Abstract
Early Cretaceous parts of the western Median Batholith (Western Fiordland Orthogneiss) represent the exposed root of a magmatic arc of dioritic to monzodioritic composition (SiO2=51–55 wt%; Na2O/K2O=3.7–8.8 in this study). We characterise for the first time the field relationships, petrography, mineralogy and geochemistry of ultramafic and mafic cumulates at Hawes Head, the largest exposure of ultramafic rocks in western Fiordland. We distinguish three related rock types at Hawes Head: hornblende peridotite (MgO=21–35 wt%); hornblendite (MgO=15–16 wt%); and pyroxenite (MgO=21 wt%). Petrogenetic relationships between the ultramafic rocks and the surrounding Misty Pluton of the Western Fiordland Orthogneiss are demonstrated by: (i) mutually cross-cutting relationships; (ii) similar mafic phases (e.g. pyroxene and amphibole) with elevated Mg-numbers (e.g. olivine Mg/(Mg+Fe)=0.77–0.82); (iii) fractionation trends in mineral geochemistry; and (iv) shared depleted heavy rare earth element patterns. In addition, the application of solid/liquid partition coefficients indicates that olivine in the ultramafic rocks at Hawes Head crystallised from a magma with Mg/(Mg+Fe)=0.54–0.57. The olivine grains therefore represent a plausible early crystallising phase of the adjacent Western Fiordland Orthogneiss (Mg/(Mg+Fe)=0.51–0.55).
Introduction
Studies of volcanic rocks in modern arc environments show relatively evolved andesitic compositions indicating sub-volcanic fractional crystallisation and/or contamination of the primary basaltic arc magmas. These models partly require the separation of early-formed crystals from ascending magma to form cumulate rocks.Footnote The fractionation of common phenocryst phases in arc lavas (plagioclase+pyroxene±olivine) is unable to produce the fundamental shapes of rare earth element (REE) patterns documented for many arc volcanoes (Davidson et al. Citation2007; Tiepolo & Tribuzio Citation2008; Kratzmann et al. Citation2010; Larocque & Canil Citation2010). Although rarely observed as a phenocryst phase due to its instability at low pressures (Rutherford & Devine Citation1988; Romick et al. Citation1992), amphibole is therefore inferred to be an important mineral during differentiation of arc magma at mid-crustal levels (Davidson et al. Citation2007).
Information about processes active in the sub-arc environment may be obtained from geophysical data and xenolith studies (Cawthorn et al. Citation1973; Arculus & Willis Citation1980; DeBari & Coleman Citation1989; Ducea & Saleeby Citation1996). However, the direct examination of rare exposures of deep arc crust is much more valuable for understanding petrogenetic processes (e.g. Kohistan arc, Pakistan: Garrido et al. Citation2006; Jagoutz et al. Citation2006; Talkeetna arc, Alaska: Greene et al. Citation2006). Ultramafic rocks at Hawes Head in western Fiordland, South Island, New Zealand, are an unusually mafic unit within the extensive Median Batholith cordilleran arc system that was active along the Pacific margin of Gondwana during the Mezozoic. Igneous activity culminated in emplacement of large volumes of magma in the Late Jurassic and Early Cretaceous. The ultramafic rocks at Hawes Head mainly comprise hornblende peridotite and hornblendite. Minor components include pyroxenite, olivine–pyroxene hornblendite and pyroxene hornblendite. Exposures around Hawes Head provide an excellent opportunity to examine crystal accumulation processes that were active in this cordilleran arc. In this contribution we describe the field relationships, petrography, whole rock geochemistry and mineral chemistry of the Hawes Head ultramafic rocks. The results have important implications for early crystallisation sequences and magma evolution in deep arc environments.
Geological setting
The Median Batholith in western New Zealand represents the eroded remnant of a major cordilleran-style magmatic arc, which developed near the Pacific margin of Gondwana during the Mesozoic (Mortimer et al. Citation1999; Mortimer Citation2004). The western margin of the batholith intrudes Early Palaeozoic metasedimentary rocks with continental affinities (New Zealand Western Province), whereas the eastern margin of the batholith intrudes ensimatic accreted Permian–Jurassic volcano-sedimentary terranes (New Zealand Eastern Province) (Mortimer et al. Citation1999; Allibone et al. Citation2009a). Plutons emplaced prior to c. 128–125 Ma are characterised by calc-alkaline compositions, low Na and Al contents and low Sr/Y values (LOSY), whereas those emplaced after c. 128–125 Ma are characterised by alkali-calcic compositions, high Na and Al contents and high Sr/Y values (HISY) (Muir et al. Citation1998; Tulloch & Kimbrough Citation2003; Allibone et al. Citation2009a, Citationb). Most calc-alkaline LOSY rocks belong to the Darran Suite, whereas the younger alkali-calcic HISY rocks include the relatively leucocratic mid-crustal Separation Point Suite and more melanocratic mid to deep crustal Western Fiordland Orthogneiss.
Flatter REE patterns of the LOSY Darran Suite distinguish them from the steeper, heavy REE-depleted signatures of the younger HISY Separation Point Suite and Western Fiordland Orthogneiss (e.g. Muir et al. Citation1998). Allibone et al. (Citation2009b) used Pearce element ratio plots to investigate the different amounts of fractionation and/or accumulation of plagioclase, clinopyroxene and hornblende within each major suite of the Median Batholith. They found that fractionation and/or accumulation of plagioclase was the key control on variation in the major element chemistry of the Darran and Separation Point suites. The major element chemistry of the Western Fiordland Orthogneiss is however characterised by limited variability, suggesting minor fractionation and/or accumulation of hornblende and clinopyroxene.
The Western Fiordland Orthogneiss is divided into seven dioritic and monzodioritic plutons emplaced at variable depths (4–9 kbar in the north and 10–18 kbar in the south) over approximately 10 million years. Peak metamorphic facies include eclogite, omphacite-, garnet-, two-pyroxene- and hornblende-granulite and amphibolite facies (Oliver Citation1977; Bradshaw Citation1989a; Clarke et al. Citation2000; Allibone et al. Citation2009b; Daczko & Haplin Citation2009; De Paoli et al. Citation2009). Ultramafic rocks at Hawes Head described in this study are surrounded by the Western Fiordland Orthogneiss Misty Pluton, which comprises relatively leucocratic two-pyroxene±hornblende, feldspathic diorite, monzodiorite and minor monzonite in the vicinity of Hawes Head. Southern and northern parts of the pluton are variably metamorphosed to more melanocratic hornblende–plagioclase±clinozoisite±biotite diorite and quartz monzodiorite with relict clinopyroxene. Rare dykes of hornblende-rich diorite also cross-cut the more leucocratic two-pyroxene diorite, suggesting at least two phases of magmatism within the pluton locally (Allibone et al. Citation2009b).
