ABSTRACT
Radiation therapy may compromise the quality of bone around dental implants, and its ability to regenerate, remodel, and revascularize. This study aimed to describe the irradiation effect on the bone microstructure of the mandible using dental implants in a canine model. Five beagle dogs were exposed to 40 Gy fractionated radiation. In total, 20 dental implants were inserted, two in the irradiated and two in the non-irradiated side. The mandible bone blocks were subjected to 3D micro-computed tomography (µCT) imaging, later evaluated histomorphometrically by light microscopy and scanning electron microscopy. Alterations in irradiated bone were observed under µCT imaging showing an increased anisotropy, porosity, and pore volume. Bone surface-to-bone volume decreased. The bone to implant contact index was significantly reduced in the irradiated bone (75.6% ± 5.8%) as compared to the non-irradiated bone (85.1% ± 6.8%). In the irradiated mandible, osteocytes with their filopodial processes, the bone beneath the periosteum, and subperiosteal veins showed structural differences but were not significant, whereas the diameter of Haversian canals were smaller statistical significant as compared to the control side. The study highlights that radiation dosage of fractioned 40 Gy causes alterations in the alveolar bone microstructure with compatible osseointegration and clinically stable dental implants.
Introduction
Oral rehabilitation of cancer patients with dental implants is a meaningful postoperative procedure to increase the quality of life. Implant therapy is widely performed to restore oral (masticatory) function after tumor surgery. However, the combination of surgery and irradiation results in local impairment of bone quality and weak bone density.Citation1 Radiotherapy negatively influences dental implant placement and is suggested to be the cause of implant loss.Citation2 Cancer survivors experience accelerated bone loss that occurs in cancer therapy, generally more rapid and severe than normal age-related osteoporosis in males or postmenopausal bone loss in females.Citation3
Regarding the placement of dental implants in the irradiated bone, it has been reported in many clinical studies that the success rate varies from 75% to 99% with a follow-up of at least 5 years.Citation4 Radiation affects all components of the irradiated bone with different degrees of sensitivity. Alteration in the mineralized structure of bone due to irradiation might result in a pathological fracture.Citation5
Implant failure at relatively early stages after surgery indicates a need to understand the initial responses of the surrounding tissue to the implant.Citation6,Citation7 Several histological and ultrastructural studies have confirmed the process of bone remodeling as a tissue response to implantation.Citation8,Citation9 It is suggested that a woven bone is formed around the implants, which are resorbed, affecting the prognosis of the implant.Citation10,Citation11 A study conducted on animals showed a more pronounced decreased implant stability in irradiated samples compared to nonirradiated.Citation12 Irradiation resulted in the arrest of the bone repair process, altered trabecular arrangement of the newly formed bone, along with a change in the osteocyte characteristics.Citation13
Some investigators suggested the possibility of bone remodeling, with bone resorption by osteoclasts and bone formation by osteoblasts occurs alternatively even after the achievement of osseointegration.Citation10 The bone remodeling effect following radiation is still unclear as it varies depending upon its dose and site. The study on rabbits suggested long-term impairment of bone remodeling after post-implantation irradiation.Citation14,Citation15 In contrast, histological analysis of mandibular bone of dogs showed a higher level of remodeling in the irradiated area than in the non-irradiated one.Citation16
µCT is becoming a routine technique that provides accurate biological imaging with isotropic resolutions to identify the bone microarchitecture. It is a standard technique to assess the 3D trabecular microstructure. The parameters such as bone surface per volume ratio, trabecular number, thickness, separation, porosity, etc. are important determinants of bone integrity and quality.Citation17
Many of the previous studies were confined to either studying the implant stability or bone microstructure changes in the newly formed bone following single-dose radiotherapy. We attempt to provide both micro-CT and histomorphometric findings to understand the bone exposed to fractioned radiotherapy. We also aim to assess the implant stability and the irradiation effects on the bone microstructure around the mandibular implants in dogs on irradiated and control sites.
