, innate cytokine responses have been measured at the mRNA level by RT-PCR.Citation138
Owing to their wide availability, the range of immunological reagents available, easy handling and cost benefits, inbred mice models are typically used as the first model of choice for proof of concept, dose ranging studies, and understanding of COPs. Subsequently, the ferret model is exploited to further investigate the protective effectiveness of a vaccine. Recent developments allowing basic analysis of cellular immune responses in ferrets should ultimately reveal whether these responses correlate with those in humans. If so, this could also shed light on the extent to which murine COPs can be translated to clinical correlates. Although care should be taken,Citation99 utilization of biomarkers for vaccine effectiveness in ferrets for development of human COP assays is regarded as an obligatory and strategic step in influenza vaccine development.
Concluding Remarks
For a range of diseases, markers of an appropriate humoral immune response have so far been the most frequently used and best validated correlates of protective immunity after vaccination. However, technological and conceptual advancements within the field of cell-mediated immunity have led to a number of new immunological read-outs with the potential to emerge as valuable correlates of vaccine-induced protection. Whereas antigen-specific production of the Th1 cytokine IFN-γ has been considered as a quantitative marker of protective immunity, recent evidence from several infectious disease models has pointed to multifunctional CD4+ T cells as an even better correlate of sustained protective immunity.Citation139 In addition, new technological possibilities to measure antigen-specific cytotoxic CD8+ T cell responses and cytokine patterns after infection or vaccination, has opened a new vista to include defined T-cell mediated effector functions in the evaluation of protective immune responses. In this context, the influenza field plays an important role as a trendsetter for a range of other viral and even bacterial diseases by guiding the development of similar COPs needed for fast design and development of new vaccines against emerging infections.
While HI assays still represent the most important COPs for evaluation and licensing of traditional influenza vaccines, new vaccine concepts targeting other antigens, delivery systems, and protective mechanisms have paved the way for several new and emerging COPs with relevance for both antibody and T-cell mediated immunity (). Specifically, the possibility to utilize conserved T-cell antigens (NP, M2, PA, and PB) and conserved HA and NA domains in universal vaccination against seasonal and pandemic influenza, highlights the need for introducing COPs that are relevant to these immune responses. Although the relative importance of the novel COPs reviewed here is difficult to rank, we now have a spectrum of relevant assays available with probably different strength for being used as a predictor of protection in different settings. Hence, the application and practical value of each COP assay may depend on the type of vaccine to be used as well as the immune responses and age groups targeted. Although several new and potential important COPs for influenza have been introduced and successfully applied in vaccine development, there is still a need for validation and standardization of such assays, including definition of protective levels, to secure their optimal use and interpretations in comparative analysis. This will also be a prerequisite before these assays can be adopted by regulatory authorities for approval purposes.
Importantly, research on influenza vaccines and COPs has not only paved the way for improved understanding of protective immune responses against influenza infection and disease, but also of which upcoming and principally different vaccine platforms that can induce the appropriate immune responses. Finally, results from the influenza field have taught us how to measure the corresponding and relevant laboratory-based COPs at the methodological level. All these aspects represent important contributions to the knowledge-based platform we need for rapidly developing efficient vaccines against emerging infections. By systematically extracting and processing the same knowledge elements from a spectrum of viral and bacterial infections, it should be possible to establish general predictive rules for optimal and fast vaccine design based on COPs.
Abbreviations: | ||
ADCC | = | antibody-dependent cell-mediated cytotoxicity |
COP | = | correlate of protection |
HA | = | hemagglutinin |
IIV | = | inactivated influenza vaccine |
LAIV | = | live attenuated influenza vaccine |
M1 | = | matrix protein |
M2 | = | ion channel protein |
M2e | = | extracellular domain of M2 |
NA | = | neuraminidase |
NP | = | nucleoprotein |
PA | = | polymerase A |
PB | = | polymerase B |
WIV | = | whole inactivated virion |
Disclosure of Potential Conflicts of Interest
No potential conflicts of interest were disclosed.
Acknowledgments
This work was conducted as part of “FASTVAC: A generic framework for FAST production and evaluation of emergency VACcines,” a project funded by the Health Program of the European Union. Drs Elly van Riet, Willem Luytjes, and Nynke Rots are kindly acknowledged for advice and Mrs Anke Smits for helping with the manuscript.
References
- Gage KL, Burkot TR, Eisen RJ, Hayes EB. Climate and vectorborne diseases. Am J Prev Med 2008; 35:436 - 50; http://dx.doi.org/10.1016/j.amepre.2008.08.030; PMID: 18929970
- Semenza JC, Menne B. Climate change and infectious diseases in Europe. Lancet Infect Dis 2009; 9:365 - 75; http://dx.doi.org/10.1016/S1473-3099(09)70104-5; PMID: 19467476
- WHO. Severe acute respiratory syndrome (SARS): Status of the outbreak and lessons for the immediate future. 2003. Available from: http://www.who.int/csr/don/2003_04_11/en/
- Assiri A, McGeer A, Perl TM, Price CS, Al Rabeeah AA, Cummings DA, Alabdullatif ZN, Assad M, Almulhim A, Makhdoom H, et al, KSA MERS-CoV Investigation Team. Hospital outbreak of Middle East respiratory syndrome coronavirus. N Engl J Med 2013; 369:407 - 16; http://dx.doi.org/10.1056/NEJMoa1306742; PMID: 23782161
- WHO. Evolution of a pandemic A(H1N1) 2009. 2013.
