Abstract
Early Palaeozoic basement rocks in South Westland consist of regionally metamorphosed Greenland Group of probable Ordovician depositional age. Correlative and formerly contiguous Gondwana margin rocks are also found in Antarctica and eastern Australia. Major protoliths are variably foliated sandstone and mudstone, with rare scattered calcareous lenses. Field, petrographic and electron microprobe data reveal a progressive, regional, southeast-wards increase in metamorphic grade in a 2600 km2 area between Martins Bay and Fox Glacier. We define new chlorite, biotite–albite, biotite–calcic plagioclase and sillimanite–microcline zones. Minerals such as cordierite and andalusite, along with semi-schistose textures, indicate a low P/T (pressure/temperature) Buchan style of regional metamorphism spanning the lower greenschist to upper amphibolite facies. This contrasts with medium to high P/T metamorphism in the Haast Schist east of the Alpine Fault. In South Westland, peak metamorphism is loosely constrained as Devonian to Carboniferous (330–375 Ma) in age; the present-day isograd pattern was mostly established by the Late Cretaceous and has not been strongly influenced by Alpine Fault deformation.
Introduction
The metamorphic geology of most New Zealand basement terranes has been relatively well characterised in reconnaissance (). Work in the last few decades, particularly in the Haast Schist and Fiordland, has identified regional metamorphic zones and related them to the structural and tectonic development of the Mesozoic Gondwana margin (e.g. Grapes & Watanabe Citation1992; Mortimer Citation2000; De Paoli et al. Citation2009; Scott et al. Citation2009a, Citationb). One geological unit that is relatively understudied from a metamorphic point of view is the Greenland Group of the Buller Terrane in New Zealand's Western Province. The Greenland Group is believed to underlie much of the offshore Challenger and Campbell plateaus and has correlatives in the Robertson Bay Group of Antarctica and the Lachlan Orogen of Australia (Gibson Citation1992). It is part of a regionally important and voluminous sedimentary unit of Early Palaeozoic Gondwana that hosts orogenic gold deposits. Limited work to date (e.g. Nathan Citation1978a, Citationb) indicates a lower greenschist facies grade of metamorphism for large areas of the Greenland Group.
Figure 1 Map of South Island, New Zealand showing major geological units and metamorphic belts. In part after Landis & Coombs (Citation1967), Mortimer (Citation2000) and White (Citation1994). Amphibolite facies rocks from the area of (box) are not shown at this scale. P, Paparoa Range; B, Bonar Range; F, Fiordland; A, Alpine Schist; O, Otago Schist.
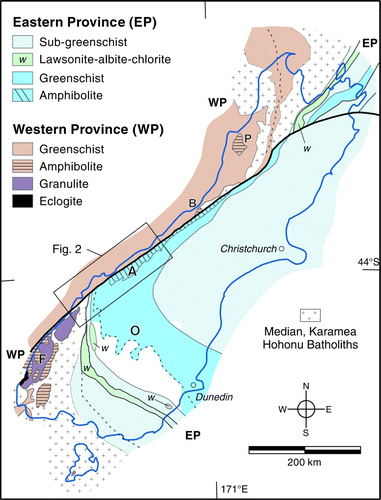
In this paper we report the results of a field and mineralogical investigation of Greenland Group rocks immediately west of the Alpine Fault in South Westland, between Martins Bay and Fox Glacier. This coastal strip is c. 250 km long but only 5–20 km wide (). In the Waitā River–Lake Paringa area (), the investigation is based on fieldwork, the examination of 125 thin sections and 420 analyses of plagioclase from 22 samples or portions of samples, supplemented by unpublished thesis work (Nathan et al. Citation1987; Bartlett Citation1993; Ryland Citation2011). The majority of these samples are metamorphosed sandstone (greywacke) and mudstone (argillite), but five calcareous concretions were also studied. In the Hope River–Arawhata River area (), we carried out field traverses and examined 18 thin sections. Elsewhere in the area of our investigation is more reconnaissance in nature and based partly on previous published work (Turner Citation1930, Citation1933), unpublished theses (Ransley Citation1983; Randall Citation2004) and field and petrographic work relating to recent 1:250 000 scale mapping of QMAP Haast sheet and adjacent sheets (Turnbull Citation2000; Cox & Barrell Citation2007; Rattenbury et al. Citation2010).
Figure 2 Location map of South Westland showing simplified geology (mainly after Rattenbury et al. Citation2010). Greenland Group index minerals, isograds and metamorphic zones are based on the more detailed work of this study. Alpine Schist isograds from Rattenbury et al. (Citation2010) and Cox & Barrell (Citation2007).
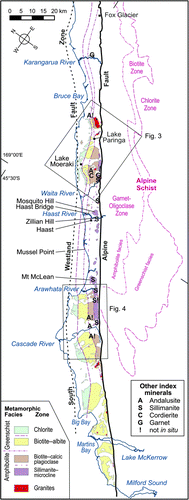
Figure 3 Map showing location of samples in the Waitā River–Lake Paringa area used for this study, and interpreted metamorphic zone boundaries. Background geology from Rattenbury et al. (Citation2010).
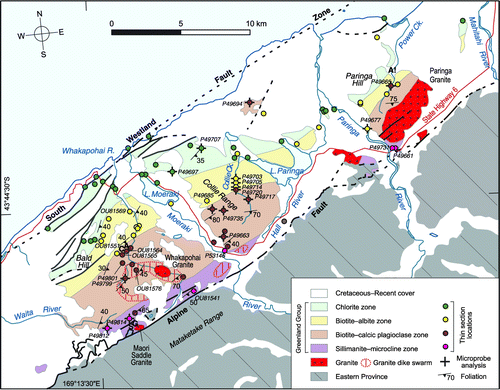
Figure 4 Map showing location of samples in the Hope River–Cascade River area used for this study, and interpreted metamorphic zone boundaries. Legend as for . Background geology from Rattenbury et al. (2010).
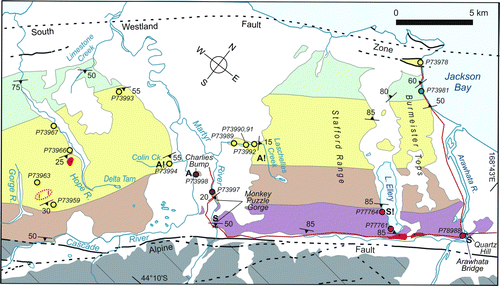
The purpose of this paper is to provide basic geological information on the metamorphism of Greenland Group rocks that can be compared and contrasted with other metamorphic sequences in New Zealand, and that can be used as a framework for regional investigations of mesothermal gold mineralisation. This paper also provides an appropriate platform for future, more detailed, metamorphic and structural studies of the Greenland Group.
Many of the samples used in this study were collected during geological mapping for the former New Zealand Geological Survey and for GNS Science. Samples prefixed ‘P’ and ‘OU’ have been catalogued in the GNS Science National Petrology Reference Collection and University of Otago Rock Collection, respectively. All sample data have been catalogued in the PETLAB database (http://pet.gns.cri.nz) from which location and analytical data can be obtained. Grid references refer to 1:50 000 New Zealand Map Series 260 maps.
Regional setting
Pre-Cretaceous rocks in South Westland are bounded on the southeast by the Alpine Fault and on the northwest by the South Westland Fault Zone (). Pockets of Late Cretaceous–Tertiary sediments rest unconformably, or as faulted outliers, above the pre-Cretaceous basement (Rattenbury et al. Citation2010). In the Haast area, a widespread veneer of Pleistocene moraines and postglacial sediments covers the pre-Quaternary rocks so that they are now only exposed as isolated hills. With minor exceptions (discussed below), there is a single, uniform pre-Cretaceous sedimentary lithology (Greenland Group, the subject of this paper) in South Westland.
In Buller and North Westland (north of our study area), the Greenland Group has been mapped at chlorite grade with local biotite hornfels and spotted schist adjacent to granite plutons (e.g. Turner Citation1933; Wellman Citation1955; Nathan Citation1978a, Citationb, Citation1996). In South Westland, apart from the pioneering reconnaissance of Turner (Citation1930, Citation1933) southwest of Haast and a summary in Rattenbury et al. (Citation2010), this is the first study to attempt to systematically document and define isograds and metamorphic zones.
