Abstract
The characteristic hoppy flavor of pale ales is known to be unstable and deteriorates during the beer’s shelf life. Metal ions, in particular iron and copper, have a prooxidative effect and therefore accelerate beer flavor deterioration. Recent studies suggest that hop constituents may complex metal ions in beer thereby suppressing their negative effect on beer flavor stability. Using benchtop wort boiling experiments and quantitating metal ions in the cast wort, this study determined that different whirlpool additions of up to 3.0 g/L hop extract or up to 17.4 g/L pellets significantly reduced metal ion concentrations in wort, especially Fe, which was reduced by a factor of up to 6 compared to the control. Based on identified dose- and product-related effects, 2.5-hL brewing trials were performed using a selection of whirlpool hopping levels with either extract or pellets revealing significant differences in resinous and sweaty flavor. The flavor of the beer hopped with 5.8 g/L pellets was perceived as 8 times more resinous and 9 times sweatier than the control revealing clear differences between extract and pellets, especially at the lower hopping levels. The beer flavor stability was predicted using ESR spectroscopy and verified by performing forced aging experiments at 30 °C and monitoring beer flavor changes by sensory evaluation. Results indicate that both extract and pellet whirlpool hopping improve beer flavor stability by reducing the formation of age-related off-flavors and by supporting the retention of flavors related to freshness with only minor variations between the two hop products.
Introduction
Hops are one of the major raw materials for brewing and are primarily used for bittering and flavor purposes during beer production. A wide range of hop products has been developed to improve brewing consistency and efficiency[Citation1] for a number of applications such as traditional kettle and whirlpool hop additions but also dry-hopping, whereby hop pellets and extracts are the most frequently used forms.[Citation2] However, it is commonly known that especially hop-derived beer properties such as bitterness[Citation3] and the characteristic floral, herbal, or citrus flavor associated with some hop varieties are not stable long-term and deteriorate over the shelf life of a beer.[Citation4,Citation5] This poses a major problem for beer shipping and storage in the brewing industry, which has not yet been sufficiently solved.
Three main factors have been identified to significantly affect the beer flavor stability, namely 1) the concentration of oxygen in the sealed container, 2) the presence of metal ions, especially Cu2+, Fe2+ and Fe3+, and Mn2+ in the product, and 3) the amount of antioxidant/antiradical compounds such as SO2 and polyphenols in the beer.[Citation6–8] The beer pH is another factor impacting flavor stability.
Over the course of the shelf life, a certain amount of ground state oxygen in the beer is transformed into reactive oxygen species resulting in the formation of hydroxyl radicals, which are highly reactive and lead to an oxidation of other compounds present in beer such as alcohols, cis-, and trans-isohomulones (isocohumulone, isohumulone, and isoadhumulone to aldehydes and ketones)[Citation9] as well as lipids.[Citation10] These reaction products are responsible for the typical stale beer flavor comprising overripe and dried fruit as well as papery and cardboard notes[Citation4,Citation5,Citation11] while the latter can also originate from oxidation during the brewing process. Thus, higher concentrations of oxygen result in a more rapid decline in beer quality over time.
Transition metal concentration is extremely important to flavor stability as the formation of hydroxyl radicals and thereby the staling of beer flavor is significantly accelerated by the presence of Fe2+, Fe3+, and Cu2+ through the Fenton (Fe2+, Fe3+) and the Haber-Weiss (Cu2+) reactions.[Citation9,Citation10,Citation12–14] Increased concentrations of Mn2+ have also been shown to decrease beer flavor stability.[Citation15] In addition, Fe3+ ions are known to form complexes with beer components that lead to haze formation.[Citation16] Metal ions in beer can originate from brewing water, malt, hops, yeast, and to a lesser extent also from metal surfaces of the brewing equipment that come into direct contact with the product.[Citation17] Compared to brewing water, malt, and yeast, hops have been shown to exhibit far higher concentrations of particular trace metal ions (e.g., Fe2+, Fe3+, and Cu2+). Nevertheless, malt plays a similarly important role as a source of metal ions due to the higher volume of malt applied for brewing.[Citation18] In general, however, most metal ions are removed from the wort as precipitates after wort boiling, further referred to as hot trub.[Citation17] It is important to note that a number of metal ions such as Ca2+, K+, and Mg2+, which primarily originate from the malt, are known to play an important role as yeast nutrients during fermentation either for yeast flocculation or as co-factors and are therefore, to some extent, desirable in wort.[Citation19–21]
There are a number of antioxidative compounds that can be formed by the yeast during fermentation. Most potent among these are thiols and sulfites, particularly SO2.[Citation13,Citation22] The antioxidant and antiradical properties of SO2 have been demonstrated in beer and occur mainly due to quenching of 1-hydroxyethyl radicals[Citation12] and as a result of its two-electron nonradical producing reaction with peroxides.[Citation23] Thus, antioxidants such as SO2 are able to increase beer flavor stability and delay staling, thereby counteracting the effects of trace metal ions and oxygen to a certain extent, which is why SO2 has been added to beer in the past. However, sulfites are considered allergens, which is why most countries have restricted or prohibited their use as food additives in recent years.[Citation24]
This leaves only the reduction of oxygen uptake during brewing and the careful selection and quality control of raw materials as factors for controlling beer flavor stability. A number of recent studies on the effect of malt-derived compounds on beer flavor stability reported mixed results. On one hand, malt-derived polyphenols were shown to significantly slow down flavor deterioration during beer storage due to their antioxidant and antiradical properties.[Citation25–27] On the other hand, Maillard reaction products containing reductone/enediol structures and originating from caramel malts and roasted malts were reported to increase radical generation in beer and thereby reducing flavor stability.[Citation28] In addition, high molecular weight compounds formed during barley and malt roasting were shown to have significant prooxidative properties, although roasted malts also contain antioxidative compounds.[Citation29] Furthermore, it has been demonstrated that the effect of malt on the beer flavor stability is highly dependent on the barley type used for malting and the growing environment.[Citation30]
In contrast, recent studies agree that compounds originating from hops are primarily beneficial for beer flavor stability. It has clearly been shown, that hop-derived polyphenols are of major importance for the beer flavor stability due to their strong antioxidative properties.[Citation7,Citation8] Wietstock et al. proposed that α-acids, iso-α-acids, and β-acids from hops cause a significant complexation of undesirable Cu2+, Fe2+, and Fe3+ ions but no complexation of Ca2+, K+, Mg2+, Mn2+, and Zn2+ ions, which are mostly desirable due to their role as yeast nutrients.[Citation31] Furthermore, it was shown that hop-derived quinone and quinoid compounds have a strong radical suppression activity, providing distinct heat protecting properties during wort boiling and in finished beer as a result of radical suppression.[Citation32] The overall evidence in recent literature suggests that the antioxidant properties of hop pellets can mostly be attributed to hop-derived polyphenols, while the antioxidant properties of hop extract are primarily a result of chelating α-acids, iso-α-acids, and β-acids. Recent studies have shown that the combination of electron spin resonance (ESR) spectroscopy and forced aging is a suitable and state-of-the-art tool to estimate and predict the flavor stability of beer by assessing the radical formation potential.[Citation6,Citation16,Citation28,Citation31–33]
Using hop extracts in brewing has multiple benefits compared to the use of kilned hop cones or pellets. These benefits include a more standardized quality, longer shelf life and less need for filtration or centrifugation to remove insoluble plant material.[Citation1] Furthermore, it has been demonstrated that supercritical CO2 extraction of hops can yield concentrated oxygenated hop oil constituents that when added to beer result in a varietal dependent intense floral, herbal, and citrus flavor as well as an increased beer bitterness.[Citation34,Citation35] The use of pre-isomerized extracts has been related to increased bitterness stability due to their higher ratio of cis-/trans-isohumulones as compared to the ratio obtained during wort boiling.[Citation36]
Thus, the objectives of this study were 1) to determine if different dosages of hop pellets or supercritical CO2 hop extract in a benchtop wort boiling system result in different concentrations of selected metal ions in wort, which are known to have an impact on beer flavor stability, 2) to apply a selection of hopping levels as pellets and extract in a pilot-scale brewing trial and to characterize their impact on the flavor of the resulting beers with a trained sensory panel, 3) to estimate the expected flavor degeneration in these beers over time by quantitating SO2 and assessing the potential for radical formation, and 4) to verify these results by monitoring changes in the flavor of the beers over 12 weeks of forced aging with a trained panel.
