Abstract
The Institute of Radioprotection and Nuclear Safety (IRSN in French) is conducting research on the impact of a fire on the behaviour of containment devices such as high efficiency particulate air (HEPA) pleated filters for radioactive materials. This work aims to study the clogging of HEPA filters in case of fire involving realistic materials (polymers making up gloves boxes, waste treatment solvent, hydraulic oil, solid material mixtures making up a trash bin, electrical cables, and cabinets) used in nuclear facilities, from the medium to large scale. The clogging kinetics of industrial pleated HEPA filters is monitored by measuring the pressure drop of the filters and the filtered air temperature at a given filtration velocity (from 0.23 to 2.1 cm/s). Upstream HEPA filters, combustion aerosols are characterized in terms of size distribution, mass concentration, composition, and particle morphology using, respectively, a DMS500 (CambustionLTD), glass fiber filter sampling, and transmission electron microscope analysis of particles deposited on TEM grids. Particles emitted denote well-known fractal morphology, are composed of carbonaceous primary particles with diameters ranging from 31 nm to 48 nm and showing an high clogging efficiency. An empirical relationship has been successfully applied to the obtained results for a larger range of fuels, filtration velocities and fire conditions.
Finally, experiments have been performed on a large-scale facility, using full-scale fire scenarios (electrical cabinet, constant, and variable filtration velocity) and a reasonable agreement was observed with our empirical relationship. At this scale, particles appear to be compact, with a complex composition and diameters close to 220 nm with a lower clogging efficiency.
Copyright 2014 American Association for Aerosol Research
INTRODUCTION
Among the accidents that may occur in a nuclear facility, fire is one of the major risks. It can damage essential safety elements and can lead to the release of radioactive materials in the facility. In such situations, knowledge of the physical characteristics of aerosols emitted is essential for the understanding of their impact on containment devices or to predict transport of the aerosols in the facility until their potential release into the atmosphere.
The aim of this study is to produce experimental data on the clogging of HEPA pleated filters in fire conditions and to extend the field of application of the empirical relationship proposed by Mocho and Ouf (Citation2011). It is motivated by the fact that although many research related to HEPA filter clogging has been carried out (Novick et al. Citation1992; Thomas et al. Citation2001; Del Fabbro et al. Citation2002), in most cases, it has focused essentially on flat filters and on particles with simple morphologies (spherical, cubic) under temperature and humidity conditions that unlike those in fire situations. To our knowledge, only Gregory et al. (Citation1991) and Hashimoto et al. (Citation1993) have proposed an empirical description of clogging of pleated HEPA filters in fire situations, but without clearly identifying the different influencing parameters. This is why IRSN has developed experimental facilities for acquiring the characteristic parameters of the combustion aerosol (particle size, morphology, physicochemical nature; Ouf et al. Citation2008, Citation2014), to then introduce them into an empirical correlation describing clogging at constant filtration velocity, of a specific type of pleated HEPA filter (Mocho and Ouf Citation2011). This article describes new results and proposes an application of the empirical relationship to realistic fire conditions.
