Abstract
Strawberry (Fragaria × ananassa) and papaya (Carica papaya) are susceptible to fungal plant pathogens such as Botrytis cinerea and Colletotrichum spp., which are controlled mainly by chemical fungicides. However, their use causes adverse health effects. Therefore, the search for ecological alternatives is essential to reduce the impact of pesticides. This study aimed to identify Botrytis spp. and Colletotrichum spp. isolated from strawberries and papaya fruits with symptoms of grey mould and anthracnose disease and to evaluate the in vitro antifungal activity of different fatty acids on their mycelial growth. Phylogenetic analysis allowed the identification of Botrytis cinerea and Colletotrichum nymphaeae isolated from strawberry and C. siamense from papaya, which have been reported as pathogens of both crops. The in vitro antifungal index (AI) of the fatty acids was determined at concentrations of 100, 1000, and 2000 µM. Inhibition assays showed AIs of 100% for B. cinerea, C. nymphaeae, and C. siamense using decanoic acid (1000 and 2000 µM). Sodium octanoate (1000 and 2000 µM) and hexanoic acid (2000 µM) also inhibited C. nymphaeae with an AI of 100%. This is the first report on the antifungal effects of decanoic acid on three isolated fungi, as well as sodium octanoate and hexanoic acid, on C. nymphaeae. These results suggest that these molecules could potentially control diseases caused by these fungi in strawberry and papaya fruits.
Résumé: La fraise (Fragaria × ananassa) et la papaye (Carica papaya) sont sensibles aux phytopathogènes fongiques, comme Botrytis cinerea et Colletotrichum spp., contre lesquels on lutte principalement avec des fongicides de synthèse. Cependant, leur utilisation a des effets néfastes pour la santé. En conséquence, la recherche de solutions de rechange écologiques est essentielle pour minimiser les effets des pesticides. Cette étude visait à identifier Botrytis spp. et Colletotrichum spp. isolés chez des fraises et des papayes affichant des symptômes de pourriture grise et d’anthracnose ainsi qu’à évaluer, in vitro, l’activité antifongique de divers acides gras sur la croissance de leur mycélium. L’analyse phylogénétique a permis d’identifier Botrytis cinerea et Colletotrichum nymphaeae isolés chez des fraises et C. siamense, chez des papayes, qui ont été rapportés en tant qu’agents pathogènes des deux cultures. L’indice antifongique (IA) in vitro des acides gras a été établi à des concentrations de 100, 1 000 et 2 000 µM. Des tests d’inhibition effectués avec de l’acide décanoïque (1 000 et 2 000 µM) ont donné des IA de 100% pour B. cinerea, C. nymphaeae et C. siamense. L’octanoate de sodium (1 000 et 2 000 µM) et l’acide hexanoïque (2 000 µM) ont également inhibé C. nymphaeae avec un IA de 100%. Il s’agit du premier rapport sur les effets antifongiques de l’acide décanoïque sur trois champignons isolés, de même que de l’octanoate de sodium et de l’acide hexanoïque sur C. nymphaeae. Ces résultats suggèrent que ces molécules pourraient contribuer à lutter contre ces maladies causées par ces champignons chez les fraises et les papayes.
Introduction
Strawberry (Fragaria × ananassa Duch.) is extensively cultivated worldwide because of its nutritional value, owing to the vitamins, minerals, antioxidants, macronutrients, and micronutrients it contains (Petrasch et al. Citation2019). Mexico is one of the main strawberry producing and exporting countries. In 2020, Mexico produced 5,47,823 tons, with Michoacán state being the leading producer, harvesting307464tons (SIAP Citation2020). Papaya (Carica papaya L.) is established worldwide as a crop. Papaya is rich in potassium, magnesium, vitamin B, ascorbic acid, and antioxidants (Farina et al. Citation2020). Mexico ranks 4th in global papaya production, reaching10,83,132 tons in 2019, and Colima ranked 2nd in the country with1,92,416 tons (SIAP Citation2019).