Methods
Canine model and specimen preparation
Five skeletally mature beagle dogs, aged 1–2 years, were used for this study. Materials used were previously described by Asikainen et al.Citation18 All mandibular premolars were extracted to create a space large enough for the application of two dental implants. The teeth were extracted under intravenous anesthesia with sodium pentobarbital (Mebunat, Orion, Espoo, Finland), 20 mg/kg. Three months after extractions, the right side of each mandible was irradiated in two sessions, each lasting 1 week. The dose for each session, 20 Gy was divided into five successive days of a week. The radiation was given under intravenous anesthesia, with an interval of approximately 1 month between the two radiation sessions to minimize mucosal damage. The total dosage was 40 Gy. The energy source used was a linear accelerator (Saturne III, CGR, France) with 6 MeV electrons. Irradiation was limited to that part of the mandible where the implants were planned. The protection of the control side was provided by an intraoral lead shield. The size of the treatment area was 4.5 × 3 cm.
Three months after ending the radiation sessions, the dental implants were inserted in the mandible under intravenous anesthesia. The implants, all fabricated of commercially pure titanium, were placed in both edentulous premolar sites. In total, 20 dental implants were inserted: four in each dog, two on the irradiated side, and two on the non-irradiated side of the mandible. This study design resulted in 10 test implants and 10 controls. The titanium implants were hollow cylinders, specifically fabricated for the present study (Straumann AG, Waldenberg, Switzerland). The diameter of the implants was 3 mm, and the insertion depth was 6 mm. The wounds were closed while exposing the supra-gingival implant parts but constrained by biting movements. All implants presented good primary stability. Procaine penicillin with dihydrostreptomycin, 1 ml/10 kg (Combiotic, Pfitzer, Switzerland) was administered daily post-operatively for 1 week.
Fixed prosthetic superstructures with the golden bridge were placed on the implants 3 months after implantation. After bridge fixation, the dogs were allowed to chew rubber bones to stimulate occlusal loading on the implants. The animals were sacrificed after 6 months, and the mandible bone blocks containing the implants were prepared using a hand saw buccolingual with external physiological cooling at a distance of about 2 cm from the nearest implant. Tissue segments were processed by bulk staining en bloc in basic fuchsin to visualize the lacuno-canalicular system.Citation19 Basic fuchsin stains cells and empty spaces in the bone matrix – blood cells, lacunae, canaliculi, microcracks. The specimens were embedded without preliminary decalcification in polymethyl methacrylate resin to prevent drying during scanning. All block biopsies were subjected to µCT imaging after that the specimens were prepared for light microscopy (LM) and scanning electron microscopy (SEM).
All procedures were approved by the Ethics Committee of the University of Kuopio (Nro 23 Zd: 04726 97,289,170; 11.7.1989). Animals were housed in the Laboratory and the experiments were carried out under the control of the University’s Guidelines for Animal Experimentation. All steps were taken to minimize pain. The bone blocks are processed as per our previous article publishedCitation18 and investigated now with new methods.
Micro-computed tomography (µCT)
A series of images (N = 352) of mandible bone blocks including the implants were scanned using Skyscan 1172 µCT (Bruker microCT, Kontich, Belgium). Images were acquired with a tube voltage of 100 kV, at 180° rotation, Al-Cu filter, and 30 µm pixel size. The images were reconstructed with NRecon and the analyses were calculated with Skyscan CT-Analyser®. The 3D visualization was done with Mathematica® 8 (Wolfram Research Inc., Champaign, IL, USA), and Skyscan CT-Analyser® and CT-Volume®. µCT analyses were made from each specimen before further processing.
The ratio of bone surface/bone volume (BS/BV), the specific bone surface can be derived easily by dividing it with the bone volume, which characterizes the complexity of structure: more surface means more complex structure per unit volume.
The volume of the pore was calculated as the total volume of all pores fully surrounded by bone divided by the mineral (bone + pores) volume.