Across Fiordland, metre- to kilometre-long bodies of hornblendite and less common pyroxenite and peridotite lie parallel to the foliation within the plutons of the Western Fiordland Orthogneiss (Oliver Citation1980; Allibone et al. Citation2009b). This study examines and characterises for the first time the petrogenesis of the largest ultramafic unit found to date at Hawes Head (Allibone et al. Citation2009b; Turnbull et al. Citation2010) at the mouth of Charles Sound (B).
Figure 1 Geological map of western Fiordland between Sutherland and Doubtful sounds showing plutons of the Western Fiordland Orthogneiss and sample locations (after Allibone et al. Citation2009b). Country rocks including the Western Province are excluded for clarity. Grid references refer to the NZMS260 1:50,000 scale topographic map series. The inset removes movement on the Alpine Fault for the South Island of New Zealand.
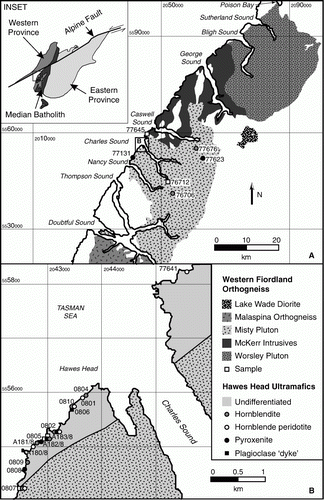
Ultramafic rocks of Hawes Head: Field relationships and petrography
Sample locations are shown in . We divide the ultramafic rocks at Hawes Head into three rock types on the basis of their mineralogy and International Union of Geological Sciences recommendations on rock nomenclature: (1) hornblende peridotite; (2) hornblendite; and (3) rare pyroxenite. Our field mapping shows mutually cross-cutting and inclusive relationships between peridotite and hornblendite, whereas pyroxenite was only found as inclusions within hornblendite.
Hornblende peridotite and rare olivine–pyroxene hornblendite
The distinguishing feature of hornblende peridotite is the presence of olivine. Olivine-bearing rocks are concentrated in the northern part of the ultramafic body at Hawes Head, but are rare in the southern part of the Hawes Head body and correlative ultramafic rocks on the northern side of Charles Sound (). Hornblende peridotites are only observed in association with hornblendite in coastal exposures at Hawes Head and rare minor inclusions within the Breaksea Orthogneiss in southwest Fiordland (Allibone et al. Citation2009b). The most common outcrops comprise uniform hornblende peridotite in igneous contact with hornblendite (A). Contact zones between the two rock types are typically c. 5 m across and contain numerous rafts and xenoliths of each rock type in the other. The xenoliths are typically 200–400 mm across and vary in shape from equant to elongate. The xenolith shapes are typically rounded and irregular. Some rounded inclusions and rare angular inclusions show ‘jig-saw’ fit textures. The inclusion–host boundary varies from sharp to gradational. Although each outcrop is generally uniform in texture, grain size and mineralogy, variations in texture exist between outcrops of hornblende peridotite. For example, some outcrops of hornblende peridotite contain rounded amphibole phenocrysts up to 30 mm long (B), whereas other outcrops contain only finer grained equigranular hornblende peridotite. Rare olivine–pyroxene hornblendite dykes or layers occur within hornblendite towards the southern end of the ultramafic body at Hawes Head (0807D).
Figure 2 Field photographs. A, Contact zone between hornblende peridotite (light colour, right) and hornblendite (dark colour, middle left). B, Hornblende peridotite with rounded phenocrysts of pargasite. C, ‘Jig-saw’ fit texture and shapes of rafts and xenoliths of hornblendite in hornblende peridotite. D, Hornblendite. E, Rare plagioclase accumulations or dykes in hornblendite. F, Contact zone between hornblendite and Misty Pluton. Note folded inclusions of hornblendite in Misty Pluton that is cut by an attenuated plagioclase-rich garnet-bearing dyke that is in turn cut by a sheet of hornblendite. G, Pyroxenite inclusions (light colour) in hornblendite (dark colour). H, ‘Jig-saw’ fit texture of rafts of pyroxenite in hornblendite.
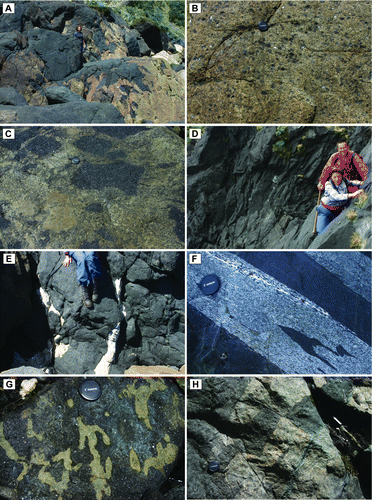
Hornblende peridotite consists of olivine (typically 1–2 mm, 35–58 vol.%), brown–green pargasite (typically 1–3 mm, 32–50 vol.%), orthopyroxene (typically 0.5–1.5 mm,<10 vol.%) and accessory ilmenite, hematite, spinel, carbonate,±rare retrograde serpentine, biotite and chlorite (A). Rare domains within the hornblende peridotite contain olivine modes up to c. 70 vol.% (C). Olivine and orthopyroxene are typically equigranular and polygonal. Pargasite is elongate, slightly coarser-grained than olivine and pyroxene, and exhibits simple twinning. Orthopyroxene displays tabular exsolution of clinopyroxene, and is typically more irregular and finer grained. Some grain boundaries contain lobate penetrations of orthopyroxene into olivine and/or pargasite. Some samples display pargasite poikiocrysts with olivine and less common orthopyroxene and ilmenite inclusions (B). Accessory minerals typically occur in clusters or as narrow films between silicates. Opaques occur as blebs at silicate grain triple junctions and as elongate exsolution lamellae within pargasite. Both ilmenite and hematite grains occur within clusters of accessory phases where they are commonly in contact.
Figure 3 Photomicrographs. A, Representative hornblende peridotite mineralogy dominated by olivine with orthopyroxene and brown–green pargasite (0810B). Field of view is 3.5 mm. B, Olivine, orthopyroxene and ilmenite inclusions in poikilitic pargasite (0801C). Field of view is 3.5 mm. C, Representative hornblendite mineralogy dominated by brown–green pargasite. Accessory opaque minerals are found as films along pargasite grain boundaries and at pargasite grain triple junctions (0805K). Field of view is 3.5 mm. D, Crystallographic control on exsolution of opaque (likely ilmenite) and transparent (likely rutile) grains in pargasite (A181/8). Field of view is 0.5 mm. E, Representative pyroxenite mineralogy dominated by clinopyroxene with orthopyroxene (77131). Field of view is 3.5 mm. F, Representative Misty Pluton plagioclase, two-pyroxene, pargasite mineralogy (A183/8). Field of view is 3.5 mm.