- Xiong X, Martin SR, Haire LF, Wharton SA, Daniels RS, Bennett MS, McCauley JW, Collins PJ, Walker PA, Skehel JJ, et al. Receptor binding by an H7N9 influenza virus from humans. Nature 2013; 499:496 - 9; http://dx.doi.org/10.1038/nature12372; PMID: 23787694
- WHO. Table of Cumulative number of confirmed human cases for avian influenza A(H5N1) reported to WHO, 2003-2013. 2013. Available from: http://www.who.int/influenza/human_animal_interface/EN_GIP_20131008CumulativeNumberH5N1cases.pdf
- Ambrose CS, Wu X, Belshe RB. The efficacy of live attenuated and inactivated influenza vaccines in children as a function of time postvaccination. Pediatr Infect Dis J 2010; 29:806 - 11; http://dx.doi.org/10.1097/INF.0b013e3181e2872f; PMID: 20458256
- Ansaldi F, Orsi A, de Florentiis D, Parodi V, Rappazzo E, Coppelli M, Durando P, Icardi G. Head-to-head comparison of an intradermal and a virosome influenza vaccine in patients over the age of 60: Evaluation of immunogenicity, cross-protection, safety and tolerability. Hum Vaccin Immunother 2013; 9; In press http://dx.doi.org/10.4161/hv.23240; PMID: 23295262
- Vesikari T, Knuf M, Wutzler P, Karvonen A, Kieninger-Baum D, Schmitt HJ, Baehner F, Borkowski A, Tsai TF, Clemens R. Oil-in-water emulsion adjuvant with influenza vaccine in young children. N Engl J Med 2011; 365:1406 - 16; http://dx.doi.org/10.1056/NEJMoa1010331; PMID: 21995388
- Treanor JJ, El Sahly H, King J, Graham I, Izikson R, Kohberger R, Patriarca P, Cox M. Protective efficacy of a trivalent recombinant hemagglutinin protein vaccine (FluBlok®) against influenza in healthy adults: a randomized, placebo-controlled trial. Vaccine 2011; 29:7733 - 9; http://dx.doi.org/10.1016/j.vaccine.2011.07.128; PMID: 21835220
- Hobson D, Curry RL, Beare AS, Ward-Gardner A. The role of serum haemagglutination-inhibiting antibody in protection against challenge infection with influenza A2 and B viruses. J Hyg (Lond) 1972; 70:767 - 77; http://dx.doi.org/10.1017/S0022172400022610; PMID: 4509641
- de Jong JC, Palache AM, Beyer WE, Rimmelzwaan GF, Boon AC, Osterhaus AD. Haemagglutination-inhibiting antibody to influenza virus. Dev Biol (Basel) 2003; 115:63 - 73; PMID: 15088777
- Treanor J, Wright PF. Immune correlates of protection against influenza in the human challenge model. Dev Biol (Basel) 2003; 115:97 - 104; PMID: 15088780
- Clements ML, Betts RF, Tierney EL, Murphy BR. Serum and nasal wash antibodies associated with resistance to experimental challenge with influenza A wild-type virus. J Clin Microbiol 1986; 24:157 - 60; PMID: 3722363
- Stephenson I, Wood JM, Nicholson KG, Zambon MC. Sialic acid receptor specificity on erythrocytes affects detection of antibody to avian influenza haemagglutinin. J Med Virol 2003; 70:391 - 8; http://dx.doi.org/10.1002/jmv.10408; PMID: 12767002
- Monto AS, Maassab HF. Ether treatment of type B influenza virus antigen for the hemagglutination inhibition test. J Clin Microbiol 1981; 13:54 - 7; PMID: 7462419
- Black S, Nicolay U, Vesikari T, Knuf M, Del Giudice G, Della Cioppa G, Tsai T, Clemens R, Rappuoli R. Hemagglutination inhibition antibody titers as a correlate of protection for inactivated influenza vaccines in children. Pediatr Infect Dis J 2011; 30:1081 - 5; http://dx.doi.org/10.1097/INF.0b013e3182367662; PMID: 21983214
- Granström M, Voordouw AC. Registration of influenza vaccines for children in Europe. Vaccine 2011; 29:7572 - 5; http://dx.doi.org/10.1016/j.vaccine.2011.08.017; PMID: 21820474
- Eichelberger M, Golding H, Hess M, Weir J, Subbarao K, Luke CJ, Friede M, Wood D. FDA/NIH/WHO public workshop on immune correlates of protection against influenza A viruses in support of pandemic vaccine development, Bethesda, Maryland, US, December 10-11, 2007. Vaccine 2008; 26:4299 - 303; http://dx.doi.org/10.1016/j.vaccine.2008.06.012; PMID: 18582523
- Haaheim LR, Katz JM. Immune correlates of protection against influenza: challenges for licensure of seasonal and pandemic influenza vaccines, Miami, FL, USA, March 1-3, 2010. Influenza Other Respir Viruses 2011; 5:288 - 95; http://dx.doi.org/10.1111/j.1750-2659.2011.00242.x; PMID: 21651740
- Forrest BD, Pride MW, Dunning AJ, Capeding MR, Chotpitayasunondh T, Tam JS, Rappaport R, Eldridge JH, Gruber WC. Correlation of cellular immune responses with protection against culture-confirmed influenza virus in young children. Clin Vaccine Immunol 2008; 15:1042 - 53; http://dx.doi.org/10.1128/CVI.00397-07; PMID: 18448618
- McElhaney JE, Xie D, Hager WD, Barry MB, Wang Y, Kleppinger A, Ewen C, Kane KP, Bleackley RC. T cell responses are better correlates of vaccine protection in the elderly. J Immunol. 2006; 176:10 6333 - 9
- McElhaney JE. Influenza vaccine responses in older adults. Ageing Res Rev 2011; 10:379 - 88; http://dx.doi.org/10.1016/j.arr.2010.10.008; PMID: 21055484
- Kreijtz JH, Fouchier RA, Rimmelzwaan GF. Immune responses to influenza virus infection. Virus Res 2011; 162:19 - 30; http://dx.doi.org/10.1016/j.virusres.2011.09.022; PMID: 21963677
- McCullers JA, Huber VC. Correlates of vaccine protection from influenza and its complications. Hum Vaccin Immunother 2012; 8:34 - 44; http://dx.doi.org/10.4161/hv.8.1.18214; PMID: 22252001
- Dormitzer PR, Tsai TF, Del Giudice G. New technologies for influenza vaccines. Hum Vaccin Immunother 2012; 8:45 - 58; http://dx.doi.org/10.4161/hv.8.1.18859; PMID: 22251994