Several plant pathogens, especially fungi, cause diseases during the pre-and post-harvest stages of both crops, deteriorating agricultural products. Therefore, it is essential to identify the microorganisms responsible and determine the potential risks associated with them (Melo et al. Citation2020). Botrytis, Colletotrichum, and Rhizopus are among the genera that cause the most significant losses to strawberries (Dean et al. Citation2012; Arceo-Martínez et al. Citation2019; Petrasch et al. Citation2019). For papaya, the most important genera are Colletotrichum, Alternaria, Rhizopus, and Aspergillus (Dean et al. Citation2012; Suárez-Quiroz et al. Citation2013).
Synthetic fungicides are usually applied to inhibit the growth of these fungi and control infections (Era et al. Citation2015). However, the indiscriminate use of these compounds has been associated with adverse effects on human health, selection of fungicide-resistant pathogens, and environmental damage (Albores-Flores et al. Citation2018). Additionally, consumers demand pesticide-free products, highlighting the need to look for environmentally friendly alternatives for plant pathogen control (Darolt et al. Citation2016).
Fatty acids are molecules with vital functions in cellular metabolism (Pohl et al. Citation2011). They function as the primary metabolic fuel and gene regulators, and are essential components of cellular membranes (Schönfeld and Wojtczak Citation2016). These molecules vary in length and saturation; there are saturated or unsaturated structures with chains of 4–28 carbons (Yoon et al. Citation2018). Molecules with fewer than eight carbon atoms (C) are called short-chain fatty acids, medium-chain fatty acids contain between 8 and 12 C, and molecules with more than 12 C are long-chain fatty acids (Bazinet and Layé Citation2014). Additionally, these molecules possess antifungal activity, are biodegradable, and can be used as food additives, because they are considered safe by the United States Food and Drug Administration (Yoon et al. Citation2018).
Previous studies have reported the antifungal properties of fatty acids against plant pathogens such as B. cinerea, Cladosporium cucumerinum, Idriella bolleyi, Phytophthora infestans, Pseudozyma rugulosa, Pythium aphanidermatum, and Sphaerotheca fuliginea. The antifungal characteristics of these molecules are due to unsaturations, different ramifications, and the length of each fatty acid. These characteristics allow fatty acids to interrupt the structure of the plasma membranes of fungi, increasing their fluidity and disorder, which results in cell death (Avis and Bélanger Citation2001; Avis Citation2007).
Previous results have suggested that fatty acids differentially inhibit the mycelial growth of pathogenic fungi. Furthermore, although multiple studies have reported the antifungal activity of these molecules (Liu et al. Citation2008; Pohl et al. Citation2011), the inhibition of Botrytis sp. and Colletotrichum sp. isolates has scarcely been studied. Therefore, this study aimed to identify the pathogenic Botrytis sp. and Colletotrichum sp. and evaluate the in vitro antifungal activity of different fatty acids on fungal mycelial growth.
Materials and methods
Fungal isolation
Fungi were isolated from infected strawberry fruit tissues at the commercial ripening stage (155 days after sowing) in orchards in Zamora, Michoacán, Mexico. The first isolate (AUC-ZM-1) was obtained from a dusty mould-covered and mummified fruit, showing typical characteristics of grey mould. The second isolate (AUC-ZM-10) was obtained from fruits with anthracnose symptoms, including sunken, dark brown, circular lesions. The third isolate (AUC-TC-5) was obtained from papaya fruits collected from crops in Tecomán, Colima, Mexico, at the commercial ripening stage (220 days after transplantation). This fruit showed anthracnose symptoms, consisting of sunken, circular lesions with orange conidial masses.
Molecular identification
The internal transcribed spacer (ITS) region of the genomic DNA was amplified using the ITS5 and ITS4 primers (White et al. Citation1990). We also amplified polymorphic coding fragment genes for accurate species identification. For Botrytis sp., fragments of glyceraldehyde-3-phosphate dehydrogenase (GAPDH), heat shock protein 60 (HSP60) (Staats et al. Citation2005), and the second-largest subunit of the RNA polymerase II partial gene (RPB2) (O’Donnell et al. Citation2007) were used. For Colletotrichum spp., β-tubulin (TUB2) (Woudenberg et al. Citation2009; Groenewald et al. Citation2013;) and a GAPDH partial gene (Templeton et al. Citation1992; Guerber et al. Citation2003;) were used. The obtained sequences were assembled using BioEdit v7.2.5 software (Hall Citation1999), and compared using BLAST (NCBI) (http://blast.ncbi.nlm.nih.gov/Blast.cgi).