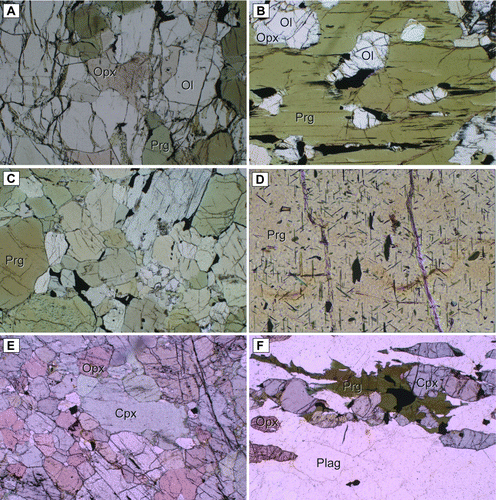
Hornblendite and rare pyroxene hornblendite
Hornblendite is distinguished by its near mono-mineralic composition and homogeneous texture. Hornblendite comprises c. 60% of the ultramafic rocks at Hawes Head and >90% of the correlative ultramafic rocks on the north side of Charles Sound (, D). The contact zone with olivine-bearing hornblende peridotite rocks is described in the previous section. Smaller bodies of hornblendite are also found as xenoliths and rafts up to several hundreds of metres long within the Misty Pluton up to 20 km inland (). In addition to inclusions of hornblende peridotite, hornblendite also contains rafts and xenoliths of pyroxenite that are found towards the south of the body of ultramafic rocks at Hawes Head. Hornblendite also hosts rare plagioclase dykes or layers 100–150 mm thick that are proximal to a contact between hornblende peridotite and hornblendite (E). Garnet was only observed in hornblendite at site 0802 in a planar trail (c. 20 mm wide) akin to garnet reaction zones, described throughout Fiordland (Bradshaw Citation1989b; Daczko et al. Citation2001; Daczko & Haplin Citation2009).
The contact between the ultramafic rocks and surrounding Misty Pluton (site 0807; B) is characterised by interlayered sheets of hornblendite and Misty Pluton diorite–monzodiorite 20–50 cm thick. Mutually inclusive and cross-cutting relationships are present between each lithology. The contact zone is strained with an east-striking foliation dipping approximately 50° south parallel to the contact. Outcrop scale sense-of-shear indicators include asymmetric foliation, sigma-type asymmetric strain shadows, shear bands and drag folds, indicating a component of dextral shear (F).
Hornblendite consists of brown–green pargasite (typically 1–7 mm, 90–98 vol.%) and accessory ilmenite, hematite, magnetite±clinopyroxene, carbonate, apatite and retrograde biotite (C). Pargasite grains are elongate and inequigranular. Grain boundaries are commonly smooth, curviplanar and sutured. Exsolution lamellae of crystallographically aligned transparent and opaque minerals are common (D). Accessory ilmenite and hematite typically form as interstitial films along grain boundaries and blebs at grain triple junctions, similar to the habits of ilmenite and hematite in hornblende peridotite samples. Carbonate and apatite form blebs at silicate grain triple junctions.
Hornblendite rafts and xenoliths within the inland parts of the Misty Pluton have a granoblastic texture characterised by 120° triple junctions. They also include minor biotite and rutile.
Pyroxenite
The distinguishing feature of pyroxenite is a dominance of clinopyroxene and lack of olivine in the assemblage. Pyroxenites are minor and concentrated in the central and southern parts of the ultramafic body at Hawes Head. Pyroxenites are only associated with hornblendite, both in the coastal exposures around Hawes Head and inclusions further inland within the Misty Pluton. Pyroxenite was not observed in contact with olivine-bearing rocks. The pyroxenite inclusions in hornblendite are typically 1–10 cm across and vary in shape from equant to elongate (G). Larger inclusions 1–10+ m across occur in some inland localities. The inclusion shapes are typically rounded and irregular. The largest inclusion at a coastal outcrop (0802) is c. 1 m across and featured ‘jig-saw’ fit textures. The inclusion–host boundary is sharp (H).
Pyroxenite samples consist of clinopyroxene (up to 2 mm, 40–75 vol. %), orthopyroxene (up to 1.5 mm, 25–60 vol. %) and variable amounts of accessory minerals(E). Accessory minerals commonly include ilmenite, hematite and amphibole±rutile, biotite and apatite. Pyroxenite xenoliths within the Misty Pluton all contain minerals that are moderately to highly polygonal.
Western Fiordland Orthogneiss–Misty Pluton
Samples of the Misty Pluton include both the relatively melanocratic hornblende-rich and paler feldspar-rich two-pyroxene varieties of dioritic gneiss present in the study area. Allibone et al. (Citation2009b) have previously described the petrographic characteristics of the Misty Pluton. The lack of olivine and 40–60 vol.% plagioclase in the Misty Pluton are important features to note for this study. Accessory minerals in the hornblende-rich dioritic gneiss include rutile, ilmenite, biotite, garnet, apatite and zircon, whereas the paler two-pyroxene dioritic gneiss includes minor amphibole and K-feldspar but lacks rutile and garnet (F). Garnet is commonly of metamorphic origin, found associated with plagioclase-rich dykes 1–3 cm thick and adjacent reaction zones. Hornblendite dykes cut garnet-bearing plagioclase-rich dykes at the contact zone between the ultramafic rocks at Hawes Head and the Misty Pluton (0807; E). The contact zone between the Misty Pluton and the ultramafic rocks is described previously in the ‘Hornblendite and rare pyroxene hornblendite’ section.
Analytical methods
Whole rock geochemical analyses of twelve samples of ultramafic rock and two samples of the Misty Pluton from the vicinity of Hawes Head are presented in . The complete electron microprobe mineral geochemical dataset from the 27 samples analysed can be downloaded from the New Zealand Journal of Geology and Geophysics website (Online Resource 1).
Table 1 Major and trace element data for ultramafic rocks from Hawes Head and the Misty Pluton.
X-ray fluorescence (XRF)
Fresh, unaltered samples were initially crushed using a hydraulic press with tungsten carbide plates until rock fragments were <1 cm3 and divided repeatedly until 500 g of material is reduced to approximately 25 g. Samples were reduced to a fine powder using a tungsten carbide barrel and ring tema mill for 90 seconds.
