Abstract
Mercury (Hg) is naturally present in warm springs and mesothermal (orogenic) gold-bearing quartz vein systems in the South Island of New Zealand. Mercury amalgamation was used historically in ore processing at gold (Au) mines, resulting in composite natural and anthropogenic Hg signatures at these sites. This study compares natural Hg enrichment of the Au vein systems, residual anthropogenic Hg added for amalgamation, and enrichment of naturally present Hg during ore processing. Mercury concentration data are presented for solids and water at historic mine sites, the modern Macraes mine, fault-related warm springs, and zones of naturally occurring cinnabar and Hg-bearing Au. Arsenic (As) concentrations are also presented, as As is the most environmentally significant element in this tectonic setting. Tailings and processing residues at historic mine sites (Blackwater mine, West Coast; Golden Point and Golden Bar, Hyde-Macraes shear zone) contain up to 1000 mg/kg Hg, and in adjacent surface waters Hg is at or slightly above background from 0.6 to 0.8 ng/L. Relative to South Island Hg, As is more environmentally significant: solid wastes at some historic mine and mineral processing sites contain up to 30.5 wt% As due to enrichment of natural As in mineralised rocks. Shallow groundwater and processing waters at the modern Macraes mine are up to 0.01 mg/L Hg due to natural Hg in mineralised rocks, and no significant Hg elevation is evident in nearby surface waters, which are <1 ng/L Hg. Mercury concentration in historic mine residues is 103 to 104 times higher than primary ore, and Hg is disproportionally increased relative to As, indicating that much of the Hg was added during the amalgamation process. Natural cinnabar deposition from warm springs results in localised, strongly elevated Hg, equal to or less than the Hg contents in historic mine processing residues. Warm spring precipitates are up to 111 mg/kg Hg and waters are 0.3 µg/L Hg, comparable to data reported for active North Island geothermal (epithermal-style) systems. Natural Hg content in the Au-bearing vein systems results in minor elevation of dissolved Hg in some catchments. South Island Au occurs as an Au-Ag-Hg alloy, up to 8.4 wt% Hg. Cinnabar (HgS) occurs in mesothermal-style veins and as detrital grains in Quaternary gravels hosting Au-Ag-Hg alloy. The Nevis River catchment water, which locally flows through gravels containing cinnabar and Hg-bearing alluvial gold, contains up to 7 ng/L dissolved Hg. This is rapidly diluted to near 3 ng/L by low-Hg waters, and no effects of anthropogenic Hg addition near alluvial mining areas were detected; the dissolved Hg is likely derived from Hg-bearing gold. Mobilisation of Hg from study sites to downstream environments is at least partially controlled by solubility of cinnabar and elemental mercury.
Introduction
Mercury (Hg) is abundant in the epithermal environment, where hydrothermal systems are associated with volcanism and shallow plutonism. Geothermal systems and epithermal gold-bearing quartz veins can have high Hg contents in waters and precipitates (White et al. Citation1970; White Citation1981; Varekamp & Buseck Citation1984; Barnes & Seward Citation1997; Gray et al. Citation1997; Sherlock Citation2005). In contrast, collisional orogenic terranes lacking significant shallow igneous activity commonly contain mesothermal (orogenic) gold-bearing quartz vein systems with little or no Hg enrichment relative to host rock (Craw Citation2001).
Mercury amalgamation has been used historically to extract gold at quartz vein hosted gold mines in both epithermal and mesothermal environments and in downstream gold placer deposits (Hylander & Meili Citation2003). A significant proportion of this anthropogenically added Hg may be lost to the surrounding environment during the amalgamation process, and Hg-rich tailings are generally discarded at the mine site (Lacerda Citation1997). Mercury remaining from ore processing can be remobilised, posing a risk to aquatic and terrestrial ecosystems (USEPA Citation1997).
Previous studies of gold mine-related Hg pollution have focused on the naturally-elevated Hg of epithermal gold deposits (Webster-Brown & Craw Citation2005) and anthropogenically elevated Hg resulting from use of Hg amalgamation in placer deposits (Lechler et al. Citation1997; Roth et al. Citation2001; Miller et al. Citation2002; Veiga et al. Citation2006). There is little published information on Hg mobilisation around mesothermal (orogenic) Au mines from either natural or anthropogenic sources. It can be difficult to distinguish this Hg from other low-level anthropogenic sources such as atmospheric fallout from industrial activity (e.g. Pacyna & Pacyna Citation2002). In this study, we address the lack of information on Hg levels in collisional orogenic settings. We present the Hg contents of solids and waters from a wide range of environments in which elevated Hg occurs in the South Island of New Zealand (), which is an orogenic greywacke terrane. The region is sparsely populated, and it is well removed from major producers of atmospheric Hg (UNEP Citation2002). Local coal seams contain little mercury (Moore et al. Citation2005), and non-mining sources of anthropogenic mercury input are minimal.
Fig. 1 South Island of New Zealand, showing locations and types of Hg-bearing mineralised systems, including the sites examined in this study (Golden Bar, Golden Point, Macraes, and Blackwater mines; Nevis River, and the Hurunui warm springs). Inset: locations of North Island Hg-bearing epithermal systems (Northland, Taupo Volcanic Zone).
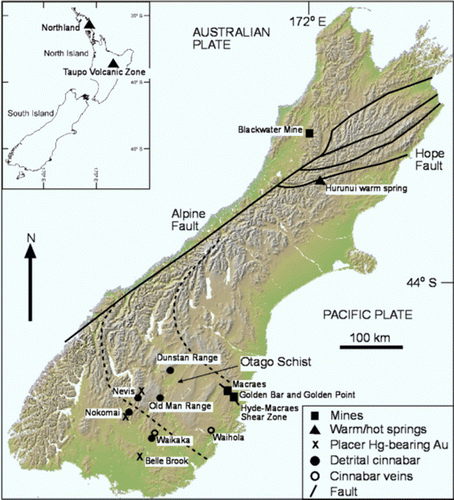
There are numerous mesothermal gold-bearing quartz vein systems in the South Island that have been mined historically, and Hg amalgamation was used at some of these during ore processing. We quantify the Hg concentration at these sites, comparing amalgamation-related Hg to natural Hg enrichment of the vein systems. We present mercury concentrations in conjunction with arsenic (As) concentrations in the same materials throughout our study, as As has been identified as the most environmentally significant element in this tectonic setting (Craw Citation2001). We also compare our South Island results with Hg and As contents of geothermal and epithermal settings in the North Island of New Zealand (), to emphasise the differences between these environments.
New Zealand tectonic setting and gold mineralisation
The two islands of New Zealand are made up of amalgamated Paleozoic and Mesozoic terranes, most of which are greywacke or schistose metamorphosed greywacke (Craw Citation2001). The currently active Pacific–Australian plate boundary traverses these terranes, causing renewed collisional tectonism. In the South Island, the plate boundary consists of the major oblique strike-slip Alpine fault, and a set of strike-slip faults in the northeast including the most active Hope Fault (). Deformation on these faults and related structures has resulted in a complex array of mountain ranges throughout the South Island (). Warm springs emanate from these strike-slip faults at sporadic intervals (Barnes et al. Citation1978). Farther northeast, the plate boundary is a subduction zone that lies immediately southeast of the North Island, and dips beneath the island. Volcanism related to this subduction activity has been active since the Miocene. There has been widespread hydrothermal activity and gold mineralisation associated with this volcanism (Cole Citation1979; Merchant Citation1986), and there are active geothermal systems in Northland and the Taupo Volcanic Zone (; White Citation1981; Davey & van Moort Citation1986; Craw et al. Citation2000; Pope et al. Citation2004).
The South Island greywacke terranes and schistose equivalents are hosts to swarms of mesothermal gold-bearing quartz veins that formed during Paleozoic and Mesozoic collisional tectonic activity, and similar vein-forming processes have been occurring along the present plate boundary since the Miocene (Craw Citation2001). Most of these vein systems have been of only minor economic significance, but two economically important deposits are examined here. The Blackwater mine was developed in a prominent and rich gold-bearing quartz vein in Buller Terrane Paleozoic metagreywackes on the west side of the South Island (; Christie & Braithwaite Citation2003). Several small deposits were developed historically along the Hyde-Macraes Shear Zone in the Otago Schist in the southeast of the island, and the large (6 million ounce) Macraes mine is currently active in this zone ().
Methods
Localities of particular interest in this study were selected in order to examine the wide range of geological settings in the South Island in which elevated Hg occurs. We have gathered new data for a warm spring locality (Hurunui) near the Hope Fault (), two historic mine processing sites (Golden Bar and Golden Point) on the Hyde-Macraes Shear Zone, and a historic mine processing site (Snowy River) at the Blackwater mine. We also collected a set of data for the Nevis River catchment in Otago Schist. The Nevis River contains detrital cinnabar and has been mined historically for alluvial gold, but is otherwise little modified by human activity. Additional observations were compiled from published and unpublished reports where mercury data were available, including mine process waters at the operating Macraes mine (Oceana Gold Ltd.), and both mineralised and unmineralised rocks in the Hyde-Macraes Shear Zone (Craw & Pacheco Citation2002; Pitcairn et al. Citation2006). The South Island data have been compared to North Island geothermal and epithermal mercury concentrations from other published works (White Citation1981; Davey & van Moort Citation1986; McKenzie et al. Citation2001; Reyes et al. Citation2002; Craw Citation2005).
Sampling
At historic mine processing facilities, samples were collected from battery building foundations, from the former locations of Hg amalgamation tables, historic tailings ponds or heaps, and in areas draining these sites. At warm spring sites, water and sediment samples were collected from water seepage points (identified by bubbles and higher temperature) within the warm spring zone.
Water samples at the Blackwater mine (Snowy River) and the Hurunui warm springs were filtered (<0.45 µm) on site into acidified plastic bottles. Water pH was monitored at sample locations using an Oakton PC 10 pH meter. At the Nevis River and Golden Point historic processing site, water samples collected for Hg analysis were filtered (<0.45 µm) on site into 1 L acid cleaned Teflon bottles using a Teflon hose and peristaltic pump (Masterflex, Cole Palmer). Trace metal clean sampling followed Kim & Hunter (Citation1997), using a two person protocol in order to avoid contamination. Mercury samples were acidified on site with 5 mL Hg-free HCl. Water samples for arsenic analysis were collected using the same methods.
Solid samples at the Snowy River and Golden Bar historic processing sites were collected in plastic bags using a clean plastic trowel. Wet sediment samples were collected in acid cleaned 500 mL glass bottles with plastic lids and were capped underwater. Wet sediment samples were air dried at room temperature before analysis.
Analytical techniques
Solid samples were analysed for arsenic and mercury by acid digestion and Inductively coupled plasma mass spectrometry (ICP-MS) at Hill Laboratories, Hamilton, New Zealand. Detection limits were 2 mg/kg for arsenic and 0.1 g/kg for mercury, excepting warm spring samples for which detection limits were 0.2 mg/kg for As and 0.01 mg/kg for Hg. Water samples from Macraes mine and the Hurunui warm springs were analysed for As and Hg by Flame Atomic Absorption Spectrometry (FAAS) at Chemsearch Laboratory, University of Otago, New Zealand. For Macraes mine samples, detection limits were 0.2 µg/L for Hg and 5 µg/L for As. For warm spring samples, detection limits were 0.08 µg/L for Hg and 1 µg/L for As.
Nevis River and Golden Point water samples were analysed for Hg in the Chemistry Department at the University of Otago using a method modified from Gill & Fitzgerald (Citation1987), which enables determination of sub-ng/L Hg levels. Samples were oxidised with Hg-free BrCl for 12 h and analysed for Hg by SnCl2 reduction, gas phase stripping, two-stage Au amalgamation, and detection by cold-vapor atomic fluorescence spectrometry. Detection limit for this method is 0.04-0.06 ng/L independent of sample volume, and analytical precision is 10% at 1 ng/L (Gill & Fitzgerald Citation1987; Gill & Bruland Citation1990). Golden Point water samples were analysed for As at Hill Laboratories, Hamilton, New Zealand, by ICP-MS with a detection limit of 1 µg/L.