Trees were constructed with MEGA V7 (Molecular Evolutionary Genetics Analysis, Version 7) (Kumar et al. Citation2016). The evolutionary history was inferred using the maximum likelihood method based on the Tamura-Nei model, with 1000 bootstrap iterations (Tamura and Nei Citation1993). The analysis included 16 and 12 nucleotide sequences for the Botrytis and Colletotrichum genera, respectively. In the final dataset, there were 747 and 170 positions for the genera, respectively. Beauveria bassiana was used as the external group sequence (GenBank accession numbers: XM 008600577 and AY679162).
In vitro mycelial growth inhibition
The effect of fatty acids (Sigma-Aldrich®, Mexico) on the fungal species was determined following the methodology described by Liu et al. (Citation2008) with some modifications. A 5-mm diameter PDA-mycelium disc was placed inverted at the centre of Petri dishes (60 × 15 mm, SyM®, Mexico) filled with potato dextrose agar (PDA) (BD Bioxon, Mexico) amended with 100, 1000, or 2000 µM of hexanoic acid (6:0), sodium octanoate (8:0), decanoic acid (10:0), lauric acid (12:0), or palmitic acid (16:0) (prepared according to the manufacturer’s instructions). The previous concentrations were selected based on Liu et al. (Citation2008). Solutions were sterilized by filtration (25-mm filters, Swinnex®, USA) through 0.22 µm pore size membranes (Merck Millipore®, USA). The inoculated dishes were incubated at 25°C ± 2.0°C for 7–10 d, including the negative controls (absolute ethanol, J.T. Baker®, Mexico, for hexanoic and decanoic acid; 0.5% Tween 20 Sigma-Aldrich®, Mexico, for lauric acid; chloroform J.T. Baker®, Mexico, for palmitic acid; and distilled water for sodium octanoate). This study also included a positive control with thiabendazole (Tecto 60®, powder, 600 g i. a. kg, MSD AGVET) and an absolute control (fungus + PDA). At the end of the incubation period, colony diameter was measured using a digital Vernier caliper (Fisher Scientific®, Mexico). The antifungal index (AI) was calculated with the following equation: AI (%) = 1 – (CDtreatment/CDcontrol) × 100, where CDtreatment = colony diameter in a Petri dish with fatty acids and CDcontrol = colony diameter in a Petri dish with a negative control (Guo et al. Citation2008). Each treatment was repeated six times in two independent experiments.
Experimental design and statistical analysis
The treatments followed a completely randomized design. AI data were transformed using the function √ x + 0.5. Results were analyzed using analysis of variance (ANOVA, P ≤ 0.05), and the means were compared using Tukey’s test (P ≤ 0.05) using SPSS-IBM Statistics version 25.
Results and discussion
In this study, three fungi were isolated from strawberries and papaya with grey mould or anthracnose disease symptoms. The sequences obtained were deposited in the GenBank database under the following accession numbers: ITS (MN595028), GAPDH (MW698862), HSP60 (MW698863), and RPB2 (MW698868) for Botrytis sp. (AUC-ZM-1). The sequence MW698862 of Botrytis (GAPDH) had 100% identity with B. cinerea sequences (GenBank: MN448500, MK791186, and LC519319), which were isolated from Camellia sinensis, Arachis hypogaea, and Ocimum minimum, respectively. Phylogenetic analysis of the partial sequence of GAPDH confirmed that the isolate belonged to the B. cinerea group (). Additionally, the HSP60 (MW698863) and RPB2 (MW698868) partial genes confirmed the results for B. cinerea by having an identity of 100% for HSP60 (MT233447, MN159921, and MK791187) and 99% for RPB2 (MG846509, MH732872, and MN448501).
Fig. 1 Phylogenetic relationship of Botrytis cinerea (AUC-ZM-1) isolated from Zamora, Michoacán, Mexico, based on a region of the glyceraldehyde-3-phosphate dehydrogenase (GAPDH) gene with a cut-off value of 70%.