Whole rock major and trace element concentrations were determined at SpectraChem Laboratory, Lower Hutt, New Zealand using a Siemens SRS 3000 sequential XRF spectrometer. For major element analysis, whole rock powders were fused into glass discs using a lithium borate flux at 1100°C. Nineteen trace elements were determined on a 10% w/w wax pressed powder disc with boric acid backing. Loss on ignition (LOI) was established by heating the samples to 1000 °C for 1 hour. Lower limits of detection and the LOI for the major oxides are 0.01 (wt%). Lower limits of detection for trace elements are 1 mg/kg with the exception of Sc, which is 2 mg/kg and Ba, Ce and La, which are 5 mg/kg.
Inductively coupled plasma-mass spectrometry (ICP-MS)
Samples were analysed for trace and rare earth elements by inductively coupled plasma-mass spectrometry (ICP-MS) at the Geochemical Analysis Unit, located in the Australian Research Council National Key Centre for Geochemical Evolution and Metallogeny of Continents at Macquarie University, Sydney, Australia. Powders were digested with concentrated HNO3 and HF and measured using 1/1000 and 1/5000 dilutions with He as the collision cell gas. Most data are taken from the 1/1000 run with the exception of Cr and Ni, which were taken from the 1/5000 run. Fourteen samples were analysed in two sets with a duplicate sample for quality control.
Diluted rock solutions were analysed by ICP-MS using an Agilent 7500cs ICP-MS Octopole reaction system with a self-aspiring micro-flow nebuliser. External calibration standards (BCR-2a) and US Geological Survey reference samples (BIR-1 and BHVO-2) were run at the start and end of both runs. Precision is typically better than 5% for each element. Analytical accuracy was determined on multiple analyses of US Geological Survey reference samples BHVO-2 and BIR-1 and is within 3% for all of the elements.
Electron microprobe analysis
Major element mineral chemistry was determined at the Geochemical Analysis Unit, Macquarie University, Sydney, Australia using a Cameca SX100 electron microprobe with five wavelength dispersive spectrometers and an energy dispersive spectrometer. Operating conditions were 15 kV accelerating voltage and 20 nA beam current using a 1–2 µm focused beam. The PAP matrix correction procedure (Pouchou & Pichoir Citation1984) was applied to all raw data. Precision and accuracy were checked by comparing analyses of a suite of geological and oxide standards of known composition.
Whole rock geochemistry
Major elements
The major oxides of the hornblende peridotite, hornblendite and pyroxenite are plotted against SiO2 in A. The hornblende peridotite has the lowest SiO2 contents (38.39–41.11 wt%). Five of the six elements shown (Al2O3, CaO, Na2O, TiO2, K2O) define linear arrays that correlate positively with increases in SiO2 content. However, a negative correlation is observed between SiO2 and MgO contents. All linear arrays project towards the average major element contents of the hornblendite samples (A). The five samples of hornblendite show little variation in their major oxide contents. They have slightly higher SiO2 contents (41.39–41.94 wt%) than the hornblende peridotite samples. The hornblendite samples have the highest Al2O3 (12.85–13.20 wt%), Na2O (2.48–2.61 wt%) and K2O (0.62–0.79 wt%) and lowest MgO (14.72–15.76 wt%) of all the rock groups. Samples of olivine–pyroxene hornblendite and pyroxenite are distinct from hornblende peridotite and hornblendite samples. They have variable but relatively high-silica contents (45.20–50.52 wt%), with limited variation in the other major elements.
Figure 4 Whole rock geochemical data. A, Major element oxide (wt%) versus SiO2. Note trend in hornblende peridotite samples, clustering of hornblendite samples, except for the olivine-pyroxene hornblendite. B, Primitive mantle normalised REE diagram showing hump pattern for 11 samples of ultramafic rock from Hawes Head. The pyroxenite sample (0805J) has a distinct pattern. Note the Misty Pluton and all ultramafic samples from Hawes Head (except for pyroxenite sample 0805J) display depletion of heavy REEs.
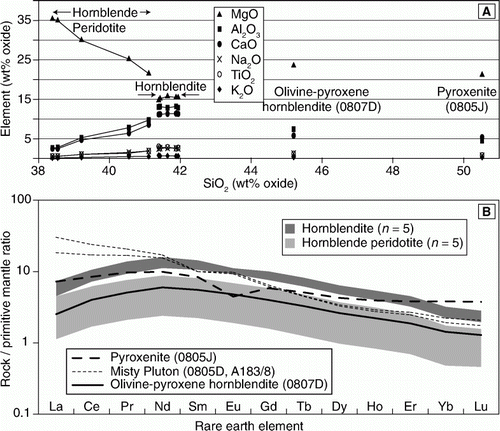
Trace and rare earth elements
The hornblende peridotite has the highest Ni contents (418–903 ppm), hornblendite the lowest (177–264 ppm) and pyroxenite is intermediate (463–620 ppm). Cr content is variable and commonly high (59–1093 ppm), whereas Rb, Zr and Nb contents are very low in all samples (<10 ppm, 16–64 ppm and <1 ppm, respectively). Sr/Y < 62. Rare earth elements are used to interpret petrogenetic relationships between the rock types. Eleven of the fourteen samples analysed show similar primitive mantle normalised patterns (B) with increasing light REE values and decreasing heavy REE values with an inflection point at Nd. One sample of pyroxenite (0805J) displays a flatter pattern with pronounced negative Eu anomaly. The two Misty Pluton samples have a similar pattern, with decreasing values throughout from light to heavy REEs.
Mineral chemistry
In this section, we outline the major element composition of the key minerals to characterise the samples and determine petrogenetic relationships between the rock types.
Olivine
Olivine is only found in samples of the hornblende peridotites and rare olivine–pyroxene hornblendites. It is not zoned and classifies as chrysolite with high Mg/(Mg+Fe) values between 0.77 and 0.82.
Amphibole
Calcic amphibole is common to all rock types except the pure pyroxenites. It classifies as pargasite to rare edenite (A). A systematic relationship between pargasite Mg-number and whole rock MgO content is apparent. The most MgO-rich hornblende peridotites contain especially magnesian pargasites, whereas the slightly less MgO-rich hornblendites contain less magnesian pargasites whose compositions overlap with some of the least magnesian pargasite grains in the hornblende peridotites. Pargasite grains from the adjacent Misty Pluton are even less magnesian, reflecting the dioritic–monzodioritic composition of the Misty Pluton (A). The amphibole compositions cluster within rock types, except for one xenolith sample of hornblendite found within the Misty Pluton (77676).
Figure 5 Mineral geochemistry data. A, Amphibole compositions (Leake et al. Citation1997) are mostly pargasite. Note no pyroxenite samples contained amphibole. B, Pyroxene ternary diagram. Enlargements show all data (lightest grey) as a background to the grouped samples, as per the key for 5A.
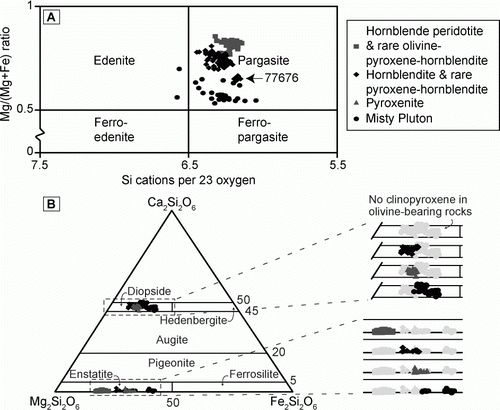
Pyroxene
Clinopyroxene is diopside and present in all rock types except for the olivine-bearing rocks. Orthopyroxene is enstatite and present in all rock types. A progressive increase in whole rock MgO content from the Misty Pluton diorite through pyroxenites, hornblendites and hornblende peridotites is reflected in increasing pyroxene Mg-numbers (B). Ti in all pyroxenes is generally less than 0.005 atoms per formula unit (apfu; on the basis of six oxygen) with the exception of two hornblendite samples (A181/8, up to 0.013; 0804H, up to 0.017), one olivine hornblende pyroxenite sample (0807D, up to 0.011) and one pyroxene hornblendite sample (0808B, up to 0.02). The tetrahedral Al values in enstatite are generally 0.05–0.10 apfu and generally 0.1–0.29 apfu in diopside.
Feldspar
Rare plagioclase is present in two hornblendite samples (0802B, 0809A) and one pyroxenite sample (0802C). Plagioclase is common in the Misty Pluton samples and a plagioclase dyke (0806A). Plagioclase compositions are variable within and across the Misty Pluton samples with Na/(Na+Ca+K)=0.57–0.86. Plagioclase compositions straddle the oligoclase–andesine boundary in the hornblendite and pyroxenite samples, with Na/(Na+Ca+K)=0.63–0.79. The plagioclase dyke (0806A) contains compositionally uniform oligoclase with Na/(Na+Ca+K)=0.75–0.76. Minor K-feldspar is present widely in the Misty Pluton (A183/8; K/(Na+Ca+K)=0.94–0.96) and very rarely in the plagioclase dyke (0806A; K/(Na+Ca+K)=0.95).
Spinel Group and Fe–Ti Oxides
Spinel group minerals are only found in the olivine-bearing rocks. Hercynitic spinel (Mg/(Mg+Fe)=0.59–0.62) was observed in all olivine-bearing samples. Magnetite was restricted to sample 0807D and contained minor amounts of Al (up to 0.07 apfu), Cr (up to 0.12 apfu), Ti (up to 0.08 apfu), Zn (up to 0.11 apfu) and Mg (up to 0.03 apfu; all on the basis of four oxygen).
Ilmenite and hematite end members of the FeTiO3–Fe2O3 series are both found within the rock types. Hematite is predominantly found in olivine-bearing rocks (0802G, 0801G, A180/8, 0802F, 0810B, 77641) and, more rarely, in hornblendite (76706, A181/8) and Misty Pluton (A183/8) samples. Ilmenite is rich in Fe3+ and found in hornblende peridotites (77641, 0802F, 0810B, 0807D, 76708, 0802G, 0801G, A180/8), hornblendites (A181/8, 0807E, 0804H, 0810A, 76706), Misty Pluton (A183/8), pyroxenite (77623) and altered pyroxenite (0805J) samples.
Discussion
The ultramafic rocks at Hawes Head in the deeply exposed arc root of Fiordland, New Zealand, provide a rare opportunity to examine potential amphibole cumulates formed within a Phanerozoic arc system. Previous studies indicate amphibole in cumulate rocks formed via two main mechanisms: (1) primary crystallisation from a magma and accumulation; and (2) as a reaction product involving consumption of early formed minerals (e.g. olivine) and hydrous melt or fluids (e.g. Larocque & Canil Citation2010). In the discussion, we argue for a cumulate origin of the ultramafic rocks at Hawes Head and explore relationships between the three components and their association with the Misty Pluton. We discuss the implications these rocks have for differentiation in magmatic arcs.
Evidence of a cumulate origin for ultramafic rocks from Hawes Head
Cumulate rocks are commonly ultramafic to mafic fractionates of a parental magma. The hornblende peridotite rocks provide the best evidence for a cumulate origin. Melt compositions with SiO2 contents as low as 38 wt%, FeO+MgO contents as high as 51 wt%, variable Cr contents up to 1000 ppm and low Rb contents less than 7 ppm are improbable. A dominance of early crystallising phases (e.g. olivine and orthopyroxene) supports a cumulate nature. Hornblende peridotite compositions plot on a tie line between olivine and hornblende and form well-defined trends in major element diagrams (A). These tie lines are essentially mixing lines between olivine-rich and amphibole-rich samples. This pattern is consistent with accumulation of variable modes of olivine and amphibole and supports our interpretation that these rocks are cumulate in origin. This inference also suggests olivine and amphibole were coeval liquidus phases. Minor intercumulus carbonate associated with clusters of spinel, ilmenite and hematite in these rocks suggests a mesocumulate texture (Wager et al. Citation1960).
The similar geochemistry of all hornblendite samples is due to their composition being dominated by one phase (amphibole). Although the major element geochemistry of the hornblendite samples overlaps basanitic melt compositions, partition coefficients for trace elements in amphibole (Adam et al. Citation2007) may be used to infer a cumulate origin for hornblendite samples. Our trace element data include a hump-shaped rare earth element pattern, high Ni and Cr and low Rb contents that point to a cumulate origin for hornblendite samples. Overlapping amphibole compositions between hornblendite and hornblende peridotite samples supports this inference. The limited amount of interstitial minerals between the amphibole grains suggests an adcumulate texture (e.g. Wager et al. Citation1960). Although difficult to argue on geochemical grounds, the similar field relationships of the pyroxenites to the other more common ultramafic lithologies at Hawes Head, their two-pyroxene mineralogy and a lack of plagioclase suggest the minor pyroxenites are also cumulates. The polygonal recrystallised textures of the pyroxenite limit interpretation of possible cumulate textures.