Mine-related mercury
Mine-related Hg in solids and associated water is derived from two distinct sources. The first is Hg in ore minerals that are a natural component of the mineralised rocks, including Hg in water that is dissolved from those minerals. The second source is Hg that is added to the ore to extract gold by amalgamation, including dissolved Hg that is derived from dissolution of these solids. In addition, ore processing activity can increase the dissolution rate of natural Hg in the ore, elevating Hg contents of mine-related waters. Mine sites in the southeast part of the South Island are open grassland, and the climate is semi-arid to temperate. The southeast region receives about 600 mm/yr rainfall in a rain shadow to the east of the Southern Alps. Rainfall is >2000 mm/yr in the warm–temperate northwest side of the South Island, and the landscape around the studied mine site is dominated by thick forest. Water is between pH 7 and 8 at all sites examined, which is typical of mesothermal terranes (Craw Citation2001).
Macraes mine, southeast South Island
The active Macraes mine is currently being developed near several historic (1880s–1940s) mines. The modern mine is a large open pit operation (ca. 200,000 oz/yr) with excavations occurring at several sites along the Hyde-Macraes Shear Zone (, A). Gold separation for the modern mine occurs by cyanidation at a processing plant on the southeastern side of Deepdell Creek (A). The ore is rich in pyrite and arsenopyrite, and mineralised rocks have elevated Hg contents (0.1 to 1 mg/kg) compared to a background of about 0.01 mg/kg in unmineralised host schist (A) (Pitcairn et al. Citation2006). The elevated Hg in the ore is at least partially hosted in the Au, which has 1–4 wt% Hg (MacKenzie & Craw Citation2005). Ore concentrate is roasted and oxidised to liberate fine-grained gold encapsulated in pyrite and arsenopyrite before cyanidation.
Fig. 2 Golden Point battery. (A) Locations of water samples collected from Deepdell Creek. (B) Hg concentrations of these samples (left to right) upstream, adjacent to, and downstream of the battery.
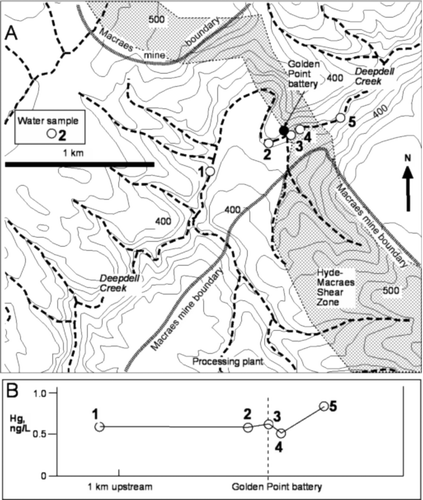
Fig. 3 (A, B) Mercury and arsenic concentrations in South Island waters and solids; mineralised and unmineralised rock values are after Pitcairn et al. (Citation2006) and Craw & Pacheco (Citation2002).
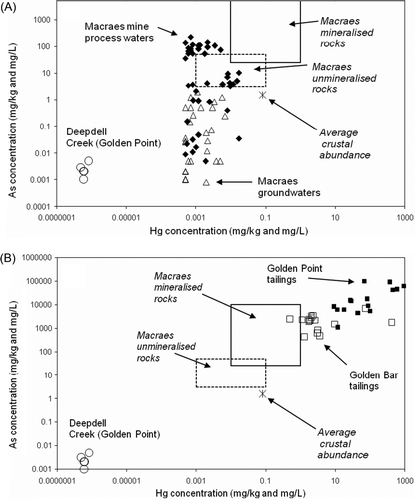
Deepdell Creek water is predominately derived from shallow groundwater in unmineralised schist (A). Dissolved Hg levels in Deepdell Creek are <1 ng/L, and dissolved As concentrations are only marginally above the detection limit of the analytical technique (B, A). Groundwaters in the mine area have dissolved (<0.45 µm) Hg concentrations up to 0.01 mg/L, with a wide range of dissolved arsenic (A). The lower limit of Hg concentrations in A is defined by the detection limit of the analytical technique. Many groundwater Hg concentrations are up to four orders of magnitude greater than background surface waters in Deepdell Creek. Deepdell Creek water is predominantly derived from shallow groundwater in unmineralised schist (A). Dissolved As concentrations in Deepdell Creek are similar to the lower end of the mine groundwater As range (A).
Mine processing waters are derived from a river with low dissolved As and Hg similar to that in Deepdell Creek. The processing waters develop distinctly elevated As concentrations (A), as a result of variable dissolution of arsenic minerals in the processing plant (Craw & Pacheco Citation2002). Comparable, but less pronounced Hg dissolution from the ore occurs, as some processing waters have Hg contents up to 0.01 mg/L.
Historic mine sites, Macraes area
Mineralised Hyde-Macraes Shear Zone rocks were historically mined by horizontal adits in hillsides (A). The Golden Point mine and associated processing plant (battery) was in Deepdell Creek and is still preserved between two parts of the active Macraes mine (A). The Golden Point battery processed the same type of ore which is currently processed at the Macraes mine, but Hg amalgamation was used rather than cyanidation to separate the gold. In the latter stages of the mine life (early 1940s), sulfide mineral concentrates were roasted and oxidised to release fine-grained gold prior to Hg amalgamation (Mains & Craw Citation2005). Tailings from the battery were discharged into Deepdell Creek and have been almost entirely removed during floods after mine closure (Black et al. Citation2004).
A small pile (1–2 m3) of tailings from the roasting process remained on the banks of Deepdell Creek beside the battery after mine closure (A). Most of this pile was removed in 2005 (Mains & Craw Citation2005), with only scattered remnants. These roasted tailings had strongly elevated As and Hg contents, with up to 1000 mg/kg Hg (B; Mains & Craw Citation2005). Downstream of the Golden Point battery, dissolved Hg levels in Deepdell Creek remain fairly constant, near 0.6 ng/L (B). Despite the historic use of Hg amalgamation, there is no detectable dissolved Hg contribution from the Golden Point battery site (B). Possible minor elevation of Hg to 0.8 ng/L (B) detected 100 m downstream of the site may be a result of incoming groundwater from the Hyde-Macraes Shear Zone (A). However, the small difference between this downstream Hg concentration and that farther upstream is close to the analytical uncertainty of 10%.
Another historic mine site, Golden Bar, is located in the Hyde-Macraes Shear Zone 5 km to the southeast of the modern Macraes mine (). The remnants of a processing battery are located on the opposite side of an ephemeral creek from the mine adits (). A small tailings impoundment was constructed immediately in front of the battery (), and this is still filled with tailings. Mining ceased in 1907 (Petchey Citation2005), and the site has been largely grassed over by natural recolonisation. Processing consisted of mercury amalgamation for unroasted ores. Rare occurrences of liquid mercury in the foundations of the battery site have been reported (Petchey Citation2005), and remnants of the processed ore have variable Hg contents (up to 417 mg/kg; ). Samples from two holes dug in the tailings impoundment show that Hg contents of these tailings have about 2 mg/kg Hg, with minor variation through the pile ( inset). The Hg contents of the tailings samples are generally lower than those of samples from the Golden Point historic mine (B). Likewise, the As contents of these Golden Bar tailings are generally lower than Golden Point material, but similar to typical Macraes ore (B).
Historic Blackwater mine site, northwest South Island
The Blackwater mine () was an extensive underground operation on the West Coast of the South Island. Gold was extracted from mesothermal quartz veins hosted in Buller Terrane metagreywackes (Christie & Braithwaite Citation2003) until the mine closed in the early 1950s. The processing site was left largely intact, although subsequent degradation has obscured some areas. Processing involved mercury amalgamation, cyanidation, and roasting of arsenopyrite-rich ore. The Snowy River battery at the Blackwater mine (, ) was selected for examination of solid wastes because of relatively good site preservation and lack of disturbance. This processing site is adjacent to Snowy River, which has a flow rate of 0.8 m3/sec and drains runoff from frequent rainfalls from a large area. Hence, no attempt was made to analyse the Hg content of these river waters.
Fig. 5 Snowy River battery, showing locations and Hg concentrations of solid samples (after Haffert & Craw 2008). Inset: Hg concentrations for vertical profile of solid samples collected from the riverbank at location A.
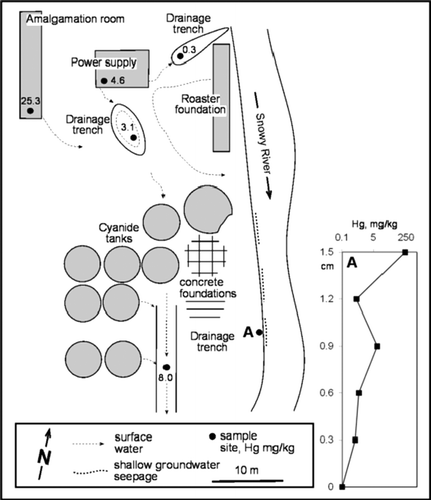
Fig. 6 Mercury and arsenic concentrations in South Island historic mine site solids; mineralised and unmineralised rock values are after Pitcairn et al. (Citation2006) and Craw & Pacheco (2002).
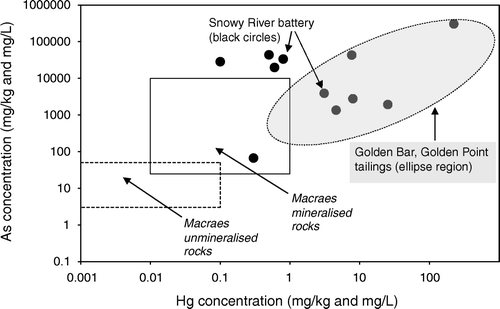
Soil and ore processing residues at the Snowy River site have a wide range of Hg contents, from 0.1 to 223 mg/kg, and some samples are up to 30.5 wt% As (, ). There is a weak positive relationship between Hg and As contents of these samples, although some very high As samples have low Hg. Samples with highest Hg and As contents have similar concentrations to Golden Bar and Golden Point tailings in the Macraes area.
Natural mercury elevation in the South Island
Warm springs
Warm springs occur along the active plate boundary, principally near the Alpine and Hope Faults (). These springs are dominated by meteoric water, and surface precipitates include calcite, iron oxyhydroxide, and quartz (Barnes et al. Citation1978). Springs near the Hope Fault have evidence for elevated Hg, based on the presence of cinnabar in precipitates (Barnes et al. Citation1978). For this study, we examined the Hurunui spring (), which proved to be the most accessible for analytical sampling purposes. Precipitates from this spring contain elevated Hg up to 111 mg/kg (). Arsenic concentrations of the precipitates are c. 1 mg/kg, and are therefore near or below As concentrations of host rocks. Water samples from the Hurunui spring have 0.2 to 0.3 µg/L dissolved (<0.45 µm) Hg, and dissolved arsenic is below the detection limit of the method used. These samples have been assigned As concentrations of 0.5 µg/L (half the detection limit) to enable plotting of the Hg data in .
Mercury-bearing gold
Gold in vein systems and the detrital deposits derived from these veins in the South Island is typically an Au-rich alloy (>90% Au) with subordinate Ag and Hg (Youngson et al. Citation2002; MacKenzie & Craw Citation2005). Au-Ag-Hg alloy containing up to 8.4 wt% Hg occurs in vein systems in the southern portion of the Otago Schist belt (MacKenzie & Craw Citation2005). Veins from east Otago contain 2–8 wt% Hg, Hyde-Macraes Shear Zone Au contains 1–4 wt% Hg, and some Central Otago vein systems contain 1–2 wt% Hg (MacKenzie & Craw Citation2005). Detrital Au with similar ranges of Hg contents occur at many mined and unmined sites in the southern South Island () (Youngson et al. Citation2002; Falconer Citation2003). Typical Au/rock concentrations in South Island deposits are <10 mg/kg, so Hg concentrations in these deposits are<1 mg/kg.