Experimental
Hop products
Citra® hop extract (Citra® Incognito®, 48.48% α-acids, 21.35% β-acids, < 6.00% moisture, 17.4 mL volatile oils per 100 g according to certificate of analysis), bittering hop extract (Flex®, 62.22% α-acids, 3.85% iso-α-acids, < 6.00% moisture, 21.5 mL volatile oils per 100 g according to certificate of analysis), both obtained by supercritical CO2 extraction, as well as Citra® hop pellets (Type 90, 11.50% α-acids, 3.20% β-acids, 0.10% iso-α-acids, HSI of 0.272, 8.40% moisture, 2 mL volatile oils per 100 g according to certificate of analysis) were provided by John I. Haas, (Yakima, WA). The hop materials were stored at −20 °C until use (pellets vacuum sealed).
Pilot-scale brewing process
Standard beers (pale ale) were brewed in 250 L pilot scale using 100% pale malt (Rahr Premium Pils) as described in the literature[Citation37] by modifying hop addition as follows. For all brews, a hop bittering extract (Flex®, John I. Haas, Yakima, WA) was added at the start of wort boiling targeting 20 IBU in the beer, further referred to as kettle hop addition. A second hop addition in the form of Citra® hop pellets or Citra® hop extract (Citra® Incognito®, John, I. Haas) at different dosage levels was given at the start of the whirlpool process, further referred to as whirlpool hop addition. The wort (∼14.5°P original gravity) was fermented with ale yeast (Wyeast 1056) at 68 °F (20 °C) for one week. Fermentation performance was monitored daily by measuring the decrease in apparent extract with a DMA 35 Density Meter (Anton Paar, Ashland, VA). Once diacetyl reduced below a detectable level, the beer was chilled to 2 °C and held for one week. The yeast was separated by decantation before racking into stainless steel kegs. Beers were stored in 50 L stainless steel kegs at 4 °C and CO2 concentrations were adjusted to 5.0 g/L. Concentrations of dissolved oxygen in the final beers were below 0.05 mg/L as determined using an Orbisphere 3100 portable dissolved oxygen measuring device (Hach, Loveland, CO). For performing beer aging experiments, beer samples originating from the same batch were filled into 20 L stainless steel kegs previously sanitized and purged with CO2 (oxygen below 0.01 mg/L), which were then stored at two different locations (Oregon State University, Corvallis and John I. Haas, Yakima) applying forced aging at 30 °C for up to 12 weeks. The beer flavor was evaluated in regular intervals by identically trained sensory panels at each of the two locations. Prior to sensory evaluation, the 20 L kegs were cooled at 4 °C for 24 h, and after sensory evaluation they were returned to 30 °C while maintaining constant CO2 levels of 5.0 g/L.
Benchtop wort boiling protocol
First wort (3 L) produced with the previously described pilot-scale brewing system was placed in electrically heated 5-L round flasks equipped with a water-cooled condenser and a thermometer. The wort was heated to boiling temperature and a kettle hop addition was given (Flex® bittering extract, John I. Haas). The wort was then boiled for 50 min. After turning off the heating, a whirlpool hop addition was given in the form of Citra® pellets or Citra® hop extract. The wort was then swiveled by hand every 5 min for 45 min to simulate an industrial whirlpool process while slowly cooling down to 82 °C. The cast wort was then separated from the hot trub by decantation. Wort and trub samples were collected in 45-mL Falcon tubes and stored at −20 °C prior to analysis.
Chemicals
Nitric acid (trace metal grade) and hydrogen peroxide (low trace metals) were purchased from VWR (Radnor, PA). ICP single element standards were purchased from Inorganic Ventures (Christiansburg, VA). Formaldehyde, p-rosaniline, and α(4-pyridyl-1-oxide)-N-tert-butylnitrone (POBN) were purchased from Merck (Darmstadt, Germany). Laboratory grade water (18.2 MΩ) was used for all dilutions.
Hot trub dry matter analysis
Hot trub samples were centrifuged in 45-mL Falcon tubes at 3500 rpm using a 5810 R benchtop centrifuge (Eppendorf, Hamburg, Germany). The supernatant was separated by decantation, and 2.5 g aliquots of the precipitate (trub) were weighed into aluminum moisture dishes (dried in desiccator). Trub and dish were then weighed to 0.001 g, heated to 103 − 104 °C in a forced air oven for 1 h, moved to a desiccator, and allowed to cool. After cooling, trub and dish were weighed again to 0.001 g and the dry matter in % was calculated as 100 − loss in weight (g) ×100/weight of sample (g), modified from.[Citation38]
Analysis of original gravity, extract, degree of fermentation, alcohol, pH, and color in beer
Beer samples were degassed and filtered using 113 V Cellulose folding filters (Whatman, Dassel, Germany). A 45 mL aliquot of each sample was analyzed for original gravity, real extract, degree of fermentation, alcohol, pH, and color (EBC) using an Alcolyzer ME (Anton Paar, Ashland, VA).
Quantitation of free SO2 in beer
Continuous Flow Rate Analysis (CFA) using an SAN Automated Wet Chemistry Analyzer (Skalar Systems, Graz, Austria) was applied to quantitate SO2 in beer samples using a Teflon membrane.[Citation39] The SO2 is released as gas from the beer at increased temperatures and dialyzed through the membrane into a formaldehyde solution to form an SO2-formaldehyde complex. At 45 °C, added p-rosaniline binds with the SO2-formaldehyde complex, resulting in a red complex, which was then colorimetrically analyzed at 560 nm. The resulting peak height was evaluated by applying a calibration line equation using the CFA software (Skalar Systems).[Citation28]
Determination of bitterness in beer
The bitterness of beer was determined by applying manual isooctane extraction as previously described.[Citation40]
Quantitation of metal ions in wort and hot trub via ICP-OES
Homogenized wort or trub samples (1 g, prepared as described under the section Hot Trub Dry Matter Analysis) were weighed into 4.5-mL Falcon tubes. Then 2 mL of pure nitric acid (trub) or 1:10 diluted nitric acid (wort) was added, tubes were loosely closed with screw caps and incubated for 1 h at 60 °C, then completely closed and incubated for another 23 h at room temperature. After incubation, 2 mL of water and 100 µL of hydrogen peroxide were added, and the samples were homogenized by manual shaking. Digested samples of wort and trub were further diluted using 2% nitric acid prior to analysis.
Ions of major (Ca, K, Mg, and Na) and minor (Cu, Fe, Mn, and Zn) elements were analyzed on an Arcos II ICP-OES (Spectro, Kleve, Germany) configured in axial (end-on) orientation. All standard and sample analyses were conducted as triplicate measurements with each measurement consisting of an 18 sec integration. A mixed calibration standard in 2% nitric acid was prepared using ICP single element standards. In run concentrations were determined against a six-point regression ranging in concentration from a blank through maximum calibration concentration ().