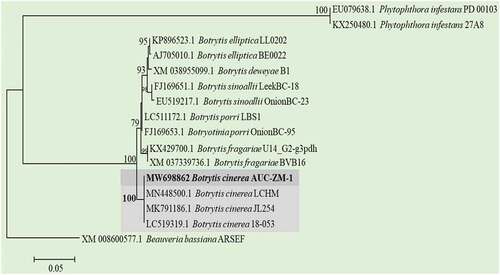
For Colletotrichum spp. (AUC-ZM-10 and AUC-TC-5), the sequences obtained were deposited in the GenBank database under the following accession numbers: ITS region (MN595025 and MN595026, respectively), GAPDH (MW698864 and MW698866, respectively), and TUB2 (MW698865 and MW698867, respectively). The MW698864 sequence of Colletotrichum sp. (AUC-ZM-10) had a 99.27% sequence identity with C. nymphaeae (GenBank: MN412439 and MN756029) isolates obtained from F. × ananassa (USA) and Capsicum annuum (South Korea), respectively. Moreover, the MW698866 sequence of Colletotrichum sp. (AUC-TC-5) had 100% identity with C. siamense sequences (GenBank: MK501893 and MK569115), which were isolated from Persea americana (Brazil) and Zea mays (China), respectively. Phylogenetic analysis of the partial sequence of the GAPDH gene confirmed that the AUC-ZM-10 isolate (accession number MW698864) belonged to the C. nymphaeae group and that the AUC-TC-5 isolate belonged to C. siamense (accession number MW698866) (). Additionally, the partial sequences of the TUB genes, MW698865 and MW698867, confirmed the C. nymphaeae and C. siamense identification, with 100% sequence identity with C. nymphaeae (AB697050 and MN304876) and C. siamense (KM885295 and KF877322).
Fig. 2 Phylogenetic relationship of Colletotrichum nymphaeae (AUC-ZM-10) isolated from Zamora, Michoacán, Mexico, and C. siamense (AUC-TC-5) isolated from Tecomán, Colima, Mexico, based on a region of the glyceraldehyde-3-phosphate dehydrogenase (GAPDH) gene with a cut-off value of 70%.
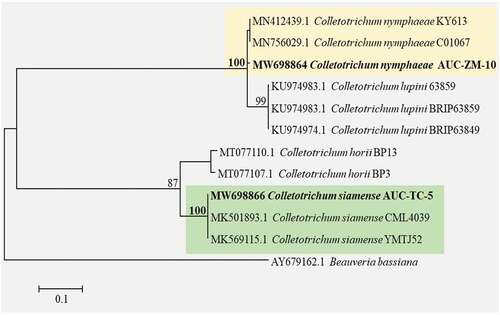
Botrytis cinerea can infect more than 200 plant species, including strawberries. It is considered one of the primary fungal pathogens of this crop globally, resulting in significant economic losses because it affects vegetative tissues, senescent organs, and fruits (Dean et al. Citation2012; Petrasch et al. Citation2019). Similarly, several authors have reported that, in recent years, C. nymphaeae, a species belonging to the Colletotrichum acutatum complex, is one of the fungal species most associated with anthracnose symptoms in various crops, such as strawberries (Nan-Yi et al. Citation2019). Moreover, C. siamense, a species belonging to the Colletotrichum gloeosporioides complex, has recently been reported as the etiologic agent of anthracnose disease in numerous crops of agricultural importance, including papaya (Zhang et al. Citation2021).
The mycelial growth of B. cinerea was inhibited by decanoic acid ( A4, A5) and lauric acid at the evaluated concentrations (P ≤ 0.05), except at 100 µM of the first acid (). Colletotrichum siamense was inhibited by hexanoic acid, sodium octanoate, and decanoic acid (P ≤ 0.05). However, only two of the evaluated concentrations of decanoic acid (1000 and 2000 µM) had an AI of 100% ( B4, B5; ). Finally, C. nymphaeae was more susceptible to the treatments because three of the fatty acids (hexanoic acid, sodium octanoate, and decanoic acid) had an AI of 100% for at least one of the concentrations used (P ≤ 0.05) ( C4, C5, C6, C7, and C8; ).