The current state of knowledge does not allow us to describe the clogging of industrial HEPA pleated filters by combustion aerosols through phenomenological law. Considering first a flat filter, in the initial clogging period, aerosols are deposited inside the filtering medium as dendrites, leading to a slight increase in the pressure drop. This can be expressed, among other things, using the filtration velocity, the diameter of the filter fibers, the characteristic diameter of the particles and various constants (Novick et al. Citation1992; Thomas et al. Citation2001). A deposit (cake) rapidly appears on the surface of the filtering medium, causing a significant increase in the pressure drop of the filter. This pressure drop induced by the cake can be generally described by the Kozeny equation (Kozeny Citation1927), but it is difficult to estimate the contribution of the soot particle cake to the overall pressure drop of the filter. Furthermore, unlike the various models proposed, Del Fabbro et al. (Citation2002) revealed through experimentation a significant increase of the clogging capacity of aerosols at low filtration velocities due to a reduction in the filtration surface area of the industrial mini pleated filters. Consequently, it is difficult to control all the parameters and constants of each phenomenological clogging model, and it was decided to establish an empirical relationship in order to describe the clogging behaviour of pleated HEPA filters. This simple approach was firstly proposed by Gregory et al. (Citation1991) or Hashimoto et al. (Citation1993) and was more recently extended by Mocho and Ouf (Citation2011) in the following form:
where R and R0 represent the air flow resistances of the clogged and unused filter, respectively (kg.s−1.m−4), Mae is the mass of aerosol deposited per filter unit of area (g/m2), Dp is the characteristic diameter of the aerosol (the mean diameter of elementary particles forming the aggregates or the median surface projected equivalent diameter of the particles if they are not of the aggregate type [m]), Vf is the filtration velocity (m/s) and a, b are experimental fitting parameters of the relationship. These empirical parameters depend on the condensate content TC (%) of the deposited particles composing the cake formed on the filter surface. The air flow resistance is calculated using R = ΔP. μ0 /(μ.Qv), where ΔP represents the pressure drop across the filter (Pa), Qv is the filtration volume flow rate (m3/s), and μ, μ0 are the dynamic viscosities of the fluid (Pa.s), respectively, at its temperature close to the filter and in normal conditions (P = 1 atm, T = 0°C).
The studied filters are mini pleated HEPA filters with glass fibers having a mean diameter equal to 1 μm, from the company CAMFIL-FARR, part number 1501.37.00, with an effective filtration surface area of 6 m2 with a nominal filtration velocity of 2.1 cm/s. As the French nuclear industry only uses HEPA filters with mini-pleats (pleat height of about 20 mm, distance between two pleats about 2 mm) and corresponding to an H14 classification (EN-1822-1), the direct parameters associated with the pleating and the characteristics of the filtering medium were not studied, and this relationship is only reliable for the studied filter. Nevertheless this relationship is relevant for describing numerous fire configurations, and in this study we propose application of this empirical approach to other fuels at an analytical scale and its extension to a fire situation occurring in a full-scale test bench, recreating the conditions of a nuclear facility and its ventilation system.
EXPERIMENTAL
Combustion Test Bench
The “BANCO” facility (Clogging test bench or “Banc de Colmatage” in French, see ) features a combustion chamber (1 m3) connected to an extraction duct that divides into two heat-insulated channels equipped with a pleated HEPA filter providing for air flow rates ranging from 50 m3/h to 450 m3/h. This allows for testing of the different filtration velocities for fixed combustion conditions (identical soot characteristics). More details are given in Mocho and Ouf (2001) and Ouf et al. (Citation2008).
On the other hand, large-scale experiments have been carried out in the “DIVA” facility (Le Saux et al. Citation2005; ), consisting of 3 rooms of 120 m3 and a ventilation system. The test instrumentation includes electronic pressure sensors for measuring the filter pressure drop and a weighing machine for measuring the fuel mass loss rate. The size distribution and the mass concentration of combustion aerosols are determined using a CAMBUSTIONLTD DMS 500 size spectrometer and glass fiber filter samples. The morphology and condensate content of the aerosols are characterized by transmission electron microscopy (Ouf et al. Citation2010) and by furnace evaporation/desiccation of filters. Experiments have been performed in the “BANCO” facility for two constant filtration velocities (Vf = 0.23 cm/s and 2.1 cm/s). In the “DIVA” facility, fires on electrical cabinets were studied for constant filtration velocity Vf of 0.23 cm/s (test named PICSEL_V1) and dynamic filtration regime with Vf ranging from 1.4 cm/s to 0.14 cm/s (test EP2). For this second test, the filtration velocity was not regulated and was dependent on the pressure drop of the HEPA filter, as the flow rate of the extraction fan varies with pressure drop upstream.
