Abstract
Before interventions to control horizontal transmission of Mycoplasma gallisepticum can be tested, a suitable experimental model should be available. Transmission dynamics in a flock can be quantified by two parameters: the average number of secondary cases infected by one typical infectious case (R 0) and the number of new infections that occur due to one infectious animal per unit of time (β). The transmission dynamics of M. gallisepticum have not been studied experimentally, so the aim of this study was to examine the horizontal transmission of M. gallisepticum. The study was carried out using a pairwise design with three different inoculation doses. Every pair consisted of an inoculated chicken and a susceptible in-contact chicken. Five susceptible individually housed chickens were placed in between pairs in order to measure airborne transmission. Infection was detected by serology, quantitative polymerase chain reaction and culture. The inoculated and in-contact chickens were equally infectious and the pairs could be regarded as independent. The R 0 was estimated to be greater than 1 (∞; 95% confidence interval, 4.5 to ∞), the estimated β was 0.22 per day and there was no significant difference between the different inoculation doses. It was concluded that the animal model as described in this study meets the conditions for the establishment of transmission dynamics of M. gallisepticum and therefore can be used to establish the quantitative effect of intervention measures on horizontal transmission.
Un modèle expérimental pour quantifier la transmission horizontale de Mycoplasma gallisepticum
Avant de pouvoir tester les interventions relatives à la maîtrise de la transmission de Mycoplasma gallisepticum, un modèle expérimental approprié doit être disponible. Les dynamiques de transmission dans un troupeau peuvent être quantifiées par deux paramètres, le nombre moyen de cas secondaires infectés à partir d'un cas infectieux typique (R 0 ) et le nombre de nouvelles infections qui apparaissent, dues à un animal infecté, par unité de temps (β). Les dynamiques de transmission de M. gallisepticum n'ont pas été étudiées expérimentalement, aussi le but de cette étude a-t-il été d'examiner la transmission horizontale de M. gallisepticum. L'étude a été effectuée en utilisant une construction par paires avec trois doses différentes d'inoculum. Chaque paire était composée d'un poulet inoculé et d'un poulet sensible contact. Cinq poulets sensibles hébergés individuellement ont été placés entre les paires de façon à mesurer la transmission aérienne. L'infection a été détectée par sérologie, par PCR quantitative et par culture. Les poulets inoculés et en contact ont été infectieux de façon équivalente et les paires ont pu être considérées comme indépendantes. Le R 0 a été estimé être supérieur à 1 (∞ (95% C.I. 4,5-∞), le β a été estimé à 0,22 par jour et il n'y a pas eu de différence significative entre les différentes doses d'inoculation. Il a été conclu que le modèle animal tel qu'il est décrit dans cette étude satisfait aux conditions pour l'établissement des dynamiques de transmission de M. gallisepticum et par conséquent peut être utilisé pour établir l'effet quantitatif des mesures d'intervention sur la transmission horizontale.
Ein Versuchsmodell zur Quantifizierung der horizontalen Übertragung von Mycoplasma gallisepticum
Bevor Maßnahmen zur Bekämpfung der horizontalen Übertragung von Mycoplasma gallisepticum getestet werden können, sollte ein geeignetes Versuchsmodell zur Verfügung stehen. Die Übertragungsdynamik in einer Herde kann durch zwei Parameter quantifiziert werden, die durchschnittliche Anzahl der sekundären Fälle ausgehend von einem typischen Infektionsfall (R 0 ) und die Anzahl der Neuinfektionen durch ein infiziertes Tier pro Zeiteinheit (). Da die Übertragungsdynamik von M. gallisepticum bislang nicht experimentell überprüft worden ist, war es Ziel dieser Studie, die horizontale Übertragung von M. gallisepticum zu untersuchen. Die Studie wurde durchgeführt unter Verwendung einer paarweisen Anordnung mit drei verschiedenen Inokulationsdosen. Jedes Paar bestand aus einem inokulierten Huhn und einem empfänglichen Kontakt-Huhn. Fünf weitere empfängliche, einzeln gehaltene Hühner wurden zwischen die Paare plaziert, um eine aeroge Übertragung zu ermitteln. Eine Infektion wurde mittels Serologie, quantitativer Polymerasekettenreaktion (Q-PCR) und Kultivierung nachgewiesen. Die inokulierten und die Kontakttierewaren gleichermaßen infiziert und die Paare konnten als unabhängig voneinander betrachtet werden. Die R 0 wurde auf größer als 1 geschätzt (∞ (95% C.I. 4,5-∞), das geschätzte β betrug 0,22 pro Tag und es gab keinen signifikanten Unterschied zwischen den verschiedenen Inokulationsdosen. Daraus wird geschlossen, dass das in dieser Studie beschriebene Tiermodell den Bedingungen für die Ermittlung der Übertragungsdynamik von M. gallisepticum entspricht und deshalb verwendet werden kann, um den quantitativen Effekt von Interventionsmaßnahmen auf die horizontale Übertragung festzustellen.
