Abstract
The metabolic syndrome is a cluster of insulin resistance, elevated blood pressure, and atherogenic dyslipidemia and is a common basis of cardiovascular diseases (CVD). Although the precise mechanism remains to be elucidated, a practical definition is needed. A worldwide definition that considers increased waist circumference as an essential component has been settled.
Visceral fat locates upstream of the liver. Free fatty acids and glycerol derived from visceral fat reach the liver and stimulate lipoprotein synthesis and gluconeogenesis, respectively. The adipose tissue produces a variety of bioactive substances conceptualized as ‘adipocytokines’. Overproduction of plasminogen activator inhibitor‐1 and tumor necrosis factor‐α seems to relate to the thrombotic and inflammatory tendency. On the other hand, adiponectin, which has antiatherogenic and antidiabetic activities, is reduced in subjects with metabolic syndrome.
In Japan, the waist circumference criterion based on visceral fat accumulation has been adopted. The concept of this syndrome has been widely publicized, and health promotion programs based on the concept have commenced in various areas of the country. Such ‘Adipo‐Do‐It’ movement is an incentive to encourage physical exercise to reduce visceral fat and is a big challenge to prevent life‐style‐related diseases and CVD.
Abbreviations | ||
AMP | = | adenosine monophosphate |
AMPK | = | AMP‐activated protein kinase |
apoB | = | apolipoprotein B |
AQP | = | aquaporin |
ARB | = | angiotensin receptor blocker |
BMI | = | body mass index |
CAD | = | coronary artery disease |
COX | = | cyclooxygenase |
CT | = | computed tomography |
CVD | = | cardiovascular disease |
EGF | = | epidermal growth factor |
ERK | = | extracellular signal‐regulated kinase |
fat | = | ROS fat reactive oxygen species |
FFA | = | free fatty acids |
HB‐EGF | = | heparin‐binding EGF‐like growth factor |
HDL‐C | = | high‐density lipoprotein cholesterol |
hs‐CRP | = | high‐sensitivity C‐reactive protein |
IDF | = | International Diabetes Federation |
KO | = | knockout |
LDL | = | low‐density lipoprotein |
MAP | = | mitogen‐activated protein |
MetS | = | the metabolic syndrome |
NCEP | = | National Cholesterol Education Program |
NF‐κB | = | nuclear factor‐kappa B |
NO | = | nitric oxide |
PAI‐1 | = | type 1 plasminogen activator inhibitor |
PPARγ | = | peroxisome proliferator‐activated receptor‐γ |
SREBP | = | sterol‐responsive element‐binding protein |
TIMP | = | tissue inhibitor of metalloproteinase |
TNF | = | tumor necrosis factor |
VCAM | = | vascular cell adhesion molecule |
VLDL | = | very low‐density lipoprotein |
WHO | = | World Health Organization |
WT | = | wild‐type |
Introduction
Cardiovascular diseases (CVD) are the major causes of morbidity and mortality in the world. Numerous epidemiological and basic scientific studies have indicated that hypercholesterolemia and elevated serum levels of low‐density lipoprotein (LDL) are major risk factors for CVD. Clinical trials have demonstrated that LDL‐lowering therapy can reduce major cardiovascular events and mortality. The secondary target of CVD prevention is the multiple risk factor syndrome, representing clustering of mild dyslipidemia, hyperglycemia, and hypertension in individuals, which is increasing throughout the world.
Obesity is a well known major risk factor for hyperlipidemia, diabetes, and hypertension. However, not all obese subjects have such disorders and, on the other hand, mildly obese subjects sometimes have multiple comorbidities. Recent clinical research demonstrated that the distribution of body fat rather than the total amount of fat is related to obesity‐linked disorders. Central obesity, especially accumulation of intra‐abdominal visceral fat, has been identified as a common basis of dyslipidemia, hyperglycemia, hypertension, and CVD based on clustering of multiple risks. At present, there is recognition that people should distinguish body fat accumulation, especially visceral fat accumulation accompanied by various metabolic disorders, from simple obesity as a body status.
Recent progress in biological research has uncovered the mechanism of differentiation and functions of adipose tissue. The most striking finding in this field is that adipose tissue is not a simple energy storage organ but rather a large endocrine organ that produces and secretes various bioactive substances called ‘adipocytokines’. Several experimental and clinical studies have demonstrated that the development of obesity‐related comorbidities and CVD are directly related to dysregulation of various adipocytokines associated with visceral obesity.
With the background of life‐styles such as physical inactivity and overnutrition, the prevalence of the multiple risk factor syndrome is increasing explosively even in East Asia including China. From this world situation, together with a better clinical and scientific understanding of obesity, the International Diabetes Federation (IDF) published the definition of ‘the metabolic syndrome’ (MetS) on the basis of central obesity (visceral fat accumulation) that is associated with multiple comorbidities, to prevent CVD Citation1. Further scientific perception and appreciation of adipocytokines and obesitopathy may help people to change their life‐styles and to reduce life‐style‐related comorbidities worldwide. Here, we review the clinical concepts and molecular mechanisms of MetS.
Key messages
The metabolic syndrome is a common basis of cardiovascular diseases with multiple atherogenic risk factors, increasing in the countries where overeating and physical inactivity are common life‐styles. A clinical definition of the metabolic syndrome is increased waist circumference plus two or more comorbidities (not simple obesity).
Visceral fat locates upstream of the liver, and free fatty acids and glycerol derived from visceral fat reach the liver, resulting in stimulation of lipoprotein synthesis and gluconeogenesis, respectively. Dysregulation of adipocytokines, such as overproduction of PAI‐1 and TNFα, and reduction of adiponectin, may be one of the molecular mechanisms of the metabolic syndrome.
Risk factor‐oriented (not simply obesity‐oriented) and scientific health promotion programs based on the concept of the metabolic syndrome will encourage physical exercise to reduce visceral fat and will help to prevent life‐style‐related diseases and consequently to minimize the risk of cardiovascular diseases.
Introduction of computed tomography scanning in obesity research and visceral fat obesity
Obese people with body mass index (BMI) greater than 30 kg/m2 are rare (approximately 2% of the general population) in East Asia including Japan. However, this frequency provoked us to pay more attention to the pathological consequences of obesity. We identified a considerable number of people with plasma glucose, lipids, or blood pressure levels that were almost normal or abnormal but much milder than would be otherwise expected in extremely obese subjects. Even when these individuals had hyperglycemia, dyslipidemia, or hypertension, these abnormalities improved markedly after only 3%–5% weight reduction despite persistence of obesity. Thus, it seemed that body weight itself does not simply determine the abnormalities in glucose, lipids, and blood pressure; i.e., body weight itself does not reflect the precise amount or properties of adipose tissue in the body. Our group introduced computed tomography (CT) scanning for precise evaluation of adipose tissue, because this imaging technique can detect adipose tissue. The amount of subcutaneous adipose tissue in the body was calculated from multi‐slice CT images Citation2. In spite of these efforts, we found no significant difference in the relationship between clinical abnormalities and the amount of adipose tissue by CT, compared with the relationship between medical parameters and BMI. However, this method was critically distinct from other techniques used for evaluation of body fat, such as impedance method, in the point that CT scan can be used for assessment of the amount of fat in the body cavity. Some obese subjects possess significant amount of tissue in the abdominal cavity with CT values similar to subcutaneous fat (), although there is considerable variation in abdominal fat distribution in the subjects. For example, some subjects have vast amounts of subcutaneous fat, while others have excess fat in the intra‐abdominal region but little subcutaneous fat. Our group investigated the relationship between abdominal fat distribution and medical health problems and found that subjects with intra‐abdominal fat had predominantly higher plasma glucose levels in glucose tolerance tests, higher plasma triglyceride levels, and higher blood pressure levels, compared with those with a predominant accumulation of subcutaneous fat Citation3,4. We designated such type of obesity, i.e. predominant accumulation of intra‐abdominal fat, as ‘visceral fat obesity’ to discriminate it from ‘subcutaneous fat obesity’ Citation5.
Syndrome X, deadly quartet, and visceral fat syndrome
In the late 1980s, a cluster of multiple risk factors in one individual, which was termed ‘multiple risk factors syndrome’, focused attention on beyond the cholesterol point of view. Reaven Citation6 at Stanford University proposed ‘syndrome X’, and Kaplan Citation7 at Texas University proposed ‘deadly quartet’. Subsequently, DeFronzo Citation8 at Texas University proposed a similar pathological entity as the ‘insulin resistance syndrome’.
In the meantime, research on obesity revealed that the site of fat accumulation, rather than the grade of obesity, is a significant determinant of various morbid conditions. The link between specific body fat distribution and development of morbid conditions that could lead to CVD was first proposed by Vague at Marseille University. In 1947, Vague Citation9 stated that android obesity, which represents fat accumulation in the upper body, posed a higher risk for diabetes mellitus than gynoid‐type obesity, which represents fat accumulation in the lower body. Subsequently in the 1980s, Kissebah Citation10 proposed upper body obesity based on the waist/hip ratio and reported its significance on the development of insulin resistance and lipid abnormality. Furthermore, Bjorntorp Citation11 described the concept of central obesity and stressed its significance for CVD.
Our group estimated the fat distribution in patients with coronary artery disease (CAD). We noted visceral fat accumulation even in people with within normal BMI. The area of visceral fat in approximately 40% of patients with CAD was greater than 100 cm2 (), although the subcutaneous fat was very thin in such patients. These individuals were positive for multiple coronary risk factors, such as hypertension, glucose intolerance, and dyslipoproteinemia Citation12. Thus, visceral fat accumulation is an attractive concept underlying CVD through the development of multiple risk factors. We thus proposed the concept of ‘visceral fat syndrome’ as a multiple risk factor clustering syndrome. Visceral fat syndrome is a condition compatible with ‘syndrome X’ or ‘deadly quartet’.
Characteristics of visceral fat
Adipose tissue provides free fatty acids (FFA) through lipolysis. Abnormality of FFA metabolism in upper body obesity is considered a cause of some metabolic defects such as dyslipidemia, insulin resistance, and vascular endothelial dysfunction. It has been reported that abdominal subcutaneous fat is lipolytically more active than lower‐body subcutaneous fat, and the excess release of FFA from abdominal subcutaneous fat may contribute to the abnormalities described in MetS Citation13. The amount of intra‐abdominal visceral fat is smaller than that of subcutaneous fat and thus the amount of FFA released by the visceral fat must contribute only a small percentage of systemic FFA delivery. However, clinical analyses of body fat distribution by CT scan revealed that glucose intolerance, plasma triglyceride level, and blood pressure level are closely related to the ratio of visceral fat area/abdominal fat area Citation3,4. Thus, the anatomical characteristics of visceral fat seem to relate to the development of these metabolic disorders. Visceral adipose tissue is located in the mesentery, where innumerable vessels run from the digestive tract to the liver and temporarily reserve excess energy as triglyceride. In response to energy demand, visceral adipose tissue quickly hydrolyzes triglyceride. FFA and glycerol released from visceral fat reach the liver via the portal vein (). FFA is re‐esterified to triglyceride and delivered to the systemic circulation as very low‐density lipoprotein (VLDL). Glycerol is used for gluconeogenesis in the liver. FFA derived from VLDL and glucose are used in various tissues in the body. The above background indicates that visceral fat is a specially developed adipose tissue and acts as a temporary energy reservoir to respond quickly to energy demand.
Figure 3. Visceral fat accumulation and hyperlipidemia. A large amount of FFA reaches the liver where it activates triglyceride(TG) synthesis. FFA inhibits intrahepatic degradation of apoB, resulting in overproduction of VLDL. Prolonged overnutrition causes lipid accumulation in visceral fat, liver, and muscle, resulting in insulin resistance.
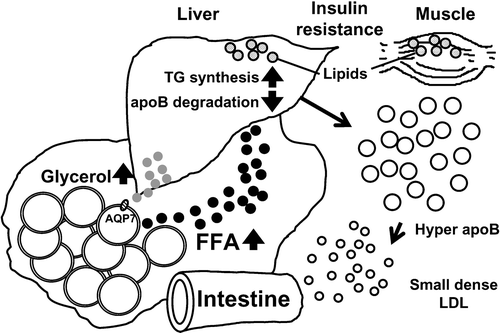
Overaccumulation of visceral fat and the large amounts of FFA result in acceleration of re‐esterification of triglycerides. Acyl Coenzyme A (CoA) synthase, which activates FFA, is upregulated in a model of visceral fat accumulation Citation14. A large proportion of apolipoprotein B (apoB), a major apolipoprotein of VLDL, is degraded in hepatocytes after synthesis through the normal pathway. However, it is reported that some FFA, such as oleate, inhibit the degradation of apoB, with consequent overproduction of VLDL Citation15. Since one particle of VLDL contains one molecule of apoB and many triglycerides, the plasma level of apoB is elevated (hyperapobetalipoproteinemia), the resultant LDL becomes small and dense (small dense LDL) after lipolysis.
Glycerol released from visceral fat is delivered to the liver. The molecular mechanism of glycerol transport across the cell membrane is not clear at present. Aquaporin (AQP) adipose, which we identified in the human adipose cDNA project and later found to be human AQP7, belongs to the aquaglyceroporin subfamily, and its main function is the control of cell membrane permeability to glycerol as well as water. Disruption of AQP7 causes disturbance of the normal rise of plasma glycerol in human and profound hypoglycemia during prolonged fasting in mice because of impaired glycerol supply to the liver Citation16. AQP7 is overexpressed in visceral fat accumulation, accompanied by portal hyperglycerolemia and systemic hyperglycemia Citation17.
Subcutaneous fat is necessary for long‐term storage of excess energy and for maintenance of insulin sensitivity. Genetic or acquired loss of normally developed subcutaneous fat causes lipodystrophic diabetes and accumulation of fat in the liver and muscle Citation18. Development of subcutaneous fat is an active process in infancy, adolescence, and pregnancy. Overeating at these ages results in massive obesity. After adolescence in men and in postmenopausal women, exposure to overnutrition does not result in effective storage of excess energy in subcutaneous fat. Visceral fat must play a role in storage of excess energy in these ages, though its capacity is somewhat limited because of the narrow intra‐abdominal space. Normally, the liver and muscle quickly take up glucose and store energy as glycogen. Accumulation of visceral fat enhances VLDL synthesis. Prolonged hyperinsulinemia further activates a series of enzymes in FFA synthesis in the liver via upregulation of the transcriptional factor sterol‐responsive element‐binding protein (SREBP)‐1c, resulting in fatty liver. Lipids also accumulate in skeletal muscles. In such circumstances, organs do not have enough capacity to take up plasma glucose in spite of very high levels of plasma insulin, subsequently resulting in insulin resistance. Genetic factor(s) will modify these processes to enhance the development of insulin resistance in individuals. To disrupt this scenario, the capacity for glucose storage must be recovered by diet or exercise.
Substantial data have also accumulated in support of the roles of various adipocyte‐derived factors in the development of insulin resistance in MetS.
Systemic survey of genes expressed in adipose tissue and adipocytokines
In addition to the localization and functional properties of visceral fat, there is evidence linking certain molecules in visceral fat to human disorders, especially CVD. What is the profile of molecules or genes expressed in subcutaneous and visceral fat? To answer this question, our group in collaboration with the human body map project team investigated the gene expression profile in adipose depots during the early 1990s Citation19. Surprisingly, we found that adipose tissues, especially visceral fat, expressed a variety of genes for secretory proteins including complement factors in the immune system, growth factors, and cytokines (). We found type 1 plasminogen activator inhibitor (PAI‐1) and heparin‐binding epidermal growth factor (EGF)‐like growth factor (HB‐EGF) in the visceral fat cDNA library. Accumulated visceral fat overproduces and secretes PAI‐1, which in turn raises the risk for thrombotic disorders Citation20. Thus, it seems that visceral fat is directly linked with CVD. We named collectively these adipocyte‐derived molecules as ‘adipocytokines’ Citation21. The ‘visceral fat syndrome’ may accelerate atherosclerosis through dysregulation of adipocytokines as well as clustering of coronary risk factors. The Harvard group of Spiegelman and coworkers Citation22 was the first to describe the contribution of adipocyte‐derived factors to obesity‐related disorders. The same group investigated changes in the cytokine profile during differentiation of adipocytes, and found overexpression of tumor necrosis factor (TNF)‐α in the adipose tissue of insulin‐resistant obese animals. They also demonstrated that blockade of TNFα improved insulin resistance. At Rockefeller University, the group led by Friedman Citation23 discovered a gene for congenital obesity in mice, leptin, which is a circulating factor derived from adipocytes and transmits a satiety signal.
Figure 4. Profile of genes expressed in human visceral fat. Genes expressed in human visceral fat were categorized according to function or subcellular localization. Approximately 30% of genes were those encoding secretory proteins.
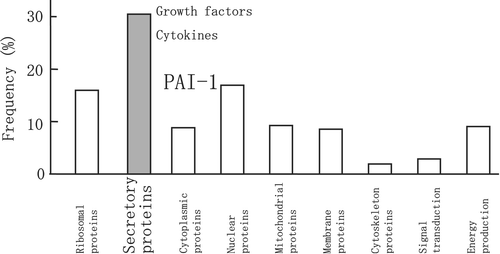
Every type of cell produces and secretes a variety of bioactive substances to control the function of the cells themselves or the surrounding environment. A single adipocyte may secrete a small amount of adipocytokines, but the total amount of adipocytokines becomes a considerable amount because the adipose tissue is one of the largest organs in the body. In this regard, the production of adipocytokines varies in response to energy balance, and simple blood tests could provide important information on abnormal synthesis and production.
Discovery of adiponectin and the concept of hypoadiponectinemia
Up to 70% of human genes had not been registered in the ‘Genbank’ database at the time of search for genes expressed in the adipose tissue. We tried to pull out unknown ‘adipocytokines’ related to the development of CVD. Through a systematic analysis of adipose‐expressed genes, we discovered a novel gene for adipocyte‐derived secretory protein, lately named ‘adiponectin’. Adiponectin is specifically expressed in the adipose tissue Citation24. The molecule has two domains, namely a collagen‐like fibrous domain and a C1q‐like globular domain. The single molecules combine and form a high‐ordered structure Citation25. Regarding its effects, adiponectin has two interesting features: 1) Its plasma concentrations decrease with increase of BMI and upon accumulation of abdominal fat Citation25,26, in contrast to PAI‐1 (); 2) The protein binds to collagens I, III, and V, which are present in the subendothelial intima Citation27. In fact, adiponectin adheres to endothelium‐injured arterial walls. This is the reason why we named this protein ‘adiponectin’.
Figure 5. Correlation between CT‐determined visceral fat area and plasma levels of adiponectin and plasminogen activator inhibitor type 1 (PAI‐1). Plasma adiponectin levels correlated negatively, and plasma PAI‐1 levels correlated positively with the visceral fat area determined by CT scan.
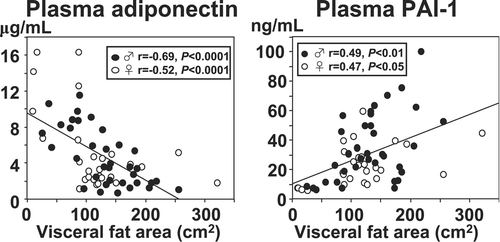
Subsequent studies identified several functions for the adipocyte‐derived protein. Adiponectin suppresses: 1) TNFα‐induced expression of adhesion molecules in vascular endothelial cells by inhibiting the nuclear translocation of nuclear factor‐kappa B (NF‐κB) Citation28,29; 2) growth factor‐induced proliferation of smooth muscle cells by inhibiting classical mitogen‐activated protein (MAP) kinase pathway Citation30; and 3) foam cell transformation and secretion of TNFα by macrophages Citation31,32 (). On the other hand, adiponectin induces the expression of tissue inhibitor of metalloproteinase (TIMP)‐1, which suppresses matrix metalloproteinase and inhibits matrix degradation to increases plaque stability Citation33. Overexpression of adiponectin in apoE knockout (KO) mice downregulated the expression of vascular cell adhesion molecule (VCAM)‐1, class A scavenger receptor, and TNFα mRNA in the aorta, and retarded the progression of fatty streak lesion Citation34. Adiponectin KO mice developed severe neointimal thickening following traumatic injury by a catheter wire Citation35. Recent studies also demonstrated that adiponectin protects the heart from ischemia‐reperfusion injury Citation36. At least part of these effects is mediated through adenosine monophosphate (AMP)‐activated protein kinase (AMPK)‐ or cyclooxygenase (COX)‐2‐dependent pathway. These observations confirmed that adiponectin suppresses the development of atherosclerotic CVD. Recently, the high‐sensitivity C‐reactive protein (hs‐CRP), an inflammatory marker, was identified as a novel risk factor for both CVD and type 2 diabetes, and adipose tissue expression levels of TNFα and CRP were augmented in adiponectin KO mice Citation37.
Figure 6. Effects of adiponectin on vascular endothelial cells, vascular smooth muscle cells, and macrophages. Administration of adiponectin suppressed TNFα‐induced expression of VCAM‐1 in endothelial cells through the inhibition of NF‐κB activation, platelet derived growth factor (PDGF) induced proliferation of vascular smooth muscle cells partly by inhibition of ERK activation, and lipopolysaccharide‐induced expression and secretion of TNFα.
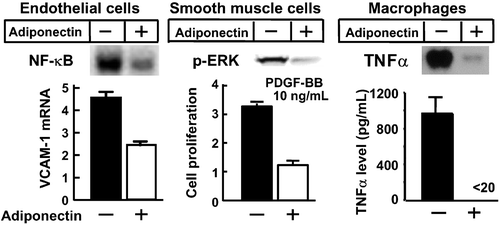
Clinically, plasma adiponectin levels are significantly lower in patients with CVD than in BMI‐matched controls Citation28. The association with CAD in individuals with plasma adiponectin level less than 4 µg/mL is twice that in the general population Citation38. The predictive effect of hypoadiponectinemia on CVD was first documented in patients with end‐stage renal disease Citation39. A study of 18,000 US men concluded that serum adiponectin level is a negative risk factor for myocardial infarction Citation40. Hypoadiponectinemia is also related to an increased risk of 5‐year mortality after ischemic stroke, independent of other risk factors Citation41. Plasma adiponectin levels correlated positively with insulin sensitivity determined by glucose clamp test in humans and nonhuman primates Citation42,43. The adiponectin KO mice develop severe insulin resistance compared with wild‐type (WT) mice Citation44. Pima Indians with high plasma adiponectin at baseline had low risk of new development of diabetes mellitus Citation45. Adiponectin has dual functions as it protects against insulin resistance and atherosclerosis. Basically, hypoadiponectinemia is caused by accumulation of visceral fat. However, phenotypic analyses of hypoadiponectinemia caused by the mutation(s) in the adiponectin gene (primary hypoadiponectinemia) will provide valuable information to understand the function(s) of this molecule. Subjects carrying a mutation of isoleucine 164 to threonine in the adiponectin gene show disturbed secretion of this mutant protein and had severe hypoadiponectinemia Citation46. Such subjects with genetic hypoadiponectinemia are also often hypertensive, hyperlipidemic, diabetic, and develop CVD Citation47.
Adiponectin activates AMP kinase and Akt, and subsequently phosphorylates endothelial nitric oxide (NO) synthase in vascular endothelial cells Citation48,49. The endothelium‐dependent vasodilation is disturbed in hypoadiponectinemia Citation50. Adiponectin KO mice develop salt‐sensitive hypertension with reduced mRNA levels of endothelial NO synthase and prostaglandin I2 synthase in the aorta Citation51 and low plasma levels of their metabolites. A more striking feature of adiponectin in hypertension is that reduction of adiponectin causes severe cardiac hypertrophy, resulting from the reduction of AMPK signaling and increase of extracellular signal‐regulated kinase (ERK) in the myocardium Citation52.
Physical inactivity and a high fat/low dietary fiber diet are known to be associated with some types of cancers. Several studies have emphasized the significant association between hypoadiponectinemia and increased risk for breast Citation53,54, endometrial Citation55, prostatic Citation56, and colorectal cancer Citation57. Recently, it has been reported that adiponectin‐deficient mice increased hepatic oxidative stress and frequently developed hepatic tumors when they were fed with a choline‐deficient l‐amino acid‐defined diet, which induced steatosis in the liver Citation58. Future studies are necessary to clarify the causality and underlying mechanisms of hypoadiponectinemia and its potential role in obesity‐related cancers.
Reduction of visceral fat improves dysregulation of adipocytokines. Furthermore, regimens that increase plasma adiponectin may be useful for prevention of CVD. At least three types of regimens with different mechanisms to raise plasma adiponectin level have been reported: 1) A selective cannabinoid‐1 receptor blocker, rimonabant, has been shown to reduce waist circumference and improved metabolic risk factors including plasma adiponectin levels in high‐risk obese subjects Citation59; 2) Unclassical sequence of the peroxisome‐proliferator‐responsive element (PPRE) was identified in adiponectin promoter Citation60. Peroxisome‐proliferator‐activated receptor‐γ (PPARγ) and its ligand, thiazolidinedione, markedly enhanced adiponectin promoter activity and increased the plasma level of adiponectin Citation61; and 3) Recent studies demonstrated that reactive oxygen species generated in obese adipose tissue designated as fat reactive oxygen species (fat ROS) can cause dysregulation of adipocytokines Citation62,63. The amount of ROS in urine correlates well with the amount of visceral fat. Administration of angiotensin receptor blocker (ARB), which is known to reduce oxidative stress in organs, did not change plasma adiponectin levels in lean, but resulted in recovery of dysregulation of plasma adiponectin in obesity in association with reduction of fat ROS in rodents Citation64. Hypoadiponectinemia could be a novel risk factor in the era, in which people are exposed by overnutrition Citation65. Life‐style changes and regimens designed to improve hypoadiponectinemia are potential strategies to prevent CVD. Further clinical evidences for the beneficial effects of correction of hypoadiponectinemia are anticipated in the future.
Establishment of the definition of metabolic syndrome
The modern life‐style characterized by overnutrition and reduced physical activities is rapidly expanding across the world. The World Health Organization (WHO) declared prioritization of prevention of CVD in the new global health care strategies in the 2002 World Health Report Citation66. While continuing the supply of food and nutrients together with anti‐infection control in developing countries where the average life span is short, the WHO has become aware that health care strategies targeting CVD can further prolong the life span of people. This conceptualization is based on the increase in the incidence of CVD in advanced countries as well as in Asian countries, especially China, due to overnutrition resulting from contentment diet and low physical activity.
The concept of multiple risk factor syndrome arising from visceral obesity has been incorporated within the concept of MetS proposed by the National Cholesterol Education Program (NCEP), and the concept of insulin resistance syndrome is the backbone of the concept of MetS defined by WHO. In such circumstances, it has become necessary also in Japan to establish diagnostic criteria for MetS that are scientifically based and convenient for clinical use. In 2004, a joint working committee for the diagnostic criteria of MetS was established with participation of representative members from eight scientific committees. The Working Committee has thus defined MetS as visceral fat accumulation expressed as gain in waist circumference plus at least two of three conditions: hypertension, dyslipidemia and hyperglycemia. The NCEP and WHO committees harmonized their work and a worldwide definition of MetS was published by IDF in 2005. The definition in Japan is basically similar to the worldwide definition. However, there are two characteristic points in the diagnostic criteria for MetS in Japan ().
Table I. Worldwide criteria and Japanese criteria for the diagnosis of the metabolic syndrome.
The primary goal of defining MetS is to diagnose subjects with visceral fat accumulation among those with two or more risk factors, improve the risk factors, and prevent CVD by reduction of visceral fat. Sometimes such patients need drug therapy. Any such effort should also include efforts to reduce visceral fat for safe therapy. Therefore, the presence of a large waist circumference, indicative of visceral fat accumulation, is a prerequisite for diagnosis. Needless to say, subjects with multiple coronary risk factors without visceral fat accumulation must be managed appropriately, but those individuals may require multiple therapeutic regimens.
The worldwide definition of MetS recommends that a cutoff point of waist circumference should be set for each ethnic population. Generally, cutoff points for the circumference for men and women are set separately. On the other hand, the Japanese criteria adopted visceral fat area of ⩾100 cm2 for both men and women. The policy undertaken by the Japanese definition is not to set more strict cutoff points for each item for women who have lower risk for CVD than men. For similar reasons, the Japanese criteria adopted a cutoff level for high‐density lipoprotein cholesterol (HDL‐C) of <40 mg/dL for both men and women as hypo‐HDL‐cholesterolemia according to the guideline of the Japan Atherosclerosis Society, while <40 mg/dL for men and <50 mg/dL for women was used by the IDF as criteria of MetS. The latter cutoff points are also based on the mean values, which is higher for women than for men. The Framingham study indicated a steep rise in the incidence of CVD in patients with HDL‐C <40 mg/dL, including women. Furthermore, The Lipid Research Clinics Prevalence Mortality Follow‐up Study suggested a cutoff value of 40 mg/dL. In the Framingham study, the risk score for CVD was higher by two points in the subgroup with an HDL‐C <40 mg/dL, irrespective of sex. Although the number of risk factors in subjects with a given visceral fat area is almost similar in both sexes, further investigation should be conducted in the future to set the cutoff point for the amount of visceral fat in men and women with respect to CVD risk. For clinical convenience, increased waist circumference is adopted as an indication of accumulation of visceral fat. Women often have more abdominal subcutaneous fat even though having a similar amount of visceral fat compared to men (). The cutoff points of waist circumference of 85 cm for men and 90 cm for women, which correspond to a visceral fat area of 100 cm2 for men and women, respectively, were adopted in the Japanese criteria. However, it is often difficult to estimate visceral fat accumulation by waist circumference in premenopausal women. Nonradiographic methods such as the bioimpedance method may be useful to estimate visceral fat accumulation Citation26.
Figure 7. Representative CT scanning images of middle‐aged man and woman. In general, women have relatively larger amounts of subcutaneous fat in the abdomen compared to men.
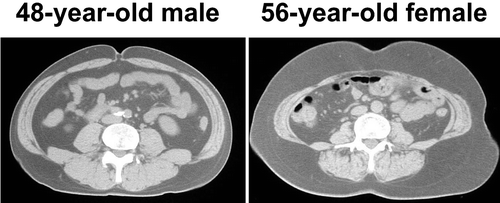
The worldwide criteria for the definition of MetS include hypertriglyceridemia and low HDL cholesterol level as two separate components. Because visceral fat accumulation and resultant insulin resistance are the underlying mechanisms, hypertriglyceridemia and low HDL cholesterol level can commonly occur as associated lipid abnormalities. Thus, hypertriglyceridemia and low HDL cholesterol level are regarded as one criterion in the Japanese definition of MetS, based on the guidelines of the Japan Atherosclerosis Society. The cutoff point of fasting plasma glucose is 110 mg/dL according to the guidelines of the Japan Diabetes Society.
The prevalence of MetS varies among countries and ethnic populations. Some populations show similar prevalence in both men and women. In some populations, the prevalence of MetS is even higher in women than in men. Although the precise molecular mechanism of MetS should be investigated further, MetS is a clinical condition associated with central obesity (visceral obesity) two or more of cardiovascular risk factors, as well as dysregulation of various adipocytokines, proinflammatory, and a tendency for thrombosis, a feature commonly seen in middle‐aged men. Such a condition should increase the global cardiometabolic risk and consequently CVD. The purpose of diagnosis of MetS is to reduce waist circumference in order to reduce the number and severity of cardiometabolic risk factors, and consequently to minimize the risk of CVD. In such a process, the selection of an appropriate population is needed.
The National Health and Nutrition Survey 2004 conducted by the Japanese Ministry of Health, Labor, and Welfare reported the prevalence of MetS in the Japanese population including randomly selected 3,421 families. The incidence of MetS increased from less than 10% in individuals aged 30–39 years to 20%–30% in men aged 50–69 years (). The incidence of MetS in women was lower than in men, but increased after the menopause. The research group on a serum lipid level survey in 2000 also reported that the incidence of MetS in 3,264 people receiving annual health examinations was relatively high in middle‐aged men and women after 50 years of age but was less in women than men Citation67. Such individuals are the target of effective preventive programs against CVD.
Figure 8. Percentages of Japanese men and women with a provisional diagnosis of metabolic syndrome. A total of 3421 families by stratified sampling were included in the National Health and Nutrition Survey 2004 by the Ministry of Health, Labor, and Welfare. The prevalence of metabolic syndrome was investigated in individuals⩾20 years of age strongly suspected of having the metabolic syndrome, based on findings of occasional blood sampling. The following criteria were adopted for the diagnosis of metabolic syndrome: Waist circumference of 85 cm for men and 90 cm for women, plus two of the following three: 1) HDL‐C level <40 mg/dL; 2) blood pressure ⩾130/85 mmHg; and 3) hemoglobin A1c ⩾5.5%. From the Report of National Health and Nutrition Survey 2004 by the Ministry of Health, Labor, and Welfare, Japan.
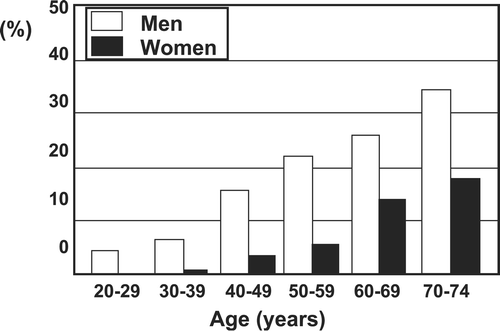
Challenges in combating metabolic syndrome
A survey was conducted through research supported by a grant from the Ministry of Labor (presently, Ministry of Health, Labor, and Welfare) of 122,051 Japanese workers from 31 industries, through regular medical health checks, over 10 years before the onset of CVD. The results of this survey revealed that subjects who suffered CVD had several abnormalities in plasma lipids and glucose, blood pressure level, and increased body weight, even though the degree of abnormalities was mild Citation68. The survey showed that individuals with at least three of four risk factors (hyperlipidemia, hyperglycemia, hypertension, and obesity) were at 30‐fold higher risk of CVD compared with age‐ and sex‐matched controls (no risk factors), and confirmed that the presence of multiple risk factor syndrome also accounted for a large part of morbidity underlying CVD in Japanese workers. Based on the results of the survey, the Ministry of Health, Labor, and Welfare and the Japan Medical Association instituted a scheme in 2001 to support workers with multiple risk factor syndrome for receiving medical checkups for CVD and guidance on life‐style changes.
Since the establishment of the definition and criteria of MetS in 2005, several social efforts have been mobilized in Japan. The mass media have recognized the importance of MetS for the public health of Japan and announced its importance to the nation through newspapers, magazines, radio, and television. These activities encouraged many people, from children to elderly people, to know what MetS is and how they should combat it. Currently, many people engage in physical activities aimed to reduce visceral fat and multiple risk factors. The second point is public health activities. Measurement of waist circumference is introduced to regular medical health checks. After the health check, public health nurses provide the individual with scientific information about the risk associated with accumulation of visceral fat, clustering of multiple risk factors, injury of arteries, and development of life‐threatening CVD. Furthermore, they also explain the results of the medical checkup and the relationship between the subject's life‐style and the abnormalities recorded in the health checkup, to promote life‐style changes. Management of eating behavior and a bond of affection among family members are essential. The subjects practice such courses in groups or individually. Subjects requiring further examination for CVD or treatment of diabetes, hypertension, and/or dyslipidemia are introduced to family physicians or specialists. Such activities called ‘Hokenshido’ in Japan eventually resulted in reduction of CVD‐related deaths in office workers (). The Japanese government plans to extend the MetS‐based medical health check to individuals aged 40–75 years from 2008. We expect that these efforts against MetS will result in effective combating of MetS and a reduction of life‐style‐related diseases and CVD. The network system of regional public health centers, family physicians, and hospitals will be required to combat MetS.
Figure 9. Management of metabolic syndrome leads to reduction of cardiovascular‐related deaths. A health promotion program directed against the metabolic syndrome was conducted in Amagasaki Municipal Government offices, Hyogo Prefecture, in 3946 workers from Hyogo. After the introduction of this program in 2000, the incidence of cardiovascular deaths in this office was clearly reduced. From data of Kenko Amagasakishi 21: Health Survey and Health Promotion Program for staff of the Amagasaki Municipal Government office in 2004.
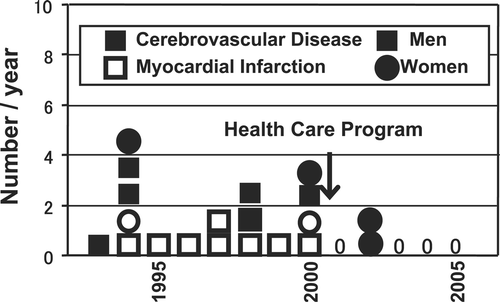
The prevalence of CVD based on a cluster of multiple risk factors is increasing in many countries, where overeating and physical inactivity are a common life‐style. However, we should never forget that there are still many countries in which people suffer from starvation and have short life spans because of a high childhood mortality rate linked to malnutrition and infectious diseases.
References
- The IDF consensus worldwide definition of the metabolic syndrome, Available from http://www.idf.org/webdata/docs/Metabolic_syndrome_definition.pdf.
- Tokunaga K., Matsuzawa Y., Ishikawa K., Tarui S. A novel technique for the determination of body fat by computed tomography. Int J Obes 1983; 7: 437–45
- Fujioka S., Matsuzawa Y., Tokunaga K., Tarui S. Contribution of intraabdominal fat accumulation to the impairment of glucose and lipid metabolism in human obesity. Metabolism 1987; 36: 54–9
- Kanai H., Matsuzawa Y., Kotani K., Keno Y., Kobatake T., Nagai Y., et al. Close correlation of intra‐abdominal fat accumulation to hypertension in obese women. Hypertension 1990; 16: 484–90
- Matsuzawa Y., Fujioka S., Tokunaga K., Tarui S. Classification of obesity with respect to morbidity. Proc Soc Exp Biol Med 1992; 200: 197–201
- Reaven G. M. Banting lecture 1988. Role of insulin resistance in human disease. Diabetes 1988; 37: 1595–607
- Kaplan N. M. The deadly quartet. Upper‐body obesity, glucose intolerance, hypertriglyceridemia, and hypertension. Arch Intern Med 1989; 149: 1514–20
- DeFronzo R. A., Ferrannini E. Insulin resistance. A multifaceted syndrome responsible for NIDDM, obesity, hypertension, dyslipidemia, and atherosclerotic cardiovascular disease. Diabetes Care 1991; 14: 173–94
- Vague J. La differentiation sexuelle facteur determinant des formes de l'obesite. Presse Med 1947; 30: 339–40
- Kissebah A. H., Vydelingum N., Murray R., Evans D. J., Hartz A. J., Kalkhoff R. K., et al. Relation of body fat distribution to metabolic complications of obesity. J Clin Endocrinol Metab 1982; 54: 254–60
- Larsson B., Svardsudd K., Welin L., Wilhelmsen L., Bjorntorp P., Tibblin G. Abdominal adipose tissue distribution, obesity, and risk of cardiovascular disease and death: 13 year follow up of participants in the study of men born in 1913. Br Med J Clin Res Ed 1984; 288: 1401–04
- Nakamura T., Tokunaga K., Shimomura I., Nishida M., Yoshida S., Kotani K., et al. Contribution of visceral fat accumulation to the development of coronary artery disease in non‐obese men. Atherosclerosis 1994; 107: 239–46
- Koutsari C., Jensen M. D. Thematic review series: patient‐oriented research. Free fatty acid metabolism in human obesity. J Lipid Res 2006; 47: 1643–50
- Kuriyama H., Yamashita S., Shimomura I., Funahashi T., Ishigami M., Aragane K., et al. Enhanced expression of hepatic acyl‐coenzyme A synthetase and microsomal triglyceride transfer protein messenger RNAs in the obese and hypertriglyceridemic rat with visceral fat accumulation. Hepatology 1998; 27: 557–62
- White A. L., Graham D. L., LeGros J., Pease R. J., Scott J. Oleate‐mediated stimulation of apolipoprotein B secretion from rat hepatoma cells. A function of the ability of apolipoprotein B to direct lipoprotein assembly and escape presecretory degradation. J Biol Chem 1992; 267: 15657–64
- Kishida K., Kuriyama H., Funahashi T., Shimomura I., Kihara S., Ouchi N., et al. Aquaporin adipose, a putative glycerol channel in adipocytes. J Biol Chem 2000; 275: 20896–902
- Maeda N., Funahashi T., Hibuse T., Nagasawa A., Kishida K., Kuriyama H., et al. Adaptation to fasting by glycerol transport through aquaporin 7 in adipose tissue. Proc Natl Acad Sci U S A 2004; 101: 17801–06
- Shimomura I., Hammer R. E., Richardson J. A., Ikemoto S., Bashmakov Y., Goldstein J. L., et al. Insulin resistance and diabetes mellitus in transgenic mice expressing nuclear SREBP‐1c in adipose tissue: model for congenital generalized lipodystrophy. Genes Dev 1998; 12: 3182–94
- Maeda K., Okubo K., Shimomura I., Mizuno K., Matsuzawa Y., Matsubara K. Analysis of an expression profile of genes in the human adipose tissue. Gene 1997; 190: 227–35
- Shimomura I., Funahashi T., Takahashi M., Maeda K., Kotani K., Nakamura T., et al. Enhanced expression of PAI‐1 in visceral fat: possible contributor to vascular disease in obesity. Nat Med 1996; 2: 800–03
- Funahashi T., Nakamura T., Shimomura I., Maeda K., Kuriyama H., Takahashi M., et al. Role of adipocytokines on the pathogenesis of atherosclerosis in visceral obesity. Intern Med 1999; 38: 202–06
- Hotamisligil G. S., Shargill N. S., Spiegelman B. M. Adipose expression of tumor necrosis factor‐alpha: direct role in obesity‐linked insulin resistance. Science 1993; 259: 87–91
- Zhang Y., Proenca R., Maffei M., Barone M., Leopold L., Friedman J. M. Positional cloning of the mouse obese gene and its human homologue. Nature 1994; 372: 425–32
- Maeda K., Okubo K., Shimomura I., Funahashi T., Matsuzawa Y., Matsubara K. cDNA cloning and expression of a novel adipose specific collagen‐like factor, apMa (Adipose Most Abundant gene transcript 1). Biochem Biophys Res Commun 1996; 221: 286–9
- Arita Y., Kihara S., Ouchi N., Takahashi M., Maeda K., Miyagawa J., et al. Paradoxical decrease of an adipose‐specific protein, adiponectin, in obesity. Biochem Biophys Res Commun 1999; 257: 79–83
- Ryo M., Nakamura T., Kihara S., Kumada M., Shibazaki S., Takahashi M., et al. Adiponectin as a biomarker of the metabolic syndrome. Circ J 2004; 68: 975–81
- Okamoto Y., Arita Y., Nishida M., Muraguchi M., Ouchi N., Takahashi M., et al. An adipocyte‐derived plasma protein, adiponectin, adheres to injured vascular walls. Horm Metab Res 2000; 32: 47–50
- Ouchi N., Kihara S., Arita Y., Maeda K., Kuriyama H., Okamoto Y., et al. Novel modulator for endothelial adhesion molecules: adipocyte‐derived plasma protein, adiponectin. Circulation 1999; 100: 2473–6
- Ouchi N., Kihara S., Arita Y., Okamoto Y., Maeda K., Kuriyama H., et al. Adiponectin, adipocyte‐derived plasma protein, inhibits endothelial NF‐kB signaling through cAMP‐dependent pathway. Circulation 2000; 102: 1296–301
- Arita Y., Kihara S., Ouchi N., Maeda K., Kuriyama H., Okamoto Y., et al. Adipocyte‐derived plasma protein, adiponectin, acts as a platelets growth factor‐BB‐binding protein and regulates growth factor‐induced common postreceptor signal in vascular smooth muscle cell. Circulation 2002; 105: 2893–8
- Ouchi N., Kihara S., Arita Y., Nishida M., Matsuyama A., Okamoto Y., et al. Adipocyte‐derived plasma protein, adiponectin, suppresses lipid accumulation and class A scavenger receptor expression in human monocyte‐derived macrophages. Circulation 2001; 103: 1057–63
- Yokota T., Oritani K., Takahashi I., Ishikawa J., Matsuyama A., Ouchi N., et al. Adiponectin, a novel member of soluble defense collagens, negatively regulates the growth of myelo‐monocytic progenitors and the functions of macrophages. Blood 2000; 96: 1723–32
- Kumada M., Kihara S., Ouchi N., Kobayashi H., Okamoto Y., Ohashi K., et al. Adiponectin specifically increased tissue inhibