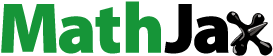
ABSTRACT
The independent and cumulative fission product yields (FPYs) and their covariance matrices were newly evaluated for thermal- and fast-neutron induced fission and spontaneous fission. We principally evaluated the independent isobaric charge distributions by taking account of available experimental data, especially putting emphasis on the shell and even-odd staggering in the fission yields by applying a Boltzmann factor including the shell and the pairing correction energies for the mass of fission products. Furthermore, the isomeric ratios of FPY were obtained by a systematic calculation based on the Hauser-Feshbach theory. Normalization of the FPY and associated covariance matrices of independent FPY were determined based on the generalised least-square method to make them consistent with important physical constraints. Especially, the FPYs were normalized to be consistent with the mass chain yield of England and Rider. The cumulative FPYs were calculated by following the relevant -decay chain by using the decay data from JENDL/DD-2015. Finally, we verified the FPYs evaluated presently by considering experimental decay heats, delayed neutron yields, aggregate antineutrino spectra, and results from post-irradiation examination. All the results have shown that the present set of FPYs were consistent with these data and free from trivial problems present in JENDL/FPY-2011.
1. Introduction
A reliable fission product yield (FPY) data with information on their uncertainty, in the form of evaluated nuclear data library, have great importance to conduct various numerical simulations that aim to quantify the inventory of spent nuclear fuel, develop next-generation reactors, mature transmutation technology, assess their safety, and so on. The evaluation for the current JENDL Fission Product Yields Data File 2011 (JENDL/FPY-2011) [Citation1] had been done by slightly modifying those stored in ENDF/B-VI [Citation2] to make the cumulative fission yield to be consistent with JENDL FP Decay Data File 2011 (JENDL/FPD-2011) [Citation1]. It contained the independent and cumulative FPY for thermal-, fast-, and 14 MeV neutron induced and spontaneous fission. These evaluations were carried out mostly in 1980s–2000s [Citation1,Citation3] and only a few modifications and updates have been adopted since then [Citation4–6]. Also, recent increase of demands in uncertainty information from many nuclear application fields for V&V (Verification and Validation) purposes stimulates the need for covariance matrices in the FPY data, which are absent in any of the currently available FPY library.
Despite many decades of progress in nuclear theories [Citation7] and models [Citation8–10], no complete and cohesive theory nor model exists so far that can estimate FPYs with sufficient accuracy as the level that is required from the nuclear applications. Particularly, the isobaric charge distribution and the isomeric yield ratio (IR) have been a long-standing problem for FPY data evaluation [Citation11,Citation12]. In order to complement the FPY data where FPY is experimentally unmeasured, some simplified models, mostly based on phenomenology, have been utilized in the past.
These models, which have been developed more than two decades ago, are quite successful and have been used for the estimation of unmeasured independent FPY. For instance, the independent FPY data libraries in later than ENDF/B-VI [Citation3] have adopted the independent FPY derived from the empirical formula proposed by Wahl [Citation13,Citation14], known as Wahl systematics, which generates the independent FPY including the full mass, charge, and individual isomer yield. The isomer ratios (IRs) were taken from a model with a simple formula proposed by Madland and England [Citation15] that is based on the spin and parity of relevant combination of nuclei. The FPY data obtained by using such models were normalised to the (reliable) mass chain yield evaluated by England and Rider [Citation3]. The JENDL/FPY-2011 library [Citation1] had been prepared basically as a copy from the FPY data in ENDF/B-VI as pointed out above. The JEFF-3.1.1 FPY library [Citation16] incorporates the evaluation activity of UKFY2 [Citation17] and UKFY3.6A [Citation16,Citation18] library, which also used the Wahl Systematics [Citation14]. Here, it is important to notice that no legitimate activity existed up to date in Japan to set up originally-evaluated FPY data library.
After decades, through technological, experimental, and theoretical advances, experimental fission studies have been improved dramatically [Citation19]. In spite of that, the empirical parametrizations used in modelling FPY have not been updated in a practical manner. For instance, isobaric charge distributions of independent FPY subsequently deviate from the simple Gaussian distribution. Such deviations are caused by staggering of stability of nuclei and are regarded as the shell- and odd-even effects. However, the shell and odd-even effects and isomeric ratio have been estimated based on rather phenomenologically in Wahl’s systematics [Citation14] by introducing simple multiplicative parameters which were determined to reproduce experimental independent FPY. Information on covariance matrices is not available as well in evaluated FPY nuclear data libraries. It is therefore necessary that the empirical parametrization used in such models should be replaced based on latest experimental and theoretical knowledge to extract systematical trends both in FPY and their covariances for purpose of extrapolation to unmeasured domain.
In order to take into account the current knowledge on experiment and nuclear theoretical model, an alternative approach for the FPY data evaluation is necessary. This requirement leads to the ideas to incorporate (1) the odd-even effect, (2) the shell effect, (3) a method to estimate yields for individual isomeric states, and (4) covariance information that contains physically sound relations to several physical constraints.
In this work, we evaluate FPY data for thermal- and fast-neutron induced fission and spontaneous fission for fissioning systems where such data exists in JENDL/FPY-2011 library. For this sake, we propose a new method that incorporates the odd-even effect and the shell effect on the isobaric charge distribution of independent FPY as a form of Boltzmann factor calculated with the shell correction energies, which are estimated by theoretical mass formula proposed by Koura et al. [Citation20]. We also utilize the Hauser-Feshbach theory to estimate the IR and generate covariance matrices for FPYs based on the Generalized Least-Square (GLS) method [Citation21]. To establish the new evaluation method by combining with the theoretical and experimental knowledge, we survey the latest experimental FPY data from EXFOR database [Citation22,Citation23] and analyze the experimental uncertainty. Thus, we generated a new evaluated FPY data for the independent and cumulative fission yields. Furthermore, we examined reliability of the newly-evaluated independent FPY data by calculating decay heats (DH), delayed neutron yields (DN), and aggregate antineutrino spectra (AS). In addition, the new FPY evaluation was further examined by burn-up simulations to be compared with the post-irradiation examination (PIE) data.
In this paper, we describe the method of evaluation of independent fission product yields in Chapter 2 and generation of the covariance matrices in Chapter 3. In Chapter 4 we validate the resultant FPY by a comparison with cumulative yield data, and by using the integral data such as DH, DN, AS, and PIE. The conclusion will be given in Chapter 5.
2. Evaluation method
2.1. Evaluation of independent fission product yields
The independent fission product yields for the population of a nucleus having charge number , mass number
and indicator
for isomeric state (
=0 for the ground and
=1 for the first isomeric state, and so on) is denoted as
. The first thing we did was a systematic survey of experimental data in EXFOR database [Citation22,Citation23]. We prepared a Perl script to extract only useful information after we downloaded the fission yield-related entries from EXFOR. As a result, we have extracted 25,670 data points including 3,150 independent FPY data. This must be compared with 14,710 data points used by Mills for the evaluation of UKFY3.6A [Citation16,Citation18]. We did not use such data as ‘ratio to ratio’ since handling of such data requires much richer experience in this field. We recognise that such data can be utilized in the future. For each data point characterized by
,
and
, we obtained the weighted average of experimental data by the least-squares method. These averages of experimental values for specific
were adopted in the evaluation if the data exist. For the unmeasured fissioning systems or data points, we took the
data in the evaluated nuclear data libraries, i.e. JENDL/FPY-2011 and JEFF-3.1.1, as the ‘pseudo’ experimental data. The ‘real’ and ‘pseudo’ experimental data were used for subsequent parameter fitting to be described below.
In most mass numbers of the fission products, the isobaric charge distribution fluctuates by the shell and odd-even effects that enhance the yields for fission products having even charge and/or neutron numbers compared to odd ones. Such a general trend is illustrated in . This feature characterizes the general trend in the FPY, so it must be taken into consideration with great care.
Figure 1. Schematic view of the charge distribution at even isobar.
is the most provable charge estimated from the unchanged charge distribution (UCD) assumption,
is the most probable charge derived from experimental data, and
, known as charge polarization, is difference of them
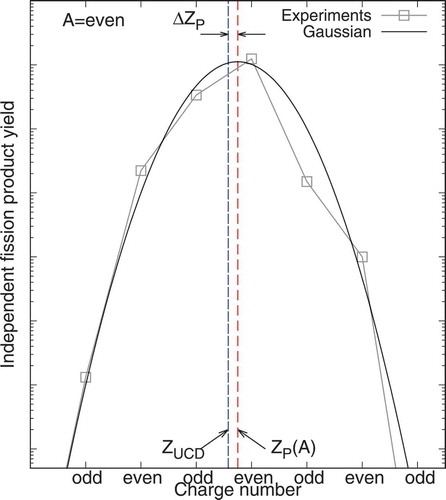
According to the scission point model of Wilkins et al. [Citation24], the independent yields as a function of the mass and proton numbers can be calculated based on a Boltzmann factor using the potential energy at the scission point as
where is a liquid-drop potential energy,
is a damping factor for the shell and pairing effects as a function of the excitation energy
, and
is a temperature of the fission product. The quantity
denotes the shell and pairing-correction energy to the potential. We presume that the right hand side of EquationEq. (1)
(1)
(1) can be approximately factorized into three parts: (1) mass yield distribution
, (2) normalized Gaussian distribution for
on each isobar
, (3) a correction factor to account for shell- and even-odd staggering
. We further introduced the fourth factor for the branching ratio to isomeric state
(=ground state or isomer)
, which is absent in Wilkins’ consideration. Then,
can be calculated as the product of these four factors as
where denotes isobaric yield for mass number
,
and
denote the width and the most-probable charge of Gauss distribution for the isobaric charge distribution,
is the shell and pairing correction energy, and
which corresponds to
in Equationeq.(1)
(1)
(1) is the dumping energy that defines the extent for washing out of the shell and pairing effects. The parameter
was adjusted for each mass number
of fission product to reproduce the odd-even staggering or distortion from the Gaussian distribution. We employ the Boltzmann-factor type of weighted
stemming from Equationeq. (1)
(1)
(1) to represent the fine structure of the isobaric charge distribution whereas the
model of Wahl has a simple product of factors which depends only on evenness or oddness of
and
.
The shell correction energy includes 2 terms. The first one is the shell correction energy taken from the KTUY mass formula [Citation20]. We used this since it is known that KTUY formula gives one of the best reproduction of nuclear ground-state masses among many nuclear mass formulae. The second term is the pairing energy, and we adopted a simple form as
The total shell correction energy is a simple sum of these terms as .
We determined the parameter to reproduce the staggering in
distribution by fitting to the ‘real’ and ‘pseudo’ experimental data. The branching ratio to isomeric state
,
, was calculated by the Hauser-Feshbach theory for nuclides that possess known isomeric states. For
U(n
,f),
were taken from Ref [Citation12]., and TALYS-1.9 [Citation25] calculations were performed for the rest of all the fissioning systems.
After adopting three factors, are normalized to the reliable mass-chain yield
evaluated by England and Rider [Citation3]. Here, we have to notice that there exists a small difference between
and
since the latter includes the effect of delayed-neutron emission during the
-decay process, which is actually a small correction. The
data of England and Rider were used as the initial guess of
, and the small difference was corrected afterwards when the whole (pre-)evaluated data were put into the GLS analysis as will be described later. Unlike the Wahl systematics, our method directly calculates
without subtracting the prompt neutron multiplicity
. The effect of prompt neutron emission was instead corrected in the process of the GLS process.
shows the list of fissioning systems prepared by our new evaluation method. Note that we evaluated FPYs for thermal-, fast-neutron induced, and spontaneous fission.