Table 1. ICP-OES calibration details.
All analyzed elements in the wort samples were within calibration limits using an 8-fold dilution. Quantitation of major and minor elements in the hot trub samples required separate dilutions of 50-fold and 10-fold, respectively.
Assessment of radical generation in beer via ESR spectroscopy
The ESR spectrophotometric system previously described[Citation28] was applied to assess the radical generation in beer by applying the spin trap reagent α(4-pyridyl-1-oxide)-N-tert-butylnitrone (POBN) and detecting formed spin trap adducts such as hydroxyethyl radicals during accelerated beer ageing (60 °C) as described in the literature.[Citation28] The ESR signal after 500 min of accelerated aging, further referred to as T500 value, was the primary parameter used to assess radical generation in this study. Sample preparation for ESR spectroscopy was performed as previously described.[Citation28]
Beer flavor evaluation
Two independent sensory panels were assembled, consisting of 8 individuals from Oregon State University and 8 individuals from John I. Haas (9 males and 7 females, age 22–63). Panelists were required to have experience evaluating beer flavor and previous experience on a descriptive panel. A room with neutral stimulus was selected for panel execution and conditions were held consistent across sensory sessions. Panelists were trained to evaluate beer flavor (orthonasal by smell and retronasal by taste) by Ultra Flash Profiling (UFP).[Citation41,Citation42] Beer samples (50 mL of each) were presented simultaneously in 250-mL beer sensory glasses sealed with lids and were evaluated at ∼15 °C. By applying UFP to the beer samples and selected reference beers, a pool of 20 attributes was developed to best represent the observed flavor differences and to represent flavor properties that are typically associated with fresh, hop-forward beer such as citrus, resinous, and grassy but also those that are associated with aged beer such as dried fruit, overripe fruit, and cardboard. Training sessions were performed using food references and aroma references for each attribute, respectively. Data collection was performed using Compusense Cloud 21.0.7713.26683 (Compusense, Canada). Separate sensory sessions were performed to evaluate the beers fresh (after maturation and diacetyl degradation), after 2 weeks, 4 weeks, 8 weeks, and 12 weeks of forced aging. The evaluations were coordinated so that the treatments were assessed on the same day at both locations (OSU, Corvallis and John I Haas, Yakima) using the beer that was stored at each site. Each sample was labeled with a three-digit random code and general randomization was utilized for the samples. The panelists were asked to assign attributes from the agreed-upon lexicon to each of the samples by using a check-all-that-apply (CATA) approach.[Citation43] For this, panelists tasted the samples and checked between 2 and 6 of the 20 attributes that best described the flavor of each sample. The outputs included the overall frequency at which each attribute was selected by the panelists to describe the flavor of the samples as reported in the form of a frequency table (CATA).
Aging scores
Overall aging scores for each beer sample and each time point of sensory evaluation during the beer aging experiment were calculated by summing the total frequencies of the age-related flavor attributes caramel, cardboard, dried fruit, honey, nutty, overripe fruit, and sweet aromatic to create an estimate for the overall amount of age-related off-flavors developed in the beer samples at each time point of evaluation. These scores were then normalized and scaled to cover a range from 0% to 100%, with 0% representing the fresh beers at time point t = 0 and 100% representing the highest amount of age-related off flavors that was observed in any of the samples over the complete period of 12 weeks forced aging. For this, the total frequencies of the respective attributes at time point t = 0 were subtracted from the summed-up frequencies at the time points 2 weeks, 4 weeks, 8 weeks, and 12 weeks to define the baseline level of these attributes for each of the fresh beers thereby creating an initial aging score of 0%. Furthermore, these baseline corrected scores were then divided by the highest overall frequency of summed up age-related attributes obtained for any of the beer samples over the storage period (frequency of 72 for the control at 12 weeks) to scale the scores between 0 and 1 or 0% and 100%, respectively.
Statistical analysis
Analysis of variance (ANOVA),[Citation44] Fisher multiple comparisons,[Citation45] Cochran’s Q test,[Citation46] and correspondence analysis (CA)[Citation47] were performed using XLSTAT (Addinsoft, v.2020.5.1).
Results and discussion
Benchtop wort boiling trial
The previously described pilot-scale brewing process was applied to produce a 250-L batch of first wort with an original gravity of 14.8 °P using 100% pale barley base malt. Then 3-L portions of this first wort were used to perform benchtop wort boiling experiments to simulate industrial wort boiling and whirlpool processes. A kettle hop addition with hop bittering extract targeting 20 IBU and a whirlpool hop addition with either hop extract or hop pellets of the widely used aroma hop variety Citra® (HBC 394) at different dosage levels were applied (). This resulted in 11 batches of cast wort including a control without whirlpool hop addition and 5 different whirlpool hopping levels with equivalent amounts of extract or pellets. The ratio of hop extract to pellets was selected based on previous experimental evidence focusing on a comparable flavor contribution of both products (1.0 g/L extract ≈ 5.8 g/L pellets, B. Buffin and M. Visgil, John I. Haas, personal communication, May 3, 2021). Each of these 11 benchtop boils was produced two additional times on separate days resulting in three independent replicates of each cast wort sample.
Table 2. Overview of the hopping levels and hop products applied for the benchtop wort boiling trials.
All cast wort and hot trub samples obtained by these benchtop wort boiling trials were processed as previously described and analyzed by ICP-OES to quantitate the metals Ca, Cu, Fe, K, Mg, Mn, Na, and Zn as ions. The concentrations obtained for the three independent replicates of each sample were then subjected to an analysis of variance (ANOVA, p = 0.05) to test for significant differences between the samples produced with extract and pellets at each dosage level for each of the metals (). Furthermore, ion concentrations of the 8 different metals were also determined in the hop extract and the hop pellets that were used for the benchtop wort boiling trial according to the same method applied for the hot trub samples (Table S1).
Figure 1. Analysis of variance (ANOVA) applied to the concentrations of selected metals quantitated in wort and hot trub samples obtained by benchtop wort boiling trials. Concentrations in hot trub were converted according to determined dry matter. Displayed are means of the three independent replicates, error bars represent standard deviations. *Significant differences (p ≤ 0.05). **Highly significant differences (p ≤ 0.001).
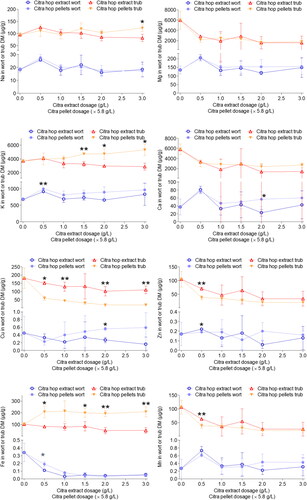
Ion concentrations in the wort samples ranged from 0.0393 µg/g Fe in ex1.5 to 996 µg/g K in pe5.8, whereas concentrations in the hot trub (dry matter) ranged from 18.3 µg/g Cu in pe17.4 to 5800 µg/g Ca in the control (Table S1). Overall, concentrations in the trub samples were significantly higher compared to those in the wort samples by a factor between 4 (Na) and 1000 (Fe). These results were well in line with recent studies indicating a separation of large quantities of metal ions in the whirlpool as part of the hot trub with only a small percentage remaining in the cast wort.[Citation17]
Neither hop dosage nor the two different hop products used for whirlpool hop addition had a significant effect on the concentrations of Mg and Na in wort and trub samples. The concentrations of Ca and Zn showed comparably high standard deviations, especially at higher hop dosages, which is why no clear dose response or hop product effect could be identified. In contrast, concentrations of K were clearly higher in the wort and trub samples produced with hop pellets with a statistical significance at some dosage levels, whereas a clear dose effect was not observed.