FIG. 2. DIVA experimental test bench for the combustion of fuels and clogging of HEPA filters on a large scale (courtesy of IRSN/PSN-RES/SA2I/LEF).
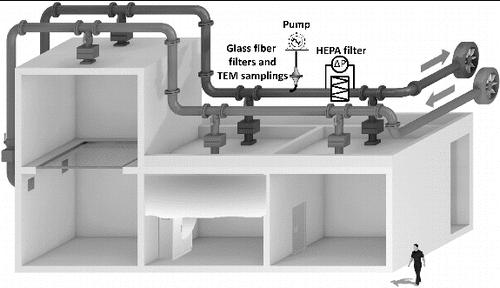
Experimental conditions will be characterized by the filtration velocity Vf, which is a key parameter for describing HEPA filter clogging and pressure drop, in addition to the air change rate in the facility (expressed in h−1), which corresponds to the ratio of the ventilation flow rate to the volume of the ventilated area. One must note that for “BANCO,” this air change rate ranges from 50 h−1 to 450 h−1 for the respective filtration velocities of 0.23 cm/s and 2.1 cm/s, while for the “DIVA” facility, the air change rate is significantly lower: It is equal to 2.5 h−1 for the PICSEL_V1 test (Vf = 0.23 cm/s) and ranges from 2.5 h−1 to 0.25 h−1 for the EP2 test (Vf = 1.4 cm/s to 0.14 cm/s). Filter clogging is characterized by the change in its airflow resistance R as a function of the deposited weight of aerosols per filter unit of area Mae.
Sample Properties
The fuels studied in the “BANCO” facility are materials commonly used in the French nuclear industry: pellets of polycarbonate (LEXAN), polymer sheet making up the radiological barrier of glove boxes (Kiowaglass; polymethyl methacrylate PMMA doped with 30% lead), electrical cables (halogen cables: B and C and non-halogen cable: A), lubricant oil (Equivis Oil), solution of hydrogenated tetrapropylen (TPH) and tributyl phosphate (TBP) used for nuclear waste retreatment and mixture of waste materials assumed to be representative of a trash bin generally produced in a nuclear industry laboratory. For the tests conducted in the “DIVA” facility, electrical cabinets similar to those used in the French nuclear industry have been considered as fuels and are composed of several electrical elements (junction block, breaker, contact block, relay, cables and PVC trunking). presents the characteristics of materials used in fire experiments at different scales.
Table 1 Properties of the studied fuels
RESULTS AND DISCUSSION
Aerosol Properties
The properties of particles useful for understanding the clogging empirical relationship developed by Mocho and Ouf (Citation2011) are summarized in for each fuel and filtration velocity. Examples of TEM micrographs are also presented in . According to these TEM images, we were able to measure the diameter of the primary particles composing the nanoparticle aggregates emitted during the combustion process. In the case of more compact particles, the equivalent surface projected diameter has been also computed on TEM images. The emission factor (mass of particles produced per mass of burnt fuel) is defined according to the following equation: ηp = Qp/Qf, where Qp is the mass flow rate of soot particles (computed according to the mass of particles sampled on the glass fiber filters and a reliable control of sampling flow rate and time duration), while Qf is the fuel mass loss rate.
Table 2 Physicochemical properties of emitted particles
For “BANCO,” in most cases, the observed particles have well-known fractal morphology (Ouf et al. Citation2008; Mocho and Ouf Citation2011; Ouf et al. Citation2014) with an organic/inorganic coating depending on the fuel composition. Hence, for these particles, the representative diameter for understanding filter clogging is the primary particle diameter Dpp ranging from 25 nm to 51 nm, in agreement with models developed for plane filters by previous authors (Kim et al. Citation2009). One must notice that this diameter has been measured on TEM micrograph and is similar to an equivalent surface projected diameter for these types of spherical primary particles. The TC content is determined by weighing the filter samples before and after thermal desorption at 130°C; this method is relevant for determining the quantity of water and volatile compounds at 130°C.