Table 1. List of the fissioning systems prepared by the new evaluation method described in this paper. Thermal and Fast denote FPY for thermal- and fast- neutron induced fission whereas Spontaneous denotes spontaneous fission. The sign ”✓” in the covariance column denotes that covariance data were evaluated for the corresponding fissioning system
2.2. Evaluation of covariance matrices
Covariance information has been recognized to be more and more important for V&V purpose of computations in nuclear technologies. Such information is especially important for FPY, since it should obey some important physical constraints such as conservation of (1) mass number , (2) charge number
. Similarly, (3) the sum of FPY must be normalized to 2.0 excluding the light-charged particles coming from the ternary fission, and (4) the sum of the heavy FPY must be normalized to 1.0. Finally, the most important constraint is that (5) the mass chain yields
and its uncertainty must converge to those given by England and Rider [Citation3], in which
and their uncertainties were carefully and extensively evaluated as the reference data. This condition determines the quantity
. This constraint is very important for FPYs since the errors in final mass-chain yield are normally very small as given by England and Rider [Citation3], while those for each
are much larger. Therefore, simple error propagation ignoring off-diagonal elements in the covariance matrix does not converge to the small uncertainties in the
given by England and Rider. All these five constraints can be summarized as an observation equation
which is linear in the FPY, where
is one of the above constraints,
denotes the sensitivity matrix, and
the vector of
. The five constraints we employed in the generalized least-square (GLS) procedure [Citation21] are given in more detail in the followings:
(1) Conservation of mass number
The number of nucleons in a fissioning system should be conserved as , where
,
and
are the proton and the nucleon numbers of the
-th fission product and the latter of the compound nucleus.
is the average mass number of light-charged particles from ternary fission such as proton, triton, and
particles, respectively. Here
stands for the prompt neutron multiplicity. The
and
data were primarily taken from the compilation of England and Rider [Citation3]. See for more details.
(2) Conversation of charge number
The numbers of the protons in a fissioning system should be conserved as , where
,
and
are the charge numbers of the fission product, the compound nucleus, and average Z value of the light-charged particles, respectively. The last quantity was taken from the compilation of England and Rider [Citation3]. See for more details.
(3) Normalization of the independent yields
The sum of should be 2.0 by its definition as
, where
denotes
.
(4) Normalization of heavier mass yields
The sum of the independent yields for the heavier fission products should be 1.0 as .
(5) Calculated mass-chain yield based on and their uncertainties should be consistent with
from the England-Rider’s evaluation and the associated uncertainty.
In this work, we estimated the covariance matrices of using the GLS method [Citation21] under the 5 constraints mentioned above. We started from diagonal prior covariance matrices
with diagonal elements taken from experimental error of
if available, or the error estimated from those based on JENDL/FPY-2011 or JEFF-3.3/FY library which is improved by introducing UKFY3.7 [Citation26] results were used as ‘pseudo’ experimental data otherwise. See for more details. In the GLS, updated posterior information can be calculated as,
where is the vector containing updated FPY data
which were adjusted to fulfil the five constraints. The symbol
denotes the diagonal prior covariance matrix as explained above. In order to apply the GLS method, we set fairly small error (
) to the first four constraints concerning to number and yield normalizations. After the GLS update process, we obtain updated yields and associated covariance matrices which are consistent with the above constraints. shows the correlation among
at
=102 and 103 for the
U(n
,f) system. Here we notice that negative correlations (denoted by blue color) appear among
for the same
. A small correlation can be found between different mass numbers. This is caused by
-delayed neutron emission which changes the mass number by 1 unit.
Figure 2. The correlation matrix of from A = 102 to A = 103 for thermal-neutron induced fission in
U. Both axes correspond to the mass number
, the charge number
, and the index for meta-stable state
or
of the fission products. Positive and negative correlations are mapped by red and blue, respectively
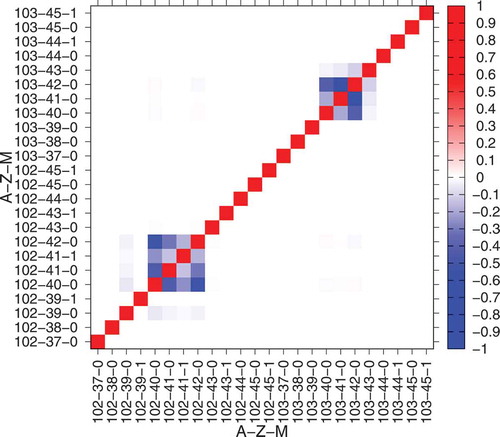
displays correlation matrices for mass distributions of independent yields. We see here also negative off-diagonal components coming from the conservation laws.
Figure 3. Correlation matrices of for thermal- (upper panels) and fast- (lower panels) neutron induced fission of selected nuclides
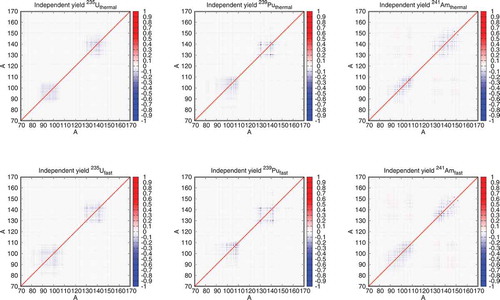
As shown in , the present evaluation gives the error for the cumulative yield which converges to that of England and Rider [Citation3], while the simple sum of errors (without correlation) is diverging.