Greenland Group protoliths
The Greenland Group consists of an Early Ordovician, quartz-rich flysch-type succession of interbedded sandstone and mudstone (Laird Citation1972). A moderate amount of petrographic and geochemical data are available, although most studied samples come from northeast of the area described here. Laird (Citation1972) showed that the sand-size fraction is dominated by detrital quartz, with minor (<6%) sodic plagioclase and minor fine-grained lithics. Roser et al. (Citation1996) summarised the petrochemistry, and showed that average Greenland Group sandstones had 71–75 wt% SiO2 and K2O > Na2O. In addition to these dominant rock types of the Greenland Group described above, some minor distinctive rock types are present locally.
In South Westland, calcite is widely disseminated in the matrix of many sandstones and mudstones. As a consequence, average whole rock CaO is generally higher than in samples from further north (c. 2.5 wt% versus 0.4 wt%; Roser et al. Citation1996). In greywackes calcite is scattered through the matrix as irregularly shaped grains, but in many argillites ankerite occurs as euhedral porphyroblasts which weather out as brown spots (e.g. P27687, P62786). Calcareous pods and concretions are found locally and, where metamorphosed, distinctive calc-silicates are formed (see later description; also Rattenbury et al. Citation2010, fig. 13B). Calc-silicate float is common in creeks draining the south side of the Collie Range () (e.g. P77780), and can also be found in situ as bands or lenses in the upper Waitā River (Bartlett Citation1993), at Arawhata Bridge () (Randall Citation2004) and at Mt McLean (P52269).
Metamorphosed calcareous horizons, including a pebbly limestone, outcrop at Big Bay (Mutch Citation1964; Laird Citation1986). Dacitic-rhyolitic volcanic rocks occur in Power Creek (e.g. P49674, 49675) and west of Lake Moeraki (P29108).
Conglomerates occur in Collie Creek (between G36/162139 and 164135) (e.g. P49714). Some conglomerate clasts consist of dacite–rhyolite, indicating that the aforementioned volcanic rocks are interbedded within the Greenland Group. On the south side of Big Bay, Laird (Citation1986) described conglomerate with clasts of sandstone, siltstone and minor mafic volcanics.
Intrusive rocks
Greenland Group forms the dominant basement lithology northwest of the Alpine Fault, but is locally intruded by small granitoid plutons or stocks. The largest pluton occurs either side of the Paringa River (). It is a 369±1 Ma granodiorite of the I-type Paringa Suite (Tulloch et al. Citation2009) cut by pegmatite dykes. A granite stock, the Whakapohai Granite, occurs in the upper Whakapohai River (Ryland Citation2011) and granite and pegmatite dykes occur in the surrounding region (). Another small pluton, Māori Saddle Granite, is found at the head of the Waitā River (Bartlett Citation1993) and has been U–Pb dated by Ryland at 385±4 Ma. Other small plutons and stocks occur near Mt McLean (Rb–Sr dated as Devonian by Aronson Citation1968), the mouth of Lake Ellery (), the Gorge River headwaters and south of Big Bay. Concentrations of granite and pegmatite dykes have also been noted in the Martyr River to Arawhata Bridge area (Turner Citation1930; Ransley Citation1983; Randall Citation2004).
Unlike North Westland, South Westland lacks batholith-size intrusions at the surface and the Greenland Group has locally been intruded only by granitoid dykes and small stocks of Late Devonian age (Figs ; Aronson Citation1968; Tulloch et al. Citation2009). There are no known Cretaceous plutons in the area of . Scattered Cretaceous lamprophyre dykes and rare outliers of Late Cretaceous and Palaeogene basalt (Cox & Barrell Citation2007; Rattenbury et al. Citation2010) are unlikely to have added enough crustal heat to affect Greenland Group regional metamorphism.
Greenland Group metamorphism
In typical low-grade Greenland Group rocks, a penetrative S1 cleavage is common and is usually (but not always) axial planar to folded bedding. Mesoscopic folds are rarely observed and 100–1000 m scale folds in bedding are inferred on the basis of local changes in facing directions and bedding-cleavage angles. Folds were not mapped as part of this study. In thin section, cleavage is manifested by preferred orientation of chlorite and sericite. Thus the lowest-grade rocks are all at least textural zone IIA as used in the Torlesse Terrane and Otago Schist of New Zealand's Eastern Province (Turnbull et al. Citation2001). Unlike the Torlesse Terrane, prehnite and pumpellyite have not been reported as matrix minerals (or found in this study, except in veins) and the lowest represented metamorphic grade in the Greenland Group appears to be the chlorite zone of the greenschist facies.
Waitā River–Lake Paringa area
Chlorite zone
In the vicinity of lakes Moeraki and Paringa (), the lowest-grade Greenland Group rocks are typically pale green to greenish-grey in colour due to the presence of finely disseminated chlorite. All samples have a distinct cleavage, marked in thin section by alignment of chlorite and sericite (A). Despite recrystallisation of the matrix, the original clastic texture is still clear. Detrital and metamorphic plagioclase composition, measured by electron microprobe, is invariably albite (), but whether the detrital plagioclase is primary or secondary is not known. Small, spherical carbonate concretions were noted in Collie Creek, and are marked in the field by brown-weathering patches which weather preferentially.
Figure 5 Thin section images of representative metamorphic rocks from the area. A. chlorite zone P49697; B. biotite–albite zone P49703; C. lower biotite–calcic plagioclase zone P49685; D. upper biotite–calcic plagioclase zone P49663; E. upper biotite–calcic plagioclase zone with biotite porphyroblasts P49801; F. P73998 upper biotite–calcic plagioclase zone with andalusite porphyroblasts (dark grey); G. sillimanite–microcline zone with coarse-grained abundant cross-hatched microcline AC-CT-44; H. sillimanite–microcline zone with large sillimanite porphyroblasts, partly replaced by fibrolitic sillimanite, and mantled by muscovite P78988. All images plane polarised light, width 3 mm except for G (cross-polarised light) and H (9 mm wide). S1 foliation is present all samples and is oriented top right to bottom left. A–D show progressive coarsening of biotite (dark), with progressive loss of detrital texture (b, biotite; a, andalusite; k, microcline; s, sillimanite).
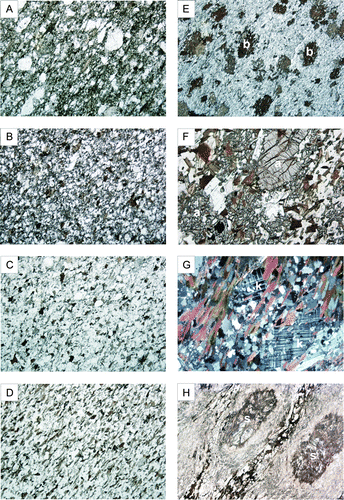
Table 1 Selected electron microprobe analyses of minerals. Analyses were performed between 2 and 30 Jan 1987 on a JEOL 8600 machine at the University of Otago using wavelength dispersive spectrometry and ZAF corrections with 15 kV and 20 nA beam conditions. Easting and northing refer to NZMS 260 map series, oxide concentrations are in wt%. Gw, greywacke; Gw+cc, greywacke with modal calcite; Bi–alb, biotite–albite; Bi–plag, Biotite–calcic plagioclase; Sill–mic, Sillimanite–microcline; An, molecular% anorthite in plagioclase feldspar. The location of P49752 is identified with a ‘G’ in the top part of .
Biotite–albite zone
Mineralogically, the biotite–albite zone is characterised by the presence of biotite in thin section (B), with albite as the only plagioclase (). Continuous sequences through the transition from the chlorite zone into the biotite zone are exposed near Lake Moeraki and in the lower reaches of Collie Creek (). The first appearance of biotite defines a biotite isograd, at virtually the same place in both sandstones and mudstones. In hand specimen, the greenish colour typical of the chlorite zone disappears at about the biotite isograd and the rock becomes dark grey. Within a few hundred metres this changes to dark grey-brown, and the rock becomes more massive and flinty in texture as the degree of recrystallisation increases. In thin section, biotite first appears as small anhedral grains in sandstones, parallel to cleavage. In accompanying mudstones, fine-grained biotite appears parallel to S1 in the matrix (up-grade, biotite occurs as better-crystallised discrete reddish-brown crystals dispersed throughout the rock). Small calcareous concretions occur in Collie Creek; their mineralogy is similar to those in the chlorite zone, except that the groundmass is recrystallised to a granoblastic aggregate of quartz, albite, calcite and biotite.
There is considerable textural variation in rocks of the biotite–albite zone, with increasing recrystallisation accompanied by weaker mesoscopic cleavage, though not necessarily microscopic fabrics, up-grade (compare A–F). In psammitic rocks, the original detrital grain size range of framework and matrix is commonly still visible, but the groundmass becomes recrystallised to a fine-grained granoblastic aggregate and the boundaries of the larger clastic quartz and feldspar are less sharp (B). Pelitic lithologies are completely recrystallised, and there is a gradual up-grade increase in the grain size of muscovite and biotite.
Biotite–calcic plagioclase zone
In psammitic and pelitic lithologies near lakes Moeraki and Paringa, the low-grade limit of the biotite–calcic plagioclase zone is marked by a change in plagioclase composition from albite to more calcic compositions. This is recognised in thin section by an increase in feldspar refractive index, and has been confirmed by numerous electron microprobe analyses (, see below). In calcareous concretions, calc-silicate assemblages include extremely calcic plagioclase (An>80), zoisite and a number of other calcium-bearing minerals (described below). These calc-silicate pods generally lack biotite and are characteristically coloured white with black speckling (amphibole).
Detailed sampling was conducted in Collie Creek, and here the down-grade limit of the biotite–calcic plagioclase zone can be located to within c. 100 m. Texturally, rocks within the zone are tough and highly recrystallised and are described in the field as hornfels. In thin section, meta-sandstone lithologies typically have granoblastic to semi-schistose and weakly gneissic textures, with variable to weak alignment of micas (C, D). However, porphyroblastic (E) and decussate (randomly oriented) textures are observed in places. Variation in grain size, especially large quartz crystals (possibly relict clasts) in a finer grained groundmass, is typical of the lower part of the zone; up-grade, the rocks become more equigranular however. Original bedding lamination, shown by variations in mica and quartz content, can sometimes be seen (e.g. P49668). Many pelitic (mica-rich) samples also contain large poikiloblasts of biotite and chloritoid, both with small quartz inclusions (e.g. P44874).
Sillimanite–microcline zone
Petrographic examination of some rocks with calcic plagioclase revealed the presence of small patches of metamorphic potassium feldspar which coexists with muscovite (G is a particularly well-developed example). Microcline has been identified petrographically by its characteristic cross-hatch twinning and low refractive index in four rocks – P49812, 49814, 49731 and 53146 – and confirmed by electron microprobe in P49812 and 49814 ().
We have not comprehensively investigated the structural state of alkali feldspar in our sample set so cannot entirely exclude the occurrence of orthoclase. However, for convenience and simplicity, we refer to the potassium feldspars as microcline. The microcline-bearing rocks define a fourth (and highest-grade) metamorphic zone in the region. Sillimanite is also an index mineral for this zone. Sillimanite in the area of was reported by Bartlett (Citation1993) and it also occurs just to the southwest (see ). Its rarity in the Waitā River–Lake Paringa area is probably due to a bias in sampling and thin sectioning of psammitic rather than pelitic protoliths. The limited number of microcline- and sillimanite-bearing samples means that the extent of this metamorphic zone as shown on Figs is not as precisely located as the other zones.
In the Waitā River–Lake Paringa area, rocks within the sillimanite–microcline zone are texturally similar to those in the biotite–calcic plagioclase zone, with hornfelsic semi-schists being present. However, there are also rocks that are clearly gneissic in hand specimen. Ryland (Citation2011) presents detrital zircon data that confirms a Greenland Group protolith for paragneiss OU81541.
Other metamorphic minerals
The Ti-bearing metamorphic phase in all the P-sample thin sections of the Waitā River–Lake Paringa area is titanite; this also applies to rocks with microcline in them. Neither garnet nor ilmenite were observed in any of the meta-sandstone or meta-mudstone lithologies, although garnet is reported from gneisses near the Alpine Fault by Bartlett (Citation1993). The persistence of metamorphic titanite throughout the zones suggests to us that it is at least partly stabilised by the high Ca composition of the protoliths. In calc-silicate nodules, pale-green pleochroic amphibole (actinolite and/or hornblende) accompanies epidote-group minerals in the biotite–calcic–plagioclase zone (P49661, 49663, 49717, 49815, 77780) but neither mineral is present in the single sample of the biotite–albite zone (P49705); none of the five calc-silicate pods examined by us contained pyroxene or garnet; Bartlett (Citation1993) recorded garnet in some calc-silicate nodules, however.
Some non-calcareous samples in the biotite–albite zone contain chloritoid poikiloblasts e.g. P49798 in the Whakapohai River. A nearby pelitic rock P49766B contains patches of cordierite in mica-rich laminae. Ryland (Citation2011) reported cordierite in OU81564 and 81565 and biotite and quartz replacement of probable cordierite in OU81551 and 81569, all in the Whakapohai River. P52824, a float boulder from Power Creek, consisted of a biotite–muscovite–andalusite assemblage with almost no quartz and feldspar. Andalusite was identified from crystal shape, having been retrograded to a mass of fine-grained muscovite.
Ryland (Citation2011) reported andalusite in situ in tributaries of the Whakapohai River in OU81564 and 81576 and Bartlett (1993) noted almandine garnet in his sillimanite sample ().
In many rocks close to the Alpine Fault, biotite is retrogressed to chlorite, plagioclase is strongly sericitised, coarse epidote is replaced by finer-grained secondary epidote aggregates and prehnite occurs as veins (e.g. P49661). Prehnite is also observed as a vein mineral in P49677 and 49731.
Hope River–Arawhata River area
Chlorite zone
From the lower Hope River northeast to Jackson Bay (), Greenland Group rocks furthest from the Alpine Fault are chlorite zone. Petrographically, they are identical to those described above from the Waitā River–Lake Paringa area. In the lower Hope River, tight, northeast-trending folds overturned to the northwest are recognised. An associated S1 axial planar slaty cleavage dips moderately to steeply southeast.
Biotite–albite zone
Towards the Alpine Fault metamorphism increases to biotite–albite grade, with biotites forming parallel to what is still a slaty cleavage. In the least-recrystallised rocks, fine-grained biotite and muscovite tend to grow parallel to foliation and largely replace fine-grained chlorite (e.g. P73967, P73993), but become increasingly porphyroblastic and decussate up-grade. In pelitic rocks at Laschelles Creek, biotite is clearly visible in hand specimen; in thin section it is larger and more decussate compared to adjacent psammites (e.g. P73989). In P73978, from the northwest end of Jackson Bay, the biotite habit is both decussate porphyroblasts and grains parallel to foliation.
Biotite–calcic plagioclase zone
Southwest of the Arawhata River, plagioclase composition has been estimated only by extinction angle and refractive index means; several thin sections indicate oligoclase rather than andesine compositions (e.g. P73997 and 73998; Turner Citation1930). Interestingly, these samples are generally calcite free suggesting that the oligoclase here versus andesine in the Waitā River–Lake Paringa area is a consequence of locally less-calcic protoliths.
Semi-schist texture is developed in the more pelitic lithologies, and metamorphic biotite and muscovite are clearly visible in hand specimen. Up-grade, bedding becomes progressively more subparallel to foliation, especially at Charlies Bump where quartz veins are tightly folded parallel to a northeast-striking moderately east-dipping foliation. Thin section microtextures reveal that much of the biotite is decussate however, and at Charlies Bump andalusite overgrows foliation (P73998) suggesting that recrystallisation outlasted strain.