The degree of anisotropy was measured by the presence or absence of preferential alignment of structures along a particular direction where value 1 is for the total anisotropy and 0 is for total isotropy
The thickness of compact bone was measured buccally and lingually as per our previous study by Heinonen V et al. (2018).Citation20
Bone-to-Implant Contact (BIC) % was measured as the length of bone contact with implant divided by the total length of implant surface at cortical bone X 100.Citation21
Preparation of the specimens
After the µCT experiments, all tissue blocks were cut parallel to the longitudinal axis of the implant in a buccolingual direction for histological examination. In total, five thin sections were prepared for the LM and about 4 mm thick slices were cut and polished for scanning electron microscopy (SEM) imaging. Consecutive sections were examined with LM and SEM, respectively ().
Figure 1. The locations of the tissue slices on the surface of alveolar bone. Five sections from each specimen were prepared for light microscopy (thin line, 40 µm) and scanning electron microscopy (4 mm block)
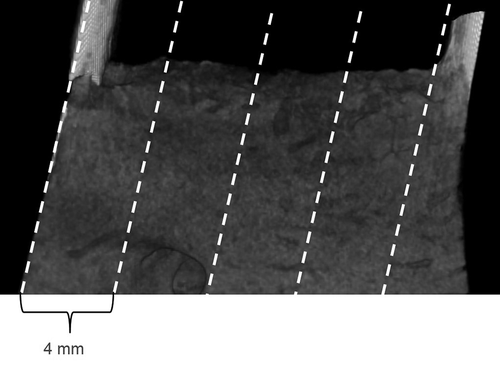
Light microscopy
Thin sections (40 µm) for analysis by LM were prepared using cutting and grinding systems (Exakt, Norderstedt, Germany). The slides were observed and photographed with a Zeiss AxioImager M2 (Carl Zeiss Microscopy GmbH, Jena, Germany) under bright field microscopy (BFM).
Scanning electron microscopy
The polished tissue blocks were acid-etched with 9% phosphoric acid for 60 seconds. Following etching, sections were immersed in sodium hypochlorite for 3 min and rinsed in distilled water.Citation22–24 The sections were placed in a desiccator overnight and thereafter the slices were coated by a thin 30–40 nm gold layer for SEM imaging. The specimens were examined and photographed using an XL30 ESEM TMP (Fei Co, Eindhoven, The Netherlands) or Zeiss Sigma HD VP (Carl Zeiss GmbH, Oberkochen, Germany) scanning electron microscope operated at 15 kV.
Histomorphometric analysis
A representative histological section was selected and compared with the µCT images, taking care to match specific homologous anatomical features in the bone anatomy (). Characteristic locations from LM histological sections were selected and compared with the SEM images (). From SEM specimens, simple histomorphometric analyses were performed manually by selecting regions of interest (ROI). ROI was selected and scanned at a magnification of 2000X and 9000X. Two investigators (TR, AMK) were blinded for the assessment of histomorphometric parameters of irradiated and control samples and put forth to study the following parameters: quantity of Haversian canals (HC), the diameter of HC (measured as a line tangential to the longest axis of the cross-section in ROIs), the thickness of new bone layer attached to the implant, and the number of veins in the lamellar and subperiosteal region.
Figure 2. The histological sections and their corresponding micro-CT images for the implants placed in the irradiated and non-irradiated mandible of a dog. For the measurements with SEM, the alveolar process of mandible was divided into three main regions of interest (ROIs): lateral areas, one buccally and one lingually, and one attached to implant. SEM images represents methods and the results are presented in . (Bars = 100 µm)
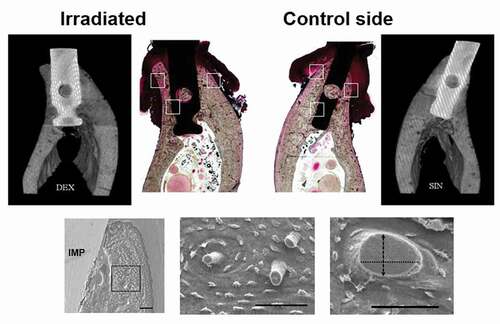
Statistical analysis
Statistical analyses were performed in SPSS Statistics Software for Windows (version 24; SPSS, Chicago, IL). For all parameters, mean values were calculated. One-way analysis of variance (ANOVA) was used to compare the microarchitecture of different parts of the bone. The paired t-test was performed for the differences between irradiated and non-irradiated bones. The results were considered statistically significant at the p <.05 level.