Fig. 3 Mycelial growth inhibition of Botrytis cinerea (A), Colletotrichum siamense (B), and C. nymphaeae (C) by fatty acids. Only those treatments that showed 100% inhibition are shown. A1, B1, and C1) Positive control: Thiabendazole. A2, B2, and C2) Absolute control: PDA + fungus. A3, B3, and C3) Negative control: Absolute ethanol for hexanoic and decanoic acid, water for sodium octanoate. A4 and A5) B. cinerea inhibition by decanoic acid (1000 and 2000 µM, respectively). B4 and B5) C. siamense inhibition by decanoic acid (1000 and 2000 µM, respectively). C4, C5, C6, C7 and C8) C. nymphaeae inhibition by hexanoic acid (2000 µM), sodium octanoate (1000 and 2000 µM, respectively), and decanoic acid (1000 and 2000 µM, respectively).
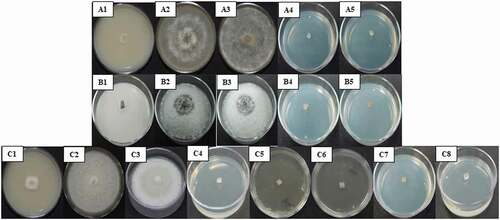
Table 1. Inhibitory effect of fatty acids on mycelial growth of Botrytis cinerea.
Table 2. Inhibitory effect of fatty acids on mycelial growth of Colletotrichum spp.
Other authors have reported the antifungal effects of saturated fatty acids on plant pathogens (Sjögren et al. Citation2003; Walters et al. Citation2003; Altieri et al. Citation2007; Leyva et al. Citation2008; Liu et al. Citation2008; Yenjit et al. Citation2010; Era et al. Citation2015; Sandoval et al. Citation2018). Here, hexanoic acid, sodium octanoate, decanoic acid, and lauric acid differentially inhibited the mycelial growth of B. cinerea, C. siamense, and C. nymphaeae. However, it is important to highlight that this is the first time the in vitro antifungal activity of fatty acids has been evaluated against fungi isolated from economically important strawberry and papaya crops in Mexico.
The most effective fatty acids were hexanoic acid, which completely inhibited C. nymphaeae; sodium octanoate, which inhibited 100% C. nymphaeae; and decanoic acid, which completely inhibited B. cinerea, C. nymphaeae, and C. siamense. These results suggest that these molecules could potentially control diseases caused by these fungi. Additionally, these molecules are commonly used as food additives because they are considered safe and biodegradable, thus representing a low risk to human health and the environment (Yoon et al. Citation2018).
In this study, short – and medium-chain fatty acids such as hexanoic acid (6:0), sodium octanoate (8:0), and decanoic acid (10:0) were the most effective against fungal growth. However, long-chain fatty acids such as lauric acid (12:0) and palmitic acid (16:0) were not as effective. Previous studies have reported that medium-chain nonanoic (9:0) and decanoic (10:0) fatty acids are the most effective antifungal agents against Paecilomyces lilacinus and P. fumosoroseus (Urbanek et al. Citation2012; Bazinet and Layé Citation2014). This effect is probably due to the low solubility of long-chain fatty acids in aqueous systems, which decreases their interaction with acyl groups of membrane phospholipids (Pohl et al. Citation2011). Therefore, long-chain fatty acids are less effective, as observed in this study.
The fungal membrane is essential for anchoring proteins and enzymes, controlling nutrient entry, and participating in signal transduction to the extracellular medium, among other functions (Avis Citation2007). Previous studies have indicated that fatty acids inhibit the growth of plant—pathogenic fungi by interacting with their cell membranes (Avis and Bélanger Citation2001). Thus, fatty acids are inserted into the phospholipid bilayer and increase their fluidity, resulting in membrane permeability changes, overall disorganization, release of intracellular components, cytoplasmic disorder, impaired energy production, protein activity, and cellular mycelial disintegration (Pohl et al. Citation2011; Li and Lee Citation2017). Previous reports have suggested that the antifungal effect of the evaluated fatty acids could be due to a mechanism of action similar to that described above. Further studies are required to confirm this hypothesis.