Cinnabar
In addition to occurring as a precipitate in some South Island warm springs, cinnabar (HgS) occurs in mesothermal-style veins in the southeast South Island (). Two outcrops are known () (Marshall Citation1918; Henderson Citation1923). Cinnabar is widespread as fine-grained (sand sized) particles accompanying detrital gold over large areas of the southeast South Island () (Marshall Citation1918; Williams Citation1974; Youngson et al. Citation2002). Most of these occurrences are in Quaternary gravel deposits that have been mined extensively for placer Au. Coarse-grained detrital cinnabar clasts have been identified near an inferred primary cinnabar vein source in the Nevis valley (). The most Hg-rich placer Au deposits occur in the Nevis and Nokomai valleys, where abundant detrital cinnabar accompanies abundant Hg-rich detrital Au (up to 38 wt% Hg; Youngson et al. Citation2002). Localised surficial liquid Hg droplets occur in gravels of an unmined alluvial fan in the Nokomai valley; this Hg is inferred to be natural (Youngson et al. Citation2002). Although Hg-bearing minerals are widespread and may be locally abundant, typical concentrations of these minerals in the Quaternary deposits are <100 mg/kg. Hence, bulk solid Hg concentrations are significantly lower.
Dissolved Hg in Nevis River
The Nevis valley () contains auriferous fluvial gravels that have been mined periodically since the 1860s. Historic mining focused on shallow (<5 m) thick gravels along active streams, with some deeper dredging in the lower Nevis basin. Hence, the Nevis valley contains three possible solid sources of Hg: cinnabar, Hg-bearing detrital gold (above), and Hg related to gold mining. However, no anthropogenic gold-mercury amalgam was found in any pan concentrates in this study. A series of water analyses for Hg was taken from the Nevis River upstream of Nevis Crossing (). A water sample was also taken from a tributary to the Nevis River on the east side of the valley at Nevis Crossing. In addition, shallow pits were dug in cinnabar-bearing auriferous gravel in the upper Nevis valley, up to 20 m from the active river, to determine if any particular solid source(s) could be identified for dissolved Hg.
Dissolved Hg analyses for the Nevis River and tributaries range between 1 and 3 ng/L (). The dissolved Hg concentration in the Nevis River is highest where detrital cinnabar is most abundant. Dissolved Hg levels reach as high as 7 ng/L in shallow (<1.5 m) groundwater in these cinnabar-bearing gravels. The dissolved Hg decreases down the Nevis valley from these relatively high concentrations, and then rises again farther downstream to1.6 ng/L (). The tributary near Nevis Crossing has even higher dissolved Hg of 2.8 ng/L.
Solubility of cinnabar in river water
Widespread occurrence of detrital cinnabar in rivers suggests that naturally elevated Hg concentrations in the South Island are partly controlled by dissolution of cinnabar. The cinnabar occurrences described above are all in fast-flowing rivers, and dilution results in low dissolved Hg contents. To obtain an indication of potential maximum levels of environmental Hg that may arise, at least locally, from the detrital cinnabar, we use experimental data reported for the solubility of HgS in oxygenated fluvial environments. Cinnabar is expected to dissolve in South Island rivers between 2.64 and 6.16 µmol/m2/day (based on experimental sulfate production), although the consequent release of Hg is up to two orders of magnitude lower due to adsorption of Hg2+ to the surface of the dissolving cinnabar (Holley et al. Citation2007). Even when attenuation of Hg occurs by adsorption, dissolved Hg may be locally elevated up to 32 µg/L in the vicinity of dissolving cinnabar (Holley et al. Citation2007). In South Island rivers, these concentrations are rapidly decreased by dilution.
Summary and discussion
Relative significance of mine-related and natural Hg in the South Island
Tailings and other processing residues from the historic mine sites described above have strongly elevated Hg contents compared to other South Island solids sampled in this study (A, B, 6). In particular, the Hg contents of these residues are 103 to 104 times higher than primary ore (B). The high Hg processing residues also have strongly elevated As contents, up to 30 wt% As (B, 6). Arsenic contents can be many orders of magnitude higher than typical mineralised rock, resulting from concentration of ore minerals in the processing plants (B). Enrichment of arsenic-bearing minerals in ore may also result in some enrichment of naturally elevated Hg in the ore (A, B). The increase in Hg content of processing residues is 10–100 times greater than that of As (B), which suggests that a significant proportion of the Hg in this material results from Hg addition during the amalgamation process. Natural cinnabar deposition from warm springs (Barnes et al. Citation1978) results in localised, strongly elevated Hg concentrations in precipitates. However, processing residues have Hg contents that are similar to, or higher than, natural precipitates from the Hurunui warm springs ().
Despite the presence of high levels of Hg contamination in small volumes of processing residues at the Golden Point battery site, there is no strong evidence for contamination of the adjacent waterway (Deepdell Creek) with dissolved Hg from the most Hg-rich residues (B). Dissolved Hg concentrations are higher in groundwaters in nearby mineralised rocks than in the Deepdell Creek (B). Minor elevation of dissolved Hg in mine processing waters at the modern Macraes mine is the result naturally elevated Hg in the ore, some of which is released during processing. Macraes mine waters have dissolved Hg concentrations similar to the Hurunui warm springs.
