ABSTRACT
Avian orthoreovirus (ARV) infections of broiler flocks cause arthritis/tenosynovitis syndrome and significant economic losses. ARV variants were detected in the USA and Canada. Viral arthritis/tenosynovitis syndrome has occurred frequently in China in recent years. In this study, a variant ARV strain associated with viral arthritis/tenosynovitis syndrome was isolated from broilers and designated as LY383. Genomic sequence and phylogenetic analysis of the σC nucleic acid and amino acid sequences revealed that the isolate was closely related to ARV field strains Reo/PA/Layer/01224B/14, Reo/PA/Broiler/1551/13, GA/14602/2014, GA/13569/2013 and GA/13542/2013, in cluster V, but distinct from most Chinese field strains or commercial vaccine strains. Experimental challenge showed that the isolate could cause arthritis/tenosynovitis syndrome in broilers, which possessed a high level of maternal antibodies induced by commercial ARV vaccines (S1133, 1733 and T98). Furthermore, viral nucleic acid could be detected in cloacal swabs of all challenged birds throughout the entire test from 5 dpi onward. These results suggest that a novel ARV genotype emerges and might become prevalent in broiler flocks in China.
RESEARCH HIGHLIGHTS
A variant avian orthoreovirus was isolated from a vaccinated broiler flock in North China.
The ARV field strain was distinct from previous China-origin ARV isolates and vaccine strains.
The current commercial ARV vaccine could not provide effective protection of broilers against the field isolate infection.
These findings indicated that variant ARV field strains might become frequent in broiler flocks in China and effective measures should be conducted to prevent and control the disease.
Introduction
Avian orthoreoviruses are widespread and cause various clinical and subclinical manifestations in chickens, turkeys, ducks, geese and other domestic poultry (Petek et al., Citation1967; Simmons et al., Citation1972; van der Heide et al., Citation1981; Marius-Jestin et al., Citation1988; Gough et al., Citation1990). ARV-infected chickens show a variety of clinical signs, including arthritis/tenosynovitis, runting-stunting syndrome, hepatitis, myocarditis, malabsorption syndrome and central nervous system disease (van der Heide, Citation2000). Moreover, ARV infection causes host immunosuppression and makes birds more susceptible to secondary infections (Montgomery et al., Citation1986; Jones, Citation2000).
ARV is a non-enveloped double-stranded RNA (dsRNA) virus, which is classified into the genus Orthoreovirus, family Reoviridae. ARV virions are icosahedral, multilayered particles of 70–80 nm in diameter (Spandidos & Graham, Citation1976). Similar to other members in this genus, the ARV genome consists of 10 dsRNA segments, including three L-class (L1, L2, L3), three M-class (M1, M2, M3) and four S-class (S1, S2, S3, S4). Each segment encodes a single protein except for the S1 segment, which encodes three proteins. The S1 gene encodes two nonstructural proteins (p10 and p17) and one structural protein (σC), which is the viral cell attachment protein and elicits ARV-specific neutralizing antibodies. The S3 gene encodes σB protein which carries group-specific neutralizing epitopes (Benavente & Martinez-Costas, Citation2007).
In recent years, some novel ARV strains were isolated from vaccinated broiler flocks with arthritis/tenosynovitis in North America, and caused huge economic losses to the poultry industry (Tang et al., Citation2016; Tang & Lu, Citation2016; Ayalew et al., Citation2017). However, viral arthritis/tenosynovitis syndrome has increased in commercial broiler flocks in North China. Previous studies revealed that the antibody titres of many vaccinated broiler flocks were abnormally high and ARV isolates shared the highest sequence homologies with vaccine strain S1133 (Zhong et al., Citation2016).
In the present study, we obtained an ARV isolate from a vaccinated broiler flock in Shandong. The complete genome was sequenced and phylogenetic analysis and comparison with classical ARV strains was conducted. We also evaluated the characterization in vitro and in vivo, and the pathogenicity in chicks vaccinated with commercial vaccines. These findings will provide reliable evidence for the prevention and control of ARV infection.
Materials and methods
Ethical statement
All animal experiments were approved by the Animal Care and Use Committee of Shandong Agricultural University and performed in accordance with the “Guidelines for Experimental Animals” of the Ministry of Science and Technology (Beijing, China).