This study shows that the isolates obtained from strawberry were identified as B. cinerea and C. nymphaeae, while the isolate obtained from papaya was C. siamense. Fatty acids differentially inhibited the fungi evaluated. Decanoic acid completely inhibited the growth of the three fungi in at least one of the evaluated concentrations, whereas hexanoic acid completely inhibited the growth of C. nymphaeae, at the highest concentration assessed. The same inhibitory effect was observed for sodium octanoate against C. nymphaeae at the medium and highest concentrations assessed. Our results suggest that these molecules could be used to control grey mould and anthracnose in strawberry, as well as anthracnose in papaya.
Disclosure statement
No potential conflict of interest was reported by the author(s).
Additional information
Funding
References
- Albores-Flores V, Marín-Saenz IJ, López-García JA, Sánchez-Gutiérrez A, Grajales-Conesa J. 2018. Antifungal property of honey on in vitro development of Colletotrichum gloeosporioides. Mex J Phytopathol. 36:423–431. doi:10.18781/R.MEX.FIT.1805-3
- Altieri C, Cardillo D, Bevilacqua A, Sinigaglia M. 2007. Inhibition of Aspergillus spp. and Penicillium spp. by fatty acids and their monoglycerides. J Food Protec. 70:1206–1212. doi:10.4315/0362-028X-70.5.1206
- Arceo-Martínez MT, Jiménez-Mejía R, Salgado-Garciglia R, Santoyo G, López-Meza JE, Loeza-Lara PD. 2019. In vitro and in vivo anti-fungal effect of chitosan on post-harvest strawberry pathogens. Agrociencia. 53:1297–1311. https://agrociencia-colpos.mx/index.php/agrociencia/article/view/1877.
- Avis TJ, Bélanger RR. 2001. Specificity and mode of action of the antifungal fatty acid cis-9-heptadecenoic acid produced by Pseudozyma flocculosa. Appl Environ Microbiol. 67:956–960. doi:10.1128/AEM.67.2.956-960.2001
- Avis TJ. 2007. Antifungal compounds that target fungal membranes: applications in plant disease control. Can J Plant Pathol. 29:323–329. doi:10.1080/07060660709507478
- Bazinet RP, Layé S. 2014. Polyunsaturated fatty acids and their metabolites in brain function and disease. Nat Rev Neurosci. 15:771–785. doi:10.1038/nrn3820
- Darolt JC, Rocha Neto AC, Di Piero RM. 2016. Effects of the protective, curative, and eradicative applications of chitosan against Penicillium expansum in apples. Braz J Microbiol. 47:1014–1019. doi:10.1016/j.bjm.2016.07.007
- Dean R, Van Kan JAL, Pretorius ZA, Hammond-Kosack KE, Di Pietro A, Spanu PD, Rudd JJ, Dickman M, Kahmann R, Ellis J, et al. 2012. The top 10 fungal pathogens in molecular plant pathology. Mol Plant Pathol. 13(4):414–430. doi:10.1111/J.1364-3703.2011.00783.X.
