Abstract
Gold-bearing normal faults of inferred Late Cretaceous age cut both Caples Terrane and Torlesse Terrane schists on the Old Man Range in Central Otago. Mineralised fault rocks are dominated by brittle-formed breccias and fault gouges, with minor hydrothermal silicification and quartz vein formation, and scattered pyrite and arsenopyrite. Gold occurs replacing silicates, encapsulated in sulphide minerals, and as free particles in vein quartz. The orientations of mineralised faults were controlled by pre-existing joints in the host rocks, with west strike in Torlesse schists and northwest strike in Caples schists. A major component of placer gold on the slopes of the Old Man Range has been derived from nearby mineralised faults, and detrital gold particles are equant and angular rather than flaky. Gold in a Late Pleistocene Clutha River channel is flaky and has been transported from outside the area, but there is little or no evidence of externally derived detrital gold along the course of a Middle Pleistocene Clutha River channel.
Introduction
The Old Man Range is one of the most prominent topographic features in Central Otago and coincides with the boundary between the two main protolith rock suites of the Otago Schist: the Caples and Torlesse terranes (, A; Mortimer Citation1993; Turnbull Citation2000). Uplift of the range has been occurring since at least the Pliocene, and some of the uplifting structures are still active (Turnbull Citation2000). This uplift and associated erosion has exposed structurally hosted orogenic gold deposits in the basement rocks of the Old Man Range. Uplift of the Old Man Range has exposed Torlesse Terrane schists in an erosional window through the structurally overlying Caples Terrane schists (, A). The orogenic gold deposits have similar styles of mineralisation in both Caples and Torlesse Terrane schists on the Old Man Range. This is one of the few places in Otago in which similar orogenic gold deposits can be observed in these two different host rocks in close proximity.
Figure 1 Geological location map of the Old Man Range and its protolith basement terranes (modified after Mortimer Citation1993; Turnbull Citation2000; MacKenzie & Craw Citation2005). Principal orogenic gold deposits, including the Hyde-Macraes Shear Zone (HMSZ), are also shown.
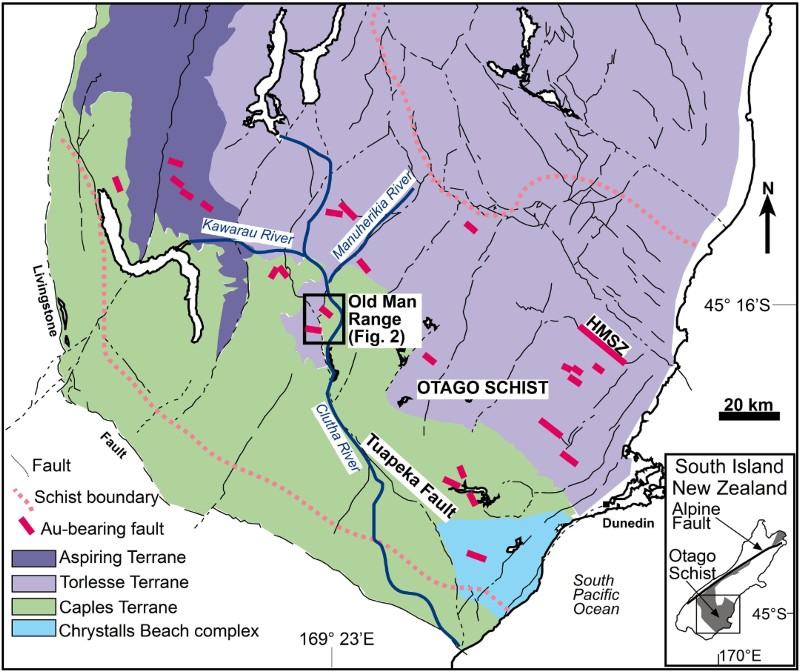
Gold eroded from these deposits has been shed into nearby streams, locally making placer deposits that have been mined historically. In addition, the rise of the Old Man Range has affected the course of the Clutha River (, A), which is the largest river draining the Otago area and which hosts important placer gold deposits that have been mined sporadically since the 1860s (Williams Citation1974; Youngson & Craw Citation1999; Craw et al. Citation2012; Craw Citation2013). Ancestral courses of the Clutha River can be identified near the base of the Old Man Range, and two of these are outlined in this article where they formed axial channels that interacted with evolving range structures.
This article provides new information on the structure and petrography of previously undescribed orogenic gold deposits on the Old Man Range on the western margin of the Otago Schist belt (), and on the nature of eroded detrital gold as it was transported and deposited in associated fluvial systems. We describe the structural and geochemical characteristics of the orogenic gold deposits, and identify key contrasts in structural controls of these gold deposits in the different host schist terranes. We then describe the sediments in the axial river channels and the debris shed towards those channels from the rising Old Man Range, in order to contrast the nature and morphology of detrital gold in placers in these different sedimentary environments associated with dynamic landscape evolution.
General setting
The Otago Schist belt of southern New Zealand consists of several metasedimentary terranes that were amalgamated during the Jurassic (; Mortimer Citation1993; MacKenzie & Craw Citation2005). The principal terranes of interest in this study, Caples and Torlesse terranes, are dominated by variably metamorphosed greywacke and argillite with subordinate metabasite horizons (Mortimer Citation1993; Turnbull Citation2000). The northeast and southwest margins of the schist belt have low-grade (subgreenschist facies) rocks that increase in metamorphic grade towards the core of the belt (Mortimer Citation1993; Turnbull Citation2000). In the core of the schist belt in the general vicinity of the Old Man Range (), the Caples Terrane rocks have been metamorphosed to lower greenschist facies and generally have planar foliation and foliation-parallel metamorphic segregation veins. The structurally underlying Torlesse Terrane rocks are upper greenschist facies schists with well-defined foliation and metamorphic segregation that has been variably disrupted by late-metamorphic folding. Numerous metabasite horizons (greenschist) occur within the Torlesse Terrane on the Old Man Range, but are absent from the Caples Terrane in that area (Turnbull Citation2000; Bierlein & Craw Citation2009). Metabasites and associated metacherts are more abundant in the Aspiring Terrane to the northwest of the Old Man Range (; Bierlein & Craw Citation2009).
Regional extensional deformation of the Otago Schist belt occurred in the middle–Late Cretaceous, during exhumation of the higher grade schists from metamorphic depths (Deckert et al. Citation2002; Gray & Foster Citation2004; Barker et al. Citation2010; Mortensen et al. Citation2010). This deformation was focused on a network of northwest- and northeast-striking normal faults that are still prominent across the schist belt, although many of them have been subsequently reactivated (; Turnbull Citation2000; Deckert et al. Citation2002; Gray & Foster Citation2004; Barker et al. Citation2010; Craw Citation2010). Further extension, erosion and marine planation in the Cenozoic led to a regional low-relief surface, the Waipounamu Erosion Surface, over the schist belt (Landis et al. Citation2008). Differential erosion and fault juxtaposition during this Cretaceous–middle Cenozoic period has controlled most of the variations in metamorphic grade exposed at the surface in the Otago Schist belt ().
Compressional deformation of schist basement and the Waipounamu Erosion Surface began in the late Oligocene–early Miocene during initiation of the Alpine Fault plate boundary through the South Island, and continued to the present (; Jackson et al. Citation1996; Turnbull Citation2000; Craw et al. Citation2012). This deformation involved reactivation of pre-existing normal faults, and development of faulted folds of schist foliation with wavelength scales of 2–20 km (Turnbull et al. Citation1993; Jackson et al. Citation1996; Turnbull Citation2000; Craw et al. Citation2012). Miocene sediments arising from the initiation of compressional uplift in Central Otago were quartz-rich fluvial and lacustrine deposits, and quartz pebble conglomerates in this sequence commonly contain abundant placer gold (Williams Citation1974; Douglas Citation1986; Youngson & Craw Citation1993, Citation1995; Youngson et al. Citation2006; Craw Citation2013). Remnants of these sediments are common features on the margins of rising mountain ranges including the Old Man Range, and the sediments are generally immediately underlain by variably oxidised and clay-altered basement schist (A; Stirling Citation1990; Craw Citation1994). The clay alteration occurred beneath the Miocene sediments, and is typically pervasive immediately below the unconformity and decreases in intensity over c. 10–50 m (Craw Citation1994; Craw et al. Citation2015).
Central Otago has an arid to semi-arid climate that initially developed in a rain shadow in the Pliocene (Chamberlain et al. Citation1999). The higher altitude (>1200 m) parts of the mountain ranges, including the Old Man Range, also developed periglacial conditions during the Pleistocene (Stirling Citation1990; Turnbull Citation2000). Periglacial processes, combined with steepening of slopes during uplift, have led to widespread landslide formation on the upper slopes of the Old Man Range, especially in the more friable Torlesse Terrane schists (A; Turnbull Citation2000). Associated colluvial deposits and alluvial fans, including debris-flow deposits, have developed on the lower slopes and margins of all actively rising ridges (Youngson & Craw Citation1993; Turnbull Citation2000).