Virus isolation
Fresh synovial fluid and tendon samples from broilers with arthritis and tenosynovitis were collected from a broiler flock in Laiyang county, Shandong province. Tendon tissues were homogenized in viral transportation medium (VTM) to obtain a 20% suspension (w/v). This suspension and synovial fluid were frozen-thawed three times and centrifuged at 6500 g at 4°C for 20 min. Aliquots of the supernatant were passed through a 0.22-μm filter and then inoculated into primary LMH (chicken liver hepatocellular carcinoma cell line) monolayer cells (ATCC CRL-2113) for 1 h at 37°C for adsorption. The isolated virus was designated LY383 and cultured for at least three passages for pathogenicity test and sequencing. The virus titres were determined by plaque assay on LMH cells. Cell cultures were monitored daily for cytopathic effects (CPE). When the CPE was over 80% developed, cell cultures were frozen-thawed three times and then centrifuged. Suspensions were stored at −70°C. The titre of infectious viral progeny was determined in LMH cells in 96-well plates as the TCID50 per 100 μL, using the Reed–Muench method (Reed & Muench, Citation1938).
Isolation of viral nucleic acid and RT–PCR
Viral nucleic acid was extracted from virus P3 stock using the RNeasy Mini Kit (QIAGEN, Valencia, CA, USA) following the manufacturer's protocol. Extracted RNA, with 40U RNase inhibitor, was stored at −20°C. Viral cDNA was generated from RNA samples using the Reverse Transcriptase M-MLV (Takara, Dalian, China) with random primers (6-mers). Partial S1 segment was amplified from the cDNA by PCR using P1/P4 primers (Kant et al., Citation2003). The RT–PCR products were purified, cloned into pMD18-T vector and transformed into DH5α E. coli competent cells, and the positive clone was sequenced (Invitrogen, Shanghai, China).
Next-generation sequencing and genome assembly
Sequencing of viral RNA was conducted by using Illumina HiSeq1500 (Axeq Technologies, Rockville, MD) protocol. A random primer cDNA library was initially generated using TotalScript™ RNA-Seq Kit (Illumina, San Diego, CA, USA), but without the initial poly-A enrichment. The cDNA preparation was monitored for quality using the standard Illumina protocol, then sequenced on an Illumina HiSeq1500 with 150-nt paired-end reads sequencing according to the manufacturer’s protocol.
The NGS raw sequence reads were analysed and assembled into contiguous sequences (contigs) using CLC Bio Genomics Workbench 10.0 software. All ARV-related aligned contigs were identified and extracted after alignment to the reference genome database (ftp://ftp.ncbi.nlm.nih.gov/blast/db/ref_viruses_rep_genomes.tar.gz). The scaffolding and ordering of the contigs for each segment were facilitated by mapping the contigs on reference viral genomes. Using the final complete genome consensus sequence, 10 segments of LY383 genome were constructed and the regions corresponding to gaps in the original reference mapping were revised. Finally, the verified consensus sequences were submitted to NCBI GenBank.
Phylogenetic analysis
Based on nucleotides and amino acids of the σC from S1 segment and the σB from S3 segment, phylogenetic analysis was carried out. Sequence alignments were performed using the ClustalW methods in Megalin program included in Lasergene 7.0 software (DNASTAR Inc., Madison WI, USA). The phylogenetic trees were constructed using the neighbour-joining method of the MEGA program (version 5.0) with bootstrap values calculated from 1000 replicates (Tamura et al., Citation2011).
Pathogenicity in chicks
One-day-old broilers were purchased from a breeder stock inoculated with three commercial ARV vaccines (S1133, 1733 and T98) against avian orthoreovirus. All chicks were housed in isolators. To evaluate the pathogenicity in chicks, forty one-day-old broilers hatched from ARV vaccinated breeder stocks were randomly divided into four groups (each n = 10). Group 1 and group 2 were inoculated intranasally with 106.1 TCID50 doses of challenge virus (ARV LY383 isolate and virulent S1133), respectively. Group 3 received an equal dose of LY383 via subcutaneous injection in the feet. The 10 remaining chicks served as a negative control group and were inoculated intranasally with 0.2 ml sterile phosphate-buffered saline (PBS). Each group of chickens was separately housed in a different isolator and monitored daily for 25 days post infection (dpi). Clinical symptoms of viral tenosynovitis/arthritis were examined and recorded. Tissue samples were collected, stained in haematoxylin–eosin (HE) and examined. Moreover, cloacal swabs from all birds were collected to monitor viral nucleic acid using one-step real-time RT–PCR (Tang & Lu, Citation2016).
Results
Virus isolation and identification
The ARV field isolate LY383 was obtained from tendons and joint fluid mixture of ARV-infected broilers ((A, B)). CPE appeared in the LMH monolayer cell cultures infected with the LY383 isolate. The CPE of LY383 manifested as numerous cells fused into discs of varying sizes during the incubation with cultured LMH cells ((C)). It was confirmed by giant or “bloom-like” CPE-positive cells as a previous study (Lu et al., Citation2015). The CPE appeared at 36 h post-inoculation after three serial cell passages. The titre of LY383 was determined to be 107.1 TCID50/0.2 mL at 48 h post-inoculation in LMH cells. The S1-based RT–PCR was applied for the detection of ARV from specimens and viral stock. RNA extractions of S1133-infected cells and non-infected cells served as positive and negative controls, respectively. A fragment of 1088-bp size was successfully amplified, purified and sequenced. Other viruses that infect broilers, such as avian influenza A virus (AIV), Newcastle disease virus (NDV), infectious bronchitis virus (IBV), infectious bursal disease virus (IBDV), Marek’s disease virus (MDV), fowl adenoviruses (FAV), avian leukosis virus (ALV), chicken infectious anaemia virus (CIAV), and reticuloendotheliosis virus (REV) were negative in viral P3 stock.
Figure 1. Pathologic and histopathologic changes in broilers and monolayer LMH cells infected by LY383. Diseased broilers showed (A) arthritis/tenosynovitis; (B) Swelling, oedema, and haemorrhages in the tendons. (C) The CPE of LMH cells manifested as numerous cells fused into discs of varying sizes. Histopathologic analysis of lesions in the small intestine showed (D) villous atrophy, the tendon showed (E) interstitial inflammation and loose connective tissue stroma, and the liver showed (F) fatty degeneration of hepatocytes, and severe haemorrhage accompanied by lymphocyte infiltration.
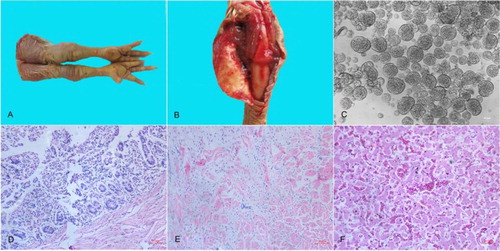
NGS data analysis
All samples were positive for ARV by RT–PCR prior to sequencing. A total of 7,312,450 sequencing reads of 186-base paired-end reads were generated by the HiSeq sequencer resulting in 1.03 Gb of sequence data from viral stocks. Those short reads that passed quality control filtering were removed following alignment to the database of chicken mRNA or rRNA sequences. The vestigial reads were aligned against viral nucleotide databases sequentially (ftp://ftp.ncbi.nlm.nih.gov/blast/db/ref_viruses_rep_genomes.tar.gz) to map the sequence composition using CLC Genomic Workbench (version 10.0). 7648 clean reads were classified as virus-related sequences.
De novo assembly of the ARV field strain genome
The mapped reads were considered to represent virus-related sequences. All these reads were assembled de novo using CLC Genomic Workbench software. One-hundred-and-seven contigs were obtained and aligned using multi-blast searching. For LY383 genome assembly, the ARV-related sequences identified covered 99% of the ARV reference genomes. The average coverage of reads was 64.08-fold. Finally, 10 contigs were generated, corresponding to the 10 genome segments of ARV. Each segment of the entire genome was remapped with ARV reference genomes and accurately assembled into a single contig.
The complete genome of LY383 isolate
The full-length consensus genome segments of LY383 were obtained and deposited in GenBank (accession no. MF183212-MF183221). The complete genome sequence of ARV LY383 was 23,467 bp in length, consisting of 10 segments which ranged from 1192 bp (S4) to 3959 bp (L1) in size. Each segment encodes a single open reading frame (ORF) except S1 segment which consists of three partially overlapping ORFs encoding p10, p18, and σC proteins. Each segment of LY383 genome was entered as a query sequence by using the NCBI BLASTN program (https://blast.ncbi.nlm.nih.gov/Blast.cgi). The sequence similarity values of each segment with reference ARV strains are listed in . These similar reference ARV strains were isolated from broilers and layers.
Table 1. Characteristics and identities of genome segments to the most similar reference strains.
Phylogenetic analysis of S1 genes
A phylogenetic tree based on S1 gene nucleotide sequences was generated to determine the genetic diversity between LY383 and other ARV isolates obtained from GenBank (). The tree contains six lineages. Phylogenetic analysis of S1 genes revealed that LY383 shared the highest sequence similarity (96.4–98.7%) with the reference strains Reo/PA/Layer/01224B/14, Reo/PA/Broiler/1551/13 and Reo/PA/Pheasant/13649/14. However, they shared a lower similarity (45.4–52.6%) with ARV vaccine strains S1133, 1733 and T98. The phylogenetic tree showed that the LY383, together with four ARV strain isolated from the United States, were clustered into a distinct lineage, while most China ARV isolates were clustered into another lineage, which contained the vaccine strains.
Figure 2. Phylogenetic tree of S1 gene sequences of the field isolate LY383 (●) and reference ARV field isolates and vaccine strains(▴). Numbers at nodes indicate bootstrap percentages obtained using 1000 replicates. LY383 was clustered into lineage 4, including some US isolates, and distinct from commercial vaccine strains.
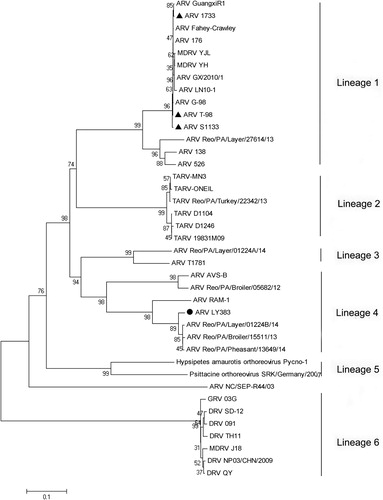
Phylogenetic and sequence analysis of σC and σB proteins
The amino acid sequences of the σC and σB proteins of LY383 were deduced from S1 and S3 coding regions, respectively. The phylogenetic tree was generated based on the amino acid sequence of the σC protein of LY383 and other 38 reference strains (). It revealed that these strains were clustered into six genotypes. In general, most strains isolated from China were grouped into genotype cluster I, which contained the reference vaccine strains. However, LY383 was closely related to the ARV field strains Reo/PA/Layer/01224B/14, Reo/PA/Broiler/1551/13, GA/14602/2014, GA/13569/2013 and GA/13542/2013, in cluster V. These strains were mainly isolated in the US in recent years (2013–2015). LY383 was obviously separated from the cluster I (vaccine strains). Pairwise distance analysis showed that LY383 shared 96.2–98.7% aa identity with field strains in cluster V. However, it was 48.6–54.3% homologous to vaccine strains.
Figure 3. Phylogenetic analysis of amino acid sequences of σC proteins between LY383 and reference ARV strains. LY383 was clustered into a distinct lineage (including US and Israel isolates) from commercial vaccine strains.
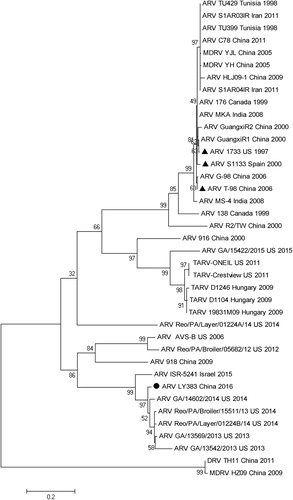
Phylogenetic analysis of the deduced amino acid sequences of σB genes showed that LY383 and 34 reference ARV strains were divided into four clusters (). Cluster II was formed by turkey-origin ARV strains, simultaneously, cluster III and IV were formed by waterfowl-origin ARV strains. All broiler/layer-origin ARV reference strains, including three vaccine strains and LY383, formed cluster I. LY383 isolate shared 94.7–98.9% aa identity with reference strains in the same cluster.
Experimental animals
To assess as accurately as possible the pathogenicity of LY383 isolate, one-day-old broiler chickens were free of common avian pathogens. Salmonella, Mycoplasma synoviae and major avian-origin viruses were absent in chickens. Serum samples of hatched chickens were prepared to detect ARV antibodies using the commercial IDEXX REO Ab Test (99-09264) (Idexx Laboratories, Westbrook, ME). All broilers for pathogenicity test were healthy and checked carefully.
Pathogenicity in broiler chickens
ARV LY383 and virulent S1133 strains were utilized to evaluate the pathogenicity in one-day-old chickens from a vaccinated breeder stock. Throughout the entire test, no deaths occurred spontaneously until 25 days of age. In group 3, the swollen footpads gradually mitigated and extended to hock joints within 36 h. Enlargement of the hock joints and articulations digitorum pedis appeared at 5 dpi and became serious. All birds in group 3 exhibited ARV-related clinical signs, including black and blue drum-sticks, growth retardation (). In addition, one chicken became paralyzed at 16 dpi. Gross lesions were observed as arthritis and tenosynovitis for hock joint and tendon. In natural infection groups, no obvious clinical signs such as limping and enlarged hock joints, occurred in chickens of group 2 during the 25-day monitoring period. Clinical signs were initially observed at 6 dpi in infected chickens (group 1), consisting of mild depression with lassitude, and palpebral oedema. Swollen joints were observed at 15 dpi and became serious, but less so than those of group 3. At the end of the experiment, six chickens were observed with obvious clinical signs (e.g. swollen hock joint and growth retardation).
Table 2. Clinical signs of broilers challenged with LY383.
Chickens having arthritis/tenosynovitis were euthanized by carbon dioxide at 25 dpi. Post mortem examination revealed tendinous synovitis and ruptured blood vessels, haemarthrosis, haemorrhage in the lungs, haemorrhaging and enlargement of the spleens, mild hepatitis and enteritis ((D–F)). Tissues (e.g. hock joint, liver and small intestine) were collected and fixed in 10% formalin buffer for histopathology. The major histopathological changes of infected chickens included infiltration of lymphocytes and macrophages in the tendons, a small amount of hepatocyte vacuolation and moderate haemorrhage accompanied by lymphocyte infiltration in the liver, villous atrophy and rupture, and mucosal smooth muscle relaxation in the small intestine.
ARV was absent in the uninfected broilers using real-time RT–PCR. Cloacal swab samples collected at 5, 10, 15, 20 and 25 dpi were tested to provide evidence of ARV shedding. In groups 1 and 3, viral RNA could be initially detected in cloacal swabs at 5 dpi and throughout the entire experiment process. In S1133 strain infected group, viral RNA could be detected early at 5 dpi and vanished at 20 dpi.
Discussion
Avian orthoreovirus is prevalent in poultry flocks worldwide and causes tenosynovitis/arthritis, and malabsorption syndrome in broiler and layer flocks. Moreover, some ARV strains were isolated in apparently healthy chickens. In general, broiler flocks vaccinated with a homologous virus may be protected against “virulent” field viruses. In recent years, tenosynovitis/arthritis syndrome has increased, and many ARV strains have been isolated from broilers in China, America, Korea, the Middle-East, and other countries (Hellal Kort et al., Citation2013; Nham et al., Citation2017; Noh et al., Citation2018). The failure of conventional vaccines, and increasing incidence of viral tenosynovitis/arthritis in the last three years means ARV has become a threat to the broiler and layer industries in North China.
In the present study, a field ARV strain LY383 was isolated from a vaccinated breeder flock in Shandong province. The incidence of tenosynovitis/arthritis in progeny broiler flocks ranged from 8% to 24%. Ten segments of LY3838 genome were sequenced by NGS, assembled, and multi-blast was performed using CLC Genomic Workbench software.
Based on the σC protein sequences, phylogenetic analysis revealed our field isolate LY383 is distinct from vaccine strains (48.6–54.3% aa identity) and other China field strains (48–55.3% aa identity). It revealed that LY383 shares low similarity with the vaccine strains. Coincidentally, LY383 is grouped into cluster V which has the lowest similarity to vaccine strains among all chicken-origin ARV isolates. Particularly, no field strain (cluster V) has been isolated in China previously. Most China ARV field strains are grouped into cluster I and are highly similar to the vaccine strains. This suggests vaccine strains can protect broilers against ARV field strains (cluster I) infection, but not the current field strain LY383. This σB could also elicit the generation of protective antibodies. The σB protein is described as a highly variable protein and carries group-specific neutralizing epitopes (Liu et al., Citation2003). Phylogenetic analysis revealed that all Chinese chicken-origin ARV field strains are highly similar to vaccine strains.
To evaluate the pathogenicity of LY383 in vaccinated chickens, a challenge test was carried out. However, broilers with tenosynovitis/arthritis appeared in LY383-infected groups (1 and 3) via intranasal or feet subcutaneous injection. Histopathological changes in the liver, small intestine and lung in infected broilers showed that LY383 could cause organ damage and physiological decline. A commercial vaccine can provide effective protection against virulent ARV strain challenge in σC genotype cluster I. The viral nucleic acid could be detected continuously in LY383-infected groups. Prolonged shedding of ARV in faeces highlights the measures to reduce the spread and control the infectious disease. Tenosynovitis/arthritis symptoms appeared to correlate with detectable viral shedding, and it might be an effective measure to eliminate diseased broilers in a timely manner.
In summary, a variant ARV strain LY383 was isolated and identified from a broiler hatched from a vaccinated breeder flock, and it can break through vaccine protection in chicks with a high level of maternal antibody. Phylogenetic analysis and challenge test suggest a new serotype ARV field strain has emerged in China which has not been described in previous studies. A large-scale epidemiological survey should be carried out to determine the prevalence of ARV genotypes. An effective vaccine is an urgent need to prevent and control this infectious disease.
Acknowledgements
HC and XD conceived and designed the experiments. HC, MY, and YT performed the experiments. YT analysed the data. HC and MY wrote the manuscript. YT and YX have done critical revision.
Disclosure statement
No potential conflict of interest was reported by the authors.
Additional information
Funding
References
- Ayalew, L.E., Gupta, A., Fricke, J., Ahmed, K.A., Popowich, S., Lockerbie, B., Tikoo, S.K., Ojkic, D., & Gomis, S. (2017). Phenotypic, genotypic and antigenic characterization of emerging avian reoviruses isolated from clinical cases of arthritis in broilers in Saskatchewan, Canada. Scientific Reports, 7, 3565. doi: 10.1038/s41598-017-02743-8
- Benavente, J. & Martinez-Costas, J. (2007). Avian reovirus: structure and biology. Virus Research, 123, 105–119. doi: 10.1016/j.virusres.2006.09.005
- Gough, R.E., Collins, M.S., Alexander, D.J. & Cox, W.J. (1990). Viruses and virus-like particles detected in samples from diseased game birds in Great Britain during 1988. Avian Pathology, 19, 331–343. doi: 10.1080/03079459008418684
- Hellal Kort, Y., Bourogaa, H., Gribaa, L., Scott-Algara, D. & Ghram, A. (2013). Molecular characterization of avian reovirus isolates in Tunisia. Virology Journal, 10, 12. doi: 10.1186/1743-422X-10-12
- Jones, R.C. (2000). Avian reovirus infections. Revue Scientifique et Technique-Office International des Epizooties, 19, 614–625. doi: 10.20506/rst.19.2.1237
- Kant, A., Balk, F., Born, L., van Roozelaar, D., Heijmans, J., Gielkens, A., & ter Huurne, A. (2003). Classification of Dutch and German avian reoviruses by sequencing the sigma C protein. Veterinary Research, 34, 203–212. doi: 10.1051/vetres:2002067
- Liu, H.J., Lee, L.H., Hsu, H.W., Kuo, L.C. & Liao, M.H. (2003). Molecular evolution of avian reovirus: evidence for genetic diversity and reassortment of the S-class genome segments and multiple cocirculating lineages. Virology, 314, 336–349. doi: 10.1016/S0042-6822(03)00415-X
- Lu, H., Tang, Y., Dunn, P.A., Wallner-Pendleton, E.A., Lin, L. & Knoll, E.A. (2015). Isolation and molecular characterization of newly emerging avian reovirus variants and novel strains in Pennsylvania, USA, 2011-2014. Scientific Reports, 5, 14727. doi: 10.1038/srep14727
- Marius-Jestin, V., Lagadic, M., Le Menec, Y. & Bennejean, G. (1988). Histological data associated with muscovy duck reovirus infection. Veterinary Record, 123, 32–33. doi: 10.1136/vr.123.1.32
- Montgomery, R.D., Villegas, P., Dawe, D.L. & Brown, J. (1986). A comparison between the effect of an avian reovirus and infectious bursal disease virus on selected aspects of the immune system of the chicken. Avian Diseases, 30, 298–308. doi: 10.2307/1590532
- Nham, E.G. Pearl, D.L., Slavic, D., Ouckama, R., Ojkic, D. & Guerin, M.T. (2017). Flock-level prevalence, geographical distribution, and seasonal variation of avian reovirus among broiler flocks in Ontario. Canadian Veterinary Journal, 58, 828–834.
- Noh, J.Y., Lee, D.H., Lim, T.H., Lee, J.H., Day, J.M. & Song, C.S. (2018). Isolation and genomic characterization of a novel avian orthoreovirus strain in Korea, 2014. Archives of Virology, 163, 1307–1316. doi: 10.1007/s00705-017-3667-8
- Petek, M., Felluga, B., Borghi, G. & Baroni, A. (1967). The Crawley agent: an avian reovirus. Archiv Fur Die Gesamte Virusforschung, 21, 413–424. doi: 10.1007/BF01241740
- Reed, L.J. & Muench, H. (1938). A simple method of estimating fifty per cent endpoints. American Journal of Epidemiology, 27, 493–497. doi: 10.1093/oxfordjournals.aje.a118408
- Simmons, D.G., Colwell, W.M., Muse, K.E. & Brewer, C.E. (1972). Isolation and characterization of an enteric reovirus causing high mortality in turkey poults. Avian Diseases, 16, 1094–1102. doi: 10.2307/1588834
- Spandidos, D.A. & Graham, A.F. (1976). Physical and chemical characterization of an avian reovirus. Journal of Virology, 19, 968–976.
- Tamura, K., Peterson, D., Peterson, N., Stecher, G., Nei, M. & Kumar, S. (2011). MEGA5: molecular evolutionary genetics analysis using maximum likelihood, evolutionary distance, and maximum parsimony methods. Molecular Biology and Evolution, 28, 2731–2739. doi: 10.1093/molbev/msr121
- Tang, Y., Lin, L., Sebastian, A. & Lu, H.G. (2016). Detection and characterization of two co-infection variant strains of avian orthoreovirus (ARV) in young layer chickens using next-generation sequencing (NGS). Scientific Reports, 6, 24519. doi: 10.1038/srep24519
- Tang, Y. & Lu, H. (2016). Whole genome alignment based one-step real-time RT-PCR for universal detection of avian orthoreoviruses of chicken, pheasant and turkey origins. Infection Genetic and Evolution, 39, 120–126. doi: 10.1016/j.meegid.2016.01.018
- van der Heide, L. (2000). The history of avian reovirus. Avian Diseases, 44, 638–641. doi: 10.2307/1593104
- van der Heide, L., Lutticken, D. & Horzinek, M. (1981). Isolation of avian reovirus as a possible etiologic agent of osteoporosis (“brittle bone disease”; “femoral head necrosis”) in broiler chickens. Avian Diseases, 25, 847-856. doi: 10.2307/1590059
- Zhong, L., Gao, L., Liu, Y., Li, K., Wang, M., Qi, X., Gao, Y., & Wang, X. (2016). Genetic and pathogenic characterisation of 11 avian reovirus isolates from northern China suggests continued evolution of virulence. Scientific Reports, 6, 35271. doi: 10.1038/srep35271