Nevis River dissolved Hg
Dissolved Hg up to 7 ng/L occurs in shallow groundwater in the upper reaches of the Nevis valley, and river waters have up to 3 ng/L dissolved Hg (). This upper portion of the valley is least disturbed by previous mining activity and is where naturally occurring Hg-bearing minerals (cinnabar, Hg-bearing Au) are abundant (). There is no evidence for the presence of anthropogenic Au-Hg amalgam in the sediments. Hence, we conclude that the observed elevated levels of dissolved Hg are natural and constitute background levels for this alluvial mining area. Gold-mercury amalgams are less stable in the environment than cinnabar and liquid Hg (Miller et al. Citation2002; Holley et al. Citation2007), and there is textural evidence for Hg leaching from rims of grains in the Nevis and Nokomai valleys (Youngson et al. Citation2002). Addition of Hg to, and loss of Hg from, Au particles can occur on time scales of days to years (Miller et al. Citation2002). This may be enhanced by microbiological activity (Miller et al. Citation2002). Hence, we conclude that leaching of Hg from natural Au-Hg amalgam particles is the most likely source of the elevated dissolved Hg in the Nevis and Nokomai valleys.
The Nevis River has average summer flows of c. 5 m3/sec, rising to c. 12 m3/sec average in winter (Otago Regional Council unpubl. data). Typical summer flow rates of c. 5 m3/sec for the lower Nevis River applied during our sampling program. This average flow rate, combined with typical dissolved Hg concentration of 3 ng/L for the lower Nevis River, implies total background flux of mercury of c. 0.5 kg/yr.
Limits to Hg and As environmental availability
Dissolution of Hg-bearing minerals, attenuation by adsorption of Hg species, and dilution control Hg environmental availability at the sites studied here. Dissolution of cinnabar may result in locally elevated dissolved Hg concentrations in natural streams up to 32 µg/L (Holley et al. Citation2007), which is an order of magnitude higher than Macraes mine waters (). However, adsorption of Hg to remaining cinnabar may limit this Hg release (Holley et al. Citation2007), and dilution ensures that dissolved Hg is <3 ng/L in rivers with abundant detrital cinnabar. Deposition of cinnabar in warm spring precipitates (Barnes et al. Citation1978) suggests that dissolved Hg concentrations in spring waters must reach saturation. However, the abundant meteoric water circulation ensures that spring water is typically greatly undersaturated with respect to cinnabar deposition (), and the actual precipitation of cinnabar at the surface is probably facilitated by evaporative concentration of these waters. Macraes mine groundwaters and process waters are well oxidised, and cinnabar precipitation would not be expected. The solubility of elemental Hg (Hg0) in water is close to 50 µg/L at 20°C (Glew & Hames Citation1971). Liquid mercury droplets are predicted to occur at the sediment-water interface when dissolved Hg exceeds this concentration. In the Nokomai valley, liquid Hg may result from locally elevated dissolved Hg in groundwater, likely sourced from proximal detrital cinnabar and Hg-bearing gold (Youngson et al. Citation2002).
Fig. 9 Summary of mercury and arsenic concentrations in South Island waters and solids; mineralised and unmineralised rock values are after Pitcairn et al. (2006) and Craw & Pacheco (2002). For comparison: (A) Hg released by cinnabar (HgS) dissolution (Holley et al. 2007); (B) solubility of liquid Hg at 20°C (Glew & Hames 1971); (C) solubility of arsenopyrite (FeAsS) at neutral pH (Craw et al. Citation2003); (D) solubility of scorodite (FeAsO4.2H2O) at neutral pH (Krause & Ettel 1988).
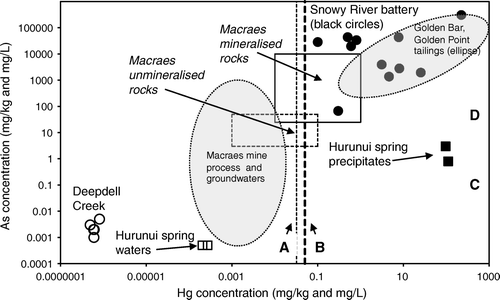
Limits to dissolved arsenic accompanying dissolved Hg in the South Island are defined by the solubility of scorodite (FeAsO4.2H2O), as determined by Krause & Ettel (Citation1988) (). Scorodite precipitation limits the As concentrations of Macraes mine waters (Craw & Pacheco Citation2002). Scorodite is an important component of exposed surfaces of oxidised, arsenopyrite-rich Macraes mine ore, and roasted concentrate residue at the Golden Point battery () (Mains & Craw Citation2005). Likewise, scorodite is a common secondary mineral in the processing residues at the Snowy River battery (, ) (Haffert & Craw Citation2008).
Comparison to epithermal systems of the North Island
North Island active or recently active epithermal (geothermal) systems typically have precipitates and hydrothermally altered zones that are enriched in Hg (; White Citation1981; Davey & van Moort Citation1986; McKenzie et al. Citation2001; Reyes et al. Citation2002; Craw Citation2005). Active cinnabar deposition has been reported in the Northland geothermal area by Davey & van Moort (Citation1986), attesting to locally high dissolved Hg. However, the levels of Hg enrichment in most of these precipitates are not significantly greater than the Hurunui warm spring precipitates (), excepting several spectacular examples from the Taupo Volcanic Zone (TVZ; ) (White Citation1981; Davey & van Moort Citation1986; Reyes et al. Citation2002; Craw Citation2005). Measured dissolved Hg concentrations in the Hurunui warm springs are within the range of values reported for North Island geothermal waters ( McKenzie et al. Citation2001; White Citation1981). The key difference between the North Island and South Island hydrothermal systems is the vastly greater volume of rock and water involved in the North Island systems. For instance, Davey & van Moort (Citation1986) reported that in Northland, the Hg flux from Ngawha Springs is 530 kg/yr; three orders of magnitude greater than the Hg flux from the Nevis River in the South Island. North Island geothermal precipitates have generally higher Hg and lower As concentrations relative to South Island Hyde-Macraes mineralised rocks (A, 7). However, South Island processing residues from historic Hg amalgamation have compositions that are similar to the most Hg-rich precipitates from North Island geothermal systems (, ).
