Abstract
This article tests the association between streamflow alteration and the alteration of ecologically significant hydraulic environments. There has been a recent shift in environmental flow assessments to develop rapid desktop-based approaches that are applicable in a regional context. Streamflow statistics (e.g. minimum monthly flow) are often chosen to predict the impact of streamflow alteration on aquatic ecosystems. The assumption that the flow–biota relationship will be obscured by the effect of how streamflow interacts with channel morphology is often acknowledged, but not quantified. In this study, streamflow statistics are derived for 19 reaches in four river systems in Victoria, Australia. Hydraulic metrics were used to quantify ecologically significant surface flow conditions (Froude number) and the area of bench inundation, shallow and deep water. Multivariate analysis was used to investigate the correlation between streamflow statistics altered with regulation and the hydraulic metrics. It was found that streamflow statistics have a weak correlation to surface flow condition and the area of shallow water under natural streamflow conditions. The results show that hydrologic statistics have limited utility in quantifying changes in hydraulic environments. A similar magnitude of flow alteration can produce diverse hydraulic results. The confounding influence of channel morphology prevents streamflow statistics being an adequate surrogate for the assessment of hydraulic alteration. Modelling flow–biota relationships in a regional context is limited by the inadequacy of streamflow statistics to model ecologically significant hydraulic function. Improving knowledge of ecohydraulically significant hydrologic statistics will improve the effectiveness of environmental flow planning to sustain instream habitat conditions. A probabilistic approach is required to enable a risk-based approach to desktop generalization of flow–biota relations.
Editor Z.W. Kundzewicz; Guest editor M. Acreman
Citation Turner, M. and Stewardson, M., 2014. Hydrologic indicators of hydraulic conditions that drive flow–biota relationships. Hydrological Sciences Journal, 59 (3–4), 659–672.
Résumé
Cet article étudie les relations entre les modifications des débits et la modification des environnements hydrauliques d’importance écologique. De nouvelles méthodes d’évaluation rapide des débits environnementaux sont récemment apparues, utilisant des ordinateurs de bureau, applicables dans un contexte régional. Des statistiques de l’écoulement fluvial (par exemple le débit minimum mensuel) ont souvent été choisies pour prévoir l’impact de la modification de l’écoulement fluvial sur les écosystèmes aquatiques. L’hypothèse selon laquelle la relation entre l’écoulement et le biote serait compliquée par la façon dont les débits interagissent avec la morphologie du chenal est souvent reconnue, mais non quantifiée. Dans cette étude, les statistiques de débits ont été calculées pour 19 biefs de quatre réseaux fluviaux de l’état de Victoria, en Australie. Les variables hydrauliques ont été utilisés pour quantifier les conditions d’importance écologique de l’écoulement superficiel (nombre de Froude), et la surface des zones inondables, des eaux peu profondes et des eaux profondes. Une analyse multivariée a été utilisée pour étudier la corrélation entre les statistiques de débits modifiés selon la réglementation et les mesures hydrauliques. On a constaté que les statistiques de débits ont une faible corrélation avec l’écoulement superficiel et la surface des zones d’eau peu profonde dans des conditions naturelles de débit. Les résultats montrent que les statistiques hydrologiques ont une utilité limitée pour quantifier les modifications des environnements hydrauliques. Une modification de même ampleur de l’écoulement peut produire des résultats hydrauliques différents. L’influence complexe de la morphologie du canal ne permet pas aux statistiques sur le débit d’évaluer adéquatement les modifications hydrauliques. La modélisation des relations entre l’écoulement et le biote dans un contexte régional est limitée par l’incapacité des statistiques sur le débit à modéliser une hydraulique écologiquement significative. L’amélioration de la connaissance de statistiques hydrologiques d’importance éco-hydraulique permettra d’améliorer l’efficacité de la planification des débits environnementaux pour maintenir les conditions d’habitat en rivière. Une approche probabiliste est nécessaire pour permettre la généralisation sur ordinateur d’une approche fondée sur le risque des relations entre écoulement et biote.
1 INTRODUCTION
Integrating environmental flow assessment into regional water resource planning will help to sustain ecosystem function as water resource demands continue to increase. The disparity in scale of regional water resource planning and reach-scale environmental flow assessments is problematic. The development of scientifically robust regional environmental flow recommendations is hindered by a lack of clear methodology to upscale the detail required in a reach-scale environmental flow assessment to a regional context. Recently, there has been a shift in research towards the development of regional environmental flow assessment methods to try and address those challenges (Richter et al. Citation2003, Arthington et al. Citation2006, Poff et al. Citation2010).
Many environmental flow assessment methods are based on the widely accepted premise of streamflow ecology relationships (Olden and Poff Citation2003, Tharme Citation2003, Arthington et al. Citation2006). Streamflow statistics are chosen to quantify streamflow conditions that will maintain or improve ecological functions or biotic longevity within a reach. Applicable to a range of river types, streamflow statistics are often applied across a catchment to aid water resource planning in a regional context. The ecologically significant streamflow statistics quantify multiple facets of natural reference conditions (i.e. magnitude, duration and frequency indices) and the alteration of those conditions with regulation. Ecological response to streamflow alteration is considered in the setting of environmental flow recommendations, which are often set to mimic natural reference conditions (Arthington et al. Citation2006). The choice of hydrologic statistics used to quantify all facets of natural flow regime is extensive (Olden and Poff Citation2003). Yet, most streamflow ecology relationships used in environmental flow assessments are theoretical, as very few ecological responses to streamflow alteration have been quantified due to a lack of data (Poff et al. Citation2010).
One challenge of regional environmental flow planning is to develop the theoretical statistical streamflow ecology relationships. Another is transferring those relationships to systems for which they were not developed, which could cause more environmental degradation than benefit (Arthington et al. Citation2006, Poff and Zimmerman Citation2010, Poff et al. Citation2010). As Poff et al. (Citation2010) stated, it is the scientific understanding of the streamflow ecology relationship that will drive the uncertainty in environmental flow recommendations in a regional context. Uncertainty in regional streamflow ecology relationships is caused by two things: first, an inadequate understanding of the large-scale hydrological and physical features that drive ecosystem function (Thomson et al. Citation2004, Chessman et al. Citation2006, Poff et al. Citation2010); and second, incorporating the variability of large-scale physical and hydrological features into the conceptual development of streamflow ecology relationships. As such, the uncertainty in regional generalized models of streamflow ecology relationships will not be negated without a better understanding of how the streamflow ecology relationship will vary across regions on a case-by-case basis (Poff and Zimmerman Citation2010).