Results
No dental implants were lost during the experiment, and all animals responded well during the experiment. Clear alterations were evident in the microstructure of the irradiated bone.
summarizes the results of µCT measurements.
Table 1. Results of 3D micro-computed tomography bone analysis measurements
Bone surface/Bone volume (BS/BV) is slightly decreased in the irradiated side and porosity, anisotropy is slightly increased in the irradiated mandible, but no statistical differences were observed between the study and control groups (p > .05). µCT images of the alveolar ridge appeared thin on the buccal site compared to the lingual site. The irradiated site did not show any significant difference in thickness of compact bone compared with the control site. Bone to Implant Contact (BIC) studied by µCT was 85.1% ± 6.8% in the nonirradiated mandible and 75.6 ± 5.8% in the irradiated side, which was statistically significant (p < .01).
Micromorphometric data concerning the bone structure of the irradiated and non-irradiated mandibular sites are shown in . The new bone layer attached to the implant was about 185 µm in thickness as observed with BFM and SEM. On SEM, osteocytes with their filopodial processes were distributed in parallel along the new bone surface, but osteons were not visible (). The lamellar bone beneath the periosteum showed a canalicular network of osteocytes in control sites but a decreased and less organized canalicular connections between osteocytes and periosteum were noticed in irradiated sites compared to the controls ().
Table 2. Micromorphometric analyses of irradiated and control sides
Figure 3. The osteon density and the osteonal structure is illustrated using bright field microscopy of bulk basic fuchsin stained sections. New bone without osteons (arrows) is directly contacting the implant seen with bright field microscopy (A) and with scanning electron microscopy (B). Osteocytes with their filopodial processes are distributed in parallel along the preexisting bone. Abbreviations: IMP = implant; OM = oral mucosa
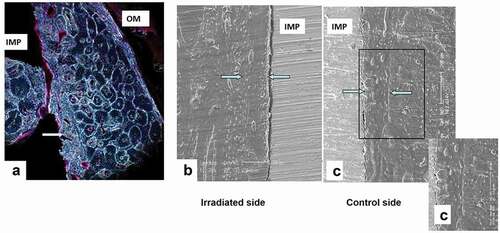
Figure 4. Scanning electron microscopy and bright field microscopy of alveolar bone (basic fuchsin). A) On the control side, the vitality of the alveolar bone beneath the periosteum is better than on the irradiated side. B and C) Osteocytes with few dendrites are located beneath the periosteum in the irradiated bone, whereas in control side, osteocytes with numerous dendrites are visible. (Bars = 100 µm)
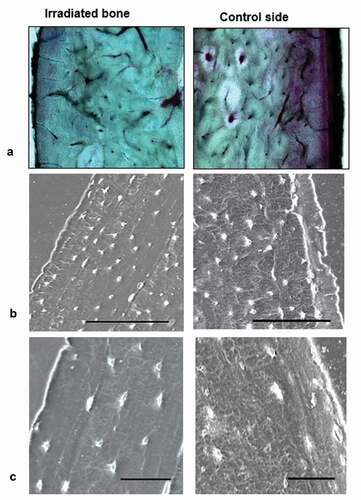
Haversian canals (HCs) were evident on both buccal and lingual sites of the alveolar bone with less number in the lingual site. Although there was no statistically significant difference in the quantity of HC, irradiated bone showed a decrease in HC quantity compared to the control site. A significant difference in the diameter of HC is exhibited between irradiated (Buccal – 33.04 ± 2.8 µm, Lingual- 27.30 ± 3.1 µm) and non-irradiated sites (Buccal – 43.08 ± 4.5 µm, Lingual- 34.13 ± 4.5 µm) displaying a mean diameter smaller in the non-irradiated sites. The number of lamellar veins is decreased on the irradiated buccal site compared to the control buccal site, which is significant (p < .05). The density of subperiosteal veins exhibited no significant changes but was increased on the lingual site compared to the buccal site of the irradiated mandible.