Figure 4. Comparison of the errors in the independent and cumulative yields of U(n
). The thin-broken line shows the diagonal element of the error given in JENDL/FPY-2011 for the independent yield. The thick broken line shows the cumulative yield obtained in the present work, while the thinner band surrounding it shows the error of corresponding cumulative yield. The dark band shows error in the cumulative yield obtained by error propagation from the covariance of independent yield but by discarding off-diagonal elements. The horizontal dot-dashed line with a gray band shows the mass-chain yield and its uncertainty given by England and Rider [Citation3]
![Figure 4. Comparison of the errors in the independent and cumulative yields of 235U(n th,f). The thin-broken line shows the diagonal element of the error given in JENDL/FPY-2011 for the independent yield. The thick broken line shows the cumulative yield obtained in the present work, while the thinner band surrounding it shows the error of corresponding cumulative yield. The dark band shows error in the cumulative yield obtained by error propagation from the covariance of independent yield but by discarding off-diagonal elements. The horizontal dot-dashed line with a gray band shows the mass-chain yield and its uncertainty given by England and Rider [Citation3]](/cms/asset/bc49d72c-05db-4110-843e-a8b5ae8d5f48/tnst_a_1813643_f0004_oc.jpg)
In , errors of chain yield for
U(n
,f) (left panel) and
Pu(n
,f) (right panel) calculated with the GLS method are shown as ratios to those given by England-Rider’s evaluation [Citation3]. It clearly shows that, if we do not take account of the correlation properly, the error of the mass chain yield is overestimated to a great extent as shown by diamonds, whereas inclusion of the off-diagonal elements in correlation matrices make the error of the mass chain yield compatible to those of England and Rider (circles).
Figure 5. Comparison of the ratio between the calculated errors C and those evaluated by England and Rider E, C/E, in the mass-chain yield, as the function of the mass of fission product for
(left panel) and
(right panel) reactions. The filled circles show results when the full covariance matrices are taken into account in the mass-chain calculation, while the open diamonds are obtained when only the diagonal components are used discarding off-diagonal correlation
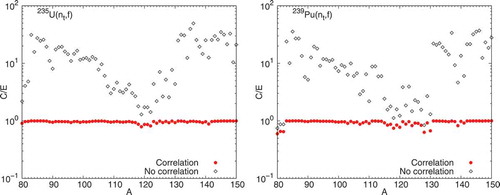
In the same manner, we evaluated the covariances of all fissioning systems as marked by ✓ in .
3. Results and discussion
3.1. Evaluated independent fission yield
shows the mass distribution of independent fission products, namely, , exhibiting that the present evaluation gives essentially the same mass distributions as JENDL/FPY-2011 since both of them were normalized to the same mass chain yield. In contrast, as shown in , the present
as a function of charge
are different from those of JENDL/FPY-2011 for
U(n
,f) and
Pu(n
,f). Therefore, distribution of elements in the spent nuclear fuel changes noticeable in some fissioning systems. This is due to the fact that we replaced
by the experimental data or their least-squares average where such data are available, and no common normalization was applied for the
distribution other than the constraint for their sum. The presently evaluated
distributions are, on the other hand, almost identical to those in JENDL/FPY-2011 for such fissioning systems as
Am(n
) where experimental data are scarce. Even though small difference in the
were brought to some of the important nuclei, we verified, as will be shown later, that the present evaluation is consistent with many integral data.
Figure 6. The mass distributions of independent FPY for thermal- (upper panels) and fast- (lower panels) neutron induced fission for U (left),
Pu (middle) and
Am (right). The presently evaluated data (filled circles) are compared with those of JENDL/FPY-2011 (open squares)
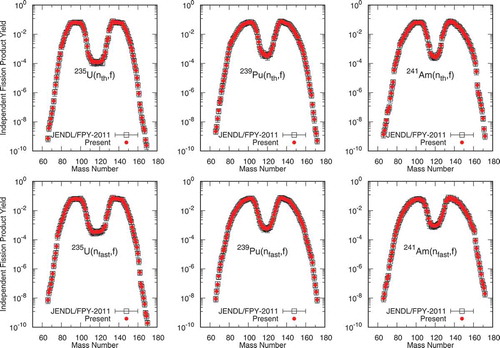
shows the isobaric charge distributions for ,
,
, and
obtained in the present work for
U(n
,f) (filled circles connected by solid lines) compared with the data in JENDL/FPY-2011, JEFF-3.1.1, experimental data, and the least-squares average of the experimental data. The present
for each mass deviates from a pure Gaussian distributions which are given as dashed curves. This is due to the
factor in Equationeq. (2)
(2)
(2) which enhances yields for even-even nuclei while hindering those for odd-odd ones in these even-mass chains. In general, our new result can successfully reproduce the overall trend of the experimental data. Please notice that the unnatural bumps seen for
in
,
for
and
for
in JENDL/FPY-2011 are removed in the present evaluation. Also, please notice that the difference between the present data and those in JENDL/FPY-2011 can be in a few orders of magnitude for some points, but mostly at peripheral region where FPY is not large.
Figure 8. The isobaric charge distributions for at
,
,
, and
from
U(n
,f). The present evaluation (filled solid circles) is plotted together with the experimental data (diamonds), the experimental weighted average (filled squares), the evaluated data in JENDL/FPY-2011 (dash-dotted line), and JEFF-3.1.1 (thin broken line), and the pure Gaussian distributions without odd-even effects (broken line)
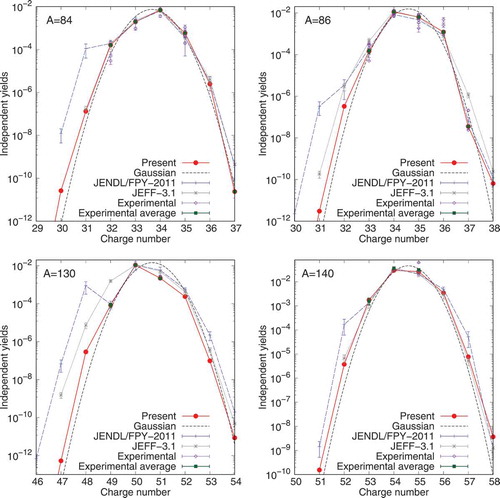
A comparison of all the independent fission product yields for the
U(n
,f) system stored in JENDL/FPY-2011 and the present evaluation and their ratios are shown in . The changes from the JENDL/FPY-2011 to the present evaluation are considerable for some nuclides, while many of them are small enough or unchanged.
3.2. Validation of the present evaluation
To ensure that the present are reliable for use in various application fields, we validated them by calculating the following quantities.
• Cumulative fission product yield by tracking successive chains of
-decay
• Delayed neutron yields (DN), FP decay heat (DH), and aggregate antineutrino spectra (AS) by the summation calculation method
• Fuel burn-up simulations
All the results were carefully compared with the available experimental data. Methods of calculation and simulation are briefly described below and the results are detailed in the following sections.
The cumulative fission product yield were calculated from
using JENDL Decay Data File 2015 (JENDL/DDF-2015) [Citation27] by tracking all the decay paths until each fission product reaches to the stable one. Then, we calculated the delayed neutron yields (DN) and the decay heat (DH) for
U, and the FP antineutrino spectra (AS) for four major fissioning systems from
U,
U,
Pu, and
Pu. For this purpose, we utilized the summation calculation method [Citation28] which solves the Bateman equation on the basis of the decay data from JENDL/DDF-2015. The results of the DN and DH calculations were compared with experimental data parameterized by Keepin et.al [Citation29] and those measured at ORNL [Citation30], Lowell [Citation31] and at Yayoi [Citation32], respectively. The results of AS are compared with experimental data by Schreckenbach Group [Citation33–36]. We further examined the present
by reactor-fuel burn-up simulations using ORIGEN2 code [Citation37] with the present and the JENDL/FPY-2011 FPY and ORJLIB40 [Citation38] as the neutron cross-sections. The calculated results are compared with the post-irradiation examination (PIE) data of UO
fuel irradiated at Mihama-3 [Citation39] and Takahama-3 [Citation40,Citation41] PWR reactors. The PIE data, burn-up histories, and fuel compositions were taken from the SFCOMPO-2.0 [Citation42] database.
3.2.1. Cumulative fission product yield
The newly evaluated independent fission yields, were converted into the cumulative yield
to be compared with the existing experiment-based data. shows the calculated cumulative yield
for the
U(n
) being compared with the data from JENDL/FPY-2011 for some selected elements from light and heavy fission products. It appears that the most of
agreed fairly well with each other in the % order of magnitude, whereas yields for some nuclei, e.g.,
Br,
Br,
Br,
Kr,
Kr,
Kr,
Te,
Te,
I, and
Xe, show large discrepancies. These nuclei are far from the stability line and
strongly depend on
in the less-stable, left-side region, where uncertainty is remarkably large due to small yield that makes measurements difficult. In general, the presently evaluated data gives curves that are smoother than those from JENDL/FPY-2011. The decay data library used for the JENDL/FPY-2011 evaluation was JENDL FP Decay Data File 2011 (JENDL/FPD-2011) [Citation1]. Thus, the cause of the difference between new evaluation and the JENDL/FPY-2011 data seems to be originated from both
and decay data library.
Figure 10. Comparison of the cumulative yields between the present evaluation (solid lines) and those taken from JENDL/FPY-2011 (broken lines) for selected nuclides from thermal-neutron induced fission of U. Only
for the ground state (
=0) are plotted
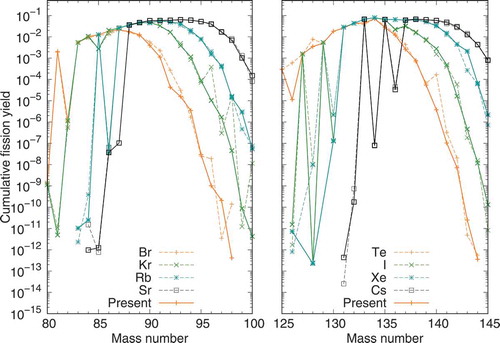
A full comparison of JENDL/FPY-2011 and the calculated of
U(n
,f) are shown in as well as the ratios of the JENDL/FPY-2011 values to the present results. The changes are modest and many of them are unchanged compared to
because many of nuclides decay to the stable nuclides.
3.2.2. Decay heat, delayed neutron, and antineutrino spectra
We examined the validity and the reliability of the new by calculating DN, DH, and AS using the summation calculation method [Citation28] and by comparing them with experimental data.
The calculated DN yield after 0.4 to 10 seconds from a burst fission in
U
reaction is shown in as a solid line compared to the experimental data analyzed by Keepin et al. [Citation29] (open squares). The dotted line was obtained by ignoring contributions from the isomeric states. The FPY of some fission products and IR are known to have a large effect on DN. The broken line exhibits a result obtained with JENDL/FPY-2011, which overpredicts the DN yield at short cooling time that leads to overestimation of the total
noticeably. As shown in , the presently evaluated data significantly improve reproducibility for delayed neutron yield by adopting IR calculated by the Hauser-Fesbach theory [Citation12,Citation25] in our new FPY evaluation.