The regional environmental flow framework: Ecological Limits of Hydrological Alteration (ELOHA) is “grounded in the premise that increasing degrees of flow alteration from baseline condition are associated with increasing ecological change” (Poff et al. Citation2010, p. 156). Poff et al. (Citation2010) acknowledge the impact of large-scale variability of hydrological and geomorphic features on the streamflow ecology relationship. They suggest that use of the streamflow ecology relationships in similar river types might overcome this confounding effect (Poff et al. Citation2010). However, this is yet to be tested.
In an attempt to strengthen and quantify generalized models, Poff and Zimmerman (Citation2010) reviewed and analysed streamflow ecology relationships in 165 papers. The response of macroinvertebrates, fish and riparian vegetation to streamflow alteration was variable and did not produce statistically robust relationships. The authors stated that while there were similar trends in some ecological responses (e.g. fish diversity), transferable, empirical relationships that could be useful in a regional environmental flow assessment were not identified. A number of factors could have influenced the weak streamflow ecology relationships: ambiguity in reporting of flow alteration; gaps in data series; inability to model multiple streamflow indices or not including geomorphic and climate characteristics that influence the variability of streamflow regimes in a regional context (Poff and Zimmerman Citation2010). In-stream biota and ecosystem function respond to changes in local-scale hydraulic conditions and resultant habitat availability. The relationship between biota and hydraulic conditions were not considered.
The ecological importance of hydraulic conditions created by the interaction of streamflow and in-channel physical features is widely accepted (Statzner et al. Citation1988, Kemp et al. Citation2000, Lamouroux and Capra Citation2002, Lamouroux and Souchon Citation2002). Also widely accepted is the different hydraulic habitat types that can be produced by the interaction of streamflow and in-channel features in contrasting river types (Brierley et al. Citation2002, Wadeson and Rowntree Citation2002). The nonlinear relationship between hydraulic conditions and streamflow is a challenge for ecologically meaningful assessments of hydraulic habitat conditions, particularly in geomorphically variable systems (Stalnaker et al. Citation1996). This article explores the question of whether streamflow statistics are an effective measure of the ecological response to hydraulic conditions. Particularly, are the confounding effects of channel morphology significant in a regional environmental flow context? This article investigates the relationship between streamflow alteration and the alteration of hydraulic environments.
2 METHODS
2.1 Study location
This study includes 19 sites from the Goulburn (five sites), Thomson (six sites), Broken (three sites) and Loddon (five sites) rivers in Victoria, Australia (). Sites are all within a temperate climate zone with no or minimal contributions from snowmelt. Higher catchment runoff occurs during the winter period. However, all sites are located downstream of large dams operated for irrigation supply, which store wet season runoff for release in the drier months to meet irrigation demands. In addition to irrigation releases, water is diverted from the upper Thomson River for Melbourne’s urban water supply.
Fig. 1 Nineteen study reaches located in the Thomson, Broken, Goulburn and Loddon river catchments, Victoria, Australia (see for site details).
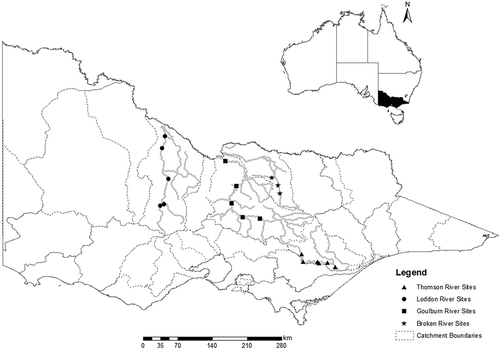
The 19 sites include samples from lowland, midland and upland river reaches (). Lowland, unconfined, sand/silt channels are features of sites in the Lower Goulburn, Loddon and Broken rivers. Generally, these reaches are dominated by pool/run habitat sequences. Midland and upland survey reaches from the Broken, Goulburn and Thomson rivers are characterized by greater in-channel morphological variability with riffles and runs interspersed by pools. Across these sites, channel gradients vary over three orders of magnitude between 0.000 05 and 0.006, bankfull channel width varies between 23 and 121 m and bankfull channel depth varies between 3.2 and 9.4 m ().
Table 1 Site characteristics: cross-sections, reach length, valley confinement and Köppen climate region.
Sites consist of a river reach (279–4200 m in length) selected specifically to represent hydraulic conditions along each river in studies of their environmental flow requirements (Cottingham et al. Citation2001, Citation2003, LREFTP Citation2002, Earthtech Citation2003). Cross-sections were surveyed by a professional surveying contractor using a total station for the use in habitat assessment for environmental flow projects in each river. Survey points are located across each cross-section to characterize the cross-section shape. This means fewer points were surveyed where there was little variation in elevation, and more points were required in areas of such banks and benches, which had greater irregularity in shape. Spacing between adjacent points is typically between 0.3 and 3 m. Cross-sections are evenly spaced to provide a systematic sample of the reach and extended for at least one meander wavelength. As a consequence, longer reaches were used in rivers with a larger meander wavelength. Between 6 and 21 channel cross-sections (spaced between 28 and 530 m apart), were surveyed along each river reach. More cross-sections are used in rivers with greater longitudinal variability in cross-section geometry to provide an adequate sample of hydraulic conditions. For example, only six cross-sections are used at Thom6, which had the lowest longitudinal variation in channel width and close to the lowest variation in channel depth across all sites.
Water surface elevation was modelled at each cross-section for a range of discharges, from low-flow to bankfull, using the one-dimensional hydraulic model HEC-RAS (Brunner Citation2001). Additional cross-sections are located at hydraulic controls where necessary for the hydraulic model, which uses a backwater calculation assuming gradually varied flow.