- Era M, Sakai S, Tanaka A, Kawahara T, Kanyama T, Morita H. 2015. Antifungal activity of fatty acid salts against Penicillium pinophilum. Japan J Food Eng. 16:99–108. doi:10.11301/jsfe.16.99
- Farina V, Tinebra I, Perrone A, Sortino G, Palazzolo E, Mannino G, Gentile C. 2020. Physicochemical, nutraceutical and sensory traits of six papaya (Carica papaya L.) cultivars grown in greenhouse conditions in the Mediterranean climate. Agronomy. 10:501. doi:10.3390/agronomy10040501
- Groenewald JZ, Nakashima C, Nishikawa J, Shin H-D, Park J-H, Jama AN, Groenewald M, Braun U, Crous PW. 2013. Species concepts in Cercospora: spotting the weeds among the roses. Stud Mycol. 75:115–170. doi:10.3114/sim0012
- Guerber JC, Liu B, Correll JC, Johnston PR. 2003. Characterization of diversity in Colletotrichum acutatum sensu lato by sequence analysis of two gene introns, mtDNA and intron RFLPs, and mating compatibility. Mycologia. 95:872–895. doi:10.2307/3762016
- Guo Z, Xing R, Liu S, Zhong Z, Ji X, Wang L, Li P. 2008. The influence of molecular weight of quaternized chitosan on antifungal activity. Carbohydr Polym. 71:694–697. doi:10.1016/j.carbpol.2007.06.027
- Hall TA. 1999. BioEdit: a user-friendly biological sequence alignment editor and analysis program for Windows 95/98/NT. Nucleic Acids Symp Ser. 41:95–98. doi:10.14601/Phytopathol_Mediterr-14998u1.29
- Kumar S, Stecher G, Tamura K. 2016. MEGA7: molecular evolutionary genetics analysis version 7.0 for Bigger Datasets. Mol Biol Evol. 33:1870–1874. doi:10.1093/molbev/msw054
- Leyva MO, Vicedo B, Finiti I, Flors V, Del Amo G, Real MD, García-Agustín P, González-Bosch C. 2008. Preventive and post-infection control of Botrytis cinerea in tomato plants by hexanoic acid. Plant Phathol. 57:1038–1046. doi:10.1111/j.1365-3059.2008.01891.x
- Li XH, Lee JH. 2017. Antibiofilm agents: a new perspective for antimicrobial strategy. J Microbiol. 55:753–766. doi:10.1007/s12275-017-7274-x
- Liu S, Ruan W, Li J, Xu H, Wang J, Gao Y, Wang J. 2008. Biological control of phytopathogenic fungi by fatty acids. Mycopathologia. 166:93–102. doi:10.1007/s11046-008-9124-1
- Melo NFCB, de Lima MAB, Stamford TLM, Galembeck A, Flores MAP, Takaki GMDC, Medeiros JADC, Stamford-Arnaud TM, Stamford TCM. 2020. In vivo and in vitro antifungal effect of fungal chitosan nanocomposite edible coating against strawberry phytopathogenic fungi. Int J Food Sci Technol. 55:3381–3391. doi:10.1111/ijfs.14669
- Nan-Yi W, Balen FB, Peres NA. 2019. Anthracnose fruit and root necrosis of strawberry are caused by a dominant species within the Colletotrichum acutatum species complex in the United States. Phytopathology. 109:1293–1301. doi:10.1094/PHYTO-12-18-0454-R.
- O’Donnell K, Sarver BAJ, Brandt M, Chang DC, Noble-Wang J, Park BJ, Sutton DA, Benjamin L, Lindsley M, Padhye A, et al. 2007. Phylogenetic diversity and microsphere array-based genotyping of human pathogenic Fusaria, including isolates from the multistate contact lens-associated U.S. keratitis outbreaks of 2005 and 2006. J Clin Microbiol. 45:2235–2248. doi:10.1128/JCM.00533-07
- Petrasch S, Knapp SJ, van Kan JAL, Blanco-Ulate B. 2019. Grey mould of strawberry, a devastating disease caused by the ubiquitous necrotrophic fungal pathogen Botrytis cinerea. Mol Plant Pathol. 20:877–892. doi:10.1111/mpp.12794
- Pohl CH, Kock JLF, Thibane VS. 2011. Antifungal free fatty acids: a review. In: Méndez-Vilas A, editor. Science against microbial pathogens: communicating current research and technology advances. Spain: Formatex Research Center; p. 448.