Concentrations of Cu were clearly higher in the wort samples produced with pellets with increasing concentrations at higher pellet dosages. For the wort samples produced with extract, an opposite trend could be observed resulting in lower Cu concentrations at higher extract dosages. Among the trub samples, those produced with pellets, however, exhibited significantly lower Cu concentrations compared to those produced with extract by a factor of 3 − 4 with an overall decrease at higher dosages for both hop products, which was more intense in the case of hop extract. This suggests an increased precipitation of Cu in the hot trub when applying hop extract compared to pellets with an increasing difference between the two products at higher dosages. While it has been suggested that the use of Cu containing antifungal agents in hop fields may result in increased Cu concentrations in hop cones,[Citation48] the hop pellets and extracts used in this study were produced from Citra® hops of the same harvest year and origin, ensuring consistent agronomic practices across all analyzed samples. Furthermore, this could not account for concentration differences of Cu by a factor of up to 4 between the samples.
A significantly higher Fe concentration was detected in the wort samples produced with pellets at lower dosage levels, which was no longer present at medium or high dosages. Among the trub samples, those produced with hop pellets exhibited significantly higher Fe concentrations by a factor of 2 − 3 compared to those produced with extract. This indicates that there was more Fe introduced to the wort by adding hop pellets. However, the majority of the Fe that was introduced as part of the hop pellets precipitated with the hot trub resulting in only minor concentration differences between the wort samples, especially at higher dosage levels.
For Mn, concentrations at lower hop dosages were higher in wort samples produced with extract, whereas at higher hop dosages, concentrations in wort samples produced with pellets were higher. However, these differences were not statistically significant and therefore inconclusive. Among the trub samples, those produced with extract overall exhibited higher concentrations of Mn, which were more or less pronounced at different dose levels.
The concentrations of all 8 quantitated metal ions in the hop pellets were many times higher than those determined in the hop extract (Table S1), especially for Ca, Fe, and Mg (by a factor of up to 2300). This can be explained by the large amount of plant material included in the hop pellets that is absent in hop extract. However, due to the relatively low volume of added hop products compared to the final wort volume, the higher amount of metal ions brought into the system by using pellets in comparison to extract did not significantly affect their concentration in the wort samples in most cases. An exception was Cu, whose concentration exhibited a significant dose-related increase by a factor of 3 when comparing the lowest (0.5 g/L) and the highest (3.0 g/L) pellet hop additions. In the case of Fe, K, and Na, there was no significant dose effect of the pellets on the concentrations in wort but higher concentrations in the trub samples were detected for pellets compared to extract, especially at higher dosages.
Overall, a direct quantitative comparison of metal ion concentrations in the trub of the different benchtop boiling trials is only possible to a limited extent due to the fact that the higher the hop pellet dosage, the higher the volume of obtained trub containing increasing amounts of hop plant material. Nevertheless, the obtained concentrations in the trub should still allow for a rough quantitative estimation of the metal ion distribution between wort and trub. Furthermore, the main focus for the following experiments lies on the metal ions in the wort, which would end up in the final beer.
Based on these observations, it can be assumed that there was an effect of whirlpool hop dosage as well as hop product on the concentration of metal ions in cast wort that was very likely to affect metal ion concentrations in beer, especially for Fe and Cu, which are known to significantly impact beer flavor stability.[Citation9,Citation10,Citation12–14] Thus, a subset of low to medium hop dosage levels was selected for a subsequent pilot-scale brewing trial to sensorially and analytically assess the specific impact of different dose levels of hop extract and hop pellets in the whirlpool on the beer flavor stability.
Brewing trial and sensory assessment
Based on the results of the benchtop wort boiling trials, a subset of six hop dosage levels (control, two dosage levels of pellets, and three dosage levels of extract) was selected to perform brewing trials at a 250 L pilot scale. Routine analysis revealed a consistent quality among the obtained beers with final ethanol concentrations of 6.39 − 6.75% vol., a final pH of 4.30 − 4.51, relative degrees of fermentation between 79.6% and 82.5%, a color of 7.8 − 8.6 EBC, and a bitterness that increased from 16 IBU to 44 IBU with increased hop dosage ().
Table 3. Original gravity, real extract, alcohol, degree of fermentation, color, pH, and bitterness in the beer samples.
The flavor of the six beers was evaluated using a CATA approach with a trained sensory panel consisting of 16 individuals and 20 predetermined flavor attributes based on reference beers and recent literature.[Citation49,Citation50] The frequency at which these attributes were used by the panelists to describe the flavor of the beers was recorded (). Overall, citrus, fruity, resinous, and sweaty were the most commonly used attributes. For resinous and sweaty, significant differences according to Cochran’s Q test (p ≤ 0.05) were identified among the beers. The control sample had a significantly lower intensity for resinous compared to samples ex0.5 and pe5.8, whereas the control and sample pe2.9 exhibited a significantly less sweaty flavor compared to sample pe5.8. Attributes floral, tropical, herbal, grassy, and sweet aromatic were used at moderate frequencies with slight differences between the beers, which were not significant. Seven attributes exhibited overall frequencies of ≤ 30% compared to the most frequent attribute citrus and were therefore least important in describing the flavor of the beers.
Table 4. Frequency of the flavor attributes selected by the panelists (N = 16) during CATA sensory evaluation of the beers produced with different whirlpool hop additions.
The beers produced with hop extract did not show a clear increase in attribute frequency at higher dosages for the descriptors that are typically associated with Citra® hops such as citrus or resinous. For most of these attributes, the frequency stayed relatively constant no matter the extract dosage, indicating that even the lowest dosage of 0.5 g/L already provided concentrations of flavor compounds that were sufficient to exceed their sensory threshold and to reach a plateau in their sensory perception. In contrast, the beers produced with pellets showed a clear dose response especially for the attributes citrus, resinous, sweaty, floral, and tropical, which were very important for the overall flavor of the beers. An increase in frequency from 7 to 9 for citrus, from 3 to 8 for resinous, from 0 to 9 for sweaty, from 4 to 6 for floral, and from 4 to 5 for tropical was observed after increasing the pellet dosage from 2.9 g/L to 5.8 g/L indicating a dose response that was not apparent with the extract. However, the sensory outcomes of the highest pellet (5.8 g/L) and extract dosages (2.0 g/L) were very similar for almost all of the 20 attributes, suggesting that in fresh beer, there are no significant flavor differences between the two hop products provided that the correct concentration ratio is used.
Evaluation of flavor stability by quantitation of SO2 and assessment of radical generation
An ESR analysis at 60 °C was applied to the beer samples to obtain T500 values as the ESR signal intensity after 500 min of forced oxygen exposure. This can be used as a measure for the radical generation potential allowing for a prediction of the beer flavor stability over time.[Citation33] Based on the results, the beers could be separated into three groups (). The control clearly exhibited the lowest flavor stability with a T500 value of 1.1 × 106. A second group consisting of beers ex0.5, ex1.0, ex2.0, and pe2.9 showed moderate T500 values of 0.62 × 106 − 0.69 × 106. Separate from all other beer samples, pe5.8 clearly exhibited the lowest T500 value of 0.48 × 106 indicating the best flavor stability between the samples. These results revealed differences in the flavor stability of the beers produced with hop extract and pellets at higher dosage levels (5.8 g/L pellets, 1.0 g/L extract) with the pellet hop addition resulting in a beer with better flavor stability at an equivalent dosage. Although resulting in the second lowest T500 value (0.62 × 106), the highest extract hop addition of 2.0 g/L (equivalent to 11.6 g/L pellets) could not reach the flavor stability of sample pe5.8. At lower hop dosages of 2.9 g/L pellets and 0.5 g/l extract, no significant differences in flavor stability between the beers could be observed. Furthermore, these results suggest that any hop addition given in the whirlpool, regardless of the type of hop product, has a positive impact on the beer flavor stability as shown by the significantly higher T500 value of the control without whirlpool hop addition compared to the other beer samples.
Figure 2. Radical generation in beer samples at forced oxygen exposure monitored over time by ESR spectroscopy at 60 °C.
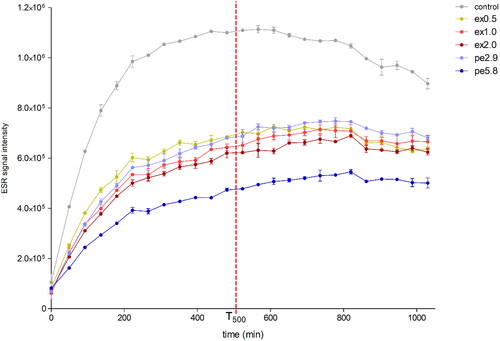
The concentration of free SO2 as determined by continuous flow analysis was ≤ 0.5 mg/L (below detection) for all six beer samples. This suggests that either there was a very low concentration of SO2 in the beers from the start or that by the time of the continuous flow analysis after approx. 3 weeks of cold storage, the majority of SO2 in the beers had already reacted by quenching of radicals despite the low oxygen concentrations. The former is more likely since it has been reported that ale yeast strains tend to produce no or lower amounts of SO2 during fermentation compared to lager yeast strains.[Citation51] These findings account for the absence of a lag phase in the ESR diagram () and indicate no significant quenching of radicals by SO2 or other antioxidative compounds in the beers from this time point on.
It is important to note that in addition to SO2, α-acids, iso-α-acids, β-acids, and especially hop-derived polyphenols are also important contributors to the antioxidative properties of beer.[Citation7,Citation8] The fact that hop pellets contain significantly higher concentrations of polyphenols than hop extract might be an explanation for the clearly lower radical generation in sample pe5.8 produced with the highest pellet dosage as detected via ESR analysis.
Beer aging experiment
To verify the results of the ESR analysis and to evaluate the specific flavor changes in the beers over time, forced aging at 30 °C was applied over a period of 12 weeks. The CO2 concentrations throughout the experiment were kept constant at 5.0 g/L. The flavor of the beers was evaluated after 2 weeks, 4 weeks, 8 weeks, and 12 weeks of forced aging by the same trained sensory panel previously applied using the same CATA method and attributes ().
Table 5. Cochran’s Q test applied to the beer sensory results obtained by CATA with a trained sensory panel over 12 weeks of forced aging.
According to recent literature[Citation50] and the sensory data presented in , the attributes caramel, cardboard, dried fruit, honey, nutty, overripe fruit, and sweet aromatic can be used to sufficiently describe the age-related off-flavors developed in beer over a period of forced or conventional aging. Combining the frequency of use for these age-related attributes, overall aging scores were calculated for each of the six beers and each time point of sensory evaluation during the beer aging experiment as previously described ().
Figure 3. Overall aging scores of the six beers produced with different whirlpool hop dosages of pellets or extract over the 12 weeks of forced aging at 30 °C displayed in % as calculated with the CATA frequencies of the age-related flavor attributes caramel, cardboard, dried fruit, honey, nutty, overripe fruit, and sweet aromatic.
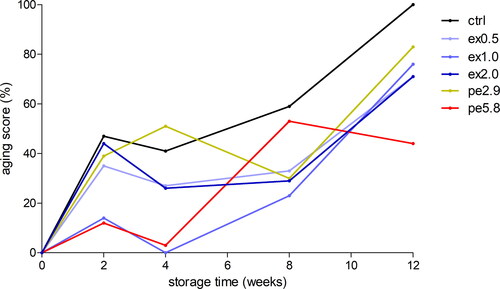
Based on these aging scores, the control beer clearly showed the highest amount of age-related off-flavors at almost every time point of evaluation, especially after the full 12 weeks of forced aging with approximately 20% more age-related flavors compared to the next highest sample pe2.9. Overall, it becomes clear from these results, that whirlpool hopping at any of the investigated dosages generally increases the flavor stability of beer, especially over longer periods of time. After 2 and 4 weeks of storage time at 30 °C, samples ex1.0 and pe5.8 exhibited clearly lower aging scores of up to 15% compared to 25 − 50% for all other treatments. However, this strong separation was no longer observable after 8 and 12 weeks of forced aging. Overall, after 12 weeks, there was a clear dose response to the intensity of developed off-flavors in the beers with those produced with higher amounts of extract or pellets exhibiting less intense off-flavors. In addition, it was revealed that the highest hop pellet dosage used to produce beer pe5.8 resulted in a significantly lower intensity of age-related off-flavors after 12 weeks with an aging score of 45% compared to 70 − 100% for all other samples. Thus, it is very unlikely that this dose-related effect was a result of masking off-flavors with increasing concentrations of hop flavor compounds since the higher dosages of extract used to produce ex1.0 and ex2.0 contributed equal or higher amounts of hop flavor compounds to the respective beers but resulted in more intense off-flavors compared to 5.8 g/L of pellets. Although the whirlpool hop additions within the applied dose range were not able to prevent the development of age-related off-flavors, they significantly delayed and reduced their formation in most cases.
To evaluate the age-related, hop dose-, and hop product-related effects on each of the assessed flavor attributes, attribute frequency data for all time points was evaluated by applying a correspondence analysis () and Cochran’s Q test (). Overall, the three factors F1, F2, and F3 displayed in the correspondence analysis biplots accounted for 57.0% of the overall variation in the data, of which F1 accounted for 33.1%, F2 for 13.4%, and F3 for 10.53%. Samples that are located in close proximity indicate overall strong similarities in flavor, whereas samples located far apart from each other indicate strong differences in flavor. Similarly, the proximity of a sensory descriptor to a sample indicates the importance of that attribute in describing the sample. The left and right parts of should be interpreted as the front view and the top view of a 3-dimensional plot with the axes F1, F2, and F3. The beer samples at the start of the experiment (t = 0) were mostly located in the upper left part of both plots closest to the attributes grassy, floral, and fruity in the left plot. In contrast, the beers produced with higher hopping dosages were located in the lower left area of the F1 vs. F2 plot closest to the attributes sweaty and resinous in accordance with previously discussed results ().
Figure 4. Correspondence Analysis (CA) and Cochran’s Q test (p ≤ 0.05) applied to the beer sensory results obtained by CATA with a trained sensory panel over 12 weeks of forced aging displayed as factors F1 vs. F2 (left) and factors F1 vs. F3 (right) overall accounting for 57.04% of the total variation.
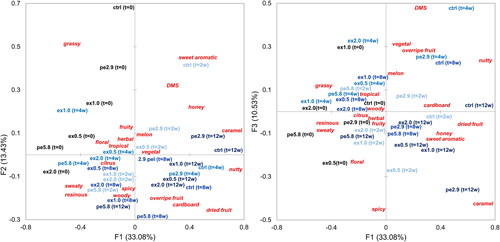
After 2 weeks of forced aging, all samples moved towards the bottom right corner in both plots and the distance between the samples decreased on axis F2, indicating stronger overall similarities compared to the initial sensory evaluation. An exception to this was the control, which was located in the upper right area of both plots close to the age-related flavor attributes cardboard, dried fruit, overripe fruit, and caramel. All other samples were characterized by a melon, fruity, herbal, tropical, sweaty, resinous, and citrus flavor, whereas the samples moved from top right (fruity, melon, and herbal) to bottom left (sweaty, resinous, woody, and spicy) with increasing hop dosage in both plots.