Relationships between ultramafic rocks from Hawes Head and their association with the Misty Pluton
The irregular, rounded, lobate and globular shape of rafts and xenoliths of hornblendite in hornblende peridotite and hornblende peridotite or pyroxenite in hornblendite are analogous to magma mingling textures (e.g. Vernon Citation1984). The simplest interpretation of these outcrop-scale textures is that each rock type behaved like a liquid at the same time, indicating each was likely a crystal mush with at least some interstitial liquid to aid fluidity during their interaction. This implies a close relationship between the ultramafic rock types. The inferred relationship is further supported by trends in the chemistry of the minerals in each rock type. For example, Mg-numbers for pargasite are highest in olivine-bearing rocks, lowest in the Misty Pluton and intermediate in hornblendites (A). A similar relationship is observed for pyroxene minerals with highest Mg-numbers in olivine-bearing rocks (orthopyroxene only), decreasing to hornblendites, pyroxenites and the lowest Mg-numbers in the Misty Pluton (observed in both clinopyroxene and orthopyroxene; B). There also exists a strong relationship in the whole rock geochemical data, whereby the linear array of hornblende peridotite samples projects towards hornblendite compositions.
The primitive mantle normalised REE patterns for the hornblende peridotite and hornblendite are very similar. This signature is a combination of the increasing ratio from La to Nd, which is a characteristic segment of the hornblende signature (Bottazzi et al. Citation1999; Davidson et al. Citation2007) and depletion of heavy REEs, interpreted as a primary feature of the parent magma. The distinct lack of Eu anomaly is consistent with a lack of plagioclase as an early-forming liquidus phase. One xenolith of pyroxenite (0805J) has a distinct REE pattern with negative Eu anomaly and flatter overall pattern, suggesting it is unrelated to the rest of the ultramafic rocks. The REE pattern of this pyroxenite more closely matches those of the Darran Suite (Muir et al. Citation1998), suggesting this sample represents a xenolith from an older source.
The mineral chemistry patterns and field relationships of ultramafic rocks at Hawes Head and the Misty Pluton indicate that olivine, pargasite and pyroxene minerals were early crystallising phases that accumulated in various proportions. An olivine–melt partition coefficient (KD=0.3; Roeder & Emslie Citation1970) is used to calculate the Mg-number of the melt that olivine grains were in equilibrium with. The result suggests olivine grains in the ultramafic rocks at Hawes Head crystallised from a melt with Mg-number=0.54–0.57. This range overlaps Mg-numbers of the Western Fiordland Orthogneiss () and is consistent with crystallisation of the cumulate minerals from the Western Fiordland Orthogneiss. In support of this interpretation, heavy REE depletion measured in the ultramafic rocks is consistent with crystallisation of the cumulate minerals from a heavy REE-depleted magma, such as the Western Fiordland Orthogneiss (Allibone et al. Citation2009b).
On the basis of field relationships, mineral assemblage, whole rock geochemistry and mineral composition, we interpret the ultramafic rocks from Hawes Head as cumulates and the three rock types as directly related.
Geochronological data are not available for the ultramafic rocks from Hawes Head. Mutually cross-cutting relationships between these rocks and the Misty Pluton indicate co-emplacement of the two units. U–Pb zircon ages indicate emplacement of the Misty Pluton at c. 120 Ma, shortly followed by garnet granulite metamorphism at c. 115 Ma (Stowell et al. Citation2010).
Magmatic differentiation in arcs
Allibone et al. (Citation2009b) used Pearce element ratio plots to examine major element composition trends in the suites of the Median Batholith. They found that geochemical trends in Darran and Separation Point suite rocks are controlled by fractionation and/or accumulation of plagioclase, as indicated by extensive linear trends on a bivariate plot of (Ca+Na+K)/Ti versus (Al–Ca)/Ti. In comparison, the Western Fiordland Orthogneiss geochemical data have a limited range of compositions on Pearce element ratio plots. However, limited fractionation and/or accumulation of clinopyroxene and/or amphibole is indicated by linear trends on a bivariate plot of (Fe+Mg)/Ti versus Ca/Ti (Allibone et al. Citation2009b). The dominance of amphibole in the ultramafic rocks at Hawes Head suggests that amphibole is likely the key mineral controlling their observed minor fractionation trends in the Western Fiordland Orthogneiss. Decreasing Dy/Yb values with increasing differentiation indices (e.g. SiO2) indicate cryptic amphibole fractionation (Davidson et al. Citation2007) in many arc suites. There is not enough published REE data on the Western Fiordland Orthogneiss to test for this relationship; this is highlighted as an area of suggested further research to test the importance of amphibole fractionation in this arc system. Comparison with experimental data for ‘dioritic’ liquids suggests that the ultramafic cumulates at Hawes Head likely crystallised from a magma with a high H2O content (>3 wt%) in order to suppress plagioclase on liquidus (Muntener et al. Citation2001), but the absence of garnet suggests H2O content was limited to less than c. 8 wt% (Alonso-Perez et al. Citation2009). The pressure of crystallisation is difficult to determine, but is likely between 0.8 and 1.2 GPa on the basis of the development of garnet granulite in the Misty Pluton (Stowell et al. Citation2010).
The ultramafic rocks at Hawes Head are an example of accumulation of the cryptic amphibole, and therefore H2O, interpreted from fractionation trends in many arcs. The density of all the ultramafic rock types at Hawes Head is estimated at between >3 g/cm3 and 3.2 g/cm3. The mingling field relationships described here between units are likely a result of density contrasts between crystal mush precursors to the hornblende peridotites, hornblendites and pyroxenites. The high density of these rocks approaches that of residual mantle peridotite, allowing recycling of cumulates like these into the mantle at the base of the arc. Other exposed deep sections of arcs, including Talkeetna and Kohistan, have large portions of the section missing (DeBari & Coleman Citation1989; Greene et al. Citation2006; Hacker et al. Citation2008). The mechanism of removal is controversial, but this may contribute to balancing the difference between primary basaltic arc magmas and the average andesitic composition of continental crust (Rudnick Citation1995; Kelemen et al. Citation2003; Kodaira et al. Citation2007).
Conclusions
We characterise three related ultramafic rock types at Hawes Head in western Fiordland – hornblende peridotite, hornblendite and pyroxenite – and show they are accumulations of early crystallising minerals from Early Cretaceous magmas of the Western Fiordland Orthogneiss. The ultramafic rocks at Hawes Head represent rarely exposed amphibole-rich cumulates inferred to be present near the base of many arcs, and point to open system evolution of arc magmas. The amphibole-rich cumulate rocks represent a reservoir for H2O storage in the lower arc crust and allow for removal of a portion of the H2O in primary arc magmas.
Electron microprobe mineral geochemical analyses
Download MS Excel (679 KB)Acknowledgements
Macquarie University Early Career Research Grant funding to NRD provided financial support to conduct this research. We thank the Department of Conservation, New Zealand for permission to visit and sample localities in the Fiordland National Park. Fieldwork was supported as part of the QMAP programme through Foundation for Research, Science and Technology (FRST) contract number C05X040. Critical reviews by two anonymous referees and the careful editorial work by M. Leybourne improved the manuscript. The analytical data were obtained using instrumentation funded by Department of Education, Science and Training (DEST) Systemic Infrastructure Grants, Australian Research Council (ARC) Linkage Infrastructure, Equipment and Facilities (LIEF), National Collaborative Research Infrastructure Strategy (NCRIS), industry partners and Macquarie University. This is contribution 844 from the Australian Research Council National Key Centre for the Geochemical Evolution and Metallogeny of Continents (http://www.gemoc.mq.edu.au).
Notes
Supplementary data available online at www.tandfonline.com/10.1080/00288306.2012.719910
Supplementary file 1 : Electron microprobe mineral geochemical analyses.
References
- Adam , J , Oberti , R , Camara , F and Green , TH . 2007 . An electron microprobe, LAM-ICP-MS and single crystal X-ray structure refinement study of the effects of pressure, melt-H2O concentration and fO2 on experimentally produced basaltic amphiboles . European Journal of Mineralogy , 19 : 641 – 655 . doi: 10.1127/0935-1221/2007/0019-1750
- Allibone , AH , Jongens , R , Scott , JM , Tulloch , AJ , Turnbull , IM , Cooper , AF , Powell , NG , Ladley , EB , King , RP and Rattenbury , MS . 2009a . Plutonic rocks of the Median Batholith in Eastern and Central Fiorldand, New Zealand: Field relations, geochemistry, correlation, and nomenclature . New Zealand Journal of Geology and Geophysics , 52 : 101 – 148 . doi: 10.1080/00288300909509882
- Allibone , AH , Jongens , R , Turnbull , IM , Milan , LA , Daczko , NR , De Paoli , MC and Tulloch , AJ . 2009b . Plutonic rocks of Western Fiorldand, New Zealand: Field relations, geochemistry, correlation, and nomenclature . New Zealand Journal of Geology and Geophysics , 52 : 379 – 415 . doi: 10.1080/00288306.2009.9518465
- Alonso-Perez , R , Muntener , O and Ulmer , P . 2009 . Igneous garnet and amphibole fractionation in the roots of island arcs: experimental constraints on andesitic liquids . Contributions to Mineralogy and Petrology , 157 : 541 – 558 . doi: 10.1007/s00410-008-0351-8
- Arculus , RJ and Willis , KJA . 1980 . The petrology of plutonic blocks and inclusions from the Lesser Antilles Island Arc . Journal of Petrology , 21 : 743 – 799 . doi: 10.1093/petrology/21.4.743
- Bottazzi , P , Tiepolo , M , Vannucci , R , Zanetti , A , Brumm , R , Foley , SF and Oberti , R . 1999 . Distinct site preferences for heavy and light REE in amphibole and the prediction of Amph/Ldree . Contributions to Mineralogy and Petrology , 137 : 36 – 45 . doi: 10.1007/s004100050580
- Bradshaw , JY . 1989a . Origin and metamorphic history of a polybaric granultic terrain, Fiordland, Southwest New Zealand . Contributions to Mineralogy and Petrology , 103 : 346 – 360 . doi: 10.1007/BF00402921
- Bradshaw , JY . 1989b . Early Cretaceous vein-related granulite in Fiordland, southwest New Zealand: a case for infiltration of mantle-derived CO2-rich fluids . Journal of Geology , 97 : 697 – 717 . doi: 10.1086/629353
- Cawthorn , RG , Curran , EB and Arculus , RJ . 1973 . A petrogenetic model for the origin of the calc-alkaline suite of Grenada, Lesser Antilles . Journal of Petrology , 14 : 327 – 337 . doi: 10.1093/petrology/14.2.327
- Clarke , GL , Klepeis , KA and Daczko , NR . 2000 . Cretaceous high-P granulites at Milford Sound, New Zealand: Metamorphic history and emplacement in a convergent margin setting . Journal of Metamorphic Geology , 18 : 359 – 374 . doi: 10.1046/j.1525-1314.2000.00259.x
- Daczko , NR and Haplin , JA . 2009 . Evidence for melt migration enhancing recrystallization of metastable assemblages in mafic lower crust, Fiordland, New Zealand . Journal of Metamorphic Geology , 27 : 167 – 185 . doi: 10.1111/j.1525-1314.2009.00811.x
- Daczko , NR , Clarke , GL and Klepeis , KA . 2001 . Transformation of two-pyroxene hornblende granulite to garnet granulite involving simultaneous melting and fracturing of lower crust, Fiordland, New Zealand . Journal of Metamorphic Geology , 19 : 549 – 562 . doi: 10.1046/j.0263-4929.2001.00328.x
- Davidson , J , Turner , S , Handley , H , Macpherson , C and Dossetto , A . 2007 . Amphibole ‘sponge’ in arc crust . Geology , 35 : 787 – 790 . doi: 10.1130/G23637A.1
- De Paoli , MC , Clarke , GL , Klepeis , KA , Allibone , AH and Turnbull , IM . 2009 . The eclogite–granulite transition: mafic and intermediate assemblages at Breaksea Sound, New Zealand . Journal of Petrology , 50 : 2307 – 2343 . doi: 10.1093/petrology/egp078
- DeBari , SM and Coleman , RG . 1989 . Examination of the deep levels of an island arc: evidence from the Tonsina Ultramafic–Mafic Assemblage, Tonsina, Alaska . Journal of Geophysical Research , 94 ( B4 ) : 4373 – 4391 .
- Ducea , MN and Saleeby , JB . 1996 . Buoyancy sources for a large, unrooted mountain range, the Sierra Nevada, California: Evidence from xenolith thermobarometry . Journal of Geophysical Research , 101 ( B4 ) : 8229 – 8244 . doi: 10.1029/95JB03452
- Garrido , CJ , Bodinier , JL , Burg , JP , Zeilinger , G , Hussain , SS , Dawood , H , Chaudhry , MN and Gervilla , F . 2006 . Petrogenesis of mafic garnet granulite in the lower crust of the Kohistan Paleo-Arc Complex (Northern Pakistan):Implications for intra-crustal differentiation of island arcs and generation of continental crust . Journal of Petrology , 47 : 1873 – 1914 . doi: 10.1093/petrology/egl030
- Greene , AR , DeBari , SM , Kelemen , PB , Blusztajn , J and Clift , PD . 2006 . A detailed geochemical study of island arc crust: The Talkeetna Arc Section, south-central Alaska . Journal of Petrology , 47 : 1051 – 1093 . doi: 10.1093/petrology/egl002
- Hacker BR , Mehl L , Kelemen PB , Rioux M , Behn MD , Luffi P 2008 . Reconstruction of the Talkeetna Intraoceanic Arc of Alaska through Thermobarometry . Journal of Geophysical Research 113 : B03204 . doi: 10.1029/2007JB005208
- Jagoutz , O , Muntener , O , Burg , JP , Ulmer , P and Jagoutz , E . 2006 . Lower continental crust formation through focused flow in km-scale melt conduits: the zoned ultramafic bodies of the Chilas Complex in the Kohistan Island Arc (NW Pakistan) . Earth and Planetary Science Letters , 242 : 320 – 342 . doi: 10.1016/j.epsl.2005.12.005
- Kelemen PB , Hanghoj K , Greene AR 2003 . One view of the geochemistry of subduction-related magmatic arcs, with emphasis on primitive andesite and lower crust . In : Rudnick RL Treatise on Geochemistry 3 : The Crust . Oxford , Elsevier-Pergamon . Pp. 593 – 659 .
- Kodaira , S , Sato , T , Takahashi , N , Miura , S , Tamura , Y , Tatsumi , Y and Kaneda , Y . 2007 . New seismological constraints on growth of continental crust in the Izu-Bonin intra-oceanic arc . Geology , 35 : 1031 – 1034 . doi: 10.1130/G23901A.1
- Kratzmann , D , Carey , S , Scasso , R and Naranjo , J-A . 2010 . Role of cryptic amphibole crystallization in magma differentiation at Hudson Volcano, Southern Volcanic Zone, Chile . Contributions to Mineralogy and Petrology , 159 : 237 – 264 . doi: 10.1007/s00410-009-0426-1
- Larocque , J and Canil , D . 2010 . The role of amphibole in the evolution of arc magmas and crust: The case from the Jurassic Bonanza Arc Section, Vancouver Island, Canada . Contributions to Mineralogy and Petrology , 159 : 475 – 492 . doi: 10.1007/s00410-009-0436-z
- Leake , BE , Woolley , AR Arps , CES . 1997 . Nomenclature of amphiboles: report of the subcommittee on Amphiboles of the International Mineralogical Association, Commission on New Minerals and Mineral Names . American Mineralogist , 82 : 1019 – 1037 .
- Mortimer , N . 2004 . New Zealand's Geological Foundations . Gondwana Research , 7 : 261 – 272 . doi: 10.1016/S1342-937X(05)70324-5
- Mortimer , N , Tulloch , AJ , Spark , RN , Walker , NW , Ladley , E , Allibone , A and Kimbrough , DL . 1999 . Overview of the Median Batholith, New Zealand: A new interpretation of geology of the Median Tectonic Zone and adjacent rocks . Journal of African Earth Sciences , 29 : 257 – 268 . doi: 10.1016/S0899-5362(99)00095-0
- Muir , RJ , Ireland , TR , Weaver , SD , Bradshaw , JD , Evans , JA , Eby , GN and Shelley , D . 1998 . Geochronology and geochemistry of a Mesozoic magmatic arc system, Fiordland, New Zealand . Journal of the Geological Society of London , 155 : 1037 – 1052 . doi: 10.1144/gsjgs.155.6.1037
- Muntener , O , Kelemen , PB and Grove , TL . 2001 . The role of H2O during crystalisation of primitive arc magmas under uppermost mantle conditions and genesis of igneous pyroxenites: An experimental study . Contributions to Mineralogy and Petrology , 141 : 643 – 658 . doi: 10.1007/s004100100266
- Oliver , GJ . 1977 . Feldspathic hornblende and garnet granulites and associated anorthosite pegmatites from Doubtful Sound, Fiordland, New Zealand . Contributions to Mineralogy and Petrology , 65 : 111 – 121 . doi: 10.1007/BF00371051
- Oliver , GJ . 1980 . Geology of the granulite and amphibolite facies gneisses of Doubtful Sound, Fiordland, New Zealand . New Zealand Journal of Geology and Geophysics , 23 : 27 – 41 . doi: 10.1080/00288306.1980.10424190
- Pouchou , JL and Pichoir , F . 1984 . A new model for quantative X-ray microanalysis of homogenous samples . Recherche Aerospatiale , 5 : 13 – 38 .
- Roeder , PL and Emslie , RF . 1970 . Olivine–liquid equilibrium . Contributions to Mineralogy and Petrology , 29 : 275 – 289 . doi: 10.1007/BF00371276
- Romick , JD , Kay , SM and Kay , RW . 1992 . The influence of amphibole fractionation on the evolution of calc-alkaline andesite and dacite tephra from the Central Aleutians, Alaska . Contributions to Mineralogy and Petrology , 112 : 101 – 118 . doi: 10.1007/BF00310958
- Rudnick , RL . 1995 . Making continental crust . Nature , 378 : 571 – 578 . doi: 10.1038/378571a0
- Rutherford , MJ and Devine , JD . 1988 . The May 18, 1980, Eruption of Mount St. Helens 3. Stability and chemistry of amphibole in the magma chamber . Journal of Geophysical Research , 93 ( B10 ) : 11949 – 11959 . doi: 10.1029/JB093iB10p11949
- Stowell HH , Parker KA , Gatewood MP 2010 . Sequential pluton emplacement, garnet granulite metamorphism, and partial melting during construction and modification of magmatic arc crust, Fiordland, New Zealand . Abstracts of the 20th Annual V.M. Goldschmidt Conference Knoxville, USA, Geochimica et Cosmochimica Acta 74 : A997 .
- Tiepolo , M and Tribuzio , R . 2008 . Petrology and U–Pb zircon geochronology of amphibole-rich cumulates with sanukitic affinity from Husky Ridge (Northern Victoria Land, Antarctica): Insights into the role of amphibole in the petrogenesis of subduction-related magmas . Journal of Petrology , 49 : 937 – 970 . doi: 10.1093/petrology/egn012
- Tulloch , AJ and Kimbrough , DL . 2003 . Paired plutonic belts in convergent margins and the development of high Sr/Y magmatism: Peninsular Ranges batholith of Baja-California and Median Batholith of New Zealand . Geological Society of America Special Paper , 374 : 275 – 295 .
- Turnbull IM , Allibone AH , Jongens R (compilers) 2010 . Geology of the Fiordland area . Institute of Geological and Nuclear Sciences 1:250 000 geological map 17 . 97 p + 1 sheet . Lower Hutt , , New Zealand . GNS Science .
- Vernon , RH . 1984 . Microgranitoid enclaves in granites - globules of hybrid magma quenched in a plutonic environment . Nature , 309 : 438 – 439 . doi: 10.1038/309438a0
- Wager , LR , Brown , GM and Wadsworth , WJ . 1960 . Types of igneous cumulates . Journal of Petrology , 1 : 73 – 85 . doi: 10.1093/petrology/1.1.73