Un modelo experimental para cuantificar la transmisión horizontal de Mycoplasma gallisepticum
Antes de que las intervenciones para controlar la transmisión horizontal Mycoplasma gallisepticum puedan ser analizadas, se necesita un modelo experimental adecuado. La dinámica de transmisión en un lote puede ser cuantificada por dos parámetros, la media de casos secundarios infectados a partir de un caso infeccioso típico (R 0 ) y el número de nuevas infecciones que ocurren debido a un animal infeccioso por unidad de tiempo (β). La dinámica de transmisión de M. gallisepticum no ha sido estudiada experimentalmente, por lo tanto el objetivo de este estudio fue el de examinar la transmisión horizontal de M. gallisepticum. El estudio fue llevado a cabo utilizando un diseño pareado con tres dosis de inoculación diferentes. Cada par consisitió en un pollo inoculado y un pollo susceptible en contacto. Cinco pollos susceptibles alojados individualmente fueron ubicados entre los pares para evaluar la transmisión aérea. La infección fue detectada mediante serología, reacción en cadena de la polimerasa cuantitativa (Q-PCR) y cultivo. Los pollos inoculados y en contacto fueron igualmente infecciosos y los pares pudieron ser contemplados como independientes. La R 0 fue estimada como mayor de 1 (∞ (95% C.I. 4.5-∞), la β estimada fue de 0.22 por día y no se encontraron diferencias significativas entre las diferentes dosis de inoculación. Se concluyó que el modelo animal que se describió en este estudio reúne las condiciones para el establecimiento de la dinámica de transmisión de M. gallisepticum y, en consecuencia, puede ser utilizado para establecer el efecto cuantitativo de las medidas de intervención en la transmisión horizontal.
Introduction
Mycoplasma gallisepticum is responsible for significant economic losses in the poultry industry. Current measures to control M. gallisepticum infection include flock testing, eradication and biosecurity programmes, and vaccination. The effect of live and inactivated vaccines on the reduction of economic losses (production loss, condemnation rates) is well described (Carpenter et al., Citation1981; Glisson & Kleven, Citation1983 Citation1985; Sasipreeyajan et al., Citation1987; Evans & Hafez, Citation1992; Evans et al., Citation1992 ;Whithear, 1996; Ley et al., Citation1997). However, information on the ability of these vaccines to reduce the horizontal transmission of M. gallisepticum is limited. Several studies have shown that vaccination reduces the number of M. gallisepticum in the trachea of individual chickens after inoculation (Hildebrand et al., Citation1983; Kleven, Citation1985; Talkington & Kleven, Citation1985; Whithear, Citation1996), but the effect of vaccination on horizontal transmission of M. gallisepticum between chickens has not yet been demonstrated experimentally.
When the aim of vaccination is to eliminate M. gallisepticum from the population, transmission has to be quantified. Transmission dynamics in a flock can be quantified by two parameters: the average number of secondary cases infected by one typical infectious case (R 0) and the number of new infections that occur due to one infectious animal per unit of time (β). The horizontal transmission can be quantified experimentally by housing inoculated and contact animals together. R 0 and β can then be estimated and compared between vaccinated and unvaccinated groups of animals (De Jong & Diekman, Citation1992; De Jong & Kimman, Citation1994). A vaccine is effective when R 0<1, as then an infected animal will infect less than one other animal on average (De Jong & Diekman, Citation1992; De Jong & Kimman, Citation1994). For adequate comparisons, it is essential that infection spreads extensively (R 0>1) in the control group. Moreover, the calculation of transmission is based on the assumption that all animals are equally infectious and susceptible. This implies that the inoculated animals that start the chain of infection should excrete approximately the same amount of M. gallisepticum as animals that are infected by contact.
Transmission experiments on M. gallisepticum have not been reported previously. Before intervention measures can be tested, a suitable experimental model is necessary. The aim of this study, therefore, was to develop an experimental model to quantify the horizontal transmission of M. gallisepticum between laying hens.
Materials and Methods
Experimental design
Two experiments (experiment 1 and experiment 2) were performed. Experiment 1 had three experimental groups, each using a different inoculation dose. Experiment 2 consisted of one experimental group and a control group. Each experimental group consisted of 25 specific pathogen free White Layers, 26 weeks of age. Every group was organized into 10 pairs of birds, with a further five single birds. The pairs were housed in the same cage and consisted of an inoculated chicken and a susceptible in-contact chicken. The size of the cages for pairs was 100×47×53 cm3. The cages were placed in one house at a distance of 65 cm and 178 cm from each other. In between the pairs the five susceptible individually housed chickens were placed at a distance of 65 cm from each other and from the pairs. The size of the cages for individual birds was 50×47×53 cm3. These individually housed chickens were incorporated in the experiment in order to measure the effect of airborne transmission and, consequently, its effect on transmission between the pairs. In experiment 1 the five individually housed chickens were left within the groups throughout the experiment (). In experiment 2, the five individually housed chickens were rotated on a daily basis with five other individually housed birds kept in another room, starting 24 h after exposure. This was done in order to increase the number of observations for airborne transmission.
Figure 1. Experimental design: each group contained 25 specific pathogen free White Layers, of which 20 were housed in pairs and five were housed singly. The cages with pairs were placed at a distance of 65 cm and 178 cm from each other. The individually housed chickens were placed between the pairs at a distance of 65 cm from each other and from the pairs. The pairs consisted of one inoculated chicken (I) and a susceptible in-contact bird (S1). Individually housed chickens were susceptible airborne-exposed birds (S2).
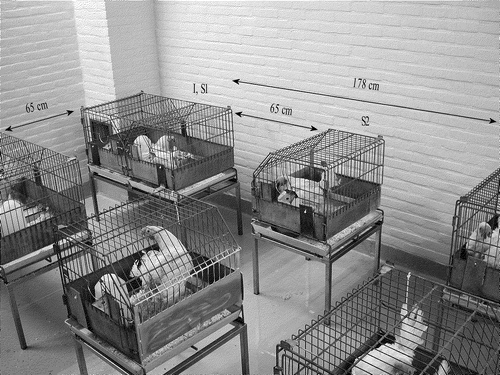
The control group in experiment 2 consisted of five specific pathogen free White Layers, 26 weeks of age, individually housed at a distance of 65 cm from each other. As airborne transmission was detected in experiment 1, a control group was added to experiment 2 in order to exclude effect of testing methods and sample handling. Each group was housed in a separate room.
Two weeks before the start of experiments 1 and 2 the chickens were randomly placed in the experimental facility to become acclimatized. After this period one chicken in each pair was removed and these chickens were housed together in a negative pressure isolator. These birds were inoculated intratracheally with 1.0 ml mycoplasma broth (MB) containing 102 colony-forming units (CFU) M. gallisepticum chicken/NL/Dev-1608Vin/99 (Mg F1999)/ml, 1.0 ml MB containing 105 CFU Mg F1999/ml or 1.0 ml MB containing 108 CFU Mg F1999/ml in experiment 1, and with 1.0 ml MB containing 104 CFU Mg F1999/ml in experiment 2. Twenty-four hours after inoculation the inoculated chickens were reintroduced to the in-contact chickens. This moment of contact was regarded as day 0.
Housing conditions
Climate conditions were the same for all groups. Relative humidity was 60% or less, the ambient temperature was 18 to 20°C and the ventilation rate was 1200 m3/h (±5%). The air was exhausted through four outlets (300 m3/h). Birds were provided 16 h of light and feed ad libitum (rearing pellets, production code 2782 CDI, irradiated with 0.9 Mrad; Hope Farm BV, Woerden, The Netherlands). The chickens were housed on wood shavings and sawdust (Woody Clean BK 10-20; Technilab-BMI, Helmond, The Netherlands).
Frequency of monitoring and handling
One day before inoculation (day–1) all chickens were confirmed to be free of Mycoplasma synoviae and M. gallisepticum by serology and polymerase chain reaction (PCR). At days 2, 3, 4, 5, 7, 9, 11, 14, 21, 25, 28, 31 and 35 after contact, tracheal samples were taken from the inoculated, in-contact, airborne-exposed and control chickens for the detection of the number of viable and non-viable M. gallisepticum by quantitative real-time PCR (Q-PCR). Additionally the presence of viable M. gallisepticum was determined by culture of tracheal samples collected at days 5, 14 and 21 from the same five inoculated chickens.
The in-contact and airborne-exposed chickens were also examined by Q-PCR at day 6 after contact. At days 7, 14, 21, 28 and 35 after contact, blood samples were taken for the detection of antibodies against M. gallisepticum.
In experiment 1 the order of sampling always started with the group inoculated with the lowest dose. In experiment 2, the negative control group was always sampled first. Within every experimental group, the individually housed birds were sampled first. Within the pairs the contact bird was sampled first. Separate clothes, face masks, plastic aprons, plastic cuffs, plastic gloves and boots were used for each experimental group and were changed daily. During sampling there was no contact between the chicken and the person who collected the sample. A different route was taken every day to approach the cages in order to prevent the introduction of systematic errors (bias). Each time that individual birds were handled, gloves, apron and cuffs were disinfected with 70% ethanol in order to kill viable M. gallisepticum.
Q-PCR and culture
Tracheal samples were collected using sterile cotton swabs (Technical Service Consultants, Heywood, UK). When only Q-PCR was performed, swabs were dipped in phosphate-buffered saline (PBS) just prior to sampling. When simultaneous Q-PCR and culture were performed, swabs were dipped in MB (Avian Mycoplasma Liquid Medium; Mycoplasma Experience, Reigate, UK) just prior to sampling. All samples were transported at 4°C and processed within 3 h for simultaneous Q-PCR and culture, and within 24 h when only Q-PCR was performed. Quantification of M. gallisepticum by Q-PCR and culture was performed as described by Mekkes & Feberwee (Citation2005).
Serology
For the M. gallisepticum rapid plate agglutination (RPA) test, the blood samples were examined by mixing 25 µl volumes of undiluted serum and antigen (Intervet International BV, Boxmeer, The Netherlands) on a white perspex plate and gently swirling the mixture. Agglutination within 2 min was considered positive—all positive samples were serially diluted from 1:2 to 1:32 in PBS and re-tested. If agglutination occurred with a dilution of 1:8 or higher, the reaction was considered to be positive (Feberwee et al., Citation2005).
The haemagglutination inhibition (HI) test for M. gallisepticum was carried out using an antigen prepared from a fresh overnight culture of M. gallisepticum (strain S6, ATCC 15302) which was diluted to yield 4 haemagglutinating units for each test. Sera were serially diluted from 1:2 to 1:32 in PBS and antigen/serum mixtures were incubated for 45 min at room temperature and for another 30 min after addition of a 1% suspension of specific pathogen free chicken red blood cells in PBS. The titre of each serum was the reciprocal of the highest dilution that completely inhibited haemagglutination. An HI titre of 8 or higher was considered positive. A sample was regarded positive only when both the RPA and HI tests were positive.