2.2 Analysis
The aim of this analysis is to test the strength of the statistical association between the alteration of ecologically relevant streamflow statistics and hydraulic metrics. This analysis includes three steps: first, defining biologically relevant streamflow statistics and their alteration between natural and historic regimes; second, defining metrics to quantify four instream hydraulic habitat features; and finally, applying a multivariate statistical analysis to investigate the effectiveness of streamflow statistics for representing alteration in hydraulic habitat features across our study sites (Hydraulic habitat features defined in Section 2.2.2).
2.2.1 Streamflow statistics
For each site, a daily time-series of streamflow was available representing two scenarios: (i) the observed streamflows under regulated conditions for a period of between 17 and 43 years and (ii) streamflows modelled for this same period in the absence of major water resource developments. Flow modelling to derive a natural (pre-regulation) flow series for all sites was based on a water balance calculation method with flow routing to relate streamflows at a study site to dam and unregulated tributary inflows into each system. Pre-regulation flows downstream of the dam were calculated as the sum of inflows to the dam plus tributary inflows. This calculation was carried out for each day of the historic record. In some cases where travel time was long, a time lag was required for flows to reach sites further downstream from the dam. As stated, all sites had been used previously in environmental flow investigations. Flow modelling was carried out in a consistent manner across all studies. The streamflow series length at each site varies between sites (), dependent on data availability and when the previous study was carried out. Analysis using the streamflow statistics compares at a site differences, rather than between site differences, negating analysis of uncertainty and error created by differences in streamflow series between sites.
Forty-one streamflow statistics were derived for each flow series (using the River Analysis Package (RAP) (eWater 2010; www.toolkit.net.au)) (). Full definition of each streamflow statistic can be found in Olden and Poff (Citation2003). For ease of replication, this article keeps the naming convention of streamflow statistics consistent with that of Olden and Poff (Citation2003). A logarithmic (LN) or square-root transformation was applied where needed to ensure streamflow statistics were normally distributed. A summary of the streamflow statistics name, notation and transformation is included in .
Table 2 Streamflow statistics. All statistics underwent LN transformation unless otherwise noted (SQRT: square-root transformation; UT: untransformed).
The sites included in this study are from regions of contrasting geomorphic and climate influences. The group of hydrologic statistics were chosen to (a) remove any bias in analysis created by the pre-selection of hydrologic statistics; (b) provide a set of hydrologic statistics that are transferable between sites that can quantify any unique hydrologic features, regardless of site type; and (c) provide a measure of duration, magnitude, frequency and variability in the streamflow regime of each site. All streamflow statistics have been noted in previous studies for their ecological influence (Olden and Poff Citation2003). Streamflow statistics were calculated for both time series at each site (modelled pre-regulation and observed regulated scenarios). Measures of change in flow regime were calculated for each site by subtracting these transformed statistics for each of the two scenarios (regulated minus pre-regulation scenarios).
2.2.2 Quantifying hydraulic features
This article uses reach-scale hydraulic metrics to quantify ecologically significant features of hydraulic habitats. In this case, a reach refers to a river length of between one and three meander wavelengths. Each hydraulic metric chosen for analysis has a known influence on or association with aquatic species as an individual or at a community level. The metrics (abbreviation and flow used in calculations) are Froude number (Fr, calculated at the minimum median monthly flow); area of channel bench inundation (Bench, calculated at median summer flow); area of shallow water (Shall < 0.2 m, calculated at median summer flow); and area of deep water (Deep > 1.0 m; calculated at median 7-day minimum). The alteration of hydraulic metrics was calculated as with the alteration of the streamflow statistics.
Impacts of streamflow regulation are often seasonally specific, altering low or high streamflow regimes. Each hydraulic habitat feature used in this study was calculated for the summer or low-flow period to reflect those seasonal impacts (summer months include December, January and February). Low-flow periods are also when variability of hydraulic habitat features within a reach often peaks (Stewardson Citation2005). Many ecological studies have found a correlation between flow depth, resultant hydraulic conditions and aquatic species abundance or diversity (Stalnaker et al. Citation1996, Maddock et al. Citation2004, Brooks et al. Citation2005). Flow/hydraulic/biota relationships have formed the basis of environmental flow recommendations for many rivers based in Victoria, Australia. One example is the area and duration of habitat availability and refuge for fish species provided by a pool of deeper water within a reach during a low-flow period. A minimum pool depth of >1 m during a low-flow period was recommended to provide refuge for fish species from prey in the Latrobe River (Earthtech Citation2007). A short period of loss of more than a week would reduce that protection. Another example is the 0.2 m shallow water threshold recommended during low-flow periods in the Latrobe and Goulburn rivers to maintain reach connectivity and protect fish passage throughout each system (Cottingham et al. Citation2003, Earthtech Citation2007). The flow statistics chosen for the calculation of the hydraulic metrics in this study are not species specific. However, they provide a range of ecologically significant hydraulic features that are not unrealistic and widely applicable.
Froude number
The Froude number, Fr, is the ratio of kinetic to gravitational forces, that is the ratio of velocity (v) to depth (D) (Fr = v/√gD) (French Citation1994), where g is acceleration due to gravity (m s-2) and D is the mean water depth at the cross-section (m). In this study, reach-scale Froude number is the average of all cross-sectional Fr values calculated as U/√(gD), where U is the cross-sectional velocity (m s-1). Mean velocities can produce different turbulence and drag force along the longitudinal profile of depth and substrate variability (Statzner et al. Citation1988, Vogel Citation1994). Use of the dimensionless Froude number moves away from issues of variability in the velocity and depth profiles and also avoids problems of contrasting scale (Wadeson Citation1994, Lamouroux and Souchon Citation2002, Wadeson and Rowntree Citation2002). Doubts over the usefulness of Fr as a habitat assessment indicator have been previously raised, as different velocity and depth characteristics can produce a similar Fr (Clifford et al. Citation2006). However, the correlation of Fr to dominant habitat features (e.g. pools and riffles) at the meso-scale (Jowett Citation1993, Wadeson and Rowntree Citation2002), across a cross-section (Zavadil et al. Citation2012), and along a reach (Turner et al. Citation2005, Lamouroux and Cattanéo Citation2006) has demonstrated its sensitivity to the interaction of streamflow and channel form. The ecological significance of Fr is indicated by an ability to distinguish between habitat types (Jowett Citation1993, Lamouroux and Capra Citation2002). Reach-scale habitat models have also correlated Fr to habitat values for fish species and community structure between reaches (Lamouroux and Capra Citation2002, Lamouroux and Souchon Citation2002). The sensitivity of Fr to dominant habitat features within a reach provides an ecologically significant indicator that is applicable in multiple reaches of contrasting geomorphic form.