With increased storage time, all six samples kept moving closer towards the bottom right of the figures and the overall differences between the samples decreased, primarily on axis F2, continuing the trend already observed after 2 weeks. Thereby, the attributes overripe fruit, cardboard, dried fruit, caramel, and nutty, which are typically associated with an aged beer flavor increased over time. In contrast, attributes such as grassy, fruity, herbal, resinous, and sweaty, which were mostly present in the fresh beers decreased over time. At the final time point of 12 weeks, there were still observable differences in flavor between the six beers, namely a stronger honey-like, caramel-like, and nutty and sweet aromatic flavor in the less hopped beers, especially in the control. While the attributes resinous and sweaty that are typically associated with fresh, hop-forward beer decreased in intensity over the storage time in all samples, beer pe5.8 was clearly separated from the other beers after 12 weeks by retaining its resinous and sweaty flavor. This was in parts also the case for beer ex2.0 but the effect of retaining the flavor of the fresh beer was less intense compared to pe5.8.
By applying Cochran’s Q test, significant differences throughout the entire experiment (all 6 samples at all 5 time points) were identified for the attributes caramel, cardboard, citrus, dried fruit, resinous, spicy, sweaty, sweet aromatic, and honey (p ≤ 0.05, ). These attributes were of particular importance for evaluating which sensory changes were a result of age-related processes and which were a result of the different hopping levels and hop products applied for producing the different beers, which will be investigated in the following sections.
Two different approaches using analysis of variance (ANOVA, p ≤ 0.05) and Fisher multiple comparison were applied to the data for the 9 attributes that previously showed significant differences according to Cochran’s Q test. The first (age-related) approach divided the data into groups depending on the 5 different time points treating all 6 beers at each time point as one sample group and pairwise compared these groups to each other (). This approach allowed for an identification of the flavor attributes that were age-related and not caused by differences in applied hop dosages and hop products. Shown by a high number of pairings exhibiting significant differences between the time points, the attributes caramel, cardboard, dried fruit, and honey were clearly age-related. Furthermore, the attributes spicy and sweet aromatic showed slight differences over time that were in small parts significant. The attributes citrus, resinous, and sweaty did not show significant differences between any of the time points and were therefore considered not age-related.
Table 6. ANOVA and Fisher multiple comparison applied to the beer sensory results obtained by CATA over 12 weeks of forced aging comparing 5 different time points and excluding attributes not exhibiting significant differences between samples or time points according to Cochran’s Q test (p ≤ 0.05).
To verify these findings and to assess how the different hopping levels affected the beer samples, a second (hop dosage-related) ANOVA and Fisher multiple comparison approach (p ≤ 0.05) divided the data into groups based on type and amount of hop dosage regardless of storage time, thus treating all 5 time points for each beer as one sample group and pairwise comparing these groups to each other (). As shown by a high number of significant group pairings, the attributes citrus and especially resinous were clearly hop dosage-related as previously suggested by the lack of significant age-related differences in these attributes (). Furthermore, the attributes sweet, aromatic, and honey, in small parts showed significant differences between hop dosages, mostly between the control and the other samples.
Table 7. ANOVA and Fisher multiple comparison applied to the beer sensory results obtained by CATA over 12 weeks of forced aging comparing 6 different hop dosages and excluding attributes not exhibiting significant differences between samples or time points according to Cochran’s Q test (p ≤ 0.05).
Overall, a lower amount of significant group pairings in the hop dosage-related approach () compared to the age-related approach () revealed that the flavor differences between the same samples at different time points were slightly larger than those between the 6 different hopping treatments at each single time point. Both approaches combined, however, revealed that the attributes caramel, cardboard, and dried fruit were clearly age-related, the attributes citrus and resinous were clearly hop dosage-related and the attributes sweet, aromatic, and honey were both age- as well as hop dosage-related, even though differences in these two attributes were more subtle. The attributes spicy and sweaty exhibited neither significant age- nor hop dosage-related differences.
Conclusion/summary
This study for the first time revealed a significant effect of whirlpool hop additions with different hop products and dosages on the concentration of metal ions in cast wort, which are known to negatively impact the beer flavor stability. Higher whirlpool hop dosages of Citra® pellets or extract resulted in comparable or lower concentrations of these metals, whereas in the case of Cu and Fe, the use of extract resulted in a more effective reduction than an equivalent amount of hop pellets.
Based on the production of beers with different whirlpool hop additions on a 2.5-hL pilot scale and the evaluation of the beers with a trained sensory panel, citrus, fruity, resinous, and sweaty were identified as the most frequently used flavor attributes, while the beers produced with higher hop dosages exhibited a greater frequency of resinous and sweaty aroma. At the highest dosage levels, there were only minor differences in beer flavor between pellets and extract, even though lower pellet dosages clearly resulted in beers with a less intense flavor. An estimation of the beer flavor stability by ESR spectroscopy and quantitation of SO2 revealed a significantly increased radical formation potential in the control compared to the beers produced with extract and pellet whirlpool hop additions suggesting that whirlpool hopping in general increases beer flavor stability. The highest pellet dosage of 5.8 g/L resulted in the lowest radical formation potential and thereby the best predicted flavor stability. Although, the predicted flavor stability in general increased with higher hop dosage, the dose-related and product-related effects in the analyzed dose range were small in scale compared to the differences between the control and all other samples.
A forced aging experiment at 30 °C over 12 weeks was conducted while evaluating the beer flavor with the same panel after 2, 4, 8, and 12 weeks. Based on overall aging scores, it was shown that whirlpool hop additions in the applied dose range were clearly able to delay and reduce the formation of age-related off flavors. However, a hop dosage of 5.8 g/L pellets clearly achieved the best flavor stability compared to all other samples. The sensory evaluation revealed that all 6 beers developed a caramel-like, cardboard-like, dried fruit-like, and overripe fruit-like flavor that increased over time with a decrease of the initially characteristic attributes of citrus and resinous. The beers produced with higher whirlpool hop additions of 5.8 g/L pellets or 2.0 g/L extract initially had stronger citrus and resinous flavors, and they best retained these properties over the 12 weeks of forced aging. The development of undesired, age-related off-flavors, such as dried fruit and caramel, was significantly reduced in these more highly hopped beers. However, it is important to note that the chosen method of forced aging at 30 °C over 12 weeks is relatively intense and corresponds to at least one if not two years of regular cool storage. That is why even the beers produced with higher hopping levels of both products showed significant age-related differences over the storage time and the levels were not able to prevent them entirely.
In conclusion, the results of this study showed that extract and pellet whirlpool hop additions are both very likely to benefit beer flavor stability with slight variations. In fresh beers, the two different products resulted in no significant differences in beer flavor properties at higher dosage levels. However, after 12 weeks of forced aging, hop pellets at higher dosage were able to better retain some of the beer flavor attributes associated with fresh beer while more effectively suppressing the formation of age-related off-flavors. Compared to the control and lower hopping levels, higher dosages of extract were able to achieve a similar effect that was slightly less intense than with a corresponding dosage of pellets. Thus, the choice between pellets and extract for the same application primarily depends on technological, economical, and logistical requirements. Future studies in this area of research could provide further insights by investigating other hop varieties besides Citra® (HBC 394), by considering an even broader range of hop dosages, by applying this concept to dry-hopped beers, by investigating the specific role of polyphenols in beer flavor stability, and by intentionally adding metal ions to beer during brewing or storage to investigate their specific effects on beer aging and flavor deterioration to identify chemical interactions of prooxidative transition metals and hop or beer constituents.