Methods
Field work for this study was conducted in and around historic placer and orogenic gold mines. The principal orogenic gold mines in mineralised faults that were examined are located on A. Samples were collected from old mine excavations and adjacent host rocks, although many of the mine sites had little mineralised rock left in outcrop. Additional mineralised samples were collected from nearby mine waste piles in order to examine more mineralised material than is available in outcrops. The samples were examined by standard transmitted and incident light petrographic techniques, and with a Zeiss Sigma VP FEG scanning electron microscope (SEM) with energy dispersion analytical attachment (EDS; University of Otago Centre for Electron Microscopy). Detrital gold was extracted and concentrated from sediments with standard gold panning techniques. The shapes and textures of resultant gold particles were examined by SEM.
A set of the most mineralised rocks from historic mines (21 samples) was selected on the basis of evidence of hydrothermal sulphides and/or silicification. These samples were analysed for gold by SGS New Zealand using fire assay with a flame atomic absorption finish. Arsenic contents were determined by XRF on pressed powder discs from the same powdered samples. In addition, five samples of sheared rock from near the unmined Buds Reef (MacKenzie & Craw Citation2004) were analysed for As and Sb via ICP-OES after acid digestion by Hill Laboratories, Hamilton, New Zealand.
Old Man Range structure
Basement structure
The Torlesse Terrane schist basement that makes up the higher parts of the Old Man Range was juxtaposed against Caples Terrane schists along the Old Man Fault (A). This fault is a lateral continuation of a regional-scale Cretaceous extensional structure, the Tuapeka Fault, which is traceable from the east coast of the South Island (; Bishop & Turnbull Citation1996; Turnbull Citation2000; Els et al. Citation2003). The higher metamorphic grade Torlesse Terrane schists have been uplifted relative to the structurally overlying Caples Terrane schists. Consequently, the Torlesse rocks at the surface on the Old Man Range are now juxtaposed to the west of some Caples rocks across the Old Man Fault, constituting an anomaly in the overall Otago Schist structure (, A, A). Caples Terrane schists also occur structurally above Torlesse Terrane schists on the western slopes of the Old Man Range (). Juxtaposed rocks on the western slopes were truncated by a Cenozoic unconformity surface. There have been several kilometres of net displacement across the Old Man Fault, based on the observed offset of the terrane boundary (Turnbull Citation2000). The Cenozoic unconformity has been shortened by only 2% by late Cenozoic movement, most of which was accommodated by folding (A; Stirling Citation1990), implying only minor Cenozoic fault displacement and predominant Cretaceous displacement.
Torlesse Terrane schist foliation on the Old Man Range generally dips shallowly although the dip becomes steeper in places, especially near the Old Man Fault (B). Prominent quartz rodding lineation on the schist foliation surfaces trend generally northwards (B). The foliation is deformed on the outcrop scale by several generations of folds from ductile similar folds to brittle kinks, with variable development of fold axial surface cleavages. The most strongly folded Torlesse schists occur within 1 km of the Old Man Fault, where ductile folds and kinks generally trend northwards. Torlesse schist outcrops are cut by numerous steeply to moderately dipping joints, and these strike mainly westwards (C). The deformed foliation is particularly susceptible to landslides on the slopes of the Old Man Range (, A; Turnbull Citation2000). Observations and data for this study were obtained primarily on in situ spurs where historic miners found exposures of mineralised rocks, rather than in the hummocky landsliding slopes of valleys. Nevertheless, most of the major streams have incised through the landslides and the underlying basement structure, especially the joints, still partially controls stream orientations (A).
Figure 3 Structural contrasts between Torlesse and Caples Terrane schist basement. A, Aerial photograph (from Otago Regional Council) of a representative transect of Old Man Range topography (dashed box in ), showing stream control by joints in schist: west strike in Torlesse Terrane and northwest strike in Caples Terrane. B–E, Stereonets (lower hemisphere) showing orientations of foliations, lineations, joints and mineralised faults in Torlesse and Caples Terrane.
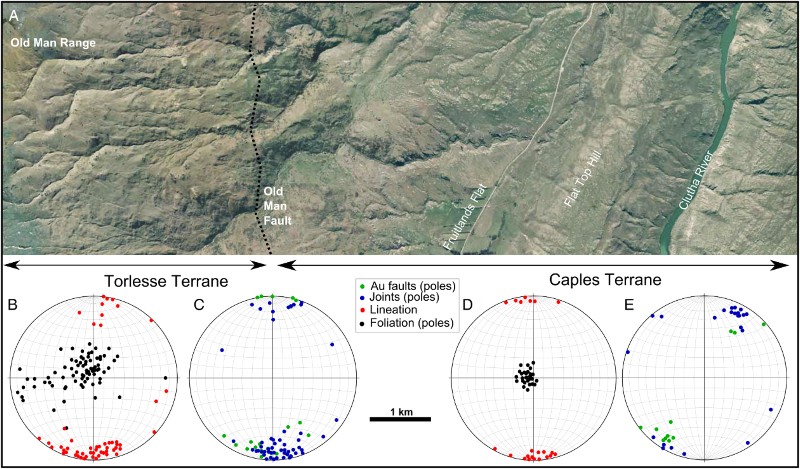
The foliation in the Caples Terrane schists is generally flat-lying or shallow-dipping, and is broadly consistent over the area surrounding the orogenic gold deposits (D). Likewise, the quartz rodding lineation trends northwards, similar to the Torlesse lineations (D). Joints in the Caples schists strike northwest (E), distinctly different from the joints in the Torlesse schists. The Caples schists are less fissile than the Torlesse schists, and crop out on the lower slopes of the Old Man Range (, A). Consequently, the Caples schists are less affected by landslides; the basement structure, especially joints, is readily discernible in surface topography (, A).
Late Cenozoic structure
The Old Man Range is a complex faulted fold of foliation, Cenozoic unconformity and Miocene sediments (A). The highest parts of the range were formed by asymmetric antiformal uplift of the Cenozoic unconformity and underlying Torlesse Terrane basement, with accompanying erosion of most of the overlying Miocene sediments (A). The landform surface reflects this asymmetric structure, with smooth topographic remnants of the Cenozoic unconformity preserved on the top and some spurs on the steeper eastern flank of the range (A; Stirling Citation1990; Jackson et al. Citation1996). Reactivation of the Old Man Fault during this deformation has contributed to this uplift, causing steepening of the eastern slopes of the range and encouraging locally deep erosional incision (, A).
Remnants of the Cenozoic unconfomity also form smooth topographic surfaces at the eastern base of the Old Man Range in the Conroys area at Fruitlands Flat and on Flat Top Hill (A). The Cenozoic unconformity has been disrupted in these areas by minor folding and associated faults. The faults have vertical offsets on the order of < 200 m, forming minor fault scarps (A). Vertical offset on the fault between Fruitlands Flat and Flat Top Hill dies out to the northeast into a shallow antiform extending to Alexandra (A). Late Cenozoic erosion into the topographically smooth schist unconformity has been locally controlled by pre-existing joints in the underlying schist, so that small streams commonly have northwest or southeast trends on Caples Terrane basement and mainly eastward trends on the Torlesse Terrane basement (A, C, E).
Orogenic gold deposits
Mineralised faults in Torlesse Terrane
A set of mineralised faults cuts the Torlesse Terrane schists on the upper slopes of the Old Man Range (A). These mineralised faults, called ‘reefs’ by early miners and in historical records, were apparently small but locally rich (> 21–73 g Au/tonne) resources, as summarised by MacKenzie & Craw (Citation2004). The faults strike west and mostly dip steeply to the north or south (C), and the Alpine Reef (A) dips moderately (c. 50°) to the north (A). The mineralised faults are generally parallel or subparallel to joints in the immediate host schist (C), although joint orientations can be variable on a larger scale. The general parallelism of joints and mineralised faults is still apparent at the moderately dipping Alpine Reef, where joints dip more gently than elsewhere (A, B), slickenlines on polished surfaces of fault planes indicate dip-slip motion and deflection of foliation in hanging walls and foot walls of the structures imply a normal sense of movement.
Figure 4 Field photographs of gold-bearing faults and associated structures. A, Outcrop of Alpine Reef. B, Jointed Torlesse Terrane schist near Alpine Reef. C, Silicified breccia with arsenopyrite in Alpine Reef. D, Fault breccia without silicification in mineralised fault in Caples Terrane schist c. 1 km southeast of Conroys Reef (A).