Area of bench inundation
The area of bench inundation (Bench) is defined in this study as the area of in-channel benches inundated by streamflow. An in-channel bench is defined as a bank-attached, fine-grained sediment deposit with a lateral gradient of less than 10% (Vietz et al. Citation2006). Created in slackwater areas, they are a prominent feature of systems characterized by a variable flow regime (Thoms et al. Citation2006, Vietz et al. Citation2006). As such, the inundation of in-channel benches plays an important role in a number of ecosystem functions. It is critical for suspended sediment deposition and entrainment (Woodyer Citation1968); creating aquatic habitat refuges from high velocities during high flows (Thoms and Sheldon Citation2000, Maddock et al. Citation2004, Vietz et al. Citation2013) and aiding the distribution of organic material and, hence, aquatic species to and from in-channel benches (Thoms et al. Citation1999). Area, depth and velocity of slackwaters have been associated with instream biota abundance and diversity (Koehn et al. Citation1994, Richardson et al. Citation2004, Humphries et al. Citation2006, Vietz et al. Citation2013). A reduction in the area of slackwater availability is a common impact of streamflow regulation (Humphries et al. Citation2002, Richardson et al. Citation2004). This has resulted in a decrease in macroinvertebrate and fish populations, refuge habitat and the retention of organic matter (Humphries et al. Citation2002, Vietz et al. Citation2013). As such, the maintenance of in-channel benches to preserve ecosystem function has been integrated into a number of environmental flow assessments in recent years (Vietz et al. Citation2006).
Area of shallow water
Here, the area of shallow water (Shall) is defined as the area of water within a reach with a depth of less than 0.2 m. Hydraulic diversity within a geomorphically diverse or uniform reach peaks during low-flow periods (Stewardson Citation2005). Consequently, a small change in streamflow can alter the diversity and variability of hydraulic features within a reach (Turner et al. Citation2005, Dyer and Thoms Citation2006, Hauer et al. Citation2011, Zavadil et al. Citation2012). The unique velocity and turbulence hydraulic features created by the interaction of shallow flows with in-channel features influence many ecosystem functions, such as community structure of benthic invertebrates (Beisel et al. Citation1998), biotic diversity (Dyer and Thoms Citation2006), macroinvertebrate distribution within a reach (Quinn and Hickey Citation1994) and the maintenance of connectivity within and between reaches. The area of shallow water is included in this study to quantify the area of low-flow hydraulic features within a reach. Many studies have quantified the alteration of low-flow variability and magnitude to assess the impact of regulation on ecosystem function (Olden and Poff Citation2003). This study investigates if streamflow statistics can quantify the alteration of the area of shallow water at low flow altered with streamflow.
Area of deep water
Deep water area (Deep) was defined by a depth greater than 1 m. Deep water within a reach is defined most often as a pool with no perceptible flow (Wadeson and Rowntree Citation2002). The area of deep water within a reach plays an important role in a number of ecosystem functions. For example, it influences fish species assemblages (Gorman and Karr Citation1978, Lamouroux and Cattanéo Citation2006) and the community structure of aquatic species (Lamouroux and Capra Citation2002), allows settling of organic matter that provides food sources for macroinvertebrates and in turn fish species (Gosselin et al. Citation2010) and, in combination with the hydraulic diversity of shallower habitats, influences the distribution of macroinvertebrates and migration of fish species throughout a river system (Stalnaker et al. Citation1996). As such, the ecological significance of the area of deep water and its contribution to habitat suitability within a reach is widely accepted. The alteration of the area of deep water by streamflow within a reach has been quantified in many studies using hydraulic habitat modelling tools such as PHABSIM (e.g. Maddock et al. Citation2004). Yet, its correlation with ecologically significant streamflow indicators is rarely tested.
2.2.3 Statistical analysis
Principal component analysis (PCA) was used to address correlation between streamflow statistics and remove redundancy. The PCA provided a statistical summary of streamflow statistics that characterize each site for further analysis (e.g. Olden and Poff Citation2003). The PCA was carried out on pre-regulation flow statistics and differences between regulated and pre-regulation statistics (natural and alteration). A varimax rotation allows easier interpretation of components (Afifi and Clark Citation1996). The resulting component matrix was used to identify streamflow statistics with the highest Eigenvalue for each component. These were used to investigate the association between streamflow alteration and the alteration of hydraulic features using a Multiple Stepwise Regression analysis (SPSS).
3 RESULTS
3.1 Redundancy in streamflow statistics
Five principal components were identified that explained 85% and 82% of variance in the pre-regulation and flow alteration statistics, respectively. In both cases, the first two components explained over 50% of variance in streamflow statistics (). This included mean maximum monthly (January) (MH2) and variability of monthly flows (July) (MA30) for the natural data group and mean maximum monthly (November) and the spread of daily flows for the alteration data group ().
Table 3 Principal component analysis.
The remaining three components of each streamflow group explained between 22% (natural) and 25% (alteration) of variance in the data groups. This includes streamflow statistics quantifying mean maximum monthly flows (August) (MH8) (natural and alteration), median of annual minimum flows (natural) (ML16), coefficient of variation of monthly flows (April) (natural) (MA27), variability of annual minima (1 day and 7 day) (alteration) (DL10) (). The five principal components (for natural and alteration groups) were used in two multiple stepwise regression analyses that investigated the relationship between streamflow statistics and the hydraulic habitat features under (i) natural and (ii) altered streamflow regimes.