At the downstream end of the Monkey Puzzle Gorge in the Martyr River, an eastward increase of textural grade is observed towards the sillimanite–microcline zone. Biotite hornfels occur at E38/527681 whereas semi-schist, with mica size >1 mm, occurs 300 m to the southeast.
Sillimanite–microcline zone
Metasediments from the upstream end of Monkey Puzzle Gorge (at the road bridge) northeast-wards to the Arawhata Bridge are gneissic and belong to the sillimanite–microcline zone (). Sillimanite is recorded in P77764 and P78988 (see also Turner Citation1930; Ransley Citation1983; Randall 1984). Plagioclase compositions range from calcic oligoclase to andesine (see also Turner Citation1933). Textures in pelitic layers are strongly gneissic, whereas interlayered meta-sandstones display more granoblastic textures. Biotite is strongly aligned parallel to foliation in both cases. Both segregation layering and boudinaged leucocratic veins are common in the pelitic layers (e.g. Rattenbury et al. Citation2010, fig. 15). Sillimanite, partly retrogressed to muscovite, commonly occurs as elongate porphyroblasts (up to 1 cm) parallel to the gneissic foliation, and adjacent strain shadows can be asymmetric (e.g. P78988, H). Good examples of sillimanite-bearing pelitic gneiss can be found on the south bank of the Arawhata Bridge where muscovite-bearing pegmatite dykes are folded with the gneiss and with calc-silicate bands (Randall Citation2004).
At the upstream end of Monkey Puzzle Gorge, gneissic foliation, compositional layering and muscovite-bearing pegmatite dykes are all subparallel, strike N–NE and dip moderately to the SE. The textural change from hornfels and semi-schist downstream of the gorge to gneiss on the upstream end occurs over a distance of <2 km (). Despite searching by us in the vicinity of the gorge and in the gorge itself (N. Barth, pers. comm. 2010), no faults have been observed to explain this rapid change in textural grade and we infer it to be gradational.
Other metamorphic minerals
Porphyroblastic chloritoid is present, accompanying biotite, as porphyroblasts in P73991 and P73992 in Laschelles Creek. At Charlies Bump elongate andalusite with inclusions of quartz, plagioclase and biotite occurs in biotite–calcic plagioclase zone (P73998; F); andalusite was also found by Turner (Citation1930) in boulders of Colin Creek, south of the Cascade River. Probable retrogressed andalusite was identified from a boulder in Laschelles Creek (Turner Citation1933).
Arawhata River–Waitā River area
Greenland Group exposure is confined to isolated hills () and we rely heavily on Randall (Citation2004) as well as scattered new observations. Most of the area is apparently underlain by sillimanite–microcline zone rocks. At almost every outcrop, pegmatitic granitic rocks, as concordant foliation-parallel intrusions, are recorded as being present (especially at Mount McLean). A narrow shear zone, in which the granites are strongly deformed and boudinaged, is seen at Mussel Point. Microcline has been confirmed in Greenland Group gneisses by Randall (Citation2004) and by us at Mount McLean (OU74696, P52270) and Quartz Hill (OU74700).
South of Mosquito Hill, fibrolitic sillimanite appears with microcline and andesine. Randall (Citation2004) reports sillimanite at Zillian Hill and the adjacent Grassy Bluff (OU74664, OU74706, 74707 74712) and at Mount McLean (OU74696). Rare garnet has been observed in hand sample at Haast Bridge, although efforts to relocate and resample this have proved unsuccessful. Randall (Citation2004) reported a zoisite-bearing calc-silicate pod at Mount McLean (OU74695) and we have observed diopside in calc-silicate sample (P52269) from the same location. In the above cases, sillimanite appears in relatively micaceous rocks of pelitic bulk composition, but is absent from accompanying psammites.
Northeast of Lake Paringa
Greenland Group outcrops are rare northeast of Bruce Bay and there are few available samples with which to establish metamorphic grade. One sample P49752 from the Karangarua River is the only rock in our entire South Westland Greenland Group sample set that contains metamorphic garnet (G in ). P49752 is a foliated garnet–biotite–muscovite–andesine–quartz gneiss (). Anhedral poikiloblastic garnets are partly retrograded to chlorite.
Discussion
Progressive compositional change of plagioclase
A continuous section from chlorite through biotite–albite to biotite–calcic plagioclase zones is exposed in Collie Creek (). Within the chlorite and biotite–albite zones the only feldspar present is albite, almost always with composition An0-1 (, ). The original detrital shape of many plagioclase grains is still clear, especially within the chlorite zone (A), but the albitised composition indicates that they have been affected by low-grade metamorphism. P49720 contains two distinct plagioclase populations, An0-2 and An34-41, that occur as discrete grains and possibly bracket the peristerite gap (Grapes & Otsuki Citation1983; Yardley Citation1989). This is taken to mark the base of the biotite–calcic plagioclase zone. Still further up-grade, the next sample in Collie Creek (P49717A) contains no albite but has a range of compositions from An26-47 with most An33-37; all are outside the peristerite range (). Other rocks in our analysed biotite–calcic plagioclase zone dataset have mean and median compositions of An29-44 (i.e. almost entirely andesine), although minimum compositions in seven out of eight samples is An19-30 (i.e. oligoclase). This presence and abundance of andesine contrasts with oligoclase compositions widely recorded in other lower amphibolite facies sequences, for example within the Haast Schist on the opposite side of the Alpine Fault (Cooper Citation1972; Turner Citation1981; Grapes & Otsuki Citation1983). We believe the variably calcic nature of the plagioclase in our dataset can be explained by the presence of modal calcite and/or zoisite in many of our analysed rocks that are caused by locally higher whole rock CaO contents in parts of South Westland (Roser et al. Citation1996). Because of this wide variation in whole rock and plagioclase compositions, we prefer to name the metamorphic zone after calcic plagioclase instead of oligoclase or andesine. We have not used feldspars in calc-silicate pods to define metamorphic zones as their anorthite content is even more variable ().
Figure 6 Plagioclase compositions used to define metamorphic zones, all from the Waitā River–Lake Paringa area except for P49752 which is a garnet-bearing sample from the Karangarua River. Note dependence of anorthite content on rock type. Samples are arranged in approximate sequence of increasing metamorphic grade.
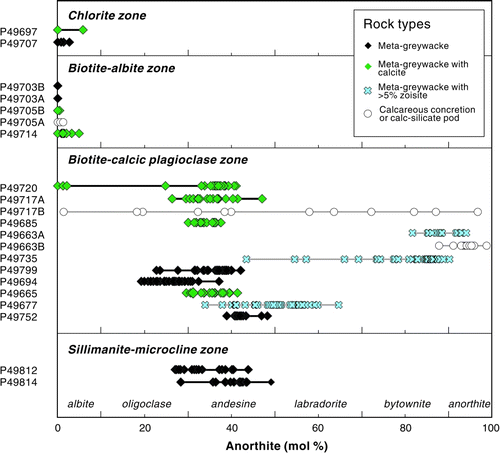
Metamorphic zones
As shown in , we define four progressive metamorphic zones of regional extent in the Greenland Group of South Westland. These zones are defined on (1) presence and absence of biotite in psammitic and pelitic lithologies; (2) changing Na-Ca-K feldspar composition in psammitic and pelitic lithologies; and (3) presence and absence of sillimanite in pelitic lithologies. Andalusite and cordierite can occur in some pelitic lithologies in the biotite–calcic plagioclase zone; however, their only occasional appearance () restricts their use as index minerals. In the limited scope of this study we have not attempted to establish prograde mineral reactions on the basis of modal changes in mineralogy.
The common use of the field term hornfels by earlier authors might be taken to suggest a contact rather than a regional style of metamorphic overprint. Microcline and sillimanite, index minerals for the highest grades of metamorphism, are indeed commonly (but not exclusively) observed adjacent to dykes, stocks and bosses and/or close to granitoid swarms, suggesting a causal relationship of metamorphism to heat sources. The alignment of biotite in some higher-grade rocks may be mimetic, that is, inherited from pre-existing alignment of older micas but without any strain field during the later biotite growth. This would explain the occurrence of micas both subparallel to foliation, with decussate textures, and a lack of strain shadows around biotite porphyroblasts (E). In contrast, other observations favour a regional rather than contact metamorphic interpretation: an S1 foliation defined by metamorphic micas is almost always present in the chlorite and biotite–albite zones (A, B) and is also usually present in biotite–calcic plagioclase and sillimanite–microcline zone rocks (C, D, F–H). In pelitic rocks in the sillimanite–microcline zone, strain appears to have accompanied peak metamorphism with elongate sillimanite porphyroblasts having adjacent strain shadows (H).