- Sandoval FMG, Jiménez MR, Santoyo G, Alva MPN, López MJE, Loeza LPD. 2018. Chitosan-fatty acids composite reduces Botrytis cinerea infection on post-harvest strawberry. Nova Scientia. 10:207–227. doi:10.21640/ns.v10i21.1599
- Schönfeld P, Wojtczak L. 2016. Short- and medium-chain fatty acids in energy metabolism: the cellular perspective. J Lipid Res. 57:943–954. doi:10.1194/jlr.R067629
- SIAP. 2019. Servicio de Información Agroalimentaria y Pesquera. [Accessed 2021 Mar 21]. https://nube.siap.gob.mx/cierreagricola/
- SIAP. 2020. Servicio de Información Agroalimentaria y Pesquera [Accessed 2021 Jan 15]. http://infosiap.siap.gob.mx:8080/agricola_siap_gobmx/AvanceNacionalSinPrograma.do
- Sjögren J, Magnusson J, Broberg A, Schnürer J, Kenne L. 2003. Antifungal 3-hydroxy fatty acids from Lactobacillus plantarum MiLAB14. Appl Environmen Microbiol. 69:7554–7557. doi:10.1128/AEM.69.12.7554-7557.2003
- Staats M, van Baarlen P, van Kan JAL. 2005. Molecular phylogeny of the plant pathogenic genus Botrytis and the evolution of host specificity. Mol Biol Evol. 22:333–346. doi:10.1093/molbev/msi020
- Suárez-Quiroz ML, Mendoza-Bautista I, Monroy-Rivera JA, de la Cruz-Medina J, Angulo-Guerrero O, González-Ríos O. 2013. Isolation, identification, and antifungal susceptibility of phytopathogenic fungi in papaya cv. Maradol (Carica papaya L.). Rev Iberoam Tecnol Post. 14:115–124. http://www.redalyc.org/articulo.oa?id=81329290004.
- Tamura K, Nei M. 1993. Estimation of the number of nucleotide substitutions in the control region of mitochondrial DNA in humans and chimpanzees. Mol Biol Evol. 10:512–526. doi:10.1093/oxfordjournals.molbev.a040023
- Templeton MD, Rikkerink EH, Solon SL, Crowhurts RN. 1992. Cloning and molecular characterization of the glyceraldehyde-3-phosphate dehydrogenase-encoding gene and cDNA from the plant pathogenic fungus Glomerella cingulata. Gene. 122:225–230. doi:10.1016/0378-1119(92)90055-t
- Urbanek A, Szadziewski R, Stepnowski P, Boros-Majewska J, Gabriel I, Dawgul M, Kamysz W, Sosnowska D, Gołębiowski M. 2012. Composition and antimicrobial activity of fatty acids detected in the hygroscopic secretion collected from the secretory setae of larvae of the biting midge Forcipomyia nigra (Diptera: ceratopogonidae). J Insect Physiol. 58:1265–1276. doi:10.1016/j.jinsphys.2012.06.014
- Walters DR, Walker RL, Walker KC. 2003. Lauric acid exhibits antifungal activity against plant pathogenic fungi. J Phytopathol. 151:228–230. doi:10.1046/j.1439-0434.2003.00713.x
- White TJ, Bruns TD, Lee SB, Taylor JW. 1990. Amplification and direct sequencing of fungal ribosomal RNA genes for phylogenetics. In: Innis MA, Gelfand DH, Sninsky JJ, White TJ, editors. PCR protocols: a guide to methods and applications. Vol. 18. New York (NY): Academic Press; p. 315–322.
- Woudenberg JHC, Aveskamp MM, de Gruyter J, Spiers AG, Crous PW. 2009. Multiple Didymella teleomorphs are linked to the Phoma clematidina morphotype. Persoonia. 22:56–62. doi:10.3767/003158509X427808
- Yenjit P, Issarakraisila M, Intana W, Chantrapromma K. 2010. Fungicidal activity of compounds extracted from the pericarp of Areca catechu against Colletotrichum gloeosporioides in vitro and in Mango fruit. Postharvest Biol Technol. 55:129–132. doi:10.1016/j.postharvbio.2009.09.003
- Yoon BK, Jackman JA, Valle-González ER, Cho NJ. 2018. Antibacterial free fatty acids and monoglycerides: biological activities, experimental testing, and therapeutic applications. Int J Mol Sci. 19:1114. doi:10.3390/ijms19041114
- Zhang Y, Sun W, Ning P, Guo T, Huang S, Tang L, Li Q, Mo J. 2021. First report of anthracnose of papaya (Carica papaya L.) caused by Colletotrichum siamense in China. Plant Dis. doi:10.1094/PDIS-10-20-2154-PDN