Discussion
This canine model study reports µCT and histomorphometric changes of irradiated mandibles with loaded dental implants. We utilized fractioned radiotherapy (40 Gy) which is clinically relevant to head and neck treatments as compared to single-dose radiotherapy models.
The canine jaw is considered very analogous to the human jaw and it represents the best potential animal model.Citation25 The turnover rate in beagle mandibles is close to the human mandible turnover rate, the remodeling period only being slightly shorter 3 months in dogs and 4–6 months in humans, respectively. The alveolar bone of the canine mandible also has high levels of intracortical remodeling, equivalent to the human mandible.Citation26 Furthermore, the turnover in the alveolar region is 2–4 times higher than in the basal portion of the mandible and 15–20 times higher than in bones from the appendicular skeleton.Citation27 Hence, canine models were used to study the microstructure of the mandible after radiotherapy.
Some experimental studies on irradiated rabbits and dogs have shown bone reactions around oral implants and obtained relatively high levels of osseointegration although less extensive than in non-irradiated animals.Citation4,Citation28 Our study found clinically that there was no difference in the stability of implants between the irradiated and control side, and no implants were lost.
µCT is a nondestructive technique, so we exposed the prepared blocks for µCT imaging so that the internal features of the same sample can be examined multiple times, and after scanning remaining intact samples are processed for additional biological testing such as examination of bone specimens with LM and SEM. The µCT parameter BS/BV characterizes the 3D bone trabecular strength and revealed a noticeable difference among groups. The degree of anisotropy, a description of how the structural elements are oriented may explain a significant part of the mechanical properties of a 3D structure. Bones are known to be anisotropic, which means they are stronger when loaded in a single direction. Healthy bone has a high degree of anisotropy; whereas degraded bone or haphazard bone deposition have a lower degree of anisotropy.Citation29 Literature shows that the increase in anisotropy is mainly due to the increase in the length of lacunae in the more porous bone region.Citation30 In our study, the pore volume, porosity, and degree of anisotropy showed a gradual increase during the healing period, but the values were not statistically significant between the irradiated and control sides of the mandibles.
It was noted in a previous studyCitation31 that osseointegration, mainly bone-to-implant contact (BIC) is never 100%. Even “clinically successful” implants have less than half of direct contact with bone. However, for successful implant treatment, stability and osseointegration attainment are necessary. BIC is essential in creating secondary stability and can be studied using micro-CT. The influence of different degrees of BIC on bone remodeling following dental implantation was evaluated and concluded that a final contact percentage of about 58%–60% has resulted when an equilibrium state is reached by bone remodeling.Citation31 More bone contact results in better implant stability.Citation32 In our study, all implants were clinically stable 6 months after loading, which might reflect that BIC appeared to increase with the healing process, but was significantly lower in the irradiated sites compared to the control sites. The BIC obtained in the irradiated site appeared adequate to achieve bone remodeling.