3.2 Hydraulic characteristics of reaches
Each reach has a unique functional relationship between the hydraulic variables and streamflow, which we modelled using HEC-RAS ( and ). Site-to-site variations in channel morphology, channel gradient and flow resistance will produce variation in these functional relations. There is no apparent consistency in these functions of discharge (standardized as percentage of the mean pre-regulation discharge) between reaches within the same river () or for upland, midland or lowland river types ().
Fig. 2 Area of bench inundation, Froude number, area of shallow water and area of deep water as a function of discharge in each survey reach of the Broken, Thomson, Loddon and Goulburn river systems.
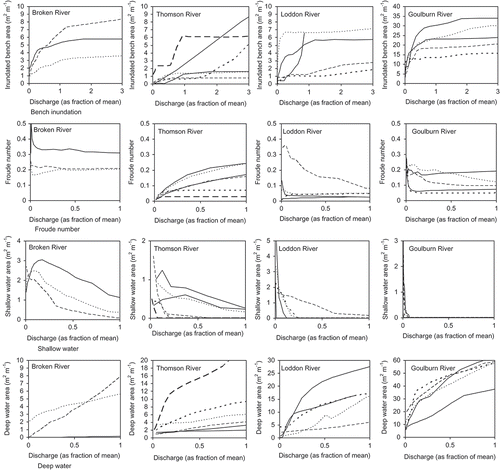
Fig. 3 Area of bench inundation, Froude number, area of shallow water and area of deep water as a function of discharge in each survey reach for lowland, midland and upland rivers.
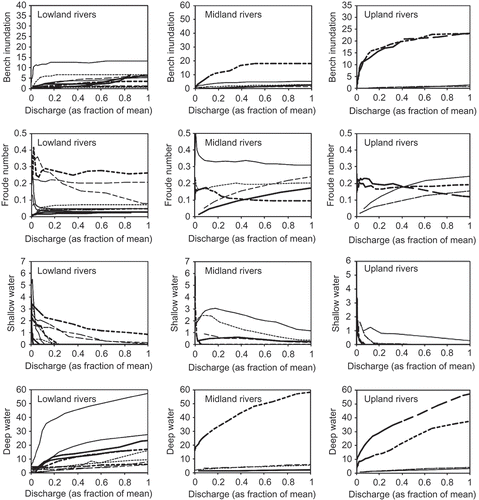
The Fr remains unchanged in at least two reaches of each river system when the magnitude of flow reaches 0.5 times the mean streamflow (). In lowland reaches such as those included in this study, this indicates that the surface flow conditions and, therefore, the influence of channel morphology on the proportion of each hydraulic habitat within the reach (e.g. pool, riffle or runs) remain unchanged after a certain streamflow magnitude has been reached. However, the sensitivity of Fr to the influence of channel morphology is evident in the remaining reaches (). The relationship between Fr and channel morphology is dynamic, altered continuously by streamflow.
The intrinsic contrast in the alteration of the area of shallow (Shall) and deep water (Deep) in each reach is evident (). The alteration of Shall and Deep with increasing discharge will be dependent on channel complexity and geomorphic composition within a reach. Variability in the area of shallow water with discharge is most evident in the Thomson and Broken river reaches. Sharp spikes in Shall in some reaches of these systems could indicate multiple pools across the channel (). As each pool fills, the collective inundation area of the pools will be greater than the area of the largest pool alone, which would be inundated last. Similarly to Fr, the area of shallow water within a reach will be sensitive to the interaction of streamflow with in-channel features. Also similarly to Fr, it would be difficult to predict how an alteration to a streamflow regime will impact the area of shallow water without in-depth knowledge of the geomorphic variability within each reach. This difficulty is magnified in a regional context.
The contrast in the size of the reaches is also evident, with the Deep threshold used in analysis (1 m), not recorded in one reach of Broken River until streamflow magnitude reached 0.4 times the mean (). The larger Deep recorded in Broken River was one of the smallest areas of deep water recorded in Goulburn River ().
3.3 Association between natural streamflow statistics and hydraulic features
Multiple stepwise regression analysis identified a correlation between streamflow statistics and hydraulic features under natural streamflow conditions. However, the strength of this association varies considerably between hydraulic features. The calculated and modelled hydraulic features under natural streamflow conditions are shown in . Natural streamflow statistics were strongly correlated to Deep and Bench within a reach (R2 = 0.82 and 0.69, respectively, ). Mean monthly flow (January) was a main predictor of both hydraulic habitat features. Mean maximum flows (January) and the median of minimum annual flows were also identified in the regression model for the area of deep water in the 19 survey reaches of this study ().
Fig. 4 Regression models for modelled vs calculated hydraulic features: (a) natural streamflow conditions and (b) alteration in streamflow statistics and hydraulic features. Symbols: × upland, ◊ midland and ☐ lowland sites.
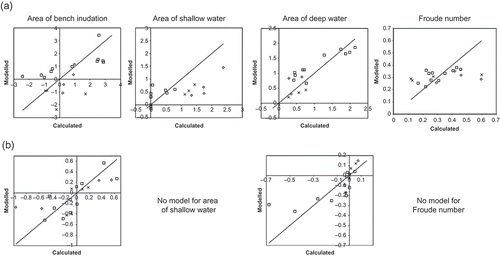
Table 4 Regression models, adjusted R2 and standard error of estimates for each hydraulic metric in the ‘natural’ and ‘alteration’ data sets.
The association of streamflow statistics to Fr and Shall is weak under natural streamflow conditions (; R2 = 0.23 and 0.21, respectively). Variability of mean monthly flows (April) and the mean monthly flow (July) were identified in the regression model for Fr and Shall, respectively (). The scatter in the relationship is considerable (). Depth of streamflow does influence surface flow conditions and was strongly correlated to the streamflow statistics. However, it is evident that streamflow statistics alone cannot predict ecologically significant hydraulic features such as the turbulence of surface flow conditions, which often distinguish mesohabitat types, created by the interaction of streamflow and in-channel features (Wadeson and Rowntree Citation2002). There is no evidence of bias in results across lowland, midland and upland sites ().
3.4 Association between alteration of streamflow statistics and hydraulic features
The alteration of streamflow statistics had minimal association with the alteration of hydraulic features across the 19 survey reaches in this study. The calculated and model altered hydraulic features are shown in . Multiple stepwise regression analysis did not identify a model for the correlation between the alteration of streamflow statistics and alteration of Froude number or the alteration of the area of shallow water within a reach ().
The alteration of the area of deep water and the area of bench inundation within a reach was correlated with the alteration of streamflow statistics (including the spread of daily flows, mean maximum monthly flows (November) and the variability annual minima flows). However, this correlation is weak (adjusted R2 = 0.53 and 0.50, respectively; ).
4 DISCUSSION
The results of this study show that streamflow statistics are a poor surrogate for ecologically significant reach-scale hydraulic metrics, particularly when examining flow alteration. Variation in some hydraulic variables may be weakly correlated with altered flow statistics, but others show little correlation in comparisons across sites. The association between streamflow alteration and the alteration of hydraulic features will be confounded by channel morphology, which will produce a nonlinear and variable relation between streamflow and hydraulic environments. Variability in the intrinsically nonlinear relationship between streamflow and hydraulic features will be exacerbated across multiple sites in a regional context. This article demonstrates the importance of between-reach variation in the nonlinear relationship between streamflow and hydraulic features for understanding the flow sensitivity of the hydraulic environment of streams.
Streamflow measurements are widely available and models of stream discharge in response to altered water management settings are in common use. The availability of observed or modelled streamflow data from multiple sites in most developed countries partly explains its wide use to characterize the flow environment of streams in freshwater ecological investigations. We deal with three types of studies in this discussion: (i) studies that attempt to explain site-to-site variation in ecological characteristics of sites (e.g. Arthington et al. Citation2006); (ii) studies that attempt to generalize ecological responses to variations in streamflow across multiple sites (e.g. Poff and Zimmerman Citation2010); and (iii) studies that use streamflow statistics as a surrogate measure for more direct ecological measures in planning environmental water requirements (e.g. Poff et al. Citation2010).
In the first type of study, those that explain the site-to-site variation in ecological responses using streamflow statistics, the degree of streamflow alteration is often taken as an indicator of the level of risk to ecosystem function (Arthington et al. Citation2006). The variation evident in nonlinear hydraulic–streamflow relationships within and between sites in this study shows that linearity in an ecological response to streamflow alteration would be minimal. A comparison of the range or gradient of streamflow alteration from natural to regulated conditions has been proposed in some studies to provide acceptable thresholds of streamflow alteration for ecosystem function (Arthington et al. Citation2006). However, the hydraulic feature–streamflow response curves show that a discharge can produce a range of hydraulic features. This would impact on the diversity and variability of habitat types within a reach.
In the second type of study, those that attempt to generalize ecological responses to variation in streamflow (e.g. Poff and Zimmerman Citation2010), the variation in hydraulic responses confounded by channel morphology across multiple sites would cause a large degree of uncertainty in results. It is suggested that attempts to model flow–biota relations should first consider the adequacy of streamflow as a surrogate for the proximate hydraulic conditions that produce biotic responses. While it is argued that this would not reduce uncertainty in flow–biota relations in its entirety, it would help to provide a foundation on which to base generalized ecological responses. The inadequacy of streamflow statistics as a surrogate measure of hydraulic environment is likely to be an important reason for limited success in identifying general relations between flow and biotic responses (e.g. Poff and Zimmerman Citation2010).
In the third type of study, the use of streamflow statistics as a surrogate measure for more direct ecological measures is flawed. This is shown in this study by the weak relationship between the alteration of streamflow statistics and hydraulic features that drive many ecosystem functions. It has been suggested that flow–biota relations may be more consistent across streams with similar geometries, perhaps identified using geomorphic river classification (as proposed by Poff et al. Citation2010). However, there is significant variability in channel geometry and hence hydraulic conditions across rivers of the same geomorphic type (Turner Citation2009). This study provides no evidence that restricting sites to one of lowland, midland or upland regions would produce a significant improvement in correlations between hydrological and hydraulic variables. Although other site classification may be considered, it would seem unlikely that site classification based on geomorphic characteristics will improve the utility of hydrological metrics to act as surrogates for hydraulic variables. Therefore, including a river classification in a regional desktop method may not provide a full solution to strengthening the flow–biota relationships.
There is a global move to develop rapid desktop approaches for establishing environmental flow requirements using streamflow data (e.g. Poff et al. Citation2010). This is a desirable objective because the cost, time and expertise to undertake detailed environmental flow studies are prohibitive in most situations and especially in developing countries. Some methods are now well-established and justified on the basis that they can provide a robust tool to establish conservative environmental flow targets, which maintain streamflow components within their natural range of variability (Richter et al. Citation1998). However, more detailed analysis is advisable when pressures to develop rivers produce levels of flow alteration that exceed conservative levels. In such cases, environmental flow planning should allow for the confounding influence of channel morphology in establishing the ecological risk associated with flow alterations. We can see from this study that the same level of flow alteration can produce quite different changes in hydraulic environments. This is likely to produce similar or even greater variability in biotic responses as a result of other factors controlling biological communities.
One other option is to integrate hydraulic metrics into regional environmental flow assessment methods to help quantify the ecological response to streamflow alteration. Some recent studies have focused on the effectiveness of generalized models of reach-scale hydraulic features (Lamouroux and Capra Citation2002, Turner et al. Citation2005, Lamouroux and Cattanéo Citation2006). The transferability and effectiveness of the dimensionless hydraulic metrics to assess ecological response in a regional context has been proven (Lamouroux and Jowett Citation2005). Reach-scale hydraulic metrics provide an avenue to quantify ecological responses to streamflow alteration in a regional context, particularly, quantifying and providing cues for the maintenance of the streamflow habitat availability relationship in a regional context. The limited utility of hydrologic statistics to quantify changes in hydraulic environments has been shown in this study. The integration of hydraulic metrics or improving knowledge of ecohydraulically significant hydrologic statistics will decrease uncertainty in regional environmental flow planning, in turn, improving its effectiveness to sustain instream habitat conditions.
REFERENCES
- Afifi, A.A. and Clark, V., 1996. Computer-aided multivariate analysis. London: Chapman and Hall.
- Arthington, A.H., et al., 2006. The challenge of providing environmental flow rules to sustain river ecosystems. Ecological Applications, 16 (4), 1311–1318. doi:10.1890/1051-0761(2006)016[1311:TCOPEF]2.0.CO;2.
- Beisel, J.-N., et al., 1998. Stream community structure in relation to spatial variation: the influence of mesohabitat characteristics. Hydrobiologia, 389, 73–88. doi:10.1023/A:1003519429979.
- Brierley, G., et al., 2002. Application of the River Styles framework as a basis for river management in New South Wales, Australia. Applied Geography, 22, 91–122. doi:10.1016/S0143-6228(01)00016-9.
- Brooks, A.J., et al., 2005. Hydraulic microhabitats and the distribution of macroinvertebrate assemblages in riffles. Freshwater Biology, 50, 331–344. doi:10.1111/j.1365-2427.2004.01322.x.
- Brunner, G.W., 2001. HEC-RAS river analysis system: hydraulic reference manual. Davis, CA: US Army Corps of Engineers.
- Chessman, B.C., Fryirs, K.A., and Brierley, G.J., 2006. Linking geomorphic character, behaviour and condition to fluvial biodiversity: implications for river management. Aquatic Conservation: Marine and Freshwater Ecosystems, 16, 267–288. doi:10.1002/aqc.724.
- Clifford, N.J., et al., 2006. Physical habitat, eco-hydraulics and river design: a review and re-evaluation of some popular concepts and methods. Aquatic Conservation: Marine and Freshwater Ecosystems, 16, 389–408. doi:10.1002/aqc.736.
- Cottingham, P., et al., 2001. Report of the Broken River scientific panel on the environmental condition and flows of the Broken River and Broken Creek. Canberra, ACT: CRC for Freshwater Ecology, University of Canberra.
- Cottingham, P., et al., 2003. Flow-related environmental issues related to the Goulburn River below Lake Eildon. A report to the Department of Sustainability and Environment, Victoria and the Murray Darling Basin Commission. Canberra, ACT: CRC for Catchment Hydrology and CRC for Freshwater Ecology.
- Dyer, F. and Thoms, M.C., 2006. Managing river flows for hydraulic diversity: an example of an upland regulated gravel-bed river. River Research and Applications, 22, 257–267. doi:10.1002/rra.909.
- Earthtech, 2003. Thomson River environmental water requirements and options to manage flow stress: Part B—Draft Final Recommendations. Unpublished Report to the West Gippsland Catchment Management Authority by the Thomson River Environmental Flows Technical Panel.
- Earthtech, 2007. Assessment of environmental flow requirements for the Latrobe River. Amended Final Recommendations Report. Unpublished report by Earthtec for the West Gippsland Catchment Management Authority, March 2007.
- French, R., 1994. Development of uniform flow concepts, open channel hydraulics. New York: McGraw-Hill.
- Gorman, O.T. and Karr, J.R., 1978. Habitat structure and stream fish communities. Ecology, 59 (3), 507–515. doi:10.2307/1936581
- Gosselin, M.P., Petts, G.E., and Maddock, I.P., 2010. Mesohabitat use by bullhead (Cottus gobio). Hydrobiologia, 652, 299–310. doi:10.1007/s10750-010-0363-z.
- Hauer, C., et al., 2011. Variability of mesohabitat characteristics in riffle-pool reaches: testing an integrative evaluation concept (FGC) for mem-application. River Research and Applications, 27, 403–430. doi:10.1002/rra.1357.
- Humphries, P., Serafini, L.G., and King, A.J., 2002. River regulation and fish larvae: variation through space and time. Freshwater Biology, 47, 1307–1331. doi:10.1046/j.1365-2427.2002.00871.x.
- Humphries, P., et al., 2006. Creating a disturbance: manipulating slackwaters in a lowland river. River Research and Applications, 22 (5), 525–542. doi:10.1002/rra.920.
- Jowett, I.G., 1993. A method for objectively identifying pool, run, and riffle habitats from physical measurements. New Zealand Journal of Marine and Freshwater Research, 27, 241–248. doi:10.1080/00288330.1993.9516563.
- Kemp, J.L., Harper, D.M., and Crosa, G.A., 2000. The habitat-scale ecohydraulics of rivers. Ecological Engineering, 16, 17–29. doi:10.1016/S0925-8574(00)00073-2.
- Koehn, J., O’Connor, N.A., and Jackson, P.D., 1994. Seasonal and size-related variation in microhabitat use by a southern Victorian stream fish assemblage. Australian Journal of Marine and Freshwater Research, 45, 1353–1366. doi:10.1071/MF9941353.
- Lamouroux, N. and Capra, H., 2002. Simple predictions of instream habitat model outputs for target fish populations. Freshwater Biology, 47 (8), 1543–1556. doi:10.1046/j.1365-2427.2002.00879.x.
- Lamouroux, N. and Cattanéo, F., 2006. Fish assemblages and stream hydraulics: consistent relations across spatial scales and regions. River Research and Applications, 22, 727–737. doi:10.1002/rra.931.
- Lamouroux, N. and Jowett, I.G., 2005. Generalized instream habitat models. Canadian Journal of Fisheries and Aquatic Sciences, 62 (1), 7–14. doi:10.1139/f04-163.
- Lamouroux, N. and Souchon, Y., 2002. Simple predictions of instream habitat model outputs for fish habitat guilds in large streams. Freshwater Biology, 47 (8), 1531–1542. doi:10.1046/j.1365-2427.2002.00880.x.
- LREFTP, 2002. Environmental flow determination of the Loddon River catchment. Final Report. Unpublished Report prepared by the Loddon River Environmental Flows scientific panel to the North Central Catchment Management Authority and Department of Natural Resources and Environment.