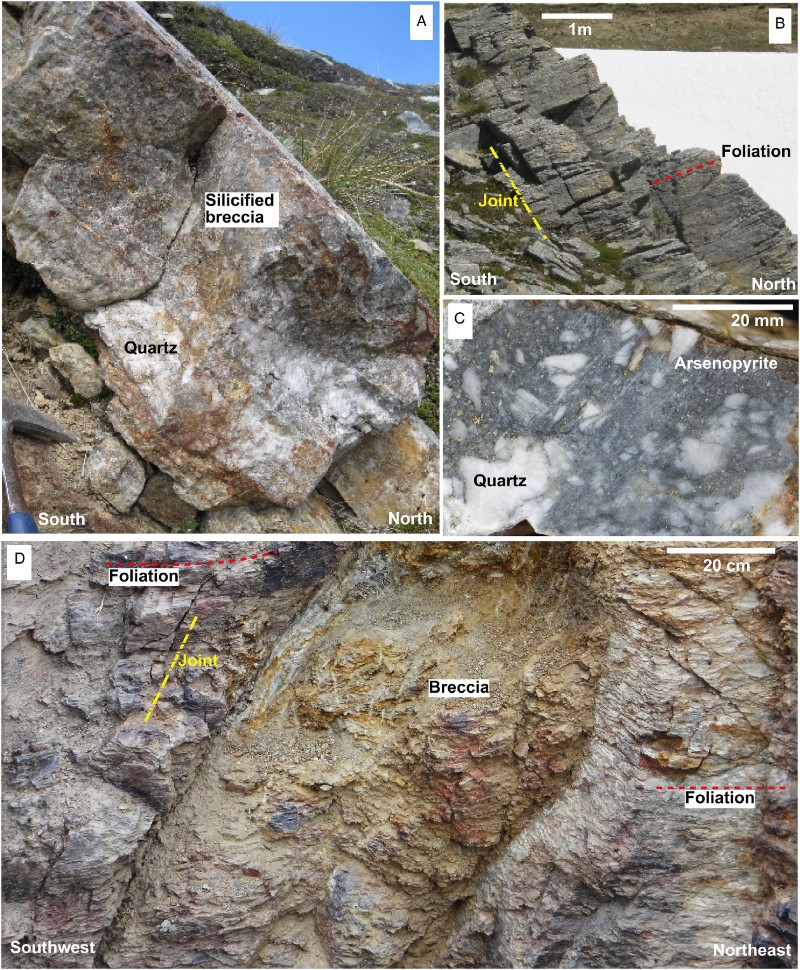
Whites and Grey's mineralised structures (A) have been mined in 5–10 m wide surface trenches by extracting the fault rocks and leaving the hanging walls and foot walls largely intact. Remaining exposure of the mineralised rocks is therefore poor. Subsidiary structures in the immediate wall rocks provide limited information on the mineralised material, and suggest that brecciated schist zones and associated thin quartz veins (some prismatic and vuggy) were the mining target. Similar material and structures are exposed in a west-striking fault zone at the Buds locality (A), although this site has not been mined. The Alpine Reef has more extensive quartz vein formation and silicification of fault breccias (A, C). These breccias include fragments of early formed hydrothermal quartz vein material that has been brecciated and re-cemented by quartz that is locally prismatic (C).
Hydrothermal sulphide minerals are rare in most of the mineralised faults, with scattered pyrite and arsenopyrite disseminated through quartz veinlets and as a replacement mineral in associated schist breccias. Arsenopyrite is relatively common in silicified portions of the Alpine Reef (C), occurring both as individual coarse grains (> 1 mm) and in fine-grained (< 0.5 mm) seams or microveinlets cutting silicified breccias. Gold occurs as separate grains in schist among the silicate minerals quartz, albite, chlorite and muscovite (A, B). Gold also commonly occurs encapsulated within the scattered sulphides, as 1–20 µm blebs (C). There has been minor alteration of metamorphic titanite to hydrothermal rutile associated with gold and sulphide mineralisation along with the localised silicification, but otherwise hydrothermal alteration is conspicuously absent.
Figure 5 Scanning electron images of gold from mineralised faults. A, B, A gold particle has replaced folded metamorphic silicates in Torlesse Terrane schist, Grays Reef. C, Surface textures of a gold grain from silicified breccia, Alpine Reef. D, E, Supergene gold from Buds Reef intergrown with iron oxyhydroxide (HFO), with localised growth of gold crystal faces. F, Angular gold grain encapsulated in arsenopyrite (now oxidised to HFO) from Conroys Reef.
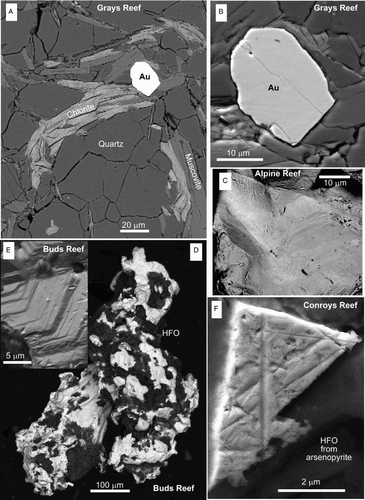
In addition to the prominent west-striking mineralised fault zone at Buds Reef (A) there is a set of more northerly striking mineralised breccia and gouge zones, with rare disrupted hydrothermal quartz veins (A–C; MacKenzie & Craw Citation2004). These breccia zones occur in Torlesse Terrane schist that has been disrupted by post-metamorphic folding near to the Old Man Fault (, A–C). The schist foliation strikes between north and northwest, and the breccia zones strike parallel or subparallel to that foliation but locally cross-cut that foliation (D, E). Brecciation during reactivation of the disrupted foliation has been at least partially responsible for development of these mineralised zones. The structures occur on a smooth ridge crest that is a remnant of the Cenozoic unconformity zone, so the rocks are more oxidised than the other mineralised faults described above. Sulphide minerals have therefore been fully decomposed, leaving only iron oxyhydroxide pseudomorphs. Likewise, gold in these structures is highly irregular in shape and has apparently been remobilised from the sulphides into the oxidised breccias, or has become intimately intergrown with iron oxyhydroxide (, 6E, F). Some crystalline supergene gold has formed in this oxidation process (E).
Figure 6 Mineralised breccia zones in north-striking structures associated with the Old Man Fault in Torlesse Terrane schists near to Buds Reef (after MacKenzie & Craw Citation2004). A–C, Cross-sections through road cuts, with measured distances from the western end of exposure. Deformed foliation and mineralised breccia zones are indicated. D, E, Stereonets (lower hemisphere) showing orientations of foliation and mineralised breccias in the cross section. F, Analytical data from mineralised breccias, showing sympathetic As and Sb enrichment. G, H, Scanning electron images of supergene gold particles from oxidised breccias. Fe ox = iron oxyhydroxide.
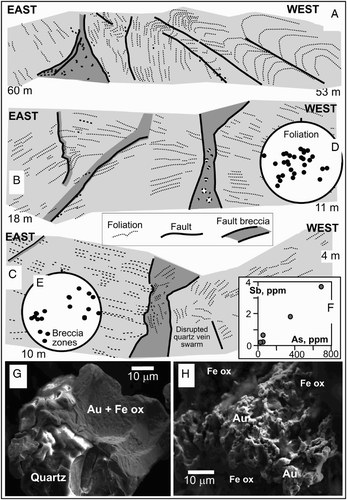
Mineralised fault rocks are almost invariably enriched in As, reflecting the hydrothermal arsenopyrite content (, 6F). However, post-mineralisation oxidation processes have leached As from the sulphide pseudomorphs in the Buds Reef area, presumably at the same time as supergene gold remobilisation was occurring (D). Nevertheless, even these oxidised rocks retain some As enrichment and also minor sympathetic Sb enrichment (F).
Mineralised faults in Caples Terrane
A swarm of gold-bearing faults strikes northwest across the Caples Terrane schist basement unconformity surface near Alexandra (A, A, B). These ‘reefs’ were mined on a small scale historically, in surface trenches and shallow (< 10 m below surface) underground tunnels. Exposures of these structures are all close to the Cenozoic unconformity (A) and the rocks have been extensively oxidised, with some associated clay alteration. While similar in general appearance to those that cut the Torlesse Terrane rocks (described above), these mineralised rocks have an additional component of alteration that obscures many of the original hydrothermal features. In this way, these Caples Terrane mineralised faults resemble the variably oxidised mineralised faults of the Buds Reef area (A ).
Figure 2 A, Hillshade topographic map of the Old Man Range showing the principal structures, mineralised faults and Clutha River channels described in this paper. Torlesse Terrane schists are indicated with a transparent pale overlay. B, Gold and As contents of samples from mineralised faults in the two basement terranes.