That some thin sections show semi-schistose textures and others more randomly oriented textures (e.g. D, E) is possibly a function of protolith (psammitic versus pelitic), of how the thin section was cut relative to foliation and of local strain conditions. At all metamorphic grades, there has been strong strain partitioning between psammitic and pelitic lithologies. The mica foliation in psammitic lithologies, even in the sillimanite–microcline zone, is often very weak, hence the widespread use of the field term ‘hornfels’. In contrast, adjacent pelitic lithologies are well-foliated and, at higher metamorphic grades, display gneissic and boudinage structures (e.g. Rattenbury et al. Citation2010, fig. 15A). The presence of a single penetrative foliation across and along the entire metamorphic sequence, including in andalusite- and sillimanite-bearing rocks (Figs ) lends support to a single regional metamorphic event.
Based on a consideration of the above, we propose a provisional interpretation in which mineral growth in a single Palaeozoic regional metamorphic event outlasted most, but not all, S1 strain in the Greenland Group. The heat source for metamorphism is probably related to intrusions. This accounts for the single foliation that is present across the SE-increasing metamorphic field gradient, as well as the lack of clear spatial association of andalusite occurrences to small granitoid bodies (). We are less convinced – but cannot entirely discount – two variations: (1) that the biotite–albite, biotite–calcic plagioclase and sillimanite–microcline zones are a Palaeozoic contact metamorphic overprint on a chlorite zone regional metamorphism with most high-grade foliations being mimetically inherited; and/or (2) that a later (Cretaceous?) thermo-tectonic event locally reactivated the Palaeozoic foliation in the sillimanite–microcline zone. Further geochronological and microtextural studies are needed to fully resolve these issues.
Conditions of metamorphism
The presence of just one foliation, presence of cordierite, andalusite and sillimanite, absence of kyanite and staurolite and extreme rarity of garnet are all indicative of regional metamorphism at relatively low pressures (Yardley Citation1989). The style of South Westland Greenland Group metamorphism is therefore akin to that seen in other relatively low pressure/temperature (P/T) orogens described from around the world (e.g. Buchan zones of northeast Scotland, Ryoke Belt of Japan and Hercynian Belt of Europe; Turner Citation1981). The simple, single penetrative foliation and low P/T metamorphism of the Greenland Group contrasts with polydeformed medium P/T Barrovian styles of metamorphism such as in the now adjacent Mesozoic Alpine and Otago schists (Grapes & Watanabe Citation1992; Mortimer Citation2000).
A relatively low P/T field gradient is supported by provisional (i.e. not backed by detailed core-rim and microtextural data) mineral compositions of our one analysed garnet-bearing sample P49752 (, ). Textural relations of feldspars and micas in P49752 are similar to that shown in D; rare anhedral garnet grains are in clean mutual contact with micas and feldspars, except for some chlorite pressure shadows partly enclosing some grains. Reconnaissance thermobarometry using the garnet–biotite thermometer (Ferry & Spear Citation1978; Kleeman & Reinhardt Citation1994) and the garnet–biotite–muscovite–plagioclase geobarometer (Ghent & Stout Citation1981; Hoisch Citation1990) yields conditions of c. 580°C and palaeo-pressures of c. 0.4 Mpa (these results should be considered indicative and provisional only). This pressure is approximately the same as the emplacement depth of the Paringa Granodiorite (Tulloch & Challis Citation2000).
Age of metamorphism
Existing Greenland Group geochronological data from the area of are plotted in , allow us to interpret the data in the context of our new metamorphic zones. Apatite fission track, zircon fission track, whole rock K–Ar and whole rock Rb–Sr isochron ages all show broad inverse relationships with metamorphic grade (equivalent to distance from the Alpine Fault), which we interpret to indicate a protracted and episodic metamorphic and exhumation history. Adams (Citation2004) has previously argued for a low-grade regional metamorphic event at 450±10 Ma followed by a younger thermal overprint associated with Devonian–Carboniferous granitoids. Our thin sections show that detrital grains, notably muscovite, are present in most chlorite zone rocks and even in some biotite–albite zone rocks. The reported Silurian–Ordovician K–Ar and Rb–Sr whole ages (even from seemingly well-crystallised pelites) are therefore likely to be a difficult-to-resolve mix of metamorphic and detrital source area ages.
Figure 7 Published apatite fission track (AFT, diamonds), zircon fission track (ZFT, squares), whole rock potassium–argon (K-Ar, circles), laser argon–argon (Ar-Ar, pentagon) and whole rock rubidium–strontium (Rb-Sr, stars) ages from metamorphosed Greenland group from the area of plotted against metamorphic grade. Two sigma errors bars shown for ZFT ages; all other age errors are less than the symbol size. The shaded colour areas (outline for Rb-Sr) highlight the range of isotope ages for the four metamorphic zones. Spread of ages in chlorite zone reflects detrital contamination, spread of ages in sillimanite–microcline zone reflects protracted exhumation. Data from Adams et al. (Citation1975), Seward & Nathan (Citation1990), Kamp et al. (Citation1992), Adams & Kelley (Citation1998), Nathan et al. (Citation2000) and Adams (Citation2004). Reference data in lower panel from Aronson (Citation1968), Ireland & Gibson (Citation1998), Nathan et al. (Citation2000), Tulloch et al. (Citation2009), Hiess et al. (Citation2010) and Scott et al. (Citation2011). MCCs, metamorphic core complexes.
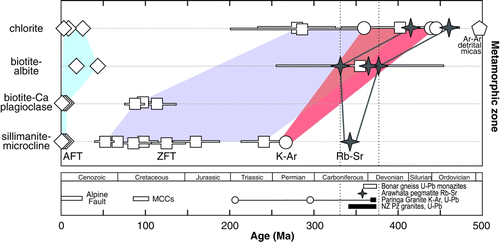
The four Rb–Sr ages from higher-grade rocks lie within a narrower 330–375 Ma range. We suggest that this is the best estimate of ages related to prograde regional metamorphism and immediate cooling that can currently be obtained from the Greenland Group in South Westland. Support for this age of metamorphism comes from a lone U–Pb age of 358 Ma from a zircon rim in OU81541 () (rejected by Ryland Citation2011), and from ages of other West Coast South Island plutonic and high-grade metamorphic rocks ().
Controls on isograd geometry
Where mapped in some detail (e.g. ) the boundaries between our metamorphic zones are unfaulted and we presume the same applies throughout South Westland. The strike of the isograds in map pattern is very well defined (), but neither our sampling density nor the local topographic relief is sufficiently high to establish the dip of isograds. The S1 foliation in South Westland generally dips moderately to steeply to the southeast (, ). Because there is no consistent steepening or shallowing of the foliation with proximity to the Alpine Fault, we infer that, to a first approximation, the entire Greenland Group between the South Westland and Alpine faults can be treated as a single rigid structural block. The unconformity beneath the mid-Cretaceous Otumotu Formation at Bald Hill and Paringa Hill (Rattenbury et al. Citation2010) indicates that, at least locally, the chlorite and biotite–albite zones had been exhumed to the surface by at least 95–105 Ma.
Alpine Fault
In South Westland, the Alpine Fault separates amphibolite facies rocks of the Western Province Greenland Group from amphibolite facies rocks of the Eastern Province Alpine Schist (). Turner (Citation1933) identified this two-sided metamorphic culmination but did not recognise the Alpine Fault. In his earlier 1930 paper he did however recognise the distinction between the ‘Older Metamorphic Series’ (Western Province) and the younger ‘Maniototo Series’ (Eastern Province). Today, the subparallelism of the isograds in the Alpine Schist to the strike of the Alpine Fault () is generally taken as evidence that Neogene vertical exhumation of at least 12–16 km (Kamp et al. Citation1992) and up to 25 km (Grapes & Watanabe Citation1992) has exerted a strong control on the isograd geometry. Can a similar explanation be invoked to explain the overall subparallelism of Greenland Group isograds to the Alpine Fault?
Kamp et al. (Citation1992) interpreted apatite fission track profiles to indicate c. 4–6 km of differential uplift of the Greenland Group since 5 Ma, perpendicular to the Alpine Fault, with greater exhumation closer to the Fault (). Seward and Nathan (Citation1990) had similar differential uplift of >3 km close to the Alpine Fault and <3 km away from it. A simple calculation shows that 2 km of recent differential uplift along a 12 km baseline results in a Late Neogene tilt of only c. 10° if the South Westland Greenland Group is treated as a solid block. We conclude that Plio-Pleistocene block-tilting appears insufficient to drastically control the map pattern of the Greenland Group isograds on the northwest side of the Alpine Fault, despite the parallelism of these features.
Cretaceous and older tectonic events
In the Cretaceous Paparoa Metamorphic Core Complex (P in ) greenschist and amphibolite facies Greenland Group rocks are juxtaposed with pronounced metamorphic break across low-angle detachment faults (Tulloch Citation1995). In the area of , Tulloch (Citation1995) advocated a ‘Haast Core’ to a hypothesised Cretaceous metamorphic core complex, with putative NW–SE-striking detachment faults near the Arawhata River and Waitā River. This was proposed principally on the basis that the rocks in the intervening region were of higher grade and contrasted with lower-grade rocks to the NE and SW, and also on the presence of nearby Otumotu Formation ‘upper plate’ sedimentary breccias at Bald Hill.
Our work, along with that of Randall (Citation2004), has confirmed that much of the coastal strip near Haast is underlain by sillimanite–microcline zone rocks (). However, no NW–SE-trending faults or discontinuities (as proposed by Tulloch Citation1995, ) have subsequently been discovered and only one small E–W-striking shear zone is reported from Mussel Point (Randall Citation2004). Furthermore, the Arawhata River–Waitā River area appears to represent only a slight widening of the sillimanite–microcline zone within the overall regional NE–SW strike of isograds (). In the upper Waitā River (), Ryland (Citation2011) also postulated a metamorphic core-complex-style detachment within our sillimanite–microcline zone. Gneissic Greenland Group (Cattle Track Gneiss OU81541) constituted the proposed lower plate and the upper plate was biotite-grade Greenland Group and Maori Saddle Granite (). While plausible, the putative detachment fault rock was seen only in three poorly exposed places and our identification of sillimanite–microcline zone rocks (P49812, 49814) in the proposed upper plate suggests that the metamorphic break is not as great as originally advocated. Given the close proximity of the Alpine Fault (within 500 m), the mylonites may instead relate to Alpine Fault movement.
Evidence for major pre-Cenozoic exhumation of the South Westland Greenland Group metamorphic basement is provided by the Otumotu Formation unconformity and by Kamp et al.'s (1992) pre-Cenozoic zircon fission track data that they interpret to indicate 4–10 km of uplift. Current evidence does not require Cretaceous metamorphic core complex geometries to explain the metamorphic zone pattern in South Westland. This does not preclude their eventual discovery but more structural and thermo-chronological evidence must be forthcoming. The 300 Ma spread of medium closure temperature (K–Ar and zircon fission track) ages in suggests to us that the presently exposed surface outcrops were episodically exhumed from 4–15 km crustal depths and/or reheated during mid-Palaeozoic and/or Cretaceous tectonic events.
Regional metamorphism in New Zealand
Landis & Coombs (Citation1967) described the New Zealand basement as a paired metamorphic belt. Since then, there has been a plethora of new work on New Zealand metamorphic rocks including development of terrane and batholith concepts for understanding regional geology, radiometric dating to clarify the ages of metamorphism and also the discovery of granulites and eclogites in Fiordland (Oliver Citation1980; De Paoli et al. Citation2009). Jadeite-bearing blueschist facies rocks have still not been found in New Zealand, lawsonite being the highest pressure index mineral from the Eastern Province. The current pressure-temperature-age summary of New Zealand metamorphic rocks, including the present work, is shown in . This confirms that the paired metamorphic belt concept no longer holds, especially when comparing metamorphic rocks of similar ages. The Jurassic–Cretaceous metamorphic rocks in the Eastern Province formed in accretionary wedge and forearc and preserve moderately high P/T field geothermal gradients. Co-eval metamorphic rocks in the Western Province lie within the flanks and roots of the Median Batholith magmatic arc. As expected, these preserve low and medium P/T events, but New Zealand's highest-pressure rocks (of eclogite and granulite facies) also come from here. The Palaeozoic metamorphic rocks, including the Greenland Group of South Westland, are mainly low P/T but some medium P/T sequences are preserved in western Fiordland (). This low P/T ‘Buchan’ style of regional metamorphism is atypical of that found in accretionary wedges (and the Greenland Group has never been suggested to have accreted in a convergent margin wedge). Syn-orogenic rift type settings have been suggested to explain Buchan-style geothermal gradients (e.g. Viete et al. Citation2010).
Figure 8 Summary of preserved metamorphic field gradients in different parts of New Zealand. Fields from 1Grapes & Watanabe (Citation1992), 2White (Citation1994), 3Ireland & Gibson (Citation1998), 4Mortimer (Citation2000), 5De Paoli et al. (Citation2009), 6Scott et al. (Citation2009a), 7Scott et al. (Citation2009b), 8Scott et al. (Citation2011), 9this study, 10Cooper (Citation1980), 11Vry et al. (Citation2008) and unpublished data. Background metamorphic facies grid after Yardley (Citation1989). k, kyanite; s, sillimanite; a, andalusite stability fields; PP-Pact, Prehnite-pumpellyite and pumpellyite-actinolite facies; Fe, Eastern and Central Fiordland; Fw, Western Fiordland; P, Paparoa Range; B, Bonar Range.
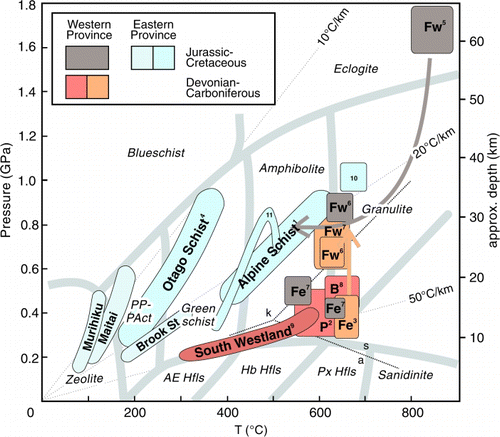
Implications for mineral exploration
Orogenic (mesothermal) gold deposits in New Zealand and elsewhere are typically hosted in lower greenschist facies host rocks (Bierlein & Crowe Citation2000; Christie & Brathwaite Citation2003; Phillips & Powell Citation2010). will thus be useful to mineral exploration programmes that use metamorphic grade as a guide. The area of onland basement between the Alpine Fault and South Westland Fault Zone (including Greenland Group under sedimentary cover) comprises only c. 26% chlorite-zone greenschist facies rocks and c. 37% each of biotite-zone greenschist facies and amphibolite facies rocks. This is not to say that there is no chance of mineralisation in higher-grade rocks: Ryland et al. (Citation2008) used the Ti-in-quartz geothermometer to study hydrothermal quartz veins in the biotite–calcic plagioclase zone in the Whakapohai River. They reported minimum quartz vein crystallisation temperatures of 325–440 °C with temperatures decreasing in the same down-grade direction as our metamorphic zones. The seemingly protracted exhumation history of the Greenland Group metamorphic sequence () would allow ample opportunity for dewatering and fracturing events since the Carboniferous. The calcic Greenland Group protoliths and small granite stocks also offer the possibility of skarn mineralisation.
Conclusions
Previously unpublished field and mineral chemistry data from the Greenland Group has been used to define provisional metamorphic mineral zones and isograds in South Westland between Big Bay and Fox Glacier. Four metamorphic zones have been established: chlorite, biotite–albite, biotite–calcic plagioclase and sillimanite–microcline. These zones are designed to be used with the major metasedimentary rock types of the Greenland Group, including variably calcareous psammites. The local presence of andalusite and cordierite, a single generation foliation, and static mineral overgrowth textures indicate a progressive low P/T facies series and a Buchan style of regional metamorphism. Metamorphic sequences in the Greenland Group block between the South Westland Fault Zone and the Alpine Fault appear continuous. Available geochronological data suggest a Devonian–Carboniferous (330–375 Ma) age for peak metamorphism with a protracted cooling and exhumation history through at least the Cretaceous. Although Greenland Group isograds in South Westland are subparallel to the Alpine Fault, Plio-Pleistocene exhumation of the Greenland Group has been relatively minor and, unlike with the Alpine Schist, has not necessarily exerted a strong control on the geometry of the metamorphic zones.
Despite the above advances, we realise that the results of this study in turn raise a number of issues that we have not addressed. These include relationship of macro, meso- and micro-structures to metamorphism, precise timing of Devonian–Carboniferous recrystallisation, whether any Cretaceous recrystallisation and/or deformation can be demonstrated in the area, detailed thermobarometry and whether partial melting occurred in the highest-grade rocks. A comparison with other gneissic units in Westland such as those in the Fraser Complex and the Bonar Range (Cox & Barrell Citation2007) should also be made. The results of the present study provide a useful regional framework for such future, more targeted, investigations.
Acknowledgements
The area between Paringa and Haast was geologically mapped in 1984–1986 in a project funded by the New Zealand Forest Service. Additional data have come from a number of unpublished sources including Otago University theses and GNS Science's QMAP project. We are grateful to Nic Barth for information on the Monkey Puzzle Gorge. We thank Neville Orr, Belinda Smith Lyttle, Delia Strong and Andreas Auer for technical assistance. We have had useful discussions with Andy Tulloch and Rose Turnbull. An earlier version of the manuscript was improved by comments from Mark Rattenbury and we also appreciate the careful reviews by James Scott and Harold Stowell. This research was supported by New Zealand Ministry of Business, Innovation and Employment Direct Core Funding to GNS Science.
References
- Adams , CJ . 2004 . Rb-Sr age and strontium isotope characteristics of the Greenland Group, Buller Terrane, New Zealand, and correlations at the East Gondwanaland margin . New Zealand Journal of Geology and Geophysics , 47 : 189 – 200 . doi: 10.1080/00288306.2004.9515047
- Adams , CJ and Kelley , S . 1998 . Provenance of Permian-Triassic and Ordovician metagraywacke terranes in New Zealand: evidence from 40Ar/39Ar dating of detrital micas . Geological Society of America Bulletin , 110 : 422 – 432 . doi: 10.1130/0016-7606(1998)110%3C0422:POPTAO%3E2.3.CO;2
- Adams , CJ , Harper , CT and Laird , MG . 1975 . K–Ar ages of low grade metasediments of the Greenland and Waiuta groups in Westland and Buller, New Zealand . New Zealand Journal of Geology and Geophysics , 18 : 39 – 48 . doi: 10.1080/00288306.1975.10426345
- Aronson , JL . 1968 . Regional geochronology of New Zealand . Geochimica et Cosmochimica Acta , 32 : 669 – 697 . doi: 10.1016/0016-7037(68)90008-2
- Bartlett BB 1993 . Geology of Maori Saddle, Cattle Track, South Westland . Unpublished BSc (Hons) thesis . Dunedin , University of Otago .
- Bierlein , FP and Crowe , DE . 2000 . “ Phanerozoic orogenic lode gold deposits ” . In Gold in 2000. Society of Economic Geologists Reviews of Economic Geology Edited by: Hagemann , SG and Brown , PE . Vol. 13 , 103 – 139 .
- Christie , AB and Brathwaite , RL . 2003 . Hydrothermal alteration in metasedimentary rock-hosted orogenic gold deposits, Reefton goldfield, South Island, New Zealand . Mineralium Deposita , 38 : 87 – 107 . doi: 10.1007/s00126-002-0280-9
- Cooper , AF . 1972 . Progressive metamorphism of metabasic rocks from the Haast Schist Group of southern New Zealand . Journal of Petrology , 13 : 457 – 492 . doi: 10.1093/petrology/13.3.457
- Cooper , AF . 1980 . Retrograde alteration of chromian kyanite in metachert and amphibolite whiteschist from the Southern Alps, New Zealand, with implications for uplift on the Alpine Fault . Contributions to Mineralogy and Petrology , 75 : 153 – 164 . doi: 10.1007/BF00389775
- Cox SC , Barrell DJA compilers 2007 . Geology of the Aoraki area . Institute of Geological & Nuclear Sciences 1:250 000 Geological Map 15 . GNS Science , Lower Hutt , , New Zealand .
- De Paoli , MC , Clarke , GL , Klepeis , KA , Allibone , AH and Turnbull , IM . 2009 . The eclogite–granulite transition: mafic and intermediate assemblages at Breaksea Sound, New Zealand . Journal of Petrology , 50 : 2307 – 2343 . doi: 10.1093/petrology/egp078
- Ferry , JM and Spear , FS . 1978 . Experimental calibration of the partitioning of Fe and Mg between biotite and garnet . Contributions to Mineralogy and Petrology , 66 : 113 – 117 . doi: 10.1007/BF00372150
- Ghent , ED and Stout , MZ . 1981 . Geobarometry and geothermometry of plagioclase-biotite-garnet-muscovite assemblages . Contributions to Mineralogy and Petrology , 76 : 92 – 97 . doi: 10.1007/BF00373688
- Gibson , GM . 1992 . Medium–high pressure metamorphic rocks of the Tuhua orogen, western New Zealand as lower crustal analogues of the Lachlan Fold Belt, SE Australia . Tectonophysics , 214 : 145 – 157 . doi: 10.1016/0040-1951(92)90194-B
- Grapes , R and Otsuki , M. 1983 . Peristerite compositions in quartzofeldspathic schists, Franz Josef-Fox Glacier area, New Zealand . Journal of Metamorphic Geology , 1 : 47 – 61 . doi: 10.1111/j.1525-1314.1983.tb00264.x
- Grapes , R and Watanabe , T . 1992 . Metamorphism and uplift of Alpine schist in the Franz Josef-Fox Glacier area of the Southern Alps, New Zealand . Journal of Metamorphic Geology , 10 : 171 – 180 . doi: 10.1111/j.1525-1314.1992.tb00077.x
- Hiess , J , Ireland , TR and Rattenbury , MS . 2010 . U-Th-Pb zircon and monazite geochronology of Western Province gneissic rocks, central-south Westland, New Zealand . New Zealand Journal of Geology and Geophysics , 53 : 241 – 269 . doi: 10.1080/00288306.2010.495450
- Hoisch , TD . 1990 . Empirical calibration of six geobarometers for the mineral assemblage quartz+muscovite+biotite+plagioclase+garnet . Contributions to Mineralogy and Petrology , 104 : 225 – 234 . doi: 10.1007/BF00306445
- Ireland , TR and Gibson , GM . 1998 . SHRIMP monazite and zircon geochronology of high grade metamorphism in New Zealand . Journal of Metamorphic Geology , 16 : 149 – 167 . doi: 10.1111/j.1525-1314.1998.00112.x
- Kamp , PJJ , Green , PF and Tippett , JM . 1992 . Tectonic architecture of the mountain front-foreland basin transition, South Island, New Zealand, assessed by fission track analysis . Tectonics , 11 : 98 – 113 . doi: 10.1029/91TC02362
- Kleeman , U and Reinhardt , J . 1994 . Garnet-biotite thermometry revisited: the effect of AlVI and Ti in biotite . European Journal of Mineralogy , 6 : 925 – 941 .
- Laird , MG . 1972 . Sedimentology of the Greenland Group in the Paparoa Range, West Coast, South Island . New Zealand Journal of Geology and Geophysics , 15 : 372 – 393 . doi: 10.1080/00288306.1972.10422338
- Laird , MG . 1986 . The nature of the Martins Bay Formation, South Westland . Geological Society of New Zealand Miscellaneous Publication , 34 : 26 – 28 .
- Landis , CA and Coombs , DS . 1967 . Metamorphic belts and orogenesis in southern New Zealand . Tectonophysics , 4 : 501 – 518 . doi: 10.1016/0040-1951(67)90014-5
- Mortimer , N . 2000 . Metamorphic discontinuities in orogenic belts: example of the garnet-biotite-albite zone in the Otago Schist, New Zealand . International Journal of Earth Sciences , 89 : 295 – 306 . doi: 10.1007/s005310000086
- Mutch AR 1964 . Geological Map of New Zealand 1:63 360 . Sheet S105 Martins Bay . Wellington , Department of Scientific and Industrial Research .
- Nathan S 1978a . Geological Map of New Zealand 1:63 360 . Sheet S44 Greymouth . Wellington , Department of Scientific and Industrial Research .
- Nathan , S 1978b . Geological Map of New Zealand 1:63 360. Sheet S31 and part . S32 Buller-Lyell. 1st ed . Wellington , Department of Scientific and Industrial Research .
- Nathan , S 1996 . Geology of the Buller Coalfield. Scale 1:50 000. Institute of Geological & Nuclear Sciences Geological Map 23 . Institute of Geological & Nuclear Sciences Lower Hutt .
- Nathan S , Stewart M , Kawachi Y 1987 . Regional metamorphism of basement rocks west of the Alpine Fault in South Westland . Geological Society of New Zealand Miscellaneous Publication 37A (unpaginated) .
- Nathan S , Thurlow C , Warnes P , Zucchetto R 2000 . Geochronology database for New Zealand rocks (2nd edition): 1961–1999 . Lower Hutt , Institute of Geological & Nuclear Sciences . Institute of Geological & Nuclear Sciences Science Report 2000/11 . 51 p.
- Oliver , GJH . 1980 . Geology of the granulite and amphibolite facies gneisses of Doubtful Sound, Fiordland, New Zealand . New Zealand Journal of Geology and Geophysics , 23 : 27 – 41 . doi: 10.1080/00288306.1980.10424190
- Phillips , GN and Powell , R . 2010 . Formation of gold deposits: a metamorphic devolatilization model . Journal of Metamorphic Geology , 28 : 689 – 710 . doi: 10.1111/j.1525-1314.2010.00887.x
- Randall S 2004 . Western Province intrusive rocks and Greenland Group greywacke near Haast, south Westland . BSc (Hons) thesis . Dunedin , University of Otago .
- Ransley JF 1983 . The geology of the Jackson River catchment, southern Westland . BSc (Hons) thesis . Dunedin , University of Otago .
- Rattenbury MS , Jongens R , Cox SC compilers 2010 . Geology of the Haast area . Institute of Geological & Nuclear Sciences 1:250 000 Geological Map 14 . GNS Science , Lower Hutt .
- Roser , BP , Cooper , RA , Nathan , S and Tulloch , AJ . 1996 . Reconnaissance sandstone geochemistry, provenance, and tectonic setting of the lower Paleozoic terranes of the West Coast and Nelson, New Zealand . New Zealand Journal of Geology and Geophysics , 39 : 1 – 16 . doi: 10.1080/00288306.1996.9514690
- Ryland CB 2011 . The geology and structural evolution of the Bald Hill-Maori Saddle region, South Westland, New Zealand . Unpublished MSc thesis. Dunedin University of Otago .
- Ryland , CB , Palin , JM and Cooper , AF . 2008 . Ti-in-quartz geothermometry: a new tool for minerals exploration . Australasian Institute of Mining and Metallurgy, New Zealand Branch, Annual Conference Proceedings , 41 : 497 – 508 .
- Scott JM , Cooper AF , Palin JM , Tulloch AJ , Kula J , Jongens R , Spell TL , Pearson NJ 2009a . Tracking the influence of a continental margin on growth of a magmatic arc, Fiordland, New Zealand, using thermobarometry, thermochronology, and zircon U-Pb and Hf isotopes . Tectonics 28 : TC6007 , doi: 10.1029/2009TC002489 .
- Scott , JM , Palin , JM , Cooper , AF , Fagereng , A and King , RP . 2009b . Polymetamorphism, zircon growth and retention of early assemblages through the dynamic evolution of a continental arc in Fiordland, New Zealand . Journal of Metamorphic Geology , 27 : 281 – 294 . doi: 10.1111/j.1525-1314.2009.00817.x
- Scott , J , Muhling , J , Fletcher , I , Billia , M , Palin , JM , Elliot , T and Gűnter , C . 2011 . The relationship of Paleozoic metamorphism and S-type magmatism on the paleo-Pacific Gondwana margin . Lithos , 127 : 522 – 534 . doi: 10.1016/j.lithos.2011.09.008
- Seward , D and Nathan , S . 1990 . Uplift history of South Westland using the fission-track dating technique . New Zealand Journal of Geology and Geophysics , 33 : 201 – 204 . doi: 10.1080/00288306.1990.10425678
- Tulloch AJ 1995 . Precious metal mineralisation associated with the Cretaceous Paparoa metamorphic core complex, New Zealand . In : Mauk JL , St George JD PACRIM Congress 1995, Auckland, New Zealand . Australasian Institute of Mining and Metallurgy 95/9 : 575 – 580 .
- Tulloch , AJ and Challis , GA . 2000 . Emplacement depths of Paleozoic-Mesozoic plutons from western New Zealand estimated by hornblende-Al geobarometry . New Zealand Journal of Geology and Geophysics , 43 : 555 – 567 . doi: 10.1080/00288306.2000.9514908
- Tulloch , AJ , Ramezani , J , Kimbrough , DL , Faure , K and Allibone , AH . 2009 . U-Pb geochronology of mid-Paleozoic plutonism in western New Zealand: implications for S-type granite generation and growth of the east Gondwana margin . Geological Society of America Bulletin , 121 : 1236 – 1261 . doi: 10.1130/B26272.1
- Turnbull IM compiler 2000 . Geology of the Wakatipu area . Institute of Geological & Nuclear Sciences 1:250 000 Geological Map 18 . GNS Science , Lower Hutt , , New Zealand .
- Turnbull , IM , Mortimer , N and Craw , D . 2001 . Textural zones in the Haast Schist – a reappraisal . New Zealand Journal of Geology and Geophysics , 44 : 171 – 183 . doi: 10.1080/00288306.2001.9514933
- Turner , FJ . 1930 . The metamorphic and ultrabasic rocks of the lower Cascade valley, south Westland . Transactions and Proceedings of the New Zealand Institute , 61 : 170 – 201 .
- Turner , FJ . 1933 . The metamorphic and intrusive rocks of southern Westland . Transactions of the New Zealand Institute , 63 : 178 – 284 .
- Turner FJ 1981 . Metamorphic petrology: mineralogical, field and tectonic aspects , 2nd edition . McGraw-Hill , New York . 524 p.
- Viete DR , Richards SW , Lister GS , Oliver GHJ , Banks GJ . 2010 . Lithospheric-scale extension during Grampian orogenesis in Scotland . In : Law RD , Butler RWH , Holdsworth RE , Krabbendam M , Strachan RA . Continental tectonics and mountain building: the legacy of Peach and Horne . London , Geological Society of London, Special Publication 335 : 121 – 160
- Vry , JK , Powell , R and Williams , J . 2008 . Establishing the P-T path for Alpine Schist, Southern Alps near Hokitika, New Zealand . Journal of Metamorphic Geology , 26 : 81 – 97 .
- Wellman HW 1955 . Geology of Bruce Bay-Haast River . New Zealand Geological Survey Bulletin 48 .
- White , PJ . 1994 . Thermobarometry of the Charleston Metamorphic group and implications for the evolution of the Paparoa Metamorphic Core Complex, New Zealand . New Zealand Journal of Geology and Geophysics , 37 : 201 – 209 . doi: 10.1080/00288306.1994.9514615
- Yardley BWD 1989 . An introduction to metamorphic petrology . Harlow , UK , Pearson Education Ltd . 248 p.