Supplemental Material
Download MS Word (48.7 KB)Acknowledgments
We thank Philip Wietstock, Technical University of Berlin (Berlin, Germany), for running ESR spectroscopy and SO2 analyses. Furthermore, we thank Michael Visgil, John I. Haas, for his assistance in planning and executing this study and Jeff Clawson, Oregon State University, Department of Food Science and Technology (Corvallis, OR), for his excellent technical assistance in brewing.
Conflict of interest
The authors declare no competing financial interest.
Additional information
Funding
Literature cited
- Hughes, P. S.; Simpson, W. J. Production and Composition of Hop Products. Tech. Q. Master Brew. Assoc. Am. 1993, 30, 146–156.
- Clarke, B. J. Hop Products. J. Inst. Brew. 1986, 92, 123–130. DOI: 10.1002/j.2050-0416.1986.tb04385.x.
- Cooman, L.; Aerts, G.; Overmeire, H.; Keukeleire, D. Alterations of the Profiles of Iso-α-Acids during Beer Ageing, Marked Instability of Trans-Iso-α-Acids and Implications for Beer Bitterness Consistency in Relation to Tetrahydroiso-α-Acids. J. Inst. Brew. 2000, 106, 169–178. DOI: 10.1002/j.2050-0416.2000.tb00054.x.
- Stewart, G. G. The Chemistry of Beer Instability. J. Chem. Educ. 2004, 81, 963–968. DOI: 10.1021/ed081p963.
- Barnette, B. M.; Shellhammer, T. H. Evaluating the Impact of Dissolved Oxygen and Aging on Dry-Hopped Aroma Stability in Beer. J. Am. Soc. Brew. Chem. 2019, 77, 179–187. DOI: 10.1080/03610470.2019.1603002.
- Marques, L.; Espinosa, M. H.; Andrews, W.; Foster, R. T. Advancing Flavor Stability Improvements in Different Beer Types Using Novel Electron Paramagnetic Resonance Area and Forced Beer Aging Methods. J. Am. Soc. Brew. Chem. 2017, 75, 35–40. DOI: 10.1094/ASBCJ-2017-1472-01.
- Jaskula-Goiris, B.; Goiris, K.; Syryn, E.; Opstaele, F. V.; Rouck, G. D.; Aerts, G.; Cooman, L. D. The Use of Hop Polyphenols during Brewing to Improve Flavor Quality and Stability of Pilsner Beer. J. Am. Soc. Brew. Chem. 2014, 72, 175–183. DOI: 10.1094/ASBCJ-2014-0616-01.
- Lermusieau, G.; Liégeois, C.; Collin, S. Reducing Power of Hop Cultivars and Beer Ageing. Food Chem. 2001, 72, 413–418. DOI: 10.1016/S0308-8146(00)00247-8.
- Kaneda, H.; Kano, Y.; Osawa, T.; Kawakishi, S.; Kamada, K. The Role of Free Radicals in Beer Oxidation. J. Am. Soc. Brew. Chem. 1989, 47, 49–53. DOI: 10.1094/ASBCJ-47-0049.
- Irwin, A. J.; Barker, R. L.; Pipasts, P. The Role of Copper, Oxygen, and Polyphenols in Beer Flavor Instability. J. Am. Soc. Brew. Chem. 1991, 49, 140–149. DOI: 10.1094/ASBCJ-49-0140.
- Malfliet, S.; Opstaele, F.; Clippeleer, J.; Syryn, E.; Goiris, K.; Cooman, L.; Aerts, G. Flavour Instability of Pale Lager Beers: Determination of Analytical Markers in Relation to Sensory Ageing. J. Inst. Brew. 2008, 114, 180–192. DOI: 10.1002/j.2050-0416.2008.tb00324.x.
- Lund, M. N.; Krämer, A. C.; Andersen, M. L. Antioxidative Mechanisms of Sulfite and Protein-Derived Thiols during Early Stages of Metal Induced Oxidative Reactions in Beer. J. Agric. Food Chem. 2015, 63, 8254–8261. DOI: 10.1021/acs.jafc.5b02617.
- Zufall, C.; Tyrell, T. The Influence of Heavy Metal Ions on Beer Flavour Stability. J. Inst. Brew. 2008, 114, 134–142. DOI: 10.1002/j.2050-0416.2008.tb00318.x.
- Pohl, P. Metals in Beer. In Beer in Health and Disease Prevention. Amsterdam: Elsevier, 2009, pp 349–358. DOI: 10.1016/B978-0-12-373891-2.X0001-6.
- Jenkins, D.; James, S.; Dehrmann, F.; Smart, K.; Cook, D. The Impacts of Copper, Iron and Manganese Metal Ions on the EPR Assessment of Beer Oxidative Stability. J. Am. Soc. Brew. Chem. 2018, 76, 50–57. DOI: 10.1080/03610470.2017.1402585.
- Kaneda, H.; Kano, Y.; Koshino, S.; Ohya-Nishiguchi, H. Behavior and Role of Iron Ions in Beer Deterioration. J. Agric. Food Chem. 1992, 40, 2102–2107. DOI: 10.1021/jf00023a013.
- Wietstock, P. C.; Kunz, T.; Waterkamp, H.; Methner, F.-J. Uptake and Release of Ca, Cu, Fe, Mg, and Zn during Beer Production. J. Am. Soc. Brew. Chem. 2015, 73, 179–184. DOI: 10.1094/ASBCJ-2015-0402-01.
- Porter, J. R.; Bamforth, C. W. Manganese in Brewing Raw Materials, Disposition during the Brewing Process, and Impact on the Flavor Instability of Beer. J. Am. Soc. Brew. Chem. 2016, 74, 87–90. DOI: 10.1094/ASBCJ-2016-2638-01.
- Montanari, L.; Mayer, H.; Marconi, O.; Fantozzi, P. Minerals in Beer. In Beer in Health and Disease Prevention, Auflage: Academic Press, 2009.
- White, C. Yeast Nutrients Make Fermentations Better. White Labs. 2012. https://www.jstrack.org/brewing/Yeast_nutrition_article.pdf
- Pohl, P. Determination and Fractionation of Metals in Beer: A Review. Food Addit Contam Part A Chem Anal Control Expo Risk Assess. 2008, 25, 693–703. DOI: 10.1080/02652030701772323.
- Bushnell, S. E.; Guinard, J.-X.; Bamforth, C. W. Effects of Sulfur Dioxide and Polyvinylpolypyrrolidone on the Flavor Stability of Beer as Measured by Sensory and Chemical Analysis. J. Am. Soc. Brew. Chem. 2003, 61, 133–141. DOI: 10.1094/ASBCJ-61-0133.
- Andersen, M. L.; Outtrup, H.; Skibsted, L. H. Potential Antioxidants in Beer Assessed by ESR Spin Trapping. J. Agric. Food Chem. 2000, 48, 3106–3111. DOI: 10.1021/jf000354+.
- Guido, L. F. Sulfites in Beer: Reviewing Regulation, Analysis and Role. Sci. Agric. 2016, 73, 189–197. DOI: 10.1590/0103-9016-2015-0290.