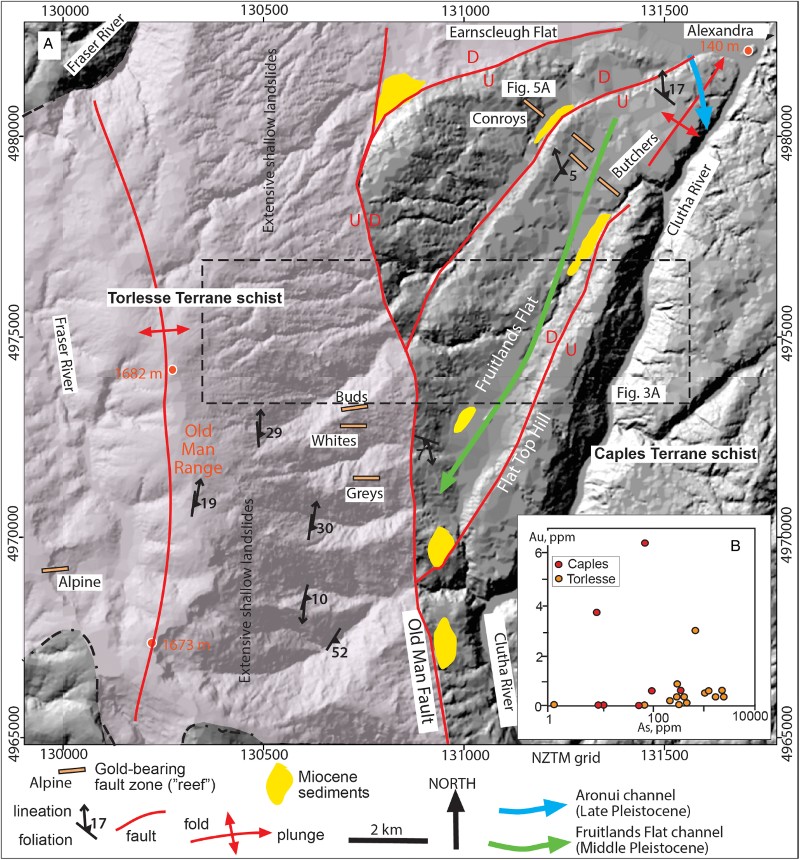
The mineralised faults in the Caples Terrane schist mainly dip steeply northeast, although some dip southwest (, D, A, B). Deflection of schist foliation adjacent to the faults is consistent with a normal sense of motion (, B). The faults commonly lie parallel or subparallel to prominent sets of joints within the host schist (, , A, C). Faults consist primarily of brecciated schist, with angular fragments ranging from 10 mm to 2 m across that have been variably rotated and internally disrupted (, B). These breccias, which are 1–4 m wide, have been cut by gouge zones typically 10–20 mm wide that consist of fine-grained crushed schist fragments.
Minor hydrothermal silicification of gouge zones and nearby breccia has occurred in some fault zones (B), but many of the faults have negligible added silica. Hydrothermal quartz veins are rare, although parts of the Conroys Reef system (A) have abundant quartz veins up to 5 cm across, cutting variably brecciated schist. Some of the quartz veins consist of prismatic quartz crystals, locally with vuggy cavities. Most quartz veins and silicified zones have been re-brecciated by subsequent fault movement. Pyrite and arsenopyrite are rare, and are scattered through silicified schist and quartz veins and occur as replacement minerals within schist breccia fragments. Extensive oxidation of the basement, associated with development of the Cenozoic unconformity, has caused almost complete decomposition of these sulphides and most calcite has been leached. However, rare relict sulphide fragments persist within some iron oxyhydroxide pseudomorphs after these sulphides, especially grains encapsulated in quartz-rich material. Gold occurs as micron -scale angular particles within these sulphide grains and their iron oxyhydroxide pseudomorphs (F; Craw et al. Citation2015). Some supergene remobilisation and localised migration of this primary gold has occurred during oxidation of the sulphides (Stephens et al. Citation2013; Craw et al. Citation2015).
Figure 7 Structure of Conroys Reef area (). A, Map of the mineralised faults and host schist structure, superimposed on aerial photograph (from Otago Regional Council). B, Sketch cross-section through a mineralised fault in a mine adit, as indicated in A. C, Photograph of jointed host schist outcrop.
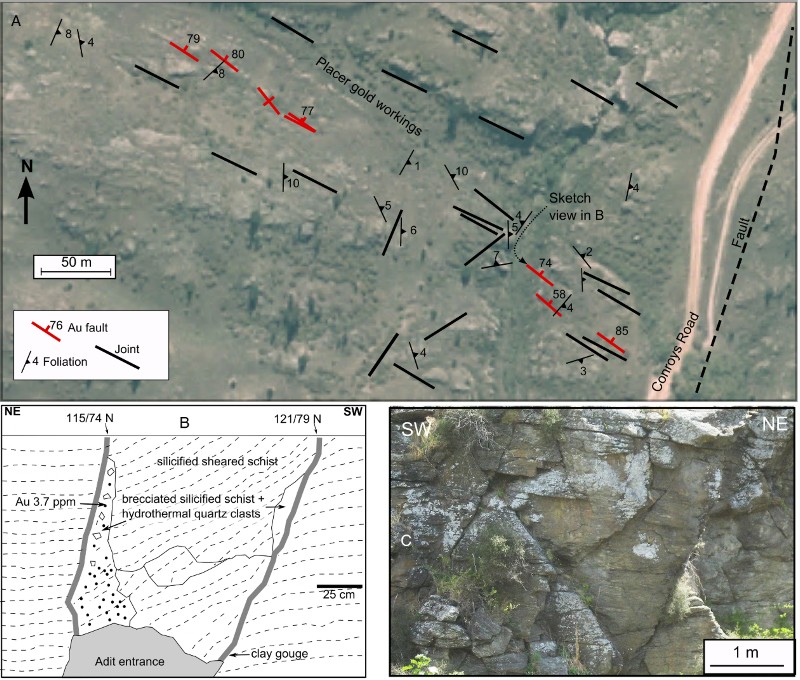
Despite the structural disruption and associated hydrothermal overprint, there has been only minor geochemical alteration in the fault zones. Anomalous Au concentrations are up to 6 ppm, and some of these are accompanied by weakly elevated (> 10 ppm) As concentrations (, B). Arsenic may have been leached from the rocks during oxidation of arsenopyrite, so these analytical data are minimal.
Sedimentary deposits
Late Cenozoic remnants
Remnants of late Cenozoic (Miocene?) non-marine deposits in the Old Man Range area are typically small in extent and restricted to thin (< 20 m) veneers of basal sediments resting on remnants of clay-altered basement in structurally controlled depressions (A). While most of these sediments are presumed to be Miocene (Douglas Citation1986; Turnbull Citation2000), localised recycling during uplift and erosion can produce essentially identical deposits of younger age, some as young as Middle Pleistocene (Stirling Citation1990; Craw et al. Citation2013). The basal sediments are commonly quartz-rich sandstones and/or conglomerates, some of which have been cemented to form silcrete (A–C). Quartz pebbles and cobbles are mainly subangular and angular, with a subordinate component of rounded and subrounded clasts (A, C). Disrupted and partially dispersed quartz-rich material from these deposits, resting on clay-altered basement (especially scattered blocks of silcrete, typically 0.5–2 m across), are all that is left of the late Cenozoic sequence in some areas.
Figure 8 Photographs of quartz clasts and gold in late Cenozoic conglomerates (). A, Partially silicified silcrete boulder, showing abundant angular and subrounded clasts, in a sedimentary remnant near Conroys Reef (). B, Gold particles in a subangular cobble of prismatic quartz from the same site as in A. C, Sedimentary remnant near Butchers Reefs (), showing a prominent rounded quartz clast (lower centre right) amid numerous subangular quartz clasts. D, Gold particles extracted from the deposit in C showing generally angular and/or equant grains, apart from one flake (lower right).
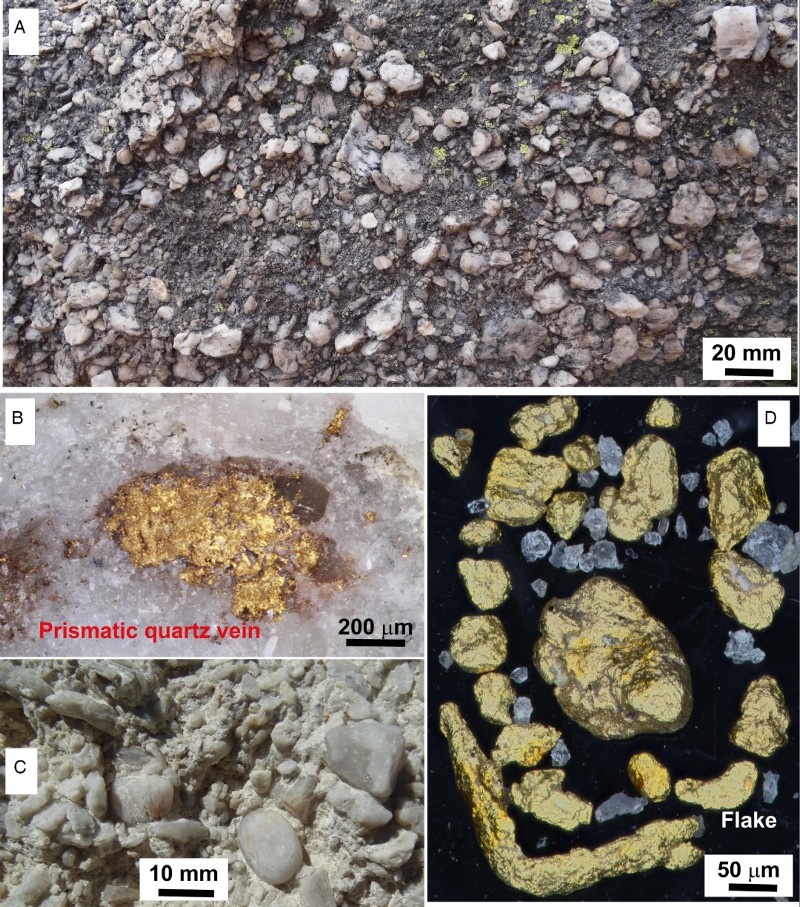
Many of these basal quartz-rich deposits, especially the conglomerates, contain detrital gold and have been the focus of past mining. A small structural depression at Potters Diggings, c. 8 km SSW of the Alpine Reef (A), contains remnants of basal quartz conglomerates that were re-deposited in the Pliocene (Stirling Citation1990), and these sediments are also auriferous . The detrital gold in many of the quartz conglomerates in central Otago is typically in the form of thin flakes 0.1–1 mm across, typical of gold that has undergone significant transport (tens to hundreds of kilometres; Youngson & Craw Citation1993, Citation1999). However, quartz conglomerates in the immediate vicinity (< 500 m) of the Conroys mineralised faults (, 7A) contain subangular cobbles up to 10 cm across of hydrothermal quartz vein material with visible gold grains (B). Similarly, quartz conglomerates near Butchers mineralised faults (A) contain angular and subrounded equant gold grains and wire gold, indicative of local derivation, with only rare flakes (D).
Pleistocene–Holocene sediments
The combination of rising mountains, aridity, localised periglacial processes and fissile schist basement has led to widespread Pleistocene–Holocene colluvium and landslides. These deposits and associated alluvial fans coat most surfaces and partially fill most valleys on range margins, with locally derived sediments up to 50 m thick. Alluvial fans include interlayered fluvial deposits, debris flows and loess horizons and fill channels cut into schist basement or remnants of late Cenozoic sediments (A–C). The Pleistocene sediments are poorly sorted, with abundant angular to subrounded schist clasts from millimetre to metre scale in a matrix of clay and silt. The fine-grained matrix, combined with some post-depositional clay alteration typical of these deposits (Chamberlain et al. Citation1999), has cemented and partially lithified many of these deposits which are consequently resistant to erosion (A–C).
Figure 9 Pleistocene alluvial fan sediments exposed in historic mines on Fruitlands Flat. Gold occurs near to the basal unconformity, indicated with a dashed line in A and B. A, Pleistocene gravels are channelised in inferred Miocene quartz sandstone. B, Close view of the unconformity in A, showing angular and poorly sorted locally derived Pleistocene gravel. C, Pleistocene gravels that unconformably lie on Caples Terrane schist basement, with a mine adit that followed the unconformity.
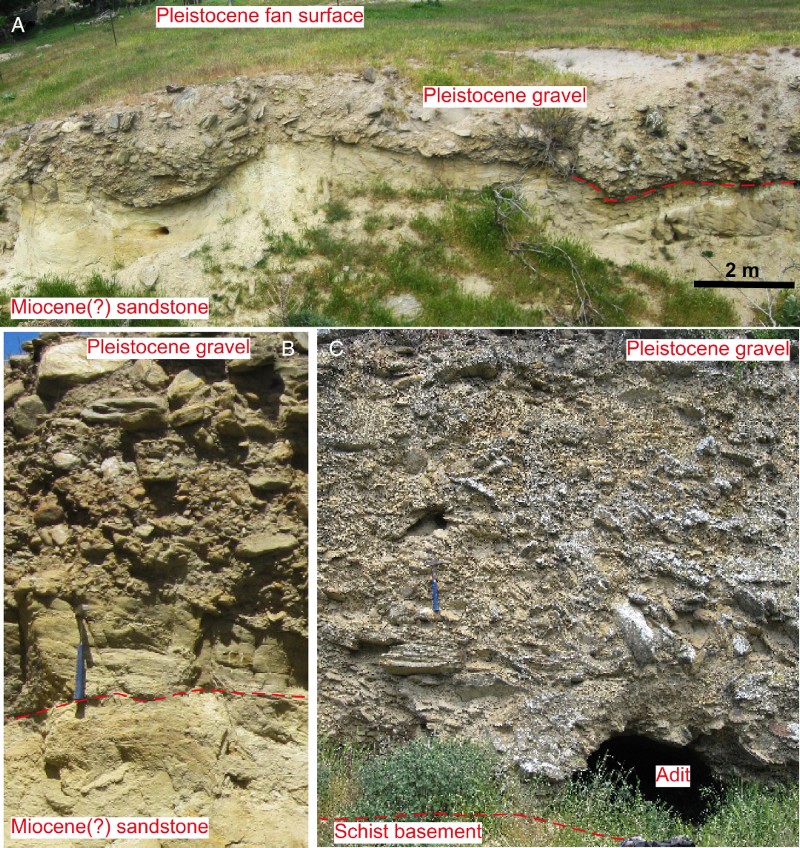
Clasts in these sediments are mostly locally derived from schist basement and/or from remnants of older quartz-rich sediments. Most clasts in the auriferous deposits on the eastern slopes of the Old Man Range and western slopes of Flat Top Hill (, 9A–C) were derived from nearby Caples Terrane basement. However, a localised deposit of subrounded auriferous gravels with Torlesse Terrane schist clasts, presumably derived from the upper slopes of the Old Man Range, lies unconformably on quartz-rich sediments at the base of Flat Top Hill (A). Detrital gold occurs scattered through these schist-dominated deposits, particularly near to their basal unconformity, either with remnant late Cenozoic sediments (A, B) or with schist basement (C). Gold particles recovered in this study are all angular and irregular in shape (A–C). Minor supergene addition of gold on to particle surfaces has occurred in situ (D), presumably accompanying the clay alteration and lithification processes.
Figure 10 Scanning electron images of locally derived detrital gold particles from Pleistocene–Holocene sediments on the Old Man Range. A, Particle from gravels in Fraser River on the western side of the crest of the range (). B, C, Particles from basal unconformity of Pleistocene gravels at Fruitlands Flat (Fig. 10). D, Surface texture of the gold particle in C, showing minor post-depositional addition of gold as spherical buds (centre and lower right) and thin plates (right).
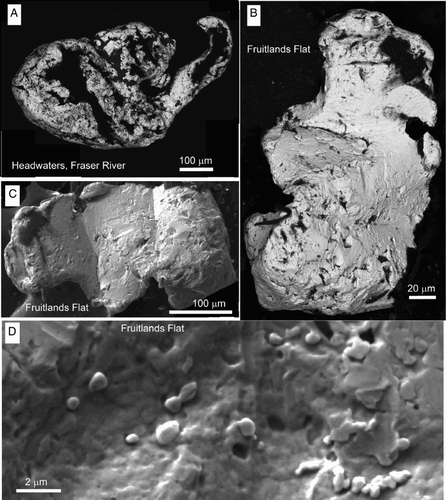
Ancestral (Pleistocene) Clutha River channels can be inferred from the presence of rounded polymict gravel deposits above the present river level on the eastern side of the Old Man Range (A; Turnbull Citation2000; Craw Citation2013). Gravels deposited by the Clutha River, both present and ancestral, are distinctly different from the adjacent and nearby locally derived colluvium and alluvial fans (A–D). Clutha sedimentary clasts are well rounded, the sediments are moderately sorted with only minor silt or clay components and the deposits are locally imbricated (B, D). Clasts include a wide range of different rock types, including schist and metamorphic quartz from both Caples and Torlesse terranes, and greywacke derived from both these terranes on the margins of the Otago Schist belt (, 11B, D). Locally high proportions of Torlesse Terrane greywacke clasts may imply substantial input from the nearby Manuherikia River (; McDonnell & Craw Citation2003). In addition, there are numerous clasts of distinctive metabasite and metachert, mostly derived from the Aspiring Terrane in the headwaters of the Clutha River and its largest tributary, the Kawarau River (; Craw et al. Citation2012; Craw Citation2013). Minor unmetamorphosed mafic volcanic clasts were also derived from the upper Clutha catchment (Craw et al. Citation2012; Craw Citation2013). Detrital gold particles in Clutha River sediments are flaky in shape, commonly with folded edges (C) reflecting progressive shape evolution during fluvial transport (Youngson & Craw Citation1999). This gold is accompanied by abundant detrital magnetite derived from the Aspiring Terrane, whereas magnetite is rare or absent from the locally derived alluvial fan sediments.
Figure 11 A schematic section of exposure of a wall of the Aronui channel of the Clutha River (Late Pleistocene; ). B–D, Lower channel sediments were transported by the Clutha River and include rounded clasts of highly variable provenance with flaky detrital gold. E, Upper sediments are locally derived angular and poorly sorted colluvium that post-dated channel abandonment, with minor rounded clasts of Torlesse Terrane greywacke recycled from the underlying Clutha sediments.
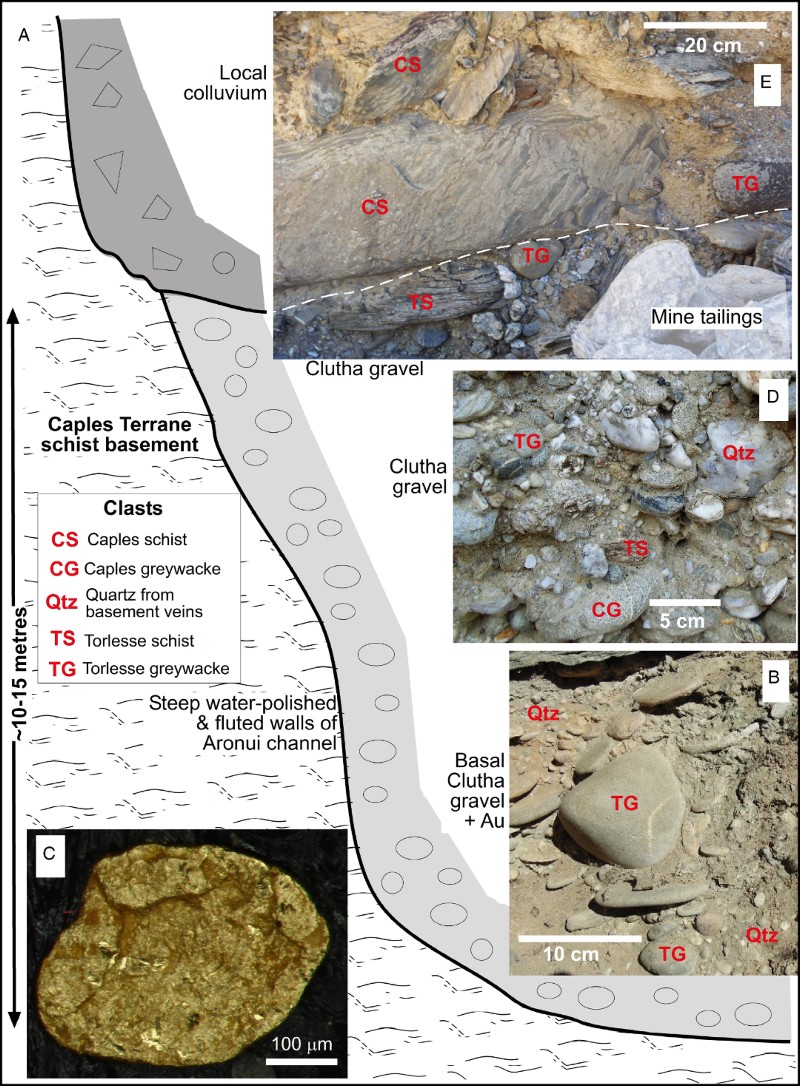
Discussion
Emplacement of orogenic gold
Orogenic gold was emplaced into the basement in Central Otago in two distinct events durng the Cretaceous (Mortensen et al. Citation2010). The earlier event (Early Cretaceous) included formation of the world-class Macraes deposit in the Hyde-Macraes Shear Zone, which is a regional-scale thrust structure (; Teagle et al. Citation1990; Mortensen et al. Citation2010). Mineralisation occurred in lower greenschist facies and subgreenschist facies rocks on the margins of the schist belt, before the high-grade (upper greenschist facies) Torlesse Terrane core of the Otago Schist belt was exhumed in Central Otago (Gray & Foster Citation2004; Mortensen et al. Citation2010). Mineralisation occurred at, or near to, the brittle–ductile transition, and quartz veins are typically massive with some ductile deformation and recrystallisation (Mortensen et al. Citation2010).
In contrast, the later mineralising event occurred during the middle–Late Cretaceous (c. 112–100 Ma; Mortensen et al. Citation2010). Orogenic gold was emplaced into normal faults that occur right across the Otago Schist belt in Central Otago, in host rocks of a wide range of metamorphic grades (; Williams Citation1974; Mackie et al. Citation2009; Mortensen et al. Citation2010). Deposits were emplaced at relatively shallow crustal levels (upper few kilometres), and are characterised by quartz veins and variably silicified breccias (Craw & Norris Citation1991; Mortensen et al. Citation2010).
Mineralised normal faults in the Old Man Range area all have similar characteristics with brittle breccias, gouge zones, variably prismatic and vuggy quartz veins and minor silicification in both Caples and Torlesse Terrane host rocks . Normal faults in the Old Man Range area were active in the middle–Late Cretaceous, and this deformation persisted at least until 96 Ma in the Alexandra area (A; Barker et al. Citation2010). The Tuapeka Fault () was also active as a normal fault in the Late Cretaceous , causing juxtaposition of different metamorphic zones (Bishop & Turnbull Citation1996; Turnbull Citation2000; Els et al. Citation2003). The northern continuation of the Tuapeka Fault as the Old Man Fault () was responsible for juxtaposition of Caples and Torlesse Terrane basement on the Old Man Range during this middle–Late Cretaceous extension. Minor gold mineralisation occurred along breccia zones associated with the Old Man Fault as an extensional structure (A–H). The structural style of mineralised faults in the Old Man Range area, and the distribution of these mineralised faults relative to the basement terranes, is therefore consistent with gold emplacement in the later mineralising event in Central Otago.
The principal distinction between mineralised faults in Caples Terrane and in Torlesse Terrane basement in the Old Man Range area lies in the orientations of most of the faults, although the Torlesse faults are more variable than the Caples faults (, 2A, 3C, E). These differing orientations of mineralised faults reflect, at least in part, differences in orientations of joints in the immediate host rocks in the two terranes (, C, E, 4A, B, 7A, C). Joint orientations in the basement developed during late metamorphic uplift (Weinberger et al. Citation2010), and therefore pre-dated the formation of the mineralised normal faults. These joints apparently controlled the orientations of later formed normal faults in the two different basement terranes (A). Additional local structural control was provided by the deformation zone associated with the Old Man Fault (–E, 12A). Further, since Torlesse Terrane schists structurally underlie the Caples Terrane rocks on the eastern side of the Old Man Range, there is presumably a change in orientation of joints upsection across the terrane boundary (A). Deeper feeder faults beneath the northwest-striking mineralised faults in the exposed Caples Terrane may therefore strike westwards in the underlying Torlesse Terrane (A).
Figure 12 Conceptual sketches showing key stages in the evolution of the gold deposits of the Old Man Range. A, Block diagram contrasting the structure of orogenic gold deposits in Late Cretaceous faults cutting Torlesse and Caples Terrane schist basement. B, Miocene non-marine sediments collect some placer gold from underlying schist basement. C, Progressive downcutting of the Clutha River in the Pleistocene has facilitated gold placer formation, mainly from locally derived mineralised faults.
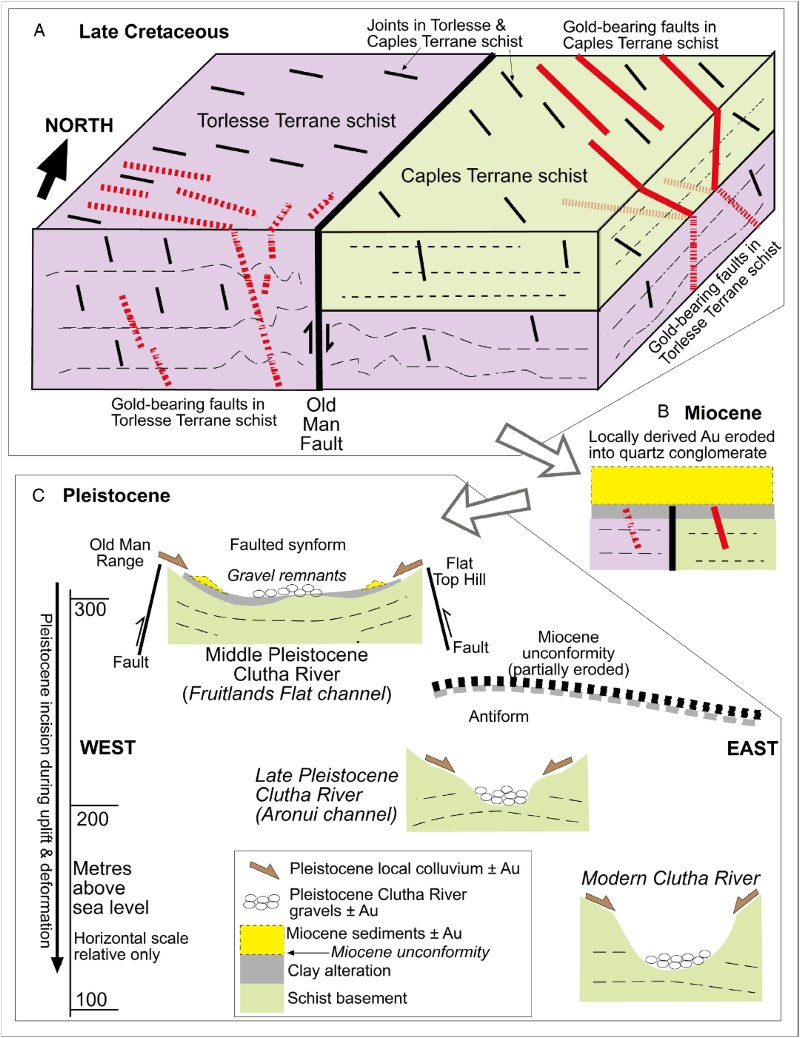
Clutha River channels
In the vicinity of the Old Man Range, the modern Clutha River channel is 50–150 m in width in a dominantly V-shaped bedrock gorge, which is incised up to 350 m into schist basement (, 3A). A Late Pleistocene palaeochannel near Alexandra, referred to as the Aronui channel here (A), is traceable for over 1.3 km as a bedrock gorge with a floor c. 70 m above present river level (, 11A). Minor remnants of Clutha River sediments at the north end of Fruitlands Flat, and at similar altitudes (c. 300 m) further south, define the location of an older channel of possible Middle Pleistocene age (A; Turnbull Citation2000). These gravels include rounded clasts of Torlesse Terrane greywacke and Aspiring Terrane metabasite and metachert clasts. These older Clutha River deposits occur in close proximity to Miocene sedimentary remnants and/or clay-altered schist of the immediately underlying Cenozoic unconformity. This Fruitlands Flat channel (A) apparently followed close to the Cenozoic unconformity surface at a low gradient, with little or no incision into the schist basement.