- Maddock, I., et al., 2004. Identifying the influence of channel morphology on physical habitat availability for native fish: application to the two-spined blackfish (Gadopsis bispinosus) in the Cotter River, Australia. Marine and Freshwater Research, 55, 173–184. doi:10.1071/MF03114.
- Olden, J.D. and Poff, N.L., 2003. Redundancy and the choice of hydrologic indices for characterizing streamflow regimes. River Research and Applications, 19 (2), 101–121. doi:10.1002/rra.700.
- Peel, M.C., Finlayson, B.L., and McMahon, T., 2007. Updated world map of the Köppen-Geiger climate classification. Hydrology and Earth System Sciences, 11, 1633–1644. doi:10.5194/hess-11-1633-2007.
- Poff, L.N. and Zimmerman, J.K.H., 2010. Ecological responses to altered flow regimes: a literature review to inform the science and management of environmental flows. Freshwater Biology, 55, 195–205.
- Poff, N.L., et al., 2010. The ecological limits of hydrologic alteration (ELOHA): a new framework for developing regional environmental flow standards. Freshwater Biology, 55, 147–170. doi:10.1111/j.1365-2427.2009.02204.x.
- Quinn, J.M. and Hickey, C.W., 1994. Hydraulic parameters and benthic invertebrate distributions in two gravel-bed New Zealand rivers. Freshwater Biology, 32, 489–500.
- Richardson, A.J., Growns, J.E., and Cook, R.A., 2004. Distribution and life history of caridean shrimps in regulated lowland rivers in Southern Australia. Marine and Freshwater Research, 55, 295–308. doi:10.1071/MF03126.
- Richter, B.D., et al., 1998. A spatial assessment of hydrologic alteration within a river network. Regulated Rivers: Research and Management, 14, 329–340. doi:10.1002/(SICI)1099-1646(199807/08)14:4<329::AID-RRR505>3.0.CO;2-E.
- Richter, B.D., et al., 2003. Ecologically sustainable water management: managing river flows for ecological integrity. Ecological Applications, 13 (1), 206–224. doi:10.1890/1051-0761(2003)013[0206:ESWMMR]2.0.CO;2.
- Stalnaker, C.B., Bovee, K.D., and Waddle, T.J., 1996. Importance of the temporal aspects of habitat hydraulics to fish population studies. Regulated Rivers: Research and Management, 12, 145–153. doi:10.1002/(SICI)1099-1646(199603)12:2/3<145::AID-RRR386>3.0.CO;2-H.
- Statzner, B., Gore, J.A., and Resh, V.H., 1988. Hydraulic stream ecology: observed patterns and potential applications. Journal of the North American Benthological Society, 7 (4), 307–360. doi:10.2307/1467296.
- Stewardson, M.J., 2005. Hydraulic geometry of stream reaches. Journal of Hydrology, 306 (1–4), 97–111.
- Tharme, R.E., 2003. A global perspective on environmental flow assessment: emerging trends in the development and application of environmental flow methodologies for rivers. River Research and Applications, 19, 397–441. doi:10.1002/rra.736.
- Thoms, M.C., Beyer, P.J., and Rogers, K.H., 2006. Variability, complexity and diversity: the geomorphology of river ecosystems in dryland regions. In: R.T. Kingsford, eds. The ecology of desert rivers. Cambridge: Cambridge University Press, 47–75.
- Thoms, M.C., Ogden, R.W., and Reid, M.A., 1999. Establishing the condition of lowland floodplain rivers: a palaeo-ecological approach. Freshwater Biology, 41, 407–423. doi:10.1046/j.1365-2427.1999.00439.x.
- Thoms, M.C. and Sheldon, F., 2000. Lowland rivers: an Australian introduction. Regulated Rivers: Research and Management, 16, 375–383. doi:10.1002/1099-1646(200009/10)16:5<375::AID-RRR591>3.0.CO;2-#.
- Thomson, J.R., Taylor, M.P., and Brierley, G.J., 2004. Are river styles ecologically meaningful? A test of the ecological significance of a geomorphic river characterization scheme. Aquatic Conservation: Marine and Freshwater Ecosystems, 14, 25–48. doi:10.1002/aqc.585.
- Turner, M., 2009. Natural spatial and temporal hydraulic habtiat variability; Implications for regional environmental flow planning. Thesis (PhD). Department of Civil Engineering, Monash University.
- Turner, M., et al., 2005. Using reach-scale hydraulic parameters to assess in-stream heterogeneity for environmental flow needs. 29th Hydrology and Water Resources Symposium, 20–23 February, Canberra, Engineers Australia.
- Vietz, G.J., Sammonds, M.J., and Stewardson, M.J., 2013. Impacts of flow regulation on slackwaters in river channels. Water Resources Research, 49, 1797–1811. doi:10.1002/wrcr.20094.
- Vietz, G.J., Stewardson, M.J., and Finlayson, B.L., 2006. Flows that form: the hydromorphology of concave-bank bench formation in the Ovens River, Australia. In: J.S. Rowan, et al., eds. Sediment dynamics and the hydromorphology of fluvial systems. Wallingford, UK: IAHS Press, IAHS Publ. 306, 267–276.
- Vogel, S., 1994. Life in moving fluids: The physical biology of flow. Princeton University Press.
- Wadeson, R.A., 1994. A geomorphological approach to the identification and classification of instream flow environments. Southern African Journal of Aquatic Sciences, 20 (1–2), 38–61. doi:10.1080/10183469.1994.9631349.
- Wadeson, R.A. and Rowntree, K.M., 2002. Mapping hydraulic biotopes for ecological flow assessments. ENVIRO FLOWS. 2002. In: Proceedings of the international conference on environmental flows for river systems (incorporating the 4th international ecohydraulics symposium), 3–8 March. Cape Town: Unpublished Proceedings.
- Woodyer, K.D., 1968. Bankfull frequency in rivers. Journal of Hydrology, 6, 114–142. doi:10.1016/0022-1694(68)90155-8.
- Zavadil, E., et al., 2012. An evaluation of surface flow types as a rapid measure of channel morphology for the geomorphic component of river condition assessments. Geomorphology, 139–140, 303–312. doi:10.1016/j.geomorph.2011.10.034.