- Lambert LC, Fauci AS. Influenza vaccines for the future. N Engl J Med 2010; 363:2036 - 44; http://dx.doi.org/10.1056/NEJMra1002842; PMID: 21083388
- Shaw A. New technologies for new influenza vaccines. Vaccine 2012; 30:4927 - 33; http://dx.doi.org/10.1016/j.vaccine.2012.04.095; PMID: 22579861
- WHO. Table for pandemic and potentially pandemic influenza vaccines. 2013. Available from: http://www.who.int/entity/immunization/research/development/Table_pandemic_vaccines.xls?ua=1
- Amorij JP, Hinrichs WLj, Frijlink HW, Wilschut JC, Huckriede A. Needle-free influenza vaccination. Lancet Infect Dis 2010; 10:699 - 711; http://dx.doi.org/10.1016/S1473-3099(10)70157-2; PMID: 20883966
- Palese P. Making better influenza virus vaccines?. Emerg Infect Dis 2006; 12:61 - 5; http://dx.doi.org/10.3201/eid1201.051043; PMID: 16494719
- Monto AS, Ohmit SE. Seasonal influenza vaccines: evolutions and future trends. Expert Rev Vaccines 2009; 8:383 - 9; http://dx.doi.org/10.1586/erv.09.9; PMID: 19348555
- Gilbert SC. Advances in the development of universal influenza vaccines. Influenza Other Respi Viruses 2013; 7:5 750 - 8; http://dx.doi.org/10.1111/irv.12013; PMID: 22998622
- Rudolph W, Ben Yedidia T. A universal influenza vaccine: where are we in the pursuit of this “Holy Grail”?. Hum Vaccin 2011; 7:10 - 1; http://dx.doi.org/10.4161/hv.7.1.14925; PMID: 21285533
- Safdar A, Cox MM. Baculovirus-expressed influenza vaccine. A novel technology for safe and expeditious vaccine production for human use. Expert Opin Investig Drugs 2007; 16:927 - 34; http://dx.doi.org/10.1517/13543784.16.7.927; PMID: 17594180
- Hillaire ML, Osterhaus AD, Rimmelzwaan GF. Induction of virus-specific cytotoxic T lymphocytes as a basis for the development of broadly protective influenza vaccines. J Biomed Biotechnol 2011; 2011:939860
- Schotsaert M, De Filette M, Fiers W, Saelens X. Universal M2 ectodomain-based influenza A vaccines: preclinical and clinical developments. Expert Rev Vaccines 2009; 8:499 - 508; http://dx.doi.org/10.1586/erv.09.6; PMID: 19348565
- The First WHO integrated meeting on development and clinical trials of Influenza vaccines that induce broadly protective and long-lasting immune responses. 2013. Available from: http://www.who.int/immunization/research/meetings_workshops/fluvaccines_devclinicaltrials_jan13/en/
- Van Kerkhove MD, Broberg E, Engelhardt OG, Wood J, Nicoll A, CONSISE steering committee. The consortium for the standardization of influenza seroepidemiology (CONSISE): a global partnership to standardize influenza seroepidemiology and develop influenza investigation protocols to inform public health policy. Influenza Other Respir Viruses 2013; 7:231 - 4; http://dx.doi.org/10.1111/irv.12068; PMID: 23280042
- Wagner R, Pfleiderer M. Reproducibility of assays for influenza vaccine immunogenicity determination: progress towards consistency. Expert Rev Vaccines 2012; 11:881 - 3; http://dx.doi.org/10.1586/erv.12.62; PMID: 23002967
- Reber A, Katz J. Immunological assessment of influenza vaccines and immune correlates of protection. Expert Rev Vaccines 2013; 12:519 - 36; http://dx.doi.org/10.1586/erv.13.35; PMID: 23659300
- Rimmelzwaan GF, McElhaney JE. Correlates of protection: novel generations of influenza vaccines. Vaccine 2008; 26:Suppl 4 D41 - 4; http://dx.doi.org/10.1016/j.vaccine.2008.07.043; PMID: 19230158
- Plotkin SA. Vaccines: correlates of vaccine-induced immunity. Clin Infect Dis. 2008; 47:3 401 - 9
- Belshe RB, Gruber WC, Mendelman PM, Mehta HB, Mahmood K, Reisinger K, Treanor J, Zangwill K, Hayden FG, Bernstein DI, et al. Correlates of immune protection induced by live, attenuated, cold-adapted, trivalent, intranasal influenza virus vaccine. J Infect Dis 2000; 181:1133 - 7; http://dx.doi.org/10.1086/315323; PMID: 10720541
- Laidlaw BJ, Decman V, Ali MA, Abt MC, Wolf AI, Monticelli LA, Mozdzanowska K, Angelosanto JM, Artis D, Erikson J, et al. Cooperativity between CD8+ T cells, non-neutralizing antibodies, and alveolar macrophages is important for heterosubtypic influenza virus immunity. PLoS Pathog 2013; 9:e1003207; http://dx.doi.org/10.1371/journal.ppat.1003207; PMID: 23516357
- Ekiert DC, Bhabha G, Elsliger MA, Friesen RH, Jongeneelen M, Throsby M, Goudsmit J, Wilson IA. Antibody recognition of a highly conserved influenza virus epitope. Science 2009; 324:246 - 51; http://dx.doi.org/10.1126/science.1171491; PMID: 19251591
- Throsby M, van den Brink E, Jongeneelen M, Poon LL, Alard P, Cornelissen L, Bakker A, Cox F, van Deventer E, Guan Y, et al. Heterosubtypic neutralizing monoclonal antibodies cross-protective against H5N1 and H1N1 recovered from human IgM+ memory B cells. PLoS One 2008; 3:e3942; http://dx.doi.org/10.1371/journal.pone.0003942; PMID: 19079604
- Sui J, Hwang WC, Perez S, Wei G, Aird D, Chen LM, Santelli E, Stec B, Cadwell G, Ali M, et al. Structural and functional bases for broad-spectrum neutralization of avian and human influenza A viruses. Nat Struct Mol Biol 2009; 16:265 - 73; http://dx.doi.org/10.1038/nsmb.1566; PMID: 19234466
- Dreyfus C, Laursen NS, Kwaks T, Zuijdgeest D, Khayat R, Ekiert DC, Lee JH, Metlagel Z, Bujny MV, Jongeneelen M, et al. Highly conserved protective epitopes on influenza B viruses. Science 2012; 337:1343 - 8; http://dx.doi.org/10.1126/science.1222908; PMID: 22878502
- Ledgerwood JE, Wei CJ, Hu Z, Gordon IJ, Enama ME, Hendel CS, McTamney PM, Pearce MB, Yassine HM, Boyington JC, et al, VRC 306 Study Team. DNA priming and influenza vaccine immunogenicity: two phase 1 open label randomised clinical trials. Lancet Infect Dis 2011; 11:916 - 24; http://dx.doi.org/10.1016/S1473-3099(11)70240-7; PMID: 21975270
- Bommakanti G, Lu X, Citron MP, Najar TA, Heidecker GJ, ter Meulen J, Varadarajan R, Liang X. Design of Escherichia coli-expressed stalk domain immunogens of H1N1 hemagglutinin that protect mice from lethal challenge. J Virol 2012; 86:13434 - 44; http://dx.doi.org/10.1128/JVI.01429-12; PMID: 23015722
- Steel J, Lowen AC, Wang TT, Yondola M, Gao Q, Haye K, García-Sastre A, Palese P. Influenza virus vaccine based on the conserved hemagglutinin stalk domain. MBio 2010; 1:1; http://dx.doi.org/10.1128/mBio.00018-10; PMID: 20689752
- Krammer F, Pica N, Hai R, Margine I, Palese P. Chimeric hemagglutinin influenza virus vaccine constructs elicit broadly protective stalk-specific antibodies. J Virol 2013; 87:6542 - 50; http://dx.doi.org/10.1128/JVI.00641-13; PMID: 23576508
- Corti D, Suguitan AL Jr., Pinna D, Silacci C, Fernandez-Rodriguez BM, Vanzetta F, Santos C, Luke CJ, Torres-Velez FJ, Temperton NJ, et al. Heterosubtypic neutralizing antibodies are produced by individuals immunized with a seasonal influenza vaccine. J Clin Invest 2010; 120:1663 - 73; http://dx.doi.org/10.1172/JCI41902; PMID: 20389023
- Murphy BR, Kasel JA, Chanock RM. Association of serum anti-neuraminidase antibody with resistance to influenza in man. N Engl J Med 1972; 286:1329 - 32; http://dx.doi.org/10.1056/NEJM197206222862502; PMID: 5027388
- Couch RB, Atmar RL, Keitel WA, Quarles JM, Wells J, Arden N, Niño D. Randomized comparative study of the serum antihemagglutinin and antineuraminidase antibody responses to six licensed trivalent influenza vaccines. Vaccine 2012; 31:190 - 5; http://dx.doi.org/10.1016/j.vaccine.2012.10.065; PMID: 23107591
- Sandbulte MR, Jimenez GS, Boon AC, Smith LR, Treanor JJ, Webby RJ. Cross-reactive neuraminidase antibodies afford partial protection against H5N1 in mice and are present in unexposed humans. PLoS Med 2007; 4:e59; http://dx.doi.org/10.1371/journal.pmed.0040059; PMID: 17298168
- Monto AS, Kendal AP. Effect of neuraminidase antibody on Hong Kong influenza. Lancet 1973; 1:7804 623 - 5; http://dx.doi.org/10.1016/S0140-6736(73)92196-X; PMID: 4121842
- Pushko P, Kort T, Nathan M, Pearce MB, Smith G, Tumpey TM. Recombinant H1N1 virus-like particle vaccine elicits protective immunity in ferrets against the 2009 pandemic H1N1 influenza virus. Vaccine 2010; 28:4771 - 6; http://dx.doi.org/10.1016/j.vaccine.2010.04.093; PMID: 20470801
- Couch RB, Atmar RL, Franco LM, Quarles JM, Wells J, Arden N, Niño D, Belmont JW. Antibody correlates and predictors of immunity to naturally occurring influenza in humans and the importance of antibody to the neuraminidase. J Infect Dis 2013; 207:974 - 81; http://dx.doi.org/10.1093/infdis/jis935; PMID: 23307936
- Lambré CR, Terzidis H, Greffard A, Webster RG. Measurement of anti-influenza neuraminidase antibody using a peroxidase-linked lectin and microtitre plates coated with natural substrates. J Immunol Methods 1990; 135:49 - 57; http://dx.doi.org/10.1016/0022-1759(90)90255-T; PMID: 1703190
- Cate TR, Rayford Y, Niño D, Winokur P, Brady R, Belshe R, Chen W, Atmar RL, Couch RB. A high dosage influenza vaccine induced significantly more neuraminidase antibody than standard vaccine among elderly subjects. Vaccine 2010; 28:2076 - 9; http://dx.doi.org/10.1016/j.vaccine.2009.12.041; PMID: 20044052
- Gravel C, Li C, Wang J, Hashem AM, Jaentschke B, Xu KW, Lorbetskie B, Gingras G, Aubin Y, Van Domselaar G, et al. Qualitative and quantitative analyses of virtually all subtypes of influenza A and B viral neuraminidases using antibodies targeting the universally conserved sequences. Vaccine 2010; 28:5774 - 84; http://dx.doi.org/10.1016/j.vaccine.2010.06.075; PMID: 20621113
- European Medicines Agency. Scientific workshop on serology assays and correlates of protection for influenza vaccines. 2010. Available from: http://www.ema.europa.eu/docs/en_GB/document_library/Report/2010/12/WC500100205.pdf
- Gavrilov V, Orekov T, Alabanza C, Porika U, Jiang H, Connolly K, Pincus S. Influenza virus-like particles as a new tool for vaccine immunogenicity testing: validation of a neuraminidase neutralizing antibody assay. J Virol Methods 2011; 173:364 - 73; http://dx.doi.org/10.1016/j.jviromet.2011.03.011; PMID: 21419169
- Hassantoufighi A, Zhang H, Sandbulte M, Gao J, Manischewitz J, King L, Golding H, Straight TM, Eichelberger MC. A practical influenza neutralization assay to simultaneously quantify hemagglutinin and neuraminidase-inhibiting antibody responses. Vaccine 2010; 28:790 - 7; http://dx.doi.org/10.1016/j.vaccine.2009.10.066; PMID: 19887135
- El Bakkouri K, Descamps F, De Filette M, Smet A, Festjens E, Birkett A, Van Rooijen N, Verbeek S, Fiers W, Saelens X.. Universal vaccine based on ectodomain of matrix protein 2 of influenza A: Fc receptors and alveolar macrophages mediate protection. J Immunol. 2011; 186:2 1022 - 31
- Jegerlehner A, Schmitz N, Storni T, Bachmann MF. Influenza A vaccine based on the extracellular domain of M2: weak protection mediated via antibody-dependent NK cell activity. J Immunol. 2004; 172:9 5598 - 605
- Shaw AR. Universal influenza vaccine: the holy grail?. Expert Rev Vaccines 2012; 11:923 - 7; http://dx.doi.org/10.1586/erv.12.73; PMID: 23002973
- Fu TM, Grimm KM, Citron MP, Freed DC, Fan J, Keller PM, Shiver JW, Liang X, Joyce JG. Comparative immunogenicity evaluations of influenza A virus M2 peptide as recombinant virus like particle or conjugate vaccines in mice and monkeys. Vaccine 2009; 27:1440 - 7; http://dx.doi.org/10.1016/j.vaccine.2008.12.034; PMID: 19146898
- Livingston BD, Higgins D, Van Nest G. Evolving strategies for the prevention of influenza infection: potential for multistrain targeting. BioDrugs 2006; 20:6 335 - 40
- Zhong W, He J, Tang X, Liu F, Lu X, Zeng H, Vafai A, Fu TM, Katz JM, Hancock K. Development and evaluation of an M2-293FT cell-based flow cytometric assay for quantification of antibody response to native form of matrix protein 2 of influenza A viruses. J Immunol Methods 2011; 369:115 - 24; http://dx.doi.org/10.1016/j.jim.2011.04.010; PMID: 21570401
- McMichael AJ, Gotch FM, Noble GR, Beare PA. Cytotoxic T-cell immunity to influenza. N Engl J Med 1983; 309:13 - 7; http://dx.doi.org/10.1056/NEJM198307073090103; PMID: 6602294
- Greenbaum JA, Kotturi MF, Kim Y, Oseroff C, Vaughan K, Salimi N, Vita R, Ponomarenko J, Scheuermann RH, Sette A, et al. Pre-existing immunity against swine-origin H1N1 influenza viruses in the general human population. Proc Natl Acad Sci U S A 2009; 106:20365 - 70; http://dx.doi.org/10.1073/pnas.0911580106; PMID: 19918065
- McElhaney JE, Gravenstein S, Upshaw CM, Hooton JW, Krause P, Drinka P, Bleackley RC. Granzyme B: a marker of risk for influenza in institutionalized older adults. Vaccine 2001; 19:3744 - 51; http://dx.doi.org/10.1016/S0264-410X(01)00087-1; PMID: 11395209
- Sridhar S, Begom S, Bermingham A, Hoschler K, Adamson W, Carman W, Bean T, Barclay W, Deeks JJ, Lalvani A. Cellular immune correlates of protection against symptomatic pandemic influenza. Nat Med 2013; 19:1305 - 12; http://dx.doi.org/10.1038/nm.3350; PMID: 24056771
- McElhaney JE. Prevention of infectious diseases in older adults through immunization: the challenge of the senescent immune response. Expert Rev Vaccines 2009; 8:593 - 606; http://dx.doi.org/10.1586/erv.09.12; PMID: 19397416
- McElhaney JE, Ewen C, Zhou X, Kane KP, Xie D, Hager WD, Barry MB, Kleppinger A, Wang Y, Bleackley RC. Granzyme B: Correlates with protection and enhanced CTL response to influenza vaccination in older adults. Vaccine 2009; 27:2418 - 25; http://dx.doi.org/10.1016/j.vaccine.2009.01.136; PMID: 19368783
- Shahid Z, Kleppinger A, Gentleman B, Falsey AR, McElhaney JE. Clinical and immunologic predictors of influenza illness among vaccinated older adults. Vaccine 2010; 28:6145 - 51; http://dx.doi.org/10.1016/j.vaccine.2010.07.036; PMID: 20646987
- Bentebibel SE, Lopez S, Obermoser G, Schmitt N, Mueller C, Harrod C, Flano E, Mejias A, Albrecht RA, Blankenship D, et al. Induction of ICOS+CXCR3+CXCR5+ TH cells correlates with antibody responses to influenza vaccination. Sci Transl Med. 2013; 5:176 176ra32
- Swain SL, McKinstry KK, Strutt TM. Expanding roles for CD4⁺ T cells in immunity to viruses. Nat Rev Immunol 2012; 12:136 - 48; PMID: 22266691
- Wilkinson TM, Li CK, Chui CS, Huang AK, Perkins M, Liebner JC, Lambkin-Williams R, Gilbert A, Oxford J, Nicholas B, et al. Preexisting influenza-specific CD4+ T cells correlate with disease protection against influenza challenge in humans. Nat Med 2012; 18:274 - 80; http://dx.doi.org/10.1038/nm.2612; PMID: 22286307
- Galli G, Medini D, Borgogni E, Zedda L, Bardelli M, Malzone C, Nuti S, Tavarini S, Sammicheli C, Hilbert AK, et al. Adjuvanted H5N1 vaccine induces early CD4+ T cell response that predicts long-term persistence of protective antibody levels. Proc Natl Acad Sci U S A 2009; 106:3877 - 82; http://dx.doi.org/10.1073/pnas.0813390106; PMID: 19237568
- Pedersen G, Major D, Roseby S, Wood J, Madhun AS, Cox RJ. Matrix-M adjuvanted virosomal H5N1 vaccine confers protection against lethal viral challenge in a murine model. Influenza Other Respir Viruses 2011; 5:426 - 37; http://dx.doi.org/10.1111/j.1750-2659.2011.00256.x; PMID: 21668670
- Kang KS, Lee N, Shin MS, Kim SD, Yu Y, Mohanty S, Belshe RB, Montgomery RR, Shaw AC, Kang I. An altered relationship of influenza vaccine-specific IgG responses with T cell immunity occurs with aging in humans. Clin Immunol 2013; 147:79 - 88; http://dx.doi.org/10.1016/j.clim.2013.02.022; PMID: 23578549
- Hillaire ML, van Trierum SE, Kreijtz JH, Bodewes R, Geelhoed-Mieras MM, Nieuwkoop NJ, Fouchier RA, Kuiken T, Osterhaus AD, Rimmelzwaan GF. Cross-protective immunity against influenza pH1N1 2009 viruses induced by seasonal influenza A (H3N2) virus is mediated by virus-specific T-cells. J Gen Virol 2011; 92:2339 - 49; http://dx.doi.org/10.1099/vir.0.033076-0; PMID: 21653752
- Hillaire M, Rimmelzwaan G, Kreijtz J.. Clearance of influenza virus infections by T cells: risk of collateral damage?. Curr Opin Virol. 2013; 3:4 430 - 7
- Sun J, Braciale TJ. Role of T cell immunity in recovery from influenza virus infection. Curr Opin Virol. 2013; 3:4 425 - 9
- Khurana S, Loving CL, Manischewitz J, King LR, Gauger PC, Henningson J, Vincent AL, Golding H. Vaccine-induced anti-HA2 antibodies promote virus fusion and enhance influenza virus respiratory disease. Sci Transl Med 2013; 5:200 200ra114
- Berthoud TK, Hamill M, Lillie PJ, Hwenda L, Collins KA, Ewer KJ, Milicic A, Poyntz HC, Lambe T, Fletcher HA, et al. Potent CD8+ T-cell immunogenicity in humans of a novel heterosubtypic influenza A vaccine, MVA-NP+M1. Clin Infect Dis. 2011; 52:1 1 - 7
- Lillie PJ, Berthoud TK, Powell TJ, Lambe T, Mullarkey C, Spencer AJ, Hamill M, Peng Y, Blais ME, Duncan CJ, et al. Preliminary assessment of the efficacy of a T-cell-based influenza vaccine, MVA-NP+M1, in humans. Clin Infect Dis. 2012; 55:1 19 - 25
- Pleguezuelos O, Robinson S, Stoloff GA, Caparrós-Wanderley W. Synthetic Influenza vaccine (FLU-v) stimulates cell mediated immunity in a double-blind, randomised, placebo-controlled Phase I trial. Vaccine 2012; 30:4655 - 60; http://dx.doi.org/10.1016/j.vaccine.2012.04.089; PMID: 22575166
- Systems IT. Flunisyn, pan-influenza strain vaccine. Trends Immunol. 2011; 32:11 524 - 31
- Gurwith M, Lock M, Taylor EM, Ishioka G, Alexander J, Mayall T, Ervin JE, Greenberg RN, Strout C, Treanor JJ, et al. Safety and immunogenicity of an oral, replicating adenovirus serotype 4 vector vaccine for H5N1 influenza: a randomised, double-blind, placebo-controlled, phase 1 study. Lancet Infect Dis 2013; 13:238 - 50; http://dx.doi.org/10.1016/S1473-3099(12)70345-6; PMID: 23369412
- Antrobus RD, Coughlan L, Berthoud TK, Dicks MD, Hill AV, Lambe T, Gilbert SC. Clinical Assessment of a Novel Recombinant Simian Adenovirus ChAdOx1 as a Vectored Vaccine Expressing Conserved Influenza A Antigens. Mol Ther 2014; 22:3 668 - 74
- Gijzen K, Liu WM, Visontai I, Oftung F, van der Werf S, Korsvold GE, Pronk I, Aaberge IS, Tütto A, Jankovics I, et al. Standardization and validation of assays determining cellular immune responses against influenza. Vaccine 2010; 28:3416 - 22; http://dx.doi.org/10.1016/j.vaccine.2010.02.076; PMID: 20206285
- Thomas PG, Keating R, Hulse-Post DJ, Doherty PC. Cell-mediated protection in influenza infection. Emerg Infect Dis 2006; 12:48 - 54; http://dx.doi.org/10.3201/eid1201.051237; PMID: 16494717
- Rose MA, Zielen S, Baumann U. Mucosal immunity and nasal influenza vaccination. Expert Rev Vaccines 2012; 11:595 - 607; http://dx.doi.org/10.1586/erv.12.31; PMID: 22827245
- Asahi Y, Yoshikawa T, Watanabe I, Iwasaki T, Hasegawa H, Sato Y, Shimada S, Nanno M, Matsuoka Y, Ohwaki M, et al. Protection against influenza virus infection in polymeric Ig receptor knockout mice immunized intranasally with adjuvant-combined vaccines. J Immunol 2002; 168:6 2930 - 8
- Taylor HP, Dimmock NJ. Mechanism of neutralization of influenza virus by secretory IgA is different from that of monomeric IgA or IgG. J Exp Med 1985; 161:198 - 209; http://dx.doi.org/10.1084/jem.161.1.198; PMID: 2981953
- Mazanec MB, Coudret CL, Fletcher DR. Intracellular neutralization of influenza virus by immunoglobulin A anti-hemagglutinin monoclonal antibodies. J Virol 1995; 69:1339 - 43; PMID: 7815518
- Belshe RB, Edwards KM, Vesikari T, Black SV, Walker RE, Hultquist M, Kemble G, Connor EM, CAIV-T Comparative Efficacy Study Group. Live attenuated versus inactivated influenza vaccine in infants and young children. N Engl J Med 2007; 356:685 - 96; http://dx.doi.org/10.1056/NEJMoa065368; PMID: 17301299
- Osterholm MT, Kelley NS, Sommer A, Belongia EA. Efficacy and effectiveness of influenza vaccines: a systematic review and meta-analysis. Lancet Infect Dis 2012; 12:36 - 44; http://dx.doi.org/10.1016/S1473-3099(11)70295-X; PMID: 22032844
- Rhorer J, Ambrose CS, Dickinson S, Hamilton H, Oleka NA, Malinoski FJ, Wittes J. Efficacy of live attenuated influenza vaccine in children: A meta-analysis of nine randomized clinical trials. Vaccine 2009; 27:1101 - 10; http://dx.doi.org/10.1016/j.vaccine.2008.11.093; PMID: 19095024
- Hoft DF, Babusis E, Worku S, Spencer CT, Lottenbach K, Truscott SM, Abate G, Sakala IG, Edwards KM, Creech CB, et al. Live and inactivated influenza vaccines induce similar humoral responses, but only live vaccines induce diverse T-cell responses in young children. J Infect Dis 2011; 204:845 - 53; http://dx.doi.org/10.1093/infdis/jir436; PMID: 21846636
- Brandtzaeg P. Induction of secretory immunity and memory at mucosal surfaces. Vaccine 2007; 25:5467 - 84; http://dx.doi.org/10.1016/j.vaccine.2006.12.001; PMID: 17227687
- Neutra MR, Kozlowski PA. Mucosal vaccines: the promise and the challenge. Nat Rev Immunol 2006; 6:148 - 58; http://dx.doi.org/10.1038/nri1777; PMID: 16491139
- Weiner HL. The mucosal milieu creates tolerogenic dendritic cells and T(R)1 and T(H)3 regulatory cells. Nat Immunol 2001; 2:671 - 2; http://dx.doi.org/10.1038/90604; PMID: 11477400
- Bessa J, Schmitz N, Hinton HJ, Schwarz K, Jegerlehner A, Bachmann MF. Efficient induction of mucosal and systemic immune responses by virus-like particles administered intranasally: implications for vaccine design. Eur J Immunol 2008; 38:114 - 26; http://dx.doi.org/10.1002/eji.200636959; PMID: 18081037
- Burt D, Mallett C, Plante M, Zimmermann J, Torossian K, Fries L. Proteosome-adjuvanted intranasal influenza vaccines: advantages, progress and future considerations. Expert Rev Vaccines 2011; 10:365 - 75; http://dx.doi.org/10.1586/erv.10.172; PMID: 21434804
- Saluja V, Amorij JP, van Roosmalen ML, Leenhouts K, Huckriede A, Hinrichs WL, Frijlink HW. Intranasal delivery of influenza subunit vaccine formulated with GEM particles as an adjuvant. AAPS J 2010; 12:109 - 16; http://dx.doi.org/10.1208/s12248-009-9168-2; PMID: 20058113
- Zhang J. Advances and future challenges in recombinant adenoviral vectored H5N1 influenza vaccines. Viruses 2012; 4:2711 - 35; http://dx.doi.org/10.3390/v4112711; PMID: 23202501
- Peters W, Brandl JR, Lindbloom JD, Martinez CJ, Scallan CD, Trager GR, Tingley DW, Kabongo ML, Tucker SN. Oral administration of an adenovirus vector encoding both an avian influenza A hemagglutinin and a TLR3 ligand induces antigen specific granzyme B and IFN-γ T cell responses in humans. Vaccine 2013; 31:1752 - 8; http://dx.doi.org/10.1016/j.vaccine.2013.01.023; PMID: 23357198
- Park HJ, Ferko B, Byun YH, Song JH, Han GY, Roethl E, Egorov A, Muster T, Seong B, Kweon MN, et al. Sublingual immunization with a live attenuated influenza a virus lacking the nonstructural protein 1 induces broad protective immunity in mice. PLoS One 2012; 7:e39921; http://dx.doi.org/10.1371/journal.pone.0039921; PMID: 22761928
- Shim BS, Choi YK, Yun CH, Lee EG, Jeon YS, Park SM, Cheon IS, Joo DH, Cho CH, Song MS, et al. Sublingual immunization with M2-based vaccine induces broad protective immunity against influenza. PLoS One 2011; 6:e27953; http://dx.doi.org/10.1371/journal.pone.0027953; PMID: 22140491
- Song JH, Nguyen HH, Cuburu N, Horimoto T, Ko SY, Park SH, Czerkinsky C, Kweon MN. Sublingual vaccination with influenza virus protects mice against lethal viral infection. Proc Natl Acad Sci U S A 2008; 105:1644 - 9; http://dx.doi.org/10.1073/pnas.0708684105; PMID: 18227512
- Shen X, Söderholm J, Lin F, Kobinger G, Bello A, Gregg DA, Broderick KE, Sardesai NY. Influenza A vaccines using linear expression cassettes delivered via electroporation afford full protection against challenge in a mouse model. Vaccine 2012; 30:6946 - 54; http://dx.doi.org/10.1016/j.vaccine.2012.02.071; PMID: 22406460
- Barría MI, Garrido JL, Stein C, Scher E, Ge Y, Engel SM, Kraus TA, Banach D, Moran TM. Localized mucosal response to intranasal live attenuated influenza vaccine in adults. J Infect Dis 2013; 207:115 - 24; http://dx.doi.org/10.1093/infdis/jis641; PMID: 23087433
- Ainai A, Tamura S, Suzuki T, van Riet E, Ito R, Odagiri T, Tashiro M, Kurata T, Hasegawa H. Intranasal vaccination with an inactivated whole influenza virus vaccine induces strong antibody responses in serum and nasal mucus of healthy adults. Hum Vaccin Immunother 2013; 9:1962 - 70; http://dx.doi.org/10.4161/hv.25458; PMID: 23896606
- Nakaya HI, Wrammert J, Lee EK, Racioppi L, Marie-Kunze S, Haining WN, Means AR, Kasturi SP, Khan N, Li GM, et al. Systems biology of vaccination for seasonal influenza in humans. Nat Immunol 2011; 12:786 - 95; http://dx.doi.org/10.1038/ni.2067; PMID: 21743478
- Querec TD, Akondy RS, Lee EK, Cao W, Nakaya HI, Teuwen D, Pirani A, Gernert K, Deng J, Marzolf B, et al. Systems biology approach predicts immunogenicity of the yellow fever vaccine in humans. Nat Immunol 2009; 10:116 - 25; http://dx.doi.org/10.1038/ni.1688; PMID: 19029902
- Lambert ND, Ovsyannikova IG, Pankratz VS, Jacobson RM, Poland GA. Understanding the immune response to seasonal influenza vaccination in older adults: a systems biology approach. Expert Rev Vaccines 2012; 11:985 - 94; http://dx.doi.org/10.1586/erv.12.61; PMID: 23002979
- Obermoser G, Presnell S, Domico K, Xu H, Wang Y, Anguiano E, Thompson-Snipes L, Ranganathan R, Zeitner B, Bjork A, et al. Systems scale interactive exploration reveals quantitative and qualitative differences in response to influenza and pneumococcal vaccines. Immunity 2013; 38:831 - 44; http://dx.doi.org/10.1016/j.immuni.2012.12.008; PMID: 23601689
- Bodewes R, Rimmelzwaan GF, Osterhaus AD. Animal models for the preclinical evaluation of candidate influenza vaccines. Expert Rev Vaccines 2010; 9:59 - 72; http://dx.doi.org/10.1586/erv.09.148; PMID: 20021306
- Belser JA, Tumpey TM. H5N1 pathogenesis studies in mammalian models. Virus Res 2013; 178:168 - 85; http://dx.doi.org/10.1016/j.virusres.2013.02.003; PMID: 23458998
- Tripp RA, Tompkins SM. Animal models for evaluation of influenza vaccines. Curr Top Microbiol Immunol 2009; 333:397 - 412; http://dx.doi.org/10.1007/978-3-540-92165-3_19; PMID: 19768416
- van der Laan JW, Herberts C, Lambkin-Williams R, Boyers A, Mann AJ, Oxford J. Animal models in influenza vaccine testing. Expert Rev Vaccines 2008; 7:783 - 93; http://dx.doi.org/10.1586/14760584.7.6.783; PMID: 18665776
- Bodewes R, Kreijtz JH, van Amerongen G, Fouchier RA, Osterhaus AD, Rimmelzwaan GF, Kuiken T. Pathogenesis of Influenza A/H5N1 virus infection in ferrets differs between intranasal and intratracheal routes of inoculation. Am J Pathol 2011; 179:30 - 6; http://dx.doi.org/10.1016/j.ajpath.2011.03.026; PMID: 21640972
- Marois I, Cloutier A, Garneau É, Richter MV. Initial infectious dose dictates the innate, adaptive, and memory responses to influenza in the respiratory tract. J Leukoc Biol 2012; 92:107 - 21; http://dx.doi.org/10.1189/jlb.1011490; PMID: 22504848
- Abdel-Ghafar AN, Chotpitayasunondh T, Gao Z, Hayden FG, Nguyen DH, de Jong MD, Naghdaliyev A, Peiris JS, Shindo N, Soeroso S, et al, Writing Committee of the Second World Health Organization Consultation on Clinical Aspects of Human Infection with Avian Influenza A (H5N1) Virus. Update on avian influenza A (H5N1) virus infection in humans. N Engl J Med 2008; 358:261 - 73; http://dx.doi.org/10.1056/NEJMra0707279; PMID: 18199865
- Korteweg C, Gu J. Pathology, molecular biology, and pathogenesis of avian influenza A (H5N1) infection in humans. Am J Pathol 2008; 172:1155 - 70; http://dx.doi.org/10.2353/ajpath.2008.070791; PMID: 18403604
- Plotnicky H, Cyblat-Chanal D, Aubry JP, Derouet F, Klinguer-Hamour C, Beck A, Bonnefoy JY, Corvaïa N. The immunodominant influenza matrix T cell epitope recognized in human induces influenza protection in HLA-A2/K(b) transgenic mice. Virology 2003; 309:320 - 9; http://dx.doi.org/10.1016/S0042-6822(03)00072-2; PMID: 12758178
- Tan AC, Deliyannis G, Bharadwaj M, Brown LE, Zeng W, Jackson DC. The design and proof of concept for a CD8(+) T cell-based vaccine inducing cross-subtype protection against influenza A virus. Immunol Cell Biol 2013; 91:96 - 104; http://dx.doi.org/10.1038/icb.2012.54; PMID: 23146941
- Martel CJ, Aasted B. Characterization of antibodies against ferret immunoglobulins, cytokines and CD markers. Vet Immunol Immunopathol 2009; 132:109 - 15; http://dx.doi.org/10.1016/j.vetimm.2009.05.011; PMID: 19505731
- Rutigliano JA, Doherty PC, Franks J, Morris MY, Reynolds C, Thomas PG. Screening monoclonal antibodies for cross-reactivity in the ferret model of influenza infection. J Immunol Methods 2008; 336:71 - 7; http://dx.doi.org/10.1016/j.jim.2008.04.003; PMID: 18485358
- Ochi A, Danesh A, Seneviratne C, Banner D, Devries ME, Rowe T, Xu L, Ran L, Czub M, Bosinger SE, et al. Cloning, expression and immunoassay detection of ferret IFN-gamma. Dev Comp Immunol 2008; 32:890 - 7; http://dx.doi.org/10.1016/j.dci.2007.12.008; PMID: 18262264
- Pillet S, Kobasa D, Meunier I, Gray M, Laddy D, Weiner DB, von Messling V, Kobinger GP. Cellular immune response in the presence of protective antibody levels correlates with protection against 1918 influenza in ferrets. Vaccine 2011; 29:6793 - 801; http://dx.doi.org/10.1016/j.vaccine.2010.12.059; PMID: 21211587
- Seder RA, Darrah PA, Roederer M. T-cell quality in memory and protection: implications for vaccine design. Nat Rev Immunol 2008; 8:247 - 58; http://dx.doi.org/10.1038/nri2274; PMID: 18323851
- Atsmon J, Kate-Ilovitz E, Shaikevich D, Singer Y, Volokhov I, Haim KY, Ben-Yedidia T. Safety and immunogenicity of multimeric-001--a novel universal influenza vaccine. J Clin Immunol 2012; 32:595 - 603; http://dx.doi.org/10.1007/s10875-011-9632-5; PMID: 22318394
- Smith LR, Wloch MK, Ye M, Reyes LR, Boutsaboualoy S, Dunne CE, Chaplin JA, Rusalov D, Rolland AP, Fisher CL, et al. Phase 1 clinical trials of the safety and immunogenicity of adjuvanted plasmid DNA vaccines encoding influenza A virus H5 hemagglutinin. Vaccine 2010; 28:2565 - 72; http://dx.doi.org/10.1016/j.vaccine.2010.01.029; PMID: 20117262
- Jones S, Evans K, McElwaine-Johnn H, Sharpe M, Oxford J, Lambkin-Williams R, Mant T, Nolan A, Zambon M, Ellis J, et al. DNA vaccination protects against an influenza challenge in a double-blind randomised placebo-controlled phase 1b clinical trial. Vaccine 2009; 27:2506 - 12; http://dx.doi.org/10.1016/j.vaccine.2009.02.061; PMID: 19368793
- Landry N, Ward BJ, Trépanier S, Montomoli E, Dargis M, Lapini G, Vézina LP. Preclinical and clinical development of plant-made virus-like particle vaccine against avian H5N1 influenza. PLoS One 2010; 5:e15559; http://dx.doi.org/10.1371/journal.pone.0015559; PMID: 21203523
- Pedersen GK, Madhun AS, Breakwell L, Hoschler K, Sjursen H, Pathirana RD, Goudsmit J, Cox RJ. T-helper 1 cells elicited by H5N1 vaccination predict seroprotection. J Infect Dis 2012; 206:158 - 66; http://dx.doi.org/10.1093/infdis/jis330; PMID: 22551811
- Hubby B, Talarico T, Maughan M, Reap EA, Berglund P, Kamrud KI, Copp L, Lewis W, Cecil C, Norberg P, et al. Development and preclinical evaluation of an alphavirus replicon vaccine for influenza. Vaccine 2007; 25:8180 - 9; http://dx.doi.org/10.1016/j.vaccine.2007.09.038; PMID: 17961878
- Taylor DN, Treanor JJ, Sheldon EA, Johnson C, Umlauf S, Song L, Kavita U, Liu G, Tussey L, Ozer K, et al. Development of VAX128, a recombinant hemagglutinin (HA) influenza-flagellin fusion vaccine with improved safety and immune response. Vaccine 2012; 30:5761 - 9; http://dx.doi.org/10.1016/j.vaccine.2012.06.086; PMID: 22796139
- Taylor DN, Treanor JJ, Strout C, Johnson C, Fitzgerald T, Kavita U, Ozer K, Tussey L, Shaw A. Induction of a potent immune response in the elderly using the TLR-5 agonist, flagellin, with a recombinant hemagglutinin influenza-flagellin fusion vaccine (VAX125, STF2.HA1 SI). Vaccine 2011; 29:4897 - 902; http://dx.doi.org/10.1016/j.vaccine.2011.05.001; PMID: 21596084
- Turley CB, Rupp RE, Johnson C, Taylor DN, Wolfson J, Tussey L, Kavita U, Stanberry L, Shaw A. Safety and immunogenicity of a recombinant M2e-flagellin influenza vaccine (STF2.4xM2e) in healthy adults. Vaccine 2011; 29:5145 - 52; http://dx.doi.org/10.1016/j.vaccine.2011.05.041; PMID: 21624416