The history of progressive eastwards migration and deeper incision of the Clutha River in the Pleistocene is summarised in C. These processes were driven by Pleistocene uplift of the Old Man Range and associated structures in the Fruitlands Flat area (A; Stirling Citation1990; Jackson et al. Citation1996). Coevally, the Clutha River occasionally flowed at higher levels than at present as thick gravel deposits accumulated in the basin immediately upstream of the Old Man Range during glacial advances (Turnbull Citation2000). The Fruitlands Flat and Aronui channels may therefore have been occupied by the river only as subsidiary channels during these periods of gravel accumulation, and this has been assumed by Turnbull (Citation2000) who used the elevations of the channels to estimate their ages in relation to gravels in the upstream basin. Downcutting into schist basement in the present river gorge has occurred at various times through the Pleistocene when the channels were not choked with glacial outwash gravel. Diversion from the Fruitlands Flat channel may have been facilitated by broadening of the Old Man Range uplift zone, including the Fruitlands Flat faulted synform and associated Flat Top Hill faulted ridge (, 12C). Similarly, diversion of the river from the Aronui channel into the present gorge may have been facilitated by progressive uplift of the antiform at the northern end of the Flat Top Hill ridge (, 12C).
Placer gold
The Clutha River has hosted placer gold mining since 1861 and more than 100 large dredges have operated at various times in the river bed and immediately adjacent gravels, including in the modern gorge to the east of the Old Man Range (A; Williams Citation1974; Craw Citation2013). The Earnscleugh Flat (A) has historically hosted several dredges , and a major modern mining operation on that flat closed in 2014. Alluvial gold is readily transported by the Clutha River and its Kawarau tributary over 200 km in river bed load (; Youngson & Craw Citation1999). Placer gold also occurs in some Clutha River palaeochannels, such as the Aronui channel (, 11A, C). The clasts in the gravel remnants in the Fruitlands Flat palaeochannel suggest that the provenance of the Clutha River sediments at the time of occupation of that channel was similar to present, including the gold-bearing Manuherikia River (Youngson & Craw Citation1993; McDonnell & Craw Citation2003; Craw Citation2013). A Middle Pleistocene ancestral Clutha River carried detrital gold through the lower reaches of what is now the Manuherikia Valley near Alexandra (; Craw Citation2013; Craw et al. Citation2013). The Clutha River has therefore apparently been an important transporter of placer gold along the flanks of the Old Man Range through the Middle–Late Pleistocene.
Despite the importance of the Clutha River as a detrital gold source, there is remarkably little evidence for flaky particles of Clutha River gold on Fruitlands Flat. Historic mine sites on Fruitlands Flat (A–C) contain relatively angular and equant gold particles (B, C), and flattened flakes are conspicuously absent. This gold occurs associated with locally derived sediments and is presumably also locally derived from orogenic deposits on the slopes above (A). These observations suggest that any Clutha River detrital gold along the Fruitlands Flat channel is still buried beneath the shallow sediments of the floor of the flat, or was removed by fluvial processes during river reorientation.
Likewise, Miocene quartz conglomerates in Central Otago commonly contain abundant distinctive flaky gold particles (Youngson & Craw Citation1993, Citation1995). These flaky particles are readily recycled into younger sediments as Miocene sediments are uplifted and eroded (Youngson & Craw Citation1993, Citation1995). However, these flaky particles are conspicuously rare or absent from the quartz-rich sediment remnants on the eastern slopes of the Old Man Range and nearby Pleistocene deposits. Instead, gold in these late Cenozoic sediments is at least partially locally derived (A, B), again presumably from the nearby orogenic deposits of the Old Man Range described above (, A, B, B).
The above observations suggest that many of the placer gold deposits of the Old Man Range area are relatively unusual in a Central Otago context, in that the gold has been largely locally derived directly from nearby small orogenic deposits. These orogenic deposits have contributed detrital gold to the Miocene quartz conglomerates and their locally recycled equivalents, and to many of the Pleistocene placers. This is in strong contrast to other parts of central Otago, where there is abundant evidence for long-distance transport of gold and repeated recycling of that gold since the middle Cretaceous exhumation of the schist belt (Youngson & Craw Citation1993, Citation1995; Youngson et al. Citation2006; Craw Citation2010, Citation2013).
Conclusions
The Old Man Range of Central Otago is a prominent geomorphological feature of the area, and this range has been actively rising during the Pleistocene. The range crest is underlain by Torlesse Terrane upper greenschist facies schist, whereas the base of the eastern flank is lower greenschist facies Caples Terrane schist. These different schist types were juxtaposed in the Cretaceous by the Old Man Fault. This fault is still active and is facilitating uplift of the range. Small orogenic gold deposits were emplaced in brittle normal faults during the Late Cretaceous. The mineralised fault rocks are dominated by schist breccias and fault gouges that have been variably silicified and veined by quartz, with only minor scattered pyrite and arsenopyrite and almost no alteration of silicates. Gold occurs encapsulated in sulphides as free particles in quartz veins is locally dispersed among silicates in wall rocks.
The mineralised faults were controlled by pre-existing structures in the host schists. Post-metamorphic joints provided the principal structural control on fault orientations. Joints and mineralised faults strike northwest in Caples Terrane schists and have similar dips. In contrast, mineralised faults in Torlesse Terrane schists mainly strike westwards, parallel to nearby joints. In addition, some brecciated fault zones in Torlesse Terrane schists adjacent to the Old Man Fault strike northwards, and some of these structures have been mineralised with minor quartz veining, sulphides and gold. Oxidation and clay alteration, possibly during the Cenozoic, has strongly affected deposits in Caples Terrane schist on the lower slopes of the Old Man Range, and most gold has been remobilised during supergene processes. Similar but less intense supergene alteration has affected some of the gold in the Torlesse Terrane schist host.
Remnants of late Cenozoic non-marine sediments occur on the Old Man Range, and some of these contain detrital gold. At least some of that detrital gold was locally derived from the nearby mineralised faults. Pleistocene alluvial fan deposits derived from drainages formed during the rise of the Old Man Range also contain locally derived detrital gold. These gold particles are equant rather than flaky, and have angular and irregular shapes reflecting their local derivation and only minor modification in streams. In contrast, the Clutha River that flows along the eastern margin of the Old Man Range contains abundant flaky detrital gold derived from elsewhere in Central Otago, via several generations of recycling. The Clutha River became progressively incised into the schist basement as the Old Man Range rose during the Middle–Late Pleistocene, leaving abandoned perched channels. The Late Pleistocene Aronui channel contains flaky alluvial gold typical of the modern Clutha River bed load. However, a higher, older, abandoned channel through what is now Fruitlands Flat has left little evidence for externally derived flaky gold and most gold in the area is locally derived, either directly from mineralised faults in the nearby schist basement or via recycling of gold in locally derived quartz conglomerates.
Acknowledgements
Alistair Campbell kindly permitted access to some of the land, as did the Department of Conservation. Technical assistance was ably provided by Luke Easterbrook-Clarke, Brent Pooley, Kat Lilly and Damian Walls, and Lisa Craw skilfully assisted with drafting diagrams. Constructive and helpful reviews by Simon Cox and Patti Durance substantially improved the presentation.
Associate Editor: Dr Richard Wysoczanski.
Funding
This research was funded by Hardie Resources Ltd and the New Zealand Ministry for Business Innovation and Employment. We particularly thank Al Richardson of Hardie Resources for his interest, enthusiasm and support for the project.