Data analysis
The transmission parameters R 0 and β were quantified using the stochastic susceptible–infectious–recovered model described by Becker (Citation1989). In the pairwise design used in our study, this model states that an in-contact animal is infected during the infectious period of its inoculated pen mate at rate β/2, and that both animals recover at rate α.
R 0 can be estimated using the final size method, a maximum likelihood method, from the total number of contact infections x using the formula: R 0= − 2x/(x−n), where n is the number of experiments. The final size method was also used to derive 95% confidence intervals of R 0 (Velthuis et al., Citation2002). This method assumes that no more in-contact infections can occur, so it will underestimate R 0 if susceptible animals are still in contact with an infectious pen mate at the end of the experiment. Due to the pairwise design, R 0 could be estimated directly from the total number of contact infections as it is known which inoculated bird infected which in-contact bird.
The maximum likelihood method to estimate β does not assume that the infectivity of the animals has stopped at the end of the experiment. This method required determination of two variables for each in-contact bird i: x i , which was 1 if the bird was infected and 0 if not; and Δt i , which was the total duration of its exposure to infectiousness of its pen mate before becoming infected (Klinkenberg et al., Citation2002). In a pairwise experiment, log β and its variance are:
The exposure time Δt i had to be determined for each in-contact bird. This required assumptions about the minimal amount of excretion (threshold) of M. gallisepticum that makes a chicken infectious to allow determination of when exposure started and when the in-contact bird became infected, and the length of the latent period (the period between infection and becoming infectious). Three excretion thresholds (0, 102 and 103 CFU Mg F1999) and four latent periods (0, 1, 2, and 3 days) were used to estimate β, and actual distributions of Δt i were visually compared with the theoretical distribution. The combination of threshold and latent period with the best fit was used for the final estimate of β.
For the estimation of the transmission parameters R 0 and β it was assumed that both the inoculated and the contact-infected chickens were equally infectious. The validity of this assumption was tested by determining, for each bird, the average log (excretion) at four randomly chosen sampling time points, starting with the first positive sample, as a measure of infectiousness. We then tested the null hypothesis that there was no relation between this measure of infectiousness and the status of the bird. The analysis was carried out using the Kruskall and Wallis rank sum test in the statistical program R, version 1.6.2. (R Development Core Team, Citation2003). The power of this test was 98% for detecting a difference of 1 (on the log scale), using the variance of the log (excretion) of the inoculated chickens (Lehmann & D'Abrera, Citation1975).
An additional assumption in the design was that the pairs could be regarded as independent, which meant that transmission between pairs was negligible in comparison with transmission within each pair.
This assumption was tested by relating the cumulative log (excretion) of the in-contact and airborne exposed (y) birds to the cumulative log (excretion) of the inoculated chickens in the same pen (x 1), and in the directly and diagonally apposed pens (x 2), with a linear regression model: y=C+a 1 x 1+a 2 x 2. Cumulative log (excretion) was determined by adding the log (excretion) data for all 35 days, using interpolation for days without measurements. C represents the constant term in the regression model (Petri & Watson, Citation1999). Separate regression models were run for in-contact and airborne exposed chickens, and the P value for a 2, as well as the estimated values of a 1 and a 2, were used to decide whether to consider the influence of chickens in other pens negligible compared with the influence of the chicken in the same pen. The analysis was carried out with the statistical program R, version 1.6.2.
Results
Clinical signs and observation of transmission
Clinical signs were not observed during the study, although more mucus was seen in the trachea during sampling and airsacculitis was observed at in the inoculated and in-contact birds.
In experiment 1 the excretion patterns in the inoculated, in-contact and airborne-exposed chickens showed similar patterns at the different inoculation doses. However the concentration of bacteria excreted differed (). Excretion by the inoculated chickens started within 3 days, depending on the dose inoculated. Excretion levels increased rapidly from day 3 to day 5 and then decreased gradually from day 7 to day 11, ending in a plateau (). In all groups, the concentrations excreted by the airborne-exposed chickens was lower than those excreted by the in-contact birds (). The excretion patterns in the inoculated in-contact and airborne-exposed chickens in experiment 2 showed similar patterns to those see in experiment 1 (data not shown).
Figure 2. Excretion patterns (mean±standard error of the mean) of inoculated, in-contact and airborne-exposed birds after inoculation of 102 CFU Mg F1999 (2A), 105 CFU Mg F1999 (2B) or 108 CFU Mg F1999 (2C). 2C: n = 9 because one inoculated bird died at day 7 after contact. Mg, M. gallisepticum.
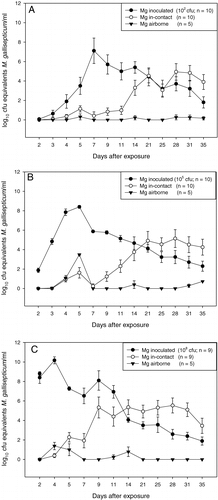
In the group inoculated with 102 CFU Mg F1999 two of the inoculated chickens excreted a relatively low concentration of M. gallisepticum at only one or two sampling points (101 and 102 CFU equivalents/ml). However, despite this low level of excretion, the in-contact birds became infected. None of the four birds developed antibodies against M. gallisepticum until day 35 after contact. In all other pairs infection was confirmed by a positive serological result in both the Mg RPA and HI test starting at day 14 for the inoculated birds and at day 21 for the in-contact chickens (A). Four of five inoculated birds that were examined by Q-PCR and culture were positive in both methods, confirming the presence of viable mycoplasmas. One inoculated chicken that shed low concentrations of M. gallisepticum (102 CFU equivalents/ml) as assessed by the Q-PCR was negative by culture.
Figure 3. Number of birds serological positive (RPA and HI ≥ 1:8) to M. gallisepticum (Mg) among inoculated, in-contact and airborne-exposed birds over the observation period of 35 days, after inoculation of 102 CFU Mg F1999 (3A), 105 CFU Mg F1999 (3B) or 108 CFU Mg F1999 (3C). 3C: n = 9 because one inoculated died bird at day 7 after contact.
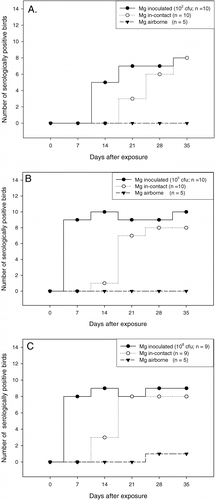
In the group inoculated with 105 CFU Mg F1999, all inoculated birds excreted M. gallisepticum starting at day 2 after contact. Infection was confirmed by positive serology starting at day 7 for the inoculated birds and at day 14 for the in-contact birds (B). The five inoculated birds examined by both culture and Q-PCR were positive in both methods, confirming the presence of viable mycoplasmas. M. gallisepticum was also detected in all in-contact chickens by Q-PCR. In two of the in-contact chickens a low concentration of M. gallisepticum was detected (101 to 103 CFU equivalents/ml). A serological response was not detected in these two birds throughout the observation period (B).
In the group inoculated with 108 CFU Mg F1999, all inoculated birds excreted M. gallisepticum throughout the observation period, with the exception of one that died at day 9. The five inoculated birds examined by culture and Q-PCR were positive in both methods, confirming the presence of viable mycoplasmas. All inoculated birds had a detectable antibody response from day 7 on. Eight in-contact birds excreted M. gallisepticum and had a detectable antibody response starting at day 14 (C). In one pair, although the inoculated bird excreted M. gallisepticum, neither excretion nor an antibody response was detected in the in-contact chicken throughout the 35 days of observation (C).
In experiment 2 results were comparable with those of experiment 1, with all but one of the inoculated chickens excreting M. gallisepticum throughout the 35-day observation period and responding serologically starting at day 7. The five inoculated birds examined by culture and Q-PCR were positive in both methods, confirming the presence of viable mycoplasmas. All but one of the in-contact birds became infected and frequently excreted M. gallisepticum throughout the 35 days after contact. All but two of the in-contact birds responded serologically starting at day 21. The control group was negative in the Mg RPA and HI tests, and by Q-PCR, throughout the experimental period (data not shown), showing that the specificity of the used detection methods was high.
In all experimental groups the individual chickens housed in between pairs excreted amounts of M. gallisepticum detectable by Q-PCR at one or more time points (). M. gallisepticum infection was, however, only confirmed by a positive RPA and HI test in one bird in the group inoculated with 108 CFU Mg F1999 (C). This bird excreted low concentrations of M. gallisepticum (102 to 103 CFU equivalents/ml).
Analysis of infectivity, homogeneity and independency of excretion
As only one in-contact chicken in the group inoculated with 108 CFU escaped infection, there was no difference in susceptibility between the inoculated and the in-contact chickens. Airborne transmission did occur. The epidemic process had not ended within the period of observation, because both the inoculated and the in-contact infected chickens were still excreting M. gallisepticum at day 35.
The estimated regression coefficient for the airborne-exposed birds (a 2=0.0073, P=0.0003), quantifying the contribution to transmission by chickens in neighbouring pens in the absence of infectious pen mates, was 100 times smaller than that for the in-contact birds (a 1=0.71, P=0.0006), which quantifies the contribution to transmission by the infectious pen mate. Moreover, a 2 was not significantly different from 0. Thus the pairs could be analysed as independent observations.
A to 2C suggest that the mean excretion levels of the in-contact and airborne-exposed chickens were lower than those of the inoculated chickens. However, statistical analysis of the excretion levels () revealed that the infected and contact chickens in all experimental groups can be regarded as equally infectious, as the concentrations excreted by the in-contact and infected birds within pairs were not significantly different (P=0.096, P=0.23 and P=0.13 for the three different inoculation doses in experiment 1, and P=0.62 for experiment 2). The concentrations excreted by in-contact and airborne-exposed chickens were significantly different from each other (P=0.0078 [dose of 102 CFU/ml] and 0.013 [dose of 108 CFU/ml] in experiment 1, and P=0.0035 in experiment 2), with the exception of the in-contact and airborne-exposed chickens within the group inoculated with 105 CFU in experiment 1 (P=0.22). Excretion by the inoculated and airborne-exposed chickens was significantly different from each other in all experimental groups (P=0.0021, P = 0.022 and P = 0.0098 for the three different inoculation doses in experiment 1, and P=0.00082 in experiment 2).