For the experiments performed on “DIVA,” the morphology of the particles is extremely compact, mainly due to specific combustion conditions and long residence time of these particles in the fire room, enhancing their ageing through physicochemical processes. Then, for these particles, the representative diameter for filter clogging is their equivalent surface projected diameter Des, which is close to 220 nm since these particles are no more composed of primary particles.
Dealing with the most relevant diameter for predicting clogging of HEPA filters for different fire scenarios, further details could be retrieved in Mocho and Ouf (Citation2011).
Comparison of Clogging Efficiencies
presents the evolution of the dimensionless filter resistance R/R0 to air flow as a function of deposited mass of aerosol per surface area of filter (measured according to several isokinetic samplings of emitted particles on 47 mm glass fibers filters conducted during each experiments). We observe higher dimensionless resistance of the filter when filtration velocity decreases (from 2.1 cm/s to 0.23 cm/s), for the same mass of deposited aerosol. This has been also observed by Mocho and Ouf (Citation2011). This faster clogging is mainly due to the surface reduction regime, which is more rapidly achieved for the lowest filtration velocity (Del Fabbro et al. Citation2002). On the other hand, it is obvious that the nature of the fuel strongly influences the pressure drop increase due to the cake formed by particles deposited on the filter surface. According to and , one could link the clogging curves to the physicochemical properties of particles emitted during thermal degradation of the studied fuels. Consequently, the smaller the particles are, the higher the pressure drop and dimensionless filter resistance to airflow, for a constant mass of particles per surface area. This result is in agreement with the theoretical prediction of Mädler et al. (Citation2006) who concluded that the porosity of the cake is inversely proportional to the nanoparticle diameter composing the cake. Nevertheless, measuring porosity is not an easy task for pleated HEPA filters, and only experimental porosities of cake formed on non-pleated filters are reported in the literature for combustion aerosols (Kim et al. Citation2009) or nanoparticle aggregates (Elmøe et al. Citation2009; Bourrous et al. Citation2014). Beyond the influence of particle size, morphology and composition are also two significant parameters; as a consequence, the highest pressure drop and dimensionless filter resistance to airflow have been reported for fractal aggregates with low condensate content (oil, TBP/TPH, PMMA). In the case of a diphasic aerosol (soot particles with condensed phase at their surface), the pressure drop is significantly lower. Nevertheless, since these particles present complex compositions, their interaction with combustion gases or even water vapor is not controlled. As reported by Popovicheva et al. (Citation2008), freshly emitted soot particles (low TC content) produced by gaseous flames are mostly hydrophobic. Considering the conclusions of Joubert et al. (Citation2010) on the effect of humidity on the clogging of pleated HEPA filters by non-hygroscopic particles, an increase of humidity for such types of particles tends to increase the pressure drop through filling of the folds by a water film. One could conclude that for low TC content, soot particles are mostly hydrophobic and then water vapor will mostly condense at the surface of the HEPA filters, increasing in the same way the pressure drop. For higher TC content, and in agreement with Popovitcheva et al. (Citation2008), soot particles tends to be hygroscopic, acting as condensation surface for water vapor, decreasing in the same way the quantity of condensed water at the surface of HEPA filters and then consequently decreasing the pressure drop.
FIG. 4. Influence of the nature of the fuel on the clogging of HEPA filters at both filtration velocities.
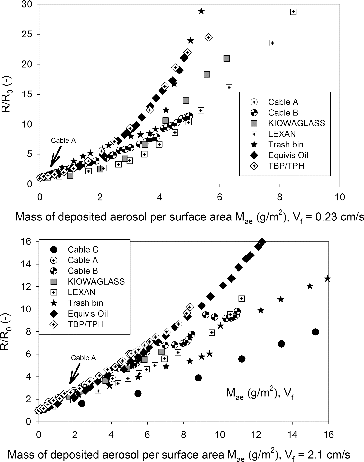
The mean uncertainty associated to the prediction of R/R0 has been estimated to 35% by considering the experimental uncertainties on Vf, Mae and Dp (respectively 15%, 25% and 15%). As presented in , a good agreement is identified between our experimental results and the empirical relationship of Mocho and Ouf (Citation2011). Considering the mean uncertainty previously reported, nearly 90% of the experimental results are well-predicted within an uncertainty interval of ±35%.
One must note that the empirical fitting parameters a and b are kept constant for all the fuels. Beyond this acceptable agreement observed for all the fuels, it is important to mention that the relationship is purely empirical and does not describe the physical phenomena occurring during HEPA filter clogging. Hence, it can only be used for filters studied (H14 media, pleat height of 20 mm, distance between two pleats of about 2 mm) for the range of constant filtration velocity (nominal to nominal divided by 9 filtration velocity), characteristic dimension of particles (primary particle size from 25 nm to 51 nm, equivalent surface projected diameter of the compact particles close to 220 nm) and condensate content (2–10%) studied.
Application to Full-Scale Fires
Finally, experimental results of clogging of HEPA filters for full-scale fires in electrical cabinets are presented in . The main discrepancy between the PICSEL_V1 and EP2 tests is that for the first test, the filtration velocity is maintained constant (0.23 cm/s), while for EP2, the filtration velocity has changed during the test (from 1.4 cm/s to 0.14 cm/s) due to the clogging of the filter itself. These experimental results are to our knowledge the first ones to offer a complete description of the physicochemical properties of fire-generated aerosols and their effect on the clogging of industrial HEPA filters. The empirical relationship has been applied to results obtained during these large-scale tests involving, for the EP2 test, time evolution of stress conditions applied to the filter. First, a good agreement is reported for PICSEL_V1, for which the filtration velocity was kept constant and considering the median equivalent surface projected diameter (222 nm) and a condensate content TC of at least 25%. This good agreement is remarkable and supports the approach based on the development of the empirical model at an analytical scale (BANCO) and its transposition to large-scale fires (DIVA).
For the second large-scale experiment, it is important to recall that the proposed relationship has been developed for a constant filtration velocity and that its application beyond its range of validation must be considered with caution. Thus, for EP2, the evolution of the filtration velocity has been taken into account for application of the empirical relationship. The dashed line presented in has been calculated by taking the mean filtration velocity reported during the experimental measurement of the deposited mass of aerosol per filter unit of area by filter sampling of time periods ranging from 20 s to 600 s. Thus, this dashed line is an extension of 9 predictions of the dimensionless filter resistance to airflow using the empirical relationship. Despite this assumption, it appeared to us that the transposition of this relationship to the dynamic filtration regime of EP2 could be helpful for future research. As a consequence, by considering the median equivalent surface projected diameter of compact particles (226 nm), their condensate content (at least 25% since we were not able to measure the TC content exactly after the large scale experiments for safety reasons) and the change in filtration velocity reported during the EP2 experiment (from 1.4 cm/s to 0.14 cm/s), a qualitative agreement between experiments and empirical relationship is noticed. Despite this reasonable prediction, it is important to keep in mind the limitations of this empirical approach; by definition, such a correlation is limited to the experimental configurations studied and must be considered as a first attempt prior to any further investigation and development of a semi-empirical or a physical model in the future.