Disclosure statement
No potential conflict of interest was reported by the authors.
References
- Barker SLL, Sibson RH, Palin JM, FitzGerald JD, Reddy S, Warr LN, et al. 2010. Cretaceous age, composition, and microstructure of pseudotachylyte in the Otago Schist, New Zealand. New Zealand Journal of Geology and Geophysics 53: 15–29. doi: 10.1080/00288301003631764
- Bierlein FP, Craw D 2009. Petrogenetic character and provenance of metabasalts in the Aspiring and Torlesse Terranes, South Island, New Zealand: implications for the gold endowment of the Otago Schist? Chemical Geology 260: 301–315. doi: 10.1016/j.chemgeo.2009.01.016
- Bishop DG, Turnbull IM 1996. Geology of the Dunedin area. Institute of Geological & Nuclear Sciences 1:250 000 geological map 21. Lower Hutt, Institute of Geological & Nuclear Sciences Ltd. 1 sheet + 52 p.
- Chamberlain CP, Poage MA, Craw D, Reynolds RC 1999. Topographic development of the Southern Alps recorded by the isotopic composition of authigenic clay minerals, South Island, New Zealand. Chemical Geology 155: 279–294. doi: 10.1016/S0009-2541(98)00165-X
- Craw D 1994. Contrasting alteration mineralogy at an unconformity beneath auriferous terrestrial sediments, Central Otago, New Zealand. Sedimentary Geology 92: 17–30. doi: 10.1016/0037-0738(94)90052-3
- Craw D 2010. Delayed accumulation of placers during exhumation of orogenic gold in southern New Zealand. Ore Geology Reviews 37: 224–235. doi: 10.1016/j.oregeorev.2010.03.006
- Craw D 2013. River drainage reorientation during placer gold accumulation, southern New Zealand. Mineralium Deposita 48: 841–860. doi: 10.1007/s00126-013-0464-5
- Craw D, Norris RJ 1991. Metamorphogenic Au-W veins and regional tectonics: mineralisation throughout the uplift history of the Haast Schist, New Zealand. New Zealand Journal of Geology and Geophysics 34: 373–383. doi: 10.1080/00288306.1991.9514476
- Craw D, Upton P, Walcott R, Burridge C, Waters J 2012. Tectonic controls on the evolution of the Clutha River catchment, New Zealand. New Zealand Journal of Geology and Geophysics 55: 345–359. doi: 10.1080/00288306.2012.709184
- Craw D, Bartle A, Fenton J, Henderson S 2013. Lithostratigraphy of gold-bearing Quaternary gravels, middle Manuherikia Valley, Central Otago, New Zealand. New Zealand Journal of Geology and Geophysics 56: 154–170. doi: 10.1080/00288306.2013.803984
- Craw D, MacKenzie DJ, Grieve P 2015. Supergene gold mobility in orogenic gold deposits, Otago Schist, New Zealand. New Zealand Journal of Geology and Geophysics 58: 123–136. doi: 10.1080/00288306.2014.997746
- Deckert H, Ring U, Mortimer N 2002. Tectonic significance of Cretaceous bivergent extensional shear zones in the Torlesse accretionary wedge, central Otago Schist, New Zealand. New Zealand Journal of Geology and Geophysics 45: 537–547. doi: 10.1080/00288306.2002.9514990
- Douglas BJ 1986. Lignite resources of Central Otago. Publication P104. Auckland, NZ Energy Research and Development Committee, University of Auckland. 368 p.
- Els BG, Youngson JH, Craw D 2003. Blue Spur Conglomerate: auriferous Late Cretaceous fluvial channel deposits adjacent to normal fault scarps, southeast Otago, New Zealand. New Zealand Journal of Geology and Geophysics 46: 123–139. doi: 10.1080/00288306.2003.9515000
- Gray DR, Foster DA 2004. 40Ar/39Ar thermochronologic constraints on deformation, metamorphism and cooling/exhumation of a Mesozoic accretionary wedge, Otago Schist, New Zealand. Tectonophysics 385: 181–210. doi: 10.1016/j.tecto.2004.05.001
- Jackson J, Norris RJ, Youngson JH 1996. The structural evolution of active fault and fold systems in Central Otago, New Zealand: evidence revealed by drainage patterns. Journal of Structural Geology 18: 217–234. doi: 10.1016/S0191-8141(96)80046-0
- Landis CA, Campbell HJ, Begg JG, Mildenhall DC, Paterson AM, Trewick SA 2008. The Waipounamu erosion surface: questioning the antiquity of the New Zealand land surface and terrestrial fauna and flora. Geological Magazine 145: 173–197. doi: 10.1017/S0016756807004268
- MacKenzie DJ, Craw D 2004. Gold in fault gouge without quartz veins, Old Man Range, Central Otago. Proceedings of the 37th Australasian Institute of Mining and Metallurgy, New Zealand Branch Annual Conference. Carlton, AusIMM. Pp. 299–308.
- MacKenzie DJ, Craw D 2005. Structural and lithological continuity and discontinuity in the Otago Schist, Central Otago, New Zealand. New Zealand Journal of Geology and Geophysics 48: 279–293. doi: 10.1080/00288306.2005.9515115
- Mackie C, MacKenzie DJ, Craw D 2009. Structural and lithological controls on gold mineralization at Oturehua on the northeastern margin of the Otago Schist, New Zealand. New Zealand Journal of Geology and Geophysics 52: 43–57. doi: 10.1080/00288300909509877
- McDonnell M, Craw D 2003. Stratigraphy and provenance of Pliocene greywacke-bearing conglomerate, Cardrona valley, Otago, New Zealand. New Zealand Journal of Geology and Geophysics 46: 425–436. doi: 10.1080/00288306.2003.9515018
- Mortensen JK, Craw D, MacKenzie DJ, Gabites JE, Ullrich T 2010. Age and origin of orogenic gold mineralisation in the Otago Schist belt, South Island, New Zealand: constraints from lead isotope and 40Ar/39Ar dating studies. Economic Geology 105: 777–793. doi: 10.2113/gsecongeo.105.4.777
- Mortimer N 1993. Jurassic tectonic history of the Otago Schist, New Zealand. Tectonics 12: 237–244. doi: 10.1029/92TC01563
- Stephens S, Craw D, MacKenzie D, Richardson A 2013. Combining geophysical data with geologic mapping for gold exploration in the Old Man Range, Central Otago. Proceedings of the 46th Australasian Institute of Mining and Metallurgy, New Zealand Branch Annual Conference. Carlton, AusIMM. Pp. 465–474.
- Stirling MW 1990. The Old Man Range and Garvie Mountains: tectonic geomorphology of the Central Otago peneplain, New Zealand. New Zealand Journal of Geology and Geophysics 33: 233–243. doi: 10.1080/00288306.1990.10425681
- Teagle DAH, Norris RJ, Craw D 1990. Structural controls on gold-bearing quartz mineralization in a duplex thrust system, Hyde-Macraes Shear Zone, Otago Schist, New Zealand. Economic Geology 85: 1711–1719. doi: 10.2113/gsecongeo.85.8.1711
- Turnbull IM 2000. Geology of the Wakatipu area. Institute of Geological & Nuclear Sciences 1:250 000 geological map 18. Lower Hutt, Institute of Geological & Nuclear Sciences Ltd. 1 sheet + 72 p.
- Turnbull IM, Craw D, Norris RJ 1993. Pre-Miocene and post-Miocene deformation in the Bannockburn basin, Central Otago, New Zealand. New Zealand Journal of Geology and Geophysics 36: 107–115. doi: 10.1080/00288306.1993.9514558
- Weinberger R, Eyal Y, Mortimer N 2010. Formation of systematic joints in metamorphic rocks due to release of residual elastic strain energy, Otago Schist, New Zealand. Journal of Structural Geology 32: 288–305. doi: 10.1016/j.jsg.2009.12.003
- Williams GJ 1974. Economic Geology of New Zealand. Australasian Institute of Mining and Metallurgy Monograph 4. 490 p.
- Youngson JH, Craw D 1993. Gold nugget growth during tectonically induced sedimentary recycling, Otago, New Zealand. Sedimentary Geology 84: 71–88. doi: 10.1016/0037-0738(93)90046-8
- Youngson JH, Craw D 1995. Evolution of placer gold deposits during regional uplift, central Otago, New Zealand. Economic Geology 90: 731–745. doi: 10.2113/gsecongeo.90.4.731
- Youngson JH, Craw D 1999. Variation in placer style, gold morphology, and gold particle behavior down gravel bed-load rivers: an example from the Shotover/Arrow–Kawarau–Clutha river system, Otago, New Zealand. Economic Geology 94: 615–633. doi: 10.2113/gsecongeo.94.5.615
- Youngson JH, Craw D, Falconer DM 2006. Evolution of Cretaceous–Cenozoic quartz pebble conglomerate gold placers during basin formation and inversion, southern New Zealand. Ore Geology Reviews 28: 451–474. doi: 10.1016/j.oregeorev.2005.02.004