Table 1. Significance of the difference in the average log10 (cumulative excretion) at four random sampling time points
Transmission parameters
As only one in-contact chicken in experiment 1 escaped infection, R 0 was significantly larger than 1 in all groups, and no significant differences in R 0 were observed between groups. In two groups of experiment 1 (doses of 102 and 105 CFU/ml), all 10 in-contact chickens became infected, resulting in an estimated R 0 of ∞ (95% confidence interval, 4.5 to ∞). In the third group in Experiment 1, eight of the remaining in-contact chickens were M. gallisepticum-positive, and R 0 was estimated to be 16.0 (95% confidence interval, 2.1 to 710). In experiment 2, one inoculation failed and the other nine in-contact chickens were infected, so R 0=∞ (95% confidence interval, 3.9 to ∞).
The estimates of β did not differ significantly between the three excretion thresholds and four latent periods. The best fit was achieved with an excretion threshold of 103 CFU and a latent period of 2 days, resulting in an estimate of β of 0.22 per day (95% confidence interval, 0.16 to 0.32).
Discussion
In this study we developed a model to quantify the horizontal transmission of M. gallisepticum between laying hens, which was validated with respect to the assumptions included in the analysis. We concluded that M. gallisepticum spread well in the model (R 0>1), that inoculated and in-contact chickens were equally infectious (homogeneity), and that, although several pairs of hens were housed in the same room, these pairs could be considered independent. Consequently, the model seems suitable to test the effectiveness of intervention strategies against M. gallisepticum infection. During the study, we observed that an inoculum of 102 CFU evoked sufficient horizontal transmission, resulting in a generally high level of excretion of M. gallisepticum. We also found that airborne transmission of M. gallisepticum occurred over short distances. However, the number of mycoplasmas excreted by birds infected by airborne transmission was much lower than that excreted by birds infected by direct contact.
Animal models to establish transmission dynamics have been described for some important bovine, porcine and avian pathogens. Some of these models have been used to establish the effect of intervention measures on the transmission of the disease (De Jong & Kinman, Citation1994; Bouma et al., Citation1997; De Wit et al., Citation1997; Nodelijk et al., Citation2001; Velthuis et al., Citation2002; Van der Goot et al., Citation2003). The advantage of transmission models over field experiments is that they offer a controlled environment in which the influence of intervention measures can be investigated.
The design of the experimental model depends on the horizontal transmission dynamics of the agent. The final size approach is based on the assumption that the epidemic process has ended at the end of the trial, with no susceptible and no infectious animals left. This is often the case for viral agents. For a bacterial agent like Actinobacillus pleuropneumoniae it has been shown that transmission is more variable (Velthuis et al., Citation2002), and could not be explained by a final size approach as this would lead to an underestimation of R 0, especially when R 0 >1. In these cases transmission has to be explained using a statistical model that assumes that the epidemic process has not yet ended before the end of the experiment (Velthuis et al., Citation2002). A one-to one setting is preferred as in this design it is known which inoculated animal infected which in-contact animal. Moreover, in such a pairwise design the estimation of the transmission parameter R 0 will be more robust, especially when R 0 is higher than 1 (Velthuis et al., Citation2002).
The development of the transmission model for M. gallisepticum was based on the knowledge about transmission from other experimental studies. In most transmission experiments, high inoculation doses were used. No information, however, was available about excretion levels in relation to the dose of inoculum (Jordan, Citation1975; Kleven, Citation1985; Yagihashi & Tajima, Citation1986; Soeripto et al., Citation1989). For the quantification of transmission this information is important. If the dose is too high there is a risk that transmission will be overestimated, while it will be underestimated if the dose is too low. Therefore this study was carried out with different doses. It has been shown that, once spread commences, M. gallisepticum can infect all the birds in a flock (Olesiuk & van Roekel, Citation1960; McMartin et al., Citation1987). Furthermore, others have suggested that lifetime persistence of M. gallisepticum cannot be excluded, as M. gallisepticum survives in the respiratory tract, and perhaps in other organs, after infection (Kleven, Citation1981 Citation1985). Based on this information we assumed that the transmission would not have terminated at the end of our observation period. Consequently, a pairwise design was chosen.
The determination of the transmission parameters was based on the assumption that all birds were equally infectious and susceptible. The results showed that at day 35 after exposure, all but one of the in-contact chickens had been infected and that almost all inoculated and in-contact chickens were excreting M. gallisepticum. It can be concluded that the inoculated and in-contact chickens were equally infectious as there were no significant differences between excretion levels, and that the pairs could be regarded as independent, as airborne transmission was limited at all inoculation doses and had no significant effect on the excretion levels of the pairs. On basis of the excretion levels of the in-contact and airborne-exposed chickens, it was possible to conclude that the amount of bacteria excreted by the in-contact chickens was mainly determined by the inoculated chicken in the same cage, enabling the influence of airborne transmission to be excluded from the model.
Although airborne transmission was detected by Q-PCR, it could not be confirmed by serology in most cases. It is known from other studies that the inoculation dose and distance between birds can influence isolation rates and M. gallisepticum RPA titres (McMartin & Adler, Citation1961; Talkington & Kleven, Citation1985; McMartin et al., Citation1987). Additionally the occurrence of false-positive results in the airborne exposed birds can be eliminated by the fact that the Q-PCR was highly specific (negative Q-PCR results in the control birds).
Our observation that airborne transmission only occurs over a short distance and at a low level cannot be extrapolated directly to the field. In this study only 25 chickens were placed in the room and the ventilation rate was 1200 m3/h. This ventilation rate per chicken is much higher than under field conditions, possibly leading to a dilution of M. gallisepticum in the air leading to a decrease in the rate of airborne transmission. Work by Jungherr (Citation1958) has suggested that increasing the ventilation rate decreases the rate of horizontal spread.
The M. gallisepticum transmission model described here may be of value to study intervention strategies against M. gallisepticum such as vaccination. If a vaccination programme against M. gallisepticum resulted in R 0<1, it will contribute to a reduction in horizontal transmission and stop the spread of infection.
Translations of the abstract in French, German and Spanish are available on the Avian Patholgy website.
Acknowledgments
This research was supported by a grant from the National Board for Poultry and Eggs (PPE) of the Netherlands. The author thank W. J. M. Landman for the photograph of the experimental design and for critically reading the manuscript.
References
- Becker , N.G. 1989 . Analysis of Infectious Disease Data. Monographs on Statistics and Applied Probability. Vol 1 , 1st edn , London : Chapman and Hall .
- Bouma , A. , de Jong , M.C.M. and Kimman , T.G. 1997 . Comparison of two pseudorabies virus vaccines, that differ in capacity to reduce virus excretion after challenge infection, in their capacity of reducing transmission of pseudorabies virus . Veterinary Microbiology , 54 : 113 – 122 .
- Carpenter , T.E. , Mallinson , E.T. , Miller , K.F. , Gentry , R.F. and Schwartz , L.D. 1981 . Vaccination with F strain Mycoplasma gallisepticum to reduce production losses in layer chickens . Avian Diseases , 25 : 404 – 409 .
- De Jong , M.C.M. and Diekman , O. 1992 . A method to calculate for computer simulated infections the threshold value R 0, that predicts whether or not the infection will spread . Preventive Veterinary Medicine , 12 : 269 – 285 .
- De Jong , M.C.M. and Kimman , T.G. 1994 . Experimental quantification of vaccine-induced reduction in virus transmission . Vaccine , 12 : 761 – 766 .
- De Smit , A.J. , de Jong , M.C.M. , de Kluijver , E.P. and Moorman , R.J.M. 2000 . Determination of the onset of the herd-immunity induced by the E2 sub-unit vaccine against classical swine fever virus . Vaccine , 18 : 1374 – 1381 .
- De Wit , J.J. , de Jong , M.C.M. , Pijpers , A. and Verheijden , J.M.H. 1997 . Transmission of infectious bronchitis virus within vaccinated and unvaccinated groups of chickens . Avian Pathology , 27 : 464 – 471 .
- Evans , R.D. and Hafez , Y.S. 1992 . Evaluation of a Mycoplasma gallisepticum strain exhibiting reduced virulence for prevention and control of poultry mycoplasmosis . Avian Diseases , 36 : 197 – 201 .
- Evans , R.D. , Hafez , Y.S. and Orthel , F.W. 1992 . Mycoplasma gallisepticum vaccination-challenge: an egg-production model . Avian Diseases , 36 : 956 – 963 .
- Feberwee , A. , Mekkes , D.R. , De Wit , J.J. , Hartman , E.G. & Pijpers , A. (2005) . Comparison of culture, PCR and different serological tests for detection of Mycoplasma gallisepticum and Mycoplasma synoviae infections . Avian Diseases , 49 , 260 – 268 .
- Glisson , J.R. and Kleven , S.H. 1983 . Mycoplasma gallisepticum vaccination: effects on egg transmission and egg production . Avian Diseases , 28 : 406 – 415 .
- Glisson , J.R. and Kleven , S.H. 1985 . Mycoplasma gallisepticum vaccination: further studies on egg transmission and egg production . Avian Diseases , 29 : 408 – 415 .
- Hildebrand , D.G. , Page , D.E. and Berg , J.R. 1983 . Mycoplasma gallisepticum (MG)—laboratory and field studies evaluating the safety and efficacy of an inactivated MG bacterin . Avian Diseases , 27 : 792 – 802 .
- Jordan , F.T.W. 1975 . Immunity to mycoplasma infections of the repiratory system in the domestic fowl and turkey . International Symposium on Immunity to Infections of the Respiratory System in Man and Animals , 28 : 590 – 596 .
- Jungherr , E.L. 1958 . The control of chronic respiratory disease. Symposium on chronic respiratory diseases, IV . American Journal of Veterinary Research , 19 : 464 – 467 .
- Kleven , S.H. 1981 . Transmissibility of F strain of Mycoplasma gallisepticum in Leghorn chickens . Avian Diseases , 25 : 1005 – 1018 .
- Kleven , S.H. 1985 . Tracheal populations of Mycoplasma gallisepticum after challenge of bacterin-vaccinated chickens . Avian Diseases , 29 : 1012 – 1017 .
- Kleven , S.H. , Khan , M.I. and Yamamoto , R. 1990 . Fingerprinting of Mycoplasma gallisepticum strains isolated from multiple age-layers vaccinated with live F strain . Avian Diseases , 34 : 984 – 990 .
- Klinkenberg , D. , de Bree , J. , Laevens , H. and de Jong , M.C.M. 2002 . Within- and between-pen transmission of Classical Swine Fever Virus: a new method to estimate the basic reproduction ratio from transmission experiments . Epidemiology and Infection , 128 : 293 – 299 .
- Kroese , A.H. & De Jong , M.C.M. (2001) . Design and analysis of transmission experiments . Proceedings of the Society of Veterinary Epidemiology and Preventive Medicine ( pp. 22 – 37 ). Noordwijkerhout , The Netherlands .
- Lehmann , E.L. and D'Abrera , H.J.M. 1975 . Nonparametrics: Statistical Methods Based on Ranks , San Francisco : Holden-Day .
- Landman , W.J.M. , Corbanie , A.A. , Feberwee , A. and van Eck , J.H.H. 2004 . Aerosolisation of Mycoplasma synoviae compared to M. gallisepticum and Enterococcus faecalis . Avian Pathology , 3 : 210 – 215 .
- Lauerman , L.H. , Chilina , A.R. , Closser , J.A. and Johansen , D. 1995 . Avian mycoplasma identification using polymerase chain reaction amplicon and restriction fragment length polymorphism analysis . Avian Diseases , 39 : 804 – 811 .
- Levisohn , S. and Kleven , S.H. 1981 . Vaccination of chickens with nonpathogenic M. gallisepticum as a means for displacement of pathogenic strains . Israel Journal of Medical Science , 20 : 982 – 984 .
- Ley , D.H. , McLaren , J.M. , Niles , A.M. , Barnes , H.J. , Miller , S.H. and Granz , G. 1997 . Transmissibility of live Mycoplasma gallisepticum vaccine strains ts-11 and 6/85 from vaccinated layer pullets to sentinel poultry . Avian Diseases , 41 : 187 – 194 .
- Mars , H.M. , de Jong , M.C.M. and van Oirschot , J.T. 2000 . A gE-negative BHV1 vaccine virus strain cannot perpetuate in cattle populations . Vaccine , 18 : 2120 – 2124 .
- McMartin , D.A. and Adler , H.E. 1961 . An immunological phenomenon in chickens following infection with Mycoplasma gallisepticum . Journal of Comparative Pathology , 71 : 311 – 323 .
- McMartin , D.A. , Khan , M.I. , Farver , T.B. and Christie , G. 1987 . Delineation of the lateral spread of Mycoplasma gallisepticum infection in chickens . Avian Diseases , 31 : 814 – 819 .
- Mekkes , D.R. & Feberwee , A. (2005) . Real-time PCR for the qualitative and quantitative detection of Mycoplasma gallisepticum . Avian Pathology , in press .
- Nodelijk , G. , de Jong , M.C.M. and van Leengoed , L. 2001 . A quantitative assessment of the effectiveness of PRRSV vaccination in pigs under experimental conditions . Vaccine , 19 : 3636 – 3644 .
- Olesiuk , M. and van Roekel , H. 1960 . Transmission of chronic respiratory disease in chickens . Avian Diseases , 4 : 348 – 368 .
- Petri , A. and Watson , P. 1999 . Statistics for Veterinary and Animal Science , Oxford : Blackwell Science .
- R Development Core Team (2003) . R: A language and Environment for Statistical Computing . Vienna : R Foundation for Statistical Computing . Available online at: http://www.R-project.org
- Sasipreeyajan , J. , Halvorson , D.A. and Newman , J.A. 1987 . Effect of Mycoplasma gallisepticum bacterin on egg transmission and egg production . Avian Diseases , 31 : 776 – 781 .
- Soeripto , Whithear , K.G. , Cottew , G.S. and Harrigan , K.E. 1989 . Virulence and transmissibility of Mycoplasma gallisepticum . Australian Veterinary Journal , 66 : 365 – 372 .
- Talkington , F.D. and Kleven , S.H. 1985 . Evaluation of protection against colonization of the chicken trachea following administration of Mycoplasma gallisepticum bacterin . Avian Diseases , 29 : 998 – 1003 .
- Turner , K.S. and Kleven , S.H. 1998 . Eradication of Live F strain Mycoplasma gallisepticum vaccine using live ts-11 on a multi-age commercial layer farm . Avian Diseases , 42 : 404 – 407 .
- Van der Goot , J.A. , Koch , G. , de Jong , M.C.M. and van Boven , M. 2003 . Transmission dynamics of low and high pathogenicity A/Chicken/Pennsylvania/83 avian influenza viruses . Avian Diseases , 47 : 939 – 941 .
- Velthuis , A.G.J. , de Jong , M.C.M. , de Bree , J. , Nodelijk , G. and van Boven , M. 2002 . Quantification of transmission of one-to-one experiments . Epidemiology and Infection , 128 : 193 – 204 .
- Whithear , K.G. 1996 . Control of avian mycoplasmosis by vaccination . Revue Scientifique et Technique , 15 : 1527 – 1553 .
- Yagihashi , T. and Tajima , M. 1986 . Antibody responses in sera and respiratory secretions from chickens infected with Mycoplasma gallisepticum . Avian Diseases , 30 : 543 – 550 .