Osteocytes are considered the major mechanosensory cells of the bone remodeling process.Citation24 The maintenance of bone around the implant is directly dependent on the osteocytic lacunar canalicular system (OLCS) constitution.Citation33 In a recent study by Mendes et al.Citation13 showed a decrease in the number of osteocytes and increased empty lacunae after irradiation, which might negatively affect the mechanosensation system resulting in impaired bone repair. We demonstrated histological alterations in the bone around the implants through LM, BFM, and SEM. Osteocytes located parallel to the new bone layer attached to the implant with few canalicular processes are observed in irradiated than in the control bone. We did not find empty cellular lacunae, but osteocytes were observed under SEM. This study demonstrated the osteocytes without osteons in the bone adjacent to implants and confirmed the finding that the newly formed bone changed to the compact bone by continuous bone remodeling as suggested by Haga et al.Citation33
There were statistically significant changes in the mean diameter of the HC, appearing narrower on irradiated sites and less in number on the lingual site compared to the controls. Takahashi et al.Citation34 indicated that after irradiation, HC grows in width for 4 weeks but in later stages, the width of HC decreases with a decrease in the number of blood vessels. In our study, the buccal site of the irradiated jaw in the lamellar bone is presented with a significant decrease in veins, whereas the lingual site changes are minimal both at the periosteum and in the lamellar bone and statistically insignificant. Dekker et alCitation35 in their recent study on the irradiated human jaw, proposed that radiation dosage of more than 50 Gy affects the smaller vessels more than the larger vessels either due to a direct effect of radiation or due to inhibition of new blood vessel formation. In addition, vascularization promotes the survival of osteocytes after irradiation has been demonstrated.Citation13 We found new bone formation around implants with decreased vascularity in the subperiosteal and lamellar region after using fractioned radiotherapy of 40 Gy. This may be due to the coexistence of histological and functional modifications affecting bone tissue and its vascular network.
In conclusion, our histomorphometric analyses showed changes in the alveolar process of the mandible around dental implants on microscopic and cellular levels. We observed that the vital irradiated bone is presented with compatible osseointegration and clinically implant stability despite alterations in the microstructure of the bone. Optimal timing of implantation and prosthetic loading is a key factor to prevent the loss of bone mass and cellular changes in irradiated mandible. The obtained results adequately provide the bone changes in an irradiated mandibular bone, which forms a basis for future clinical studies to validate clinical protocols.
Acknowledgments
The authors would like to thank Ritva Savolainen and Virpi Miettinen, SIB Labs, University of Eastern Finland, for their kind help in the preparation of the specimens.
Disclosure Statement
The authors report no conflicts of interest. The authors alone are responsible for the content and writing of the paper.
References
- Brennan MT, Elting LS, Spijkervet FK. Systematic reviews of oral complications from cancer therapies. Oral Care Study Group, MASCC/ISOO; methodology and quality of the literature. Support Care Cancer. 2010;18(8):979–984. doi:https://doi.org/10.1007/s00520-010-0856-3.
- Alsaadi G, Quirynen M, Komárek A, van Steenberghe D. Impact of local and systemic factors on the incidence of late oral implant loss. Clin Oral Implants Res. 2008;19:670–676.
- Guise T. Bone loss and fracture risk associated with cancer therapy. Oncologist. 2006;11(10):1121–1131. doi:https://doi.org/10.1634/theoncologist.11-10-1121.
- Brasseur M, Brogniez V, Grêgoire V, et al. Effects of irradiation on bone remodelling around mandibular implants: an experimental study on gogs. Int J Oral Maxillofac Surg. 2006;35(9):850–855. doi:https://doi.org/10.1016/j.ijom.2006.03.016.
- Jegoux F, Malard O, Goyenvalle E, Aguado E, Daculsi G. Radiation effects on bone healing and reconstruction: interpretation of the literature. Oral Surg Oral Med Oral Pathol Oral Radiol Endodontol. 2010;109(2):173–184.doi:https://doi.org/10.1016/j.tripleo.2009.10.001.
- Friberg B, Jemt T, Lekholm U. Early failures in 4,641 consecutively placed Branemark dental implants: a study from stage 1 surgery to the connection of completed prostheses. Int J Oral Maxillofac Implants. 1991;6:142–146.
- Salonen MA, Oikarinen K, Virtanen K, Pernu H. Failures in the osseointegration of endosseous implants. Int J Oral Maxillofac Implants. 1993;8:92–97.