Figure 12. Calculated delayed neutron yield (DN) from burst U(n
,f) reaction as a function of cooling time. Solid and dotted lines correspond to the results with or without isomer ratio calculated by the Hauser-Feshbach calculations, respectively. Broken line exhibits results by JENDL/FPY-2011. Experimental DN analyzed by Keepin et.al [Citation29] are also plotted as open squares. An error of 5% was temporarily assigned to the Keepin’s results
![Figure 12. Calculated delayed neutron yield (DN) from burst 235U(n th,f) reaction as a function of cooling time. Solid and dotted lines correspond to the results with or without isomer ratio calculated by the Hauser-Feshbach calculations, respectively. Broken line exhibits results by JENDL/FPY-2011. Experimental DN analyzed by Keepin et.al [Citation29] are also plotted as open squares. An error of 5% was temporarily assigned to the Keepin’s results](/cms/asset/576f609e-8414-4609-9f9c-a4f881d66a54/tnst_a_1813643_f0012_oc.jpg)
shows the -ray (bottom panel) and the
-ray (top panel) components of DH after a burst fission in
U. The results calculated for thermal-neutron induced fission in
U show overall good agreement with the experimental data measured at ORNL [Citation30], Lowell [Citation31] and at Yayoi [Citation32]. Though Yayoi is a small fast reactor with its neutron-flux peaking around 500 keV, the difference between the thermal- and the fast-neutron induced fission in this cooling-time region has been calculated to be less than 2
3% and measurements support this except the
-ray component in 300
6000s cooling which will be discussed below. The calculations using the present and the JENDL/FPY-2011 yields well reproduce the three experiments almost within the experimental error bars except for the
component in the ORNL data from 300 to 6000s. It is known that DH calculation is often affected by the famous Pandemonium problem which may be present in the decay-data [Citation43]. However, combination of the JENDL decay data and present FPY data is hardly affected by it. It should be noted that there is no recent experiment nor calculation which supports the ORNL data among others in this particular cooling-time range.
Figure 13. Calculated -ray (lower) and
-ray (upper) components of the decay heat (DH) after a fission burst in
U compared with experimental data. The calculations are based on the present
(solid line), JENDL/FPY-2011 (dashed line), and experimental data from ORNL [Citation30], Lowell [Citation31] and Yayoi [Citation32]. Note that the vertical axis stands for the decay power (MeV/s) multiplied by the cooling time (s) for concise display on the linear s
![Figure 13. Calculated β-ray (lower) and γ-ray (upper) components of the decay heat (DH) after a fission burst in 235U compared with experimental data. The calculations are based on the present YI(Z,A) (solid line), JENDL/FPY-2011 (dashed line), and experimental data from ORNL [Citation30], Lowell [Citation31] and Yayoi [Citation32]. Note that the vertical axis stands for the decay power (MeV/s) multiplied by the cooling time (s) for concise display on the linear s](/cms/asset/d5fc59e7-dfef-4a9f-9cea-6bb459dca4e3/tnst_a_1813643_f0013_oc.jpg)
Antineutrinos emitted from an aggregation of -decaying FP nuclides provide us with another good benchmark for FPY data validation. A typical form of this is the so-called reactor antineutrino. We calculated AS from four fissioning nuclides under neutron irradiation after the method used in Ref [Citation44]. compares the calculated results with experiment-based AS which were converted from measured spectra of simultaneously emitted electrons that share the
-transition energy only with antineutrinos. The electron measurements were done for
U by Shreckenbach et al. [Citation33],
U by Haag et al. [Citation34],
Pu by Feilitzsch et al. [Citation35] and for
Pu by Hahn et al. [Citation36]. Here we adopted the AS converted by Mueller et al. [Citation45] instead of the old conversions by the original authors themselves except for
U for which no recently improved conversion exists. The agreement achieved by using the present FPYs (solid lines) looks better than the calculation based on JENDL/FPY-2011 (broken lines). It should also be noted that the differences among fissioning nuclides are well followed by the calculations which come only from differences in FPY data. The good agreement between the calculation and the experiments supports the reliability of the present FPY evaluation especially for high Q
-valued odd-odd nuclides [Citation46].
3.2.3. Validation by burn-up calculations
We conducted a fuel burn-up simulation of power reactors to obtain the spent-fuel FP compositions using the new installed in ORIGEN-2 code [Citation37,Citation38] and compared them with the PIE results from Takahama and Mihama power plants. shows the ratio between the simulated and the PIE results. They are shown in C/E
(%) with the error bars which come from experimental errors for the cases where they are available. In general, both the results based on the present
and the JENDL yields show the same level of agreement with the PIE data. However, the C/E
for Sm isotopes in the Takahama-3 () simulation are improved compared to the case when JENDL/FPY-2011 is used. The C/E
in the Mihama-3 () tend to follow JENDL/FPY-2011 trends. Here, it is important to notice that the reproductivity of burn-up calculations are crucial for the reactor applications and we can conclude that the present
has enough capability for these aims.