Conclusions
Mercury is naturally present in the South Island mesothermal environment in mineralised schist, Hg-bearing vein and detrital gold, as vein-hosted and detrital cinnabar, and in warm spring precipitates. Waters proximal to these occurrences contain elevated Hg up to 0.01 mg/L, with the most concentrated Hg in shallow groundwaters in mineralised zones, and in modern mine processing waters due to dissolution of naturally Hg-enriched Au ore. Mercury has been added anthropogenically for Hg amalgamation at some historic gold mines in the South Island. This anthropogenic signature is strong in solids at these sites, where ore processing residues contain up to 1000 mg/kg Hg. However, Hg is nearly absent in adjacent surface waters, which are 0.8 ng/L or less. Solid wastes at some historic mine and mineral processing sites contain spectacularly high levels of As up to 30.5 wt%, and relative to Hg, As is of greater environmental significance. South Island warm spring precipitates have Hg content comparable to North Island epithermal systems, and similar levels of dissolved Hg. However, relative to the South Island, North Island hydrothermal systems are characterised by far larger volumes of water and rock, resulting in greater Hg flux. In the South Island, natural enrichment of vein systems, concentration of natural Hg during Au ore processing, and anthropogenic addition of Hg during historic Au mining contribute to occurrences of elevated Hg. Mobilisation of this Hg to downstream environments is at least partially controlled by the cinnabar (HgS) and liquid mercury (Hg0) solubility.
Acknowledgements
We gratefully acknowledge financial support from the University of Otago, the Rotary Foundation, and the New Zealand Foundation for Research, Science, and Technology. We thank John Watson, David Barr, Damian Walls, and Lorraine Paterson for assistance with analyses, John Taylor, Shaun Barker, Tina Bayer, Laura Haffert, Adam Martin, John Youngson, and John Vorhies for discussions and assistance in the field, and Barry Anderson and Keith Hunter for technical support and equipment. We are grateful to Oceana Gold Ltd. for providing access to the Macraes mine sites, and to the New Zealand Department of Conservation for access to the Blackwater mine site. Suggestions from Robert Kerrich and an anonymous reviewer improved the manuscript.
References
- Barnes , I , Downes , CJ and Hulston , JR . 1978 . Warm springs, South Island, New Zealand, and their potentials to yield laumontite . American Journal of Science , 278 : 1412 – 1427 .
- Barnes , HL and Seward , TM . 1997 . “ Geothermal systems and mercury deposits ” . In Geochemistry of hydrothermal ore deposits , 3rd ed , Edited by: Barnes , HL . 699 – 736 . New York : John Wiley & Sons, Inc .
- Black , A , Craw , D , Youngson , JH and Karubaba , J . 2004 . Natural recovery rates of a river system impacted by mine tailing discharge: Shag River, East Otago, New Zealand . Journal of Geochemical Exploration , 84 : 21 – 34 .
- Christie , AB and Brathwaite , RL . 2003 . Hydrothermal alteration in metasedimentary rock- hosted orogenic gold deposits, Reefton goldfield, South Island, New Zealand . Mineralium Deposita , 38 : 87 – 107 .
- Cole , JW . 1979 . Structure, petrology and genesis of Cenozoic volcanism, Taupo volcanic zone, New Zealand: a review . New Zealand Journal of Geology and Geophysics , 22 : 631 – 657 .
- Craw , D . 2001 . Tectonic controls on gold deposits and their environmental impact, New Zealand . Journal of Geochemical Exploration , 73 : 43 – 56 .
- Craw , D . 2005 . Potential anthropogenic mobilization of mercury and arsenic from soils on mineralised rocks, Northland, New Zealand . Journal of Environmental Management , 74 : 283 – 292 .
- Craw , D , Chappell , D and Reay , A . 2000 . Environmental mercury and arsenic sources in fossil hydrothermal systems, Northland, New Zealand . Environmental Geology , 39 : 875 – 887 .
- Craw , D and Pacheco , L . 2002 . Mobilisation and bioavailability of arsenic around mesothermal gold deposits in a semiarid environment, Otago, New Zealand . The Scientific World , 2 : 308 – 319 .
- Craw , D , Falconer , D and Youngson , JH . 2003 . Environmental arsenopyrite stability and dissolution; theory, experiment, and field observations . Chemical Geology , 199 : 71 – 82 .
- Davey , HA and van Moort , JC . 1986 . Current mercury deposition at Ngawha Springs, New Zealand . Applied Geochemistry , 1 : 75 – 93 .
- Falconer D 2003 . Sediment-hosted gold and sulphide mineralization, Belle-Brook, Southland, New Zealand . Unpublished MSc thesis, University of Otago, Dunedin
- Gill , GA and Fitzgerald , WF . 1987 . Picomolar mercury measurements in seawater and other materials using stannous chloride reduction and 2-stage gold amalgamation with gas phase detection . Marine Chemistry , 20 : 227 – 243 .
- Gill , GA and Bruland , KW . 1990 . Mercury speciation in surface freshwater systems in California and other areas . Environmental Science and Technology , 24 : 1392 – 1400 .
- Glew , DN and Hames , DA . 1971 . Aqueous nonelectrolyte solutions. Part X. Mercury solubility in water . Canadian Journal of Chemistry , 49 : 3114 – 3118 .
- Gray , JE , Gent , CA , Snee , LW and Wilson , FH . 1997 . Epithermal mercury-antimony and gold-bearing vein deposits of southwestern Alaska . Economic Geology Monograph , 9 : 287 – 305 .
- Haffert , L and Craw , D . 2008 . Mineralogical controls on environmental mobility of arsenic from historic mine processing residues, New Zealand . Applied Geochemistry , 23 : 1467 – 1483 .
- Henderson J 1923 . Cinnabar in Greenvale Survey District near Waikaia, Southland . 17th Annual Report of the New Zealand Geological Survey: 12 .
- Holley , EA , McQuillan , AJ , Craw , D , Kim , JP and Sander , SG . 2007 . Mercury mobilization by oxidative dissolution of cinnabar (α-HgS) and metacinnabar (β-HgS) . Chemical Geology , 240 : 313 – 325 .
- Hylander , LD and Meili , M . 2003 . 500 years of mercury production: global annual inventory by region until 2000 and associated emissions . Science of the Total Environment , 304 : 13 – 27 .