- Listgarten MA. Soft and hard tissue response to endosseous dental implants. Anat Rec. 1996;245(2):410–425. doi:https://doi.org/10.1002/(SICI)1097-0185(199606)245:2<410::AID-AR20>3.0.CO;2-R.
- Marco F, Milena F, Gianluca G, Vittoria O. Peri-implant osteogenesis in health and osteoporosis. Micron. 2005;36(7–8):630–644. doi:https://doi.org/10.1016/j.micron.2005.07.008.
- Berglundh T, Abrahamsson I, Lang NP, Lindhe J. De novo alveolar bone formation adjacent to endosseous implants. Clin Oral Implants Res. 2003;14(3):251–262. doi:https://doi.org/10.1034/j.1600-0501.2003.00972.x.
- Abrahamsson I, Berglundh T, Linder E, Lang NP, Lindhe J. Early bone formation adjacent to rough and turned endosseous implant surfaces. An experimental study in the dog. Clin Oral Implants Res. 2004;15(4):381–392. doi:https://doi.org/10.1111/j.1600-0501.2004.01082.x.
- Verdonck HW, Meijer GJ, Nieman FH, Stoll C, Riediger D, de Baat C. Quantitative computed tomography bone mineral density measurements in irradiated and non-irradiated minipig alveolar bone: an experimental study. Clin Oral Implants Res. 2008;19(5):465–468. doi:https://doi.org/10.1111/j.1600-0501.2007.01496.x.
- Mendes EM, Irie MS, Rabelo GD, et al. Effects of ionizing radiation on woven bone: influence on the osteocyte lacunar network, collagen maturation, and microarchitecture. Clin Oral Investig. 2020;24(8):2763–2771. doi:https://doi.org/10.1007/s00784-019-03138-x.
- Schön R, Ohno K, Kudo M, Michi KI. Peri-implant tissue reaction in bone irradiated the fifth day after implantation in rabbits: histologic and histomorphometric measurements. Int J Oral Maxillofac Implants. 1996;11:228–238.
- Kudo M, Matsui Y, Ohno K, Michi KI. A histomorphometric study of the tissue reaction around hydroxyapatite implants irradiated after placement. J Oral Maxillofac Surg. 2001;59(3):293–300. doi:https://doi.org/10.1053/joms.2001.20998.
- Brogniez V, Nyssen-Behets C, Gre´-goire V, Reychler H, Lengele´ B. Implant osseointegration in the irradiated mandible. A comparative study in dogs with a microradiographic and histologic assessment. Clin Oral Implants Res. 2002;13(3):234–242. doi:https://doi.org/10.1034/j.1600-0501.2002.130302.x.
- Nogueira LP, Braz D, Barroso RC, et al. 3D histomorphometric quantification of trabecular bones by computed microtomography using synchrotron radiation. Micron. 2010;41(8):990–996. doi:https://doi.org/10.1016/j.micron.2010.06.013.
- Asikainen P, Klemetti E, Kotilainen R, et al. Osseointegration of dental implants in bone irradiated with 40, 50 or 60 Gy doses. An experimental study with beagle dogs. Clin Oral Implants Res. 1998;9(1):20–25. doi:https://doi.org/10.1034/j.1600-0501.1998.090103.x.
- Burr DB, Stafford T. Validity of the bulk-staining technique to separate artifactual from in vivo bone microdamage. Clin Orthop Relat Res. 1990;260:305–308. doi:https://doi.org/10.1097/00003086-199011000-00047.
- Heinonen V, Routsalainen TJ, Paavola L, et al. Alveolar bone remodelling after tooth extraction in irradiated mandible: an experimental study with canine model. Ultrastruct Pathol. 2018;42(2):124–132. doi:https://doi.org/10.1080/01913123.2017.1422829.
- de Faria Vasconcelos K, Dos Santos Corpas L, da Silveira BM, et al. MicroCT assessment of bone microarchitecture in implant sites reconstructed with autogenous and xenogenous grafts: a pilot study. Clin Oral Implants Res. 2017;28(3):308–313. doi:https://doi.org/10.1111/clr.12799.