Figure 15. Ratio of calculation to experimental (C/E) of post-irradiation examinations (PIE) of UO fuel irradiated at Mihama-3 [Citation39] and Takahama-3 [Citation40,Citation41]. The squares show the burn-up calculation results obtained by using JENDL/FPY-2011, and filled circles show the results using the present FPY data. The error bar shows the experimental error
![Figure 15. Ratio of calculation to experimental (C/E) of post-irradiation examinations (PIE) of UO 2 fuel irradiated at Mihama-3 [Citation39] and Takahama-3 [Citation40,Citation41]. The squares show the burn-up calculation results obtained by using JENDL/FPY-2011, and filled circles show the results using the present FPY data. The error bar shows the experimental error](/cms/asset/6b704664-e0c9-494a-b9ad-b888f96e11a1/tnst_a_1813643_f0015_oc.jpg)
4. Conclusion
We evaluated the independent fission product yields and produced a reliable set of , associated covariance matrices, and prepared those corresponding to cumulative fission yield data for 49 fissioning systems as the first and full legitimate activity in Japan in order to accomplish an original and complete data set of FPYs. The evaluation carried out here reflects the most recent experimental data and includes a novel shell- and pairing-correction methodology as well as the covariance matrices being prepared elaborately. In the calculation of the isomeric ratio, we got rid of the phenomenology which had been used in most of past FPY evaluations and introduced a modern nuclear statistical model. Our transition from phenomenology to models with solid physical basis as far as possible makes it much easier to effectively feed back the going progress in fission physics [Citation12,Citation47,Citation48] into the future revision of the FPY data library. Systematical trends for the model parameters we obtained in the present work will be published in a forthcoming paper, which will enable us to extend the present method to wider range of fissioning systems. Our present results can be incorporated into any evaluated nuclear data library for wide range of data users in a numerous numbers of technological and scientific application fields. It is to be noted that the presently evaluated FPY data are compiled in ENDF-6 format [Citation49] and to be adopted in the next version of JENDL FPY library.
Supplemental Material
Download PDF (13.5 MB)Acknowledgments
This work comprises results of the ”Research and development of an innovative transmutation system of LLFP by fast reactors”, entrusted to the Tokyo Institute of Technology by the Ministry of Education, Culture, Sports, Science and Technology of Japan (MEXT), and ”Development of a nuclear fuel cycle concept using reduced environmental load light water reactors” entrusted to Toshiba Energy Systems by the Ministry of Education, Cultures, Sports, Science and Technology of Japan (MEXT) under ”MEXT nuclear system research and development Program” and KAKENHI Grant Number 18K03642 from Japan Society for the Promotion of Science (JSPS).
Disclosure statement
No potential conflict of interest was reported by the authors.
Supplementary material
Supplemental data for this article can be accessed here.
Additional information
Funding
References
- Katakura J, J ENDLFP Decay data file 2011 and fission yields data file 2011. Japan Atomic Energy Agency; 2012. JAEA-Data/Code 2011-025.
- Dunford CL. Evaluated nuclear data file, ENDF/B-VI. In: Qaim SM, editor. Nuclear data for science and technology. Berlin, Heidelberg: Springer Berlin Heidelberg; 1992. p. 788–792.
- England TR, Rider BF Evaluation and compilation of fission product yields. Los Alamos National Laboratory; 1994. ENDF-349, LA-UR-94-3106.
- Kawano T, Chadwick MB. Estimation of 239Pu independent and cumulative fission product yields from the chain yield data using a Bayesian technique. J Nucl Sci Technol. 2013;50(10):1034–1042.
- Brown DA, Chadwick MB, Capote R, et al. ENDF/B-VIII.0: the 8th major release of the nuclear reaction data library with CIELO-project cross sections, new standards and thermal scattering data. Nuclear Data Sheets. 2018;148:1–142. Special Issue on Nuclear Reaction Data.
- Katakura J-I, Minato F, Ohgama K. Revision of the JENDL FP fission yield data. EPJ Web Conf. 2016;111:08004.
- Schunck N, Robledo LM. Microscopic theory of nuclear fission: a review. Rep Prog Phys. 2016 oct;79(11):116301.
- Schmidt K-H, Jurado B, Amouroux C, et al. General description of fission observables: GEF model code. Nucl Data Sheets. 2016;131:107–221. [ Special Issue on Nuclear Reaction Data].
- Ishizuka C, Usang MD, Ivanyuk FA, et al. Four-dimensional Langevin approach to low-energy nuclear fission of 236U. Phys Rev C. 2017;96:064616.
- Sierk AJ. Langevin model of low-energy fission. Phys Rev C. 2017 Sep;96(3):034603.
- Stetcu I, Talou P, Kawano T, et al. Isomer production ratios and the angular momentum distribution of fission fragments. Phys Rev C. 2013 Oct;88(4):044603.
- Okumura S, Kawano T, Jaffke P, et al. 235U(n, f) Independent fission product yield and isomeric ratio calculated with the statistical Hauser-Feshbach theory. J Nucl Sci Technol. 2018;1–15.
- Wahl AC. Nuclear-charge distribution and delayed-neutron yields for thermal-neutron-induced fission of 235U, 233U, and 239Pu and for spontaneous fission of 252Cf. At Data Nucl Data Tables. 1988;39(1):1–156.
- Wahl AC Systematics of fission-product yields. Los Alamos National Laboratory; 2002. LA-13928.
- Madland DG, England TR. The influence of isomeric states on independent fission product yields. Nucl Sci Eng. 1977;64(4):859–865.
- Kellett MA, Bersillon O, Mills RW The JEFF-3.1/-3.1.1 radioactive decay data and fission yields sub-libraries. OECD NEA; 2009. JEFF Report 20 OECD NEA.
- James MF, Mills RW, Weaver DR. A new evaluation of fission product yields and the production of a new library (UKFY2) of independent and cumulative yields. Prog Nucl Energy. 1991;26(1):1–29.
- Mills RW. Fission product yield evaluation: Appendix 5. to his PhD thesis, University of Birmingham; 1995.
- Andreyev AN, Nishio K, Schmidt KH. Nuclear fission: a review of experimental advances and phenomenology. Rep Prog Phys. 2017 nov;81(1):016301.
- Koura H, Tachibana T, Uno M, et al. Nuclidic mass formula on a spherical basis with an improved even-odd term. Prog Theor Phys. 2005 02;113(2):305–325. .
- Fiorito L, Stankovskiy A, den Eynde GV, et al. Generation of fission yield covariances to correct discrepancies in the nuclear data libraries. Ann Nucl Energy. 2016;88:12–23.