- Kim , JP and Hunter , KA . 1997 . Aqueous chemistry of major ions and trace metals in the Takaka–Cobb River system, New Zealand . Marine and Freshwater Research , 48 : 257 – 266 .
- Krause , E and Ettel , VA . 1988 . Solubility and stability of scorodite, FeAsO4.2H2O: new data and further discussion . American Mineralogist , 73 : 850 – 854 .
- Lacerda , LD . 1997 . Global mercury emissions from gold and silver mining. Water, Air . and Soil Pollution , 97 : 209 – 221 .
- Lechler , PJ , Miller , JR , Hsu , LC and Desilets , MO . 1997 . Mercury mobility at the Carson River Superfund Site, west–central Nevada, USA: interpretation of mercury speciation data in mill tailings, soils, and sediments . Journal of Geochemical Exploration , 58 : 259 – 267 .
- MacKenzie , DJ and Craw , D . 2005 . The mercury and silver contents of gold in quartz vein deposits, Otago Schist . New Zealand Journal of Geology and Geophysics , 48 : 265 – 278 .
- McKenzie , EJ , Brown , KL , Cady , SL and Campbell , KA . 2001 . Trace metal chemistry and silicification of microorganisms in geothermal sinter, Taupo Volcanic Zone, New Zealand . Geothermics , 30 : 483 – 502 .
- Mains , D and Craw , D . 2005 . Composition and mineralogy of historic gold processing residues, east Otago, New Zealand . New Zealand Journal of Geology and Geophysics , 48 : 641 – 647 .
- Marshall P 1918 . The geology of the Tuapeka district. NZ Geological Survey Bulletin 19 .
- Merchant RJ 1986 . Mineralisation in the Thames district, Coromandel. Guide to the active epithermal (geothermal) systems and precious metal deposits of New Zealand . In : Henley RW , Hedenquist JW , Roberts PJ MonographSeries . Mineralium Deposita 26 : 147 163 .
- Miller , JW , Callahan , JE and Craig , JR . 2002 . Mercury interactions in a simulated gold placer . Applied Geochemistry , 17 : 21 – 28 .
- Moore , TA , Zhongsheng , L , Nelson , CM , Finkelman , RB and Boyd , R . 2005 . “ Concentration of trace elements in coal beds ” . In Metal contaminants in New Zealand , Edited by: Moore , TA , Black , A , Centeno , JA , Harding , JS and Trumm , JS . 212 – 230 . Christchurch, NZ : Resolutionz Press .
- Pacyna , EG and Pacyna , JM . 2002 . Global emission of mercury from anthropogenic sources in 1995 . Water, Air, and Soil Pollution , 137 : 149 – 165 .
- PG Petchey 2005 . Golden Bar Mine, Macraes; Archeological investigations prepared for Oceana Gold Ltd .
- Pitcairn , IK , Teagle , DA , Craw , D , Olvio , GR , Kerrich , R and Brewer , TS . 2006 . Sources of metals and fluids in orogenic gold deposits: insights from the Otago and Alpine Schists, New Zealand . Economic Geology , 101 : 1525 – 1546 .
- Pope , JG , McConchie , DM , Clark , MD and Brown , KL . 2004 . Diurnal variations in the chemistry of geothermal fluids after discharge, Champagne Pool, Waiotapu, New Zealand . Chemical Geology , 203 : 253 – 272 .
- Reyes , AG , Trompetter , WJ , Britten , K and Searle , J . 2002 . Mineral deposits in the Rotokawa geothermal pipelines, New Zealand . Journal of Volcanology and Geothermal Research , 119 : 214 – 239 .
- Roth , DA , Taylor , HE , Domagalski , J , Dileanis , P , Peart , DB , Antweiler , RC and Alpers , CN . 2001 . Distribution of inorganic mercury in Sacramento River water and suspended colloidal sediment material . Archives of Environmental Contamination and Toxicology , 40 : 161 – 172 .
- Sherlock , RL . 2005 . The relationship between the McLaughlin gold–mercury deposit and hydrothermal systems in the Geysers–Clear Lake area, northern Coast Ranges, California . Ore Geology Reviews , 26 : 349 – 382 .
- UNEP 2002 . Global mercury assessment report . http://www.chem.unep.ch/MERCURY/Report/GMA-report-TOC.htm [accessed 17 April 2007]
- USEPA 1997 . Mercury study report to Congress. Washington DC, US Environmental Protection Agency . EPA-453/R-97-003 .
- Varekamp , JC and Buseck , PR . 1984 . The speciation of mercury in hydrothermal systems, with applications to ore deposition . Geochimica et Cosmochimica Acta , 48 : 177 – 185 .
- Veiga , MM , Maxson , PA and Hylander , LD . 2006 . Origin and consumption of mercury in small-scale gold mining . Journal of Cleaner Production , 14 : 436 – 447 .
- Webster-Brown , J and Craw , D . 2005 . “ Examples of trace metal mobility around historic and modern metal mines ” . In Metal contaminants in New Zealand , Edited by: Moore , TA , Black , A , Centeno , JA , Harding , JS and Trumm , JS . 212 – 230 . Christchurch, NZ : Resolutionz Press .
- White DE , Hinkle ME , Barnes I 1970 . Mercury contents of natural thermal and mineral fluids . USGS Professional Paper 713 . 25 28 .
- White DE 1981 . Active geothermal systems and hydrothermal ore deposits . Economic Geology 75th Anniversary Volume : 392 423 .
- Williams , JG . 1974 . Economic geology of New Zealand . Australasian Institute of Mining and Metallurgy Monograph , 4 : 191
- Youngson , JH , Woperis , P , Kerr , LC and Craw , D . 2002 . Au-Ag-Hg and Au-Ag alloys in Nokomai and Nevis valley placers, northern Southland and Central Otago, New Zealand, and their implications for placer-source relationships . New Zealand Journal of Geology and Geophysics , 45 : 53 – 69 .