CONCLUSIONS
This study presents new results to the scientific community of solid/gas filtration. To our knowledge, it is the first study with a high level of description of the behaviour of this type of HEPA filter with a complete description of the physicochemical properties of aerosols emitted during a fire. This study allows us to identify experimental parameters that influence the behaviour of HEPA filter clogging in case of fire. The filtration velocity, characteristic diameter (diameter of primary particles for the case of fractal aggregates, median surface projected equivalent diameter for the case of compact particles), and the condensate content, have been identified as major parameters that must be taken into account to better understand the clogging of industrial pleated HEPA filters. The most critical situation in terms of clogging will be encountered for lower filtration rates associated with particles of fractal morphology and with condensate contents lower than 5%. Because this work aimed to meet needs related to assessing the safety of nuclear facilities, it proved useful to propose an empirical relationship fitting the experimental results in an acceptable way (holding constant the empirical parameters a and b of this correlation). This relationship is purely empirical and can be used to predict the level of clogging of the filters studied (H14 media, folds up to about 20 mm, distance between two folds of about 2 mm) in static filtration (constant filtration velocity) for the range of filtration velocity (nominal to nominal divided by 9 filtration velocity), characteristic dimensions of particles (primary particle size between 31 nm and 48 nm, equivalent surface projected diameter of the compact particles of the order of 220 nm) and condensate content (2–25%) experimentally studied.
Finally, the correlation was applied to the case of clogging of a HEPA filter in full-scale with constant filtration velocity Vf of 0.23 cm/s (PICSEL_V1) and dynamic filtration regime (variable filtration velocity EP2). Considering, in this relationship, the evolution of particle size and filtration velocity measured experimentally, it can account, qualitatively, for the evolution of the pressure drop across the filter during the tests. Such a comparison does not allow us to determine the relevance of the correlation developed under static conditions to describe the behaviour of a filter in fire configurations different from those considered in this study. To do this, it is essential to maintain our efforts, in particular by studying the dynamic filtration regime for reference aerosols (solid, liquid and simple shapes) less complex than combustion aerosols.
Moreover, the results of this study provide an important experimental database of HEPA filter clogging due to combustion aerosols that can be used in the future to develop a semi-empirical model. Beyond the prospects for improvement related to clogging, further research is needed, firstly, to characterize the condensable phase on the surface of the filters and, secondly, to understand the adsorption and desorption of organic/inorganic components at the surface of fire-generated aerosols.
NOMENCLATURE
Latin Symbols
a | = | empirical constant (m3/g), |
b | = | empirical constant (m8/(g2.s2)), |
Dp | = | characteristic diameter of the deposited particles (nm), |
Dpp | = | median primary particle or monomer diameter (nm), |
Des | = | median equivalent surface projected diameter of particles (nm), |
HEPA | = | High Efficiency Particulate Air, |
LEXAN | = | bisphenol A polycarbonate, |
Mae | = | mass of deposited aerosol per filter surface area (g/m2), |
PMMA | = | polymethyl methacrylate, |
Qv | = | filtration volume flow rate (m3/s), |
R | = | aeraulic resistance of the filter (kg.s−1.m−4), |
R0 | = | aeraulic resistance of the unused filter (kg.s−1.m−4), |
TBP | = | tributyl phosphate, |
TC | = | condensate content of the aerosol (%), |
TPH | = | hydrogenated tetrapropylen, |
Vf | = | filtration velocity (m/s), |
Greek Symbols
ΔP | = | pressure drop of filter (Pa), |
μ, μ0 | = | dynamic viscosity of the filtration air (Pa.s), |
ηp | = | mass emission factor of particles (%). |
ACKNOWLEDGMENTS
The authors thank M. Maillé for performing the TEM analysis of combustion aerosols and S. Bétremieux, P. Ainé, L. Audouin, F. Gensdarmes, F. Hurel, and J. C. Laborde for their help and support. The authors also thank the team of the “Laboratoire d’Expérimentation des Feux” of IRSN in Cadarache (France) where the PICSEL_V1 and EP2 experiments were conducted.
Additional information
Funding
REFERENCES
- Bourrous, S., Bouilloux, L., Ouf, F.-X., Appert-Collin, J.-C., Thomas, D., Tampère, L., et al. (2014). Measurement of the Nanoparticles Distribution in Flat and Pleated Filters During Clogging. Aerosol Sci. Tech., 48(4):392–400.