- Otuka N, Dupont E, Semkova V, et al. Towards a more complete and accurate experimental nuclear reaction data library (exfor): international collaboration between nuclear reaction data centres (NRDC). Nucl Data Sheets. 2014;120:272–276.
- Zerkin VV, Pritychenko B. The experimental nuclear reaction data (EXFOR): extended computer database and Web retrieval system. Nucl Instrum Methods Phys Res A. 2018;888:31–43.
- Wilkins BD, Steinberg EP, Chasman RR. Scission-point model of nuclear fission based on deformed-shell effects. Phys Rev C. 1976 Nov;14(5):1832–1863.
- Koning AJ, Rochman D. Modern nuclear data evaluation with the TALYS code system. Nucl Data Sheets. 2012;113(12):2841–2934. Special Issue on Nuclear Reaction Data. .
- Mills RW, Plompen A, Hambsch F-J. A new UK fission yield evaluation UKFY3.7. EPJ Web Conf. 2017;146:04008.
- Katakura J, Minato F JENDL decay data file 2015. Japan Atomic Energy Agency; 2016. JAEA-Data/Code 2015-030.
- Oyamatsu K, Takeuchi H, Sagisaka M, et al. New method for calculating aggregate fission product decay heat with full use of macroscopic-measurement data. J Nucl Sci Technol. 2001;38(7):477–487.
- Keepin GR, Wimett TF, Zeigler RK. Delayed neutrons from fissionable isotopes of uranium, plutonium and thorium. J Nucl Energy. 1957;6(1):IN2– 21.
- Dickens JK, Love TA, McConnell JW, et al. Fission-product energy release for times following thermal-neutron fission of 235U between 2 and 14 000 s. Nucl Sci Eng. 1980;74(2):106–129.
- Couchell GP, Campbell JM, Li S, et al. A study of gamma-ray and beta-particle decay heat following thermal neutron induced fission of 235U. In: Proceedings of the International Conference on Nuclear Data for Science and Technology, Vol. 2. La Grange park, IL, USA: American Nuclear Society, Inc.; 1994. p. 966–969.
- Akiyama M, An S Measurements of fission-product decay heat for fast reactors. In: Proceedings of the International Conference on Nuclear Data for Science and Technology. Dordrecht, Holland: Reidel Publication; 1982. p. 237–244.
- Schreckenbach K, Colvin G, Gelletly W, et al. Determination of the antineutrino spectrum from 235U thermal neutron fission products up to 9.5 MeV. Phys Lett B. 1985;160(4):325–330.
- Haag N, Gütlein A, Hofmann M, et al. Experimental determination of the antineutrino spectrum of the fission products of 238U. Phys Rev Lett. 2014 Mar;112:122501.
- von Feilitzsch F, Hahn AA, Schreckenbach K. Experimental beta-spectra from 239Pu and 235U thermal neutron fission products and their correlated antineutrino spectra. Phys Lett B. 1982;118(1):162–166.
- Hahn AA, Schreckenbach K, Gelletly W, et al. Antineutrino spectra from 241Pu and 239Pu thermal neutron fission products. Phys Lett B. 1989;218(3):365–368.
- Croff AG. ORIGEN2: a versatile computer code for calculating the nuclide compositions and characteristics of nuclear materials. Nucl Technol. 1983;62(3):335–352.
- Okumura K, Sugino K, Kojima K, et al. Set of ORIGEN2 cross section libraries based on JENDL-4.0; ORLIBJ40. JAEA-Data/Code 2012-032. 2013.
- Department of Chemistry JAEA Tokai Research Establishment. Dissolution study group in the department of chemistry, Dissolution Studies of Spent Nuclear Fuels; 1991.
- Nakahara Y, Suyama K, Agency TSJAE. Dissolution study group in the department of chemistry, Dissolution Studies of Spent Nuclear Fuels; 1991.
- Nakahara Y, Suyama K, Inagawa J, et al. Nuclide composition benchmark data set for verifying burnup codes on spent light water reactor fuels. Nucl Technol. 2002;137(2):111–126. .
- Michel-Sendis F, Gauld I, Martinez JS, et al. SFCOMPO-2.0: an OECD NEA database of spent nuclear fuel isotopic assays, reactor design specifications, and operating data. Ann Nucl Energy. 2017;110:779–788.
- Hardy JC, Carraz LC, Jonson B, et al. The essential decay of pandemonium: A demonstration of errors in complex beta-decay schemes. Phys Lett B. 1977;71(2):307–310.
- Yoshida T, Tachibana T, Okumura S, et al. Spectral anomaly of reactor antineutrinos based on theoretical energy spectra. Phys Rev C. 2018 Oct;98(4):041303.
- Mueller TA, Lhuillier D, Fallot M, et al. Improved predictions of reactor antineutrino spectra. Phys Rev C. 2011 May;83(5):054615. .
- Yoshida T, Tachibana T, Hagura N, et al. Composition, decomposition and analysis of reactor antineutrino and electron spectra based on gross theory of beta-decay and summation method. Prog Nucl Energy. 2016;88:175–182.
- Usang MD, Ivanyuk FA, Ishizuka C, et al. Correlated transitions in TKE and mass distributions of fission fragments described by 4-D Langevin equation. Sci Rep. 2019 Feb;9(1):1525.
- Ishizuka C, Zhang X, Usang MD, et al. Effect of the doubly magic shell closures in 132Sn and 208Pb on the mass distribution of fission fragments of superheavy nuclei. Phys Rev C. 2020 Jan;101:011601(R).
- Herman M, Trkov A ENDF-6 formats manual. Brookhaven National Laboratory; 2009. ENDF-102, BNL-90365-2009.