- Feng JQ, Ward LM, Liu S, et al. Loss of DMP1 causes rickets and osteomalacia and identifies the role for osteocytes in mineral metabolism. Nat Genet. 2006;38(11):1310–1315. doi:https://doi.org/10.1038/ng1905.
- Kubek DJ, GattoneII VH, Allen MR. Methodological assessment of acid-etching for visualizing the osteocyte lacunar-canalicular networks using scanning electron microscopy. Microsc Res Tech. 2010;73:182–186.
- Lampi T, Dekker H, Ten Bruggenkate CM, et al. Acid-etching technique of non-decalcified bone samples for visualizing osteocyte-lacuno-canalicular network using scanning electron microscope. Ultrastruct Pathol. 2018;42(1):74–79. doi:https://doi.org/10.1080/01913123.2017.1384418.
- Burr DB, Allen MR. Mandibular necrosis in beagle dogs treated with bisphosphonates. Orthod Craniofac Res. 2009;12(3):221–228. doi:https://doi.org/10.1111/j.1601-6343.2009.01456.x.
- Allen MR, Burr DB. Mandible matrix necrosis in beagle dogs after 3 years of daily oral bisphosphonate treatment. J Oral Maxillofac Surg. 2008;66(5):987–994. doi:https://doi.org/10.1016/j.joms.2008.01.038.
- Huja SS, Fernandez SA, Hill KJ, Li Y. Remodeling dynamics in the alveolar process in skeletally mature dogs. Anat Rec A Discov Mol Cell Evol Biol. 2006;288(12):1243-1249. doi:https://doi.org/10.1002/ar.a.20396.
- Visch LL, Van Waas MAJ, Schmitz PIM, Levendag PC. A clinical evaluation of implants in irradiated oral cancer patients. J Dent Res. 2002;81(12):856–859. doi:https://doi.org/10.1177/154405910208101212.
- Cole HA, Ohba T, Ichikawa J, et al. Micro-computed tomography derived anisotropy detects tumor provoked deviations in bone in an orthotopic osteosarcoma murine model. PLoS One. Jun 3 2014;9(6):e97381. doi:https://doi.org/10.1371/journal.pone.0097381.
- Dong P, Haupert S, Hesse B, et al. 3D osteocyte lacunar morphometric properties and distributions in human femoral cortical bone using synchrotron radiation micro-CT images. Bone. Mar 2014;60:172–185. doi:https://doi.org/10.1016/j.bone.2013.12.008.
- Lian Z, Guan H, Ivanovski S, Loo YC, Johnson NW, Zhang H. Effect of bone to implant contact percentage on bone remodelling surrounding a dental implant. Int J Oral Maxillofac Surg. 2010;39(7):690–698. doi:https://doi.org/10.1016/j.ijom.2010.03.020.
- Park YS, Yi KY, Lee IS, Jung YC. Correlation between microtomography and histomorphometry for assessment of implant osteointegration. Clin Oral Implants Res. 2005;16:156–160. doi:https://doi.org/10.1111/j.1600-0501.2004.01083.x.
- Haga M, Nozawa-Inoue K, Li M, Oda K, Maeda T, Morphological A. Analysis on the osteocytic lacunar canalicular system in bone surrounding dental implants. Oral Biol. 2011;294(6):1074–1082.
- Takahashi S, Sugimoto M, Kotoura Y, Sasai K, Oka M, Yamamuro T. Long-term changes in the Harversian systems following high – dose irradiation. an ultrastructural and quantitative histomorphology study. J Bone Joint Surg. 1994;76(5):722–738.doi:https://doi.org/10.2106/00004623-199405000-00014.
- Dekker H, Bravenboer N, Dijk DV, et al. The irradiated human mandible: a quantitative study on bone vascularity. Oral Oncol. 2018;87:126–130. doi:https://doi.org/10.1016/j.oraloncology.2018.10.030.