- Del Fabbro, L., Laborde, J.-C., Merlin, P., and Ricciardi, L. (2002). Air Flows and Pressure Drop Modelling for Different Pleated Industrial Filters. Filtr., Sep. 39:35–40.
- Elmøe, T. D., Tricoli, A., Grunwaldt, J.-D., and Pratsinis, S. E. (2009). Filtration of Nanoparticles: Evolution of Cake Structure and Pressure-Drop. J. Aerosol Sci., 40:965–981.
- Gregory, W. S., Martin, R. A., White, B. W., Nichols, B. D., Smith, P. R., Leslie, I. H., et al. (1991). Fires in Large Scale Ventilation Systems. Nucl. Eng. Des., 125:337–345.
- Hashimoto, K., Nishio, G., and Soda, K. (1993). Fire Behaviour and Filter Plugging During a Postulated Solvent Fire in the Extraction Process of a Nuclear Fuel Reprocessing Plant. Nucl. Tech., 101(2):218–226.
- Joubert, A., Laborde, J.-C., Bouilloux, L., Callé-Chazelet, S., and Thomas, D. (2010). Influence of Humidity on Clogging of Flat and Pleated HEPA Filters. Aerosol Sci. Tech., 44(12):1065–1076.
- Kim, S. C., Wang, J., Shin, G. W., Scheckmann, J. H., and Pui, D. Y. H. (2009). Structural Properties and Filter Loading Characteristics of Soot Agglomerates. Aerosol Sci. Tech., 43(4):344–355.
- Kozeny, J. (1927). Über die kapillare Leitung des Wassers im Boden, Sitzungsberichte der Akademie der Wissenschaften Wien, 136:271–306.
- Le Saux, W., Pretrel, H., and Such, J.-M. (2005). The DIVA ‘multi-room’ Experimental Facility and the DIVA-0 Program. 8th International Symposium on Fire Safety Science, Beijing, China.
- Mädler, L., Lall, A. A., and Friedlander, K. (2006). One-Step Aerosol Synthesis of Nanoparticle Agglomerate Films: Simulation of Film Porosity and Thickness. Nanotech., 17:4783–4795.
- Mocho, V.-M., and Ouf, F.-X. (2011). Clogging of Industrial Pleated High Efficiency Particulate Air (HEPA) Filters in the Event of Fire. Nucl. Eng. Des., 241:1785–1794.
- Novick, V. J., Monson, P. R., and Ellison, P. E. (1992). The Effect of Solid Particle Mass Loading on the Pressure Drop of HEPA Filters. J. Aerosol Sci., 23:657–665.
- Ouf, F.-X., Mocho, V.-M., Pontreau, S., Wang, Z., Ferry, D., and Yon, J. (2014). Physico-Chemical Properties of Aerosol Emitted in Case of Fire Involving Materials Used in the Nuclear Industry. J. Hazard. Mater.
- Ouf, F.-X., Vendel, J., Coppalle, A., Weill, M., and Yon, J. (2008). Characterization of Soot Particles in the Plumes of Over-Ventilated Diffusion Flames. Comb. Sci. Tech., 180:674–698.
- Ouf, F.-X., Yon, J., Ausset, P., Coppalle, A., and Maillé, M. (2010). Influence of Sampling and Storage Protocol on Fractal Morphology of Soot Studied by Transmission Electron Microscopy. Aerosol Sci. Tech., 44:1005–1017.
- Popovicheva, O. B., Persiantseva, N. M., Tishkova, V., Shonija, N. K., and Zubareva, N. A. (2008). Quantification of Water Uptake by Soot Particles. Environ. Res. Lett., 3:025009.
- Thomas, D., Penicot, P., Contal, P., Leclerc, D., and Vendel, J. (2001). Clogging of Fibrous Filters by Solid Aerosol Particles: Experimental and Modelling Study. Chem. Eng. Sci., 56:3549–3561.