- Mikyška, A.; Hrabak, M.; Hašková, D.; Šrogl, J. The Role of Malt and Hop Polyphenols in Beer Quality, Flavour and Haze Stability. J. Inst. Brew. 2002, 108, 78–85. DOI: 10.1002/j.2050-0416.2002.tb00128.x.
- Guido, L. F.; Curto, A. F.; Boivin, P.; Benismail, N.; Gonçalves, C. R.; Barros, A. A. Correlation of Malt Quality Parameters and Beer Flavor Stability: Multivariate Analysis. J. Agric. Food Chem. 2007, 55, 728–733. DOI: 10.1021/jf0623079.
- Kellner, V.; Mikyška, A.; Prokeš, J.; Hašková, D.; Čulík, J.; Čejka, P. The Influence of Malt Polyphenols and Individual Phenolic Substances on Beer Quality and Colloidal and Sensory Stability. Prague. Proc. Eur. Brew. Conv. Congr. Prague 2005, 61, 528–538.
- Kunz, T.; Strähmel, A.; Cortés, N.; Kroh, L. W.; Methner, F.-J. Influence of Intermediate Maillard Reaction Products with Enediol Structure on the Oxidative Stability of Beverages. J. Am. Soc. Brew. Chem. 2013, 71, 114–123. DOI: 10.1094/ASBCJ-2013-0429-01.
- Carvalho, D. O.; Øgendal, L. H.; Andersen, M. L.; Guido, L. F. High Molecular Weight Compounds Generated by Roasting Barley Malt Are Pro‑Oxidants in Metal‑Catalyzed Oxidations. Eur. Food Res. Technol. 2016, 242, 1545–1553. DOI: 10.1007/s00217-016-2655-7.
- Bettenhausen, H. M.; Barr, L.; Broeckling, C. D.; Chaparro, J. M.; Holbrook, C.; Sedin, D.; Heuberger, A. L. Influence of Malt Source on Beer Chemistry, Flavor, and Flavor Stability. Food Res Int. 2018, 113, 487–504. DOI: 10.1016/j.foodres.2018.07.024.
- Wietstock, P. C.; Kunz, T.; F.-J, M. Metal Chelation Behavior of Hop Acids in Buffered Model Systems. BrSc. 2016, 69, 56–64.
- Ting, P. L.; Lusk, L.; Refling, J.; Kay, S.; Ryder, D. Identification of Antiradical Hop Compounds. J. Am. Soc. Brew. Chem. 2008, 66, 116–126. DOI: 10.1094/ASBCJ-2008-0310-01.
- Uchida, M.; Ono, M. Improvement for Oxidative Flavor Stability of Beer—Role of OH-Radical in Beer Oxidation. J. Am. Soc. Brew. Chem. 1996, 54, 198–204. DOI: 10.1094/ASBCJ-54-0198.
- Opstaele, F. V.; Goiris, K.; Rouck, G. D.; Aerts, G.; Cooman, L. D. Production of Novel Varietal Hop Aromas by Supercritical Fluid Extraction of Hop Pellets—Part 2: Preparation of Single Variety Floral, Citrus, and Spicy Hop Oil Essences by Density Programmed Supercritical Fluid Extraction. J. Supercrit. Fluids. 2012, 71, 147–161. DOI: 10.1016/j.supflu.2012.06.004.
- Opstaele, F. V.; Goiris, K.; Rouck, G. D.; Aerts, G.; Cooman, L. D. Production of Novel Varietal Hop Aromas by Supercritical Fluid Extraction of Hop Pellets. Part 1: Preparation of Single Variety Total Hop Essential Oils and Polar Hop Essences. Cerevisia. 2013, 37, 97–108. DOI: 10.1016/j.cervis.2012.12.002.
- Jaskula, B.; Goiris, K.; Opstaele, F. V.; Rouck, G. D.; Aerts, G.; Cooman, L. D. Hopping Technology in Relation to α-Acids Isomerization Yield, Final Utilization, and Stability of Beer Bitterness. J. Am. Soc. Brew. Chem. 2009, 67, 44–57. DOI: 10.1094/ASBCJ-2009-0106-01.
- Rubottom, L. N.; Lafontaine, S. R.; Hauser, D. G.; Pereira, C.; Shellhammer, T. H. Hop Kilning Temperature Sensitivity of Dextrin-Reducing Enzymes in Hops. J. Am. Soc. Brew. Chem. 2021, 80, 75–83. DOI: 10.1080/03610470.2021.1903290.
- ASBC. Moisture, ASBC Methods of Analysis. 2008, pp. 1–2. https://www.asbcnet.org/Methods/pages/default.aspx.
- Kunz, T.; Schiwek, V.; Harms, D. Optimized Analysis Methods for the Determination of SO2 in Beer and Malt. Brauwelt Int. 2009, 27, 216–220.
- ASBC. Bitterness, ASBC Methods of Analysis. 2020, pp. 1–10. https://www.asbcnet.org/Methods/pages/default.aspx.
- Dehlholm, C.; Brockhoff, P. B.; Meinert, L.; Aaslyng, M. D.; Bredie, W. L. Rapid Descriptive Sensory Methods–Comparison of Free Multiple Sorting, Partial Napping, Napping, Flash Profiling and Conventional Profiling. Food Qual. Prefer. 2012, 26, 267–277. DOI: 10.1016/j.foodqual.2012.02.012.
- Varela, P.; Ares, G. Sensory Profiling, the Blurred Line between Sensory and Consumer Science. A Review of Novel Methods for Product Characterization. Food Res. Int. 2012, 48, 893–908. DOI: 10.1016/j.foodres.2012.06.037.
- Ares, G.; Antúnez, L.; Giménez, A.; Roigard, C. M.; Pineau, B.; Hunter, D. C.; Jaeger, S. R. Further Investigations into the Reproducibility of Check-All-That-Apply (CATA) Questions for Sensory Product Characterization Elicited by Consumers. Food Qual. Prefer. 2014, 36, 111–121. DOI: 10.1016/j.foodqual.2014.03.010.
- Larson, M. G. Analysis of Variance. Circulation 2008, 117, 115–121. DOI: 10.1161/CIRCULATIONAHA.107.654335.
- Games, P. A.; Howell, J. F. Pairwise Multiple Comparison Procedures with Unequal N’s and/or Variances: A Monte Carlo Study. J. Educ. Stat. 1976, 1, 113–125. DOI: 10.2307/1164979.
- Patil, K. D. Cochran’s Q Test: Exact Distribution. J. Am. Stat. Assoc. 1975, 70, 186–189. DOI: 10.1080/01621459.1975.10480285.
- Greenacre, M. J. Correspondence Analysis. WIREs. Comp. Stat. 2010, 2, 613–619. DOI: 10.1002/wics.114.
- Weihrauch, F.; Schwarz, J.; Sterler, A. Downy Mildew Control in Organic Hops: how Much Copper is Actually Needed. International Hop Growers’ Convention, 2011, Lublin.
- ASBC: Beer Flavor Map, Second Edition, 2017. https://www.asbcnet.org/Methods/pages/default.aspx.
- Vanderhaegen, B.; Delvaux, F.; Daenen, L.; Verachtert, H.; Delvaux, F. R. Aging Characteristics of Different Beer Types. Food Chem. 2007, 103, 404–412. DOI: 10.1016/j.foodchem.2006.07.062.
- Duan, W.; Roddick, F. A.; Higgins, V. J.; Rogers, P. J. A Parallel Analysis of H2S and SO2 Formation by Brewing Yeast in Response to Sulfur-Containing Amino Acids and Ammonium Ions. J. Am. Soc. Brew. Chem. 2004, 62, 35–41. DOI: 10.1094/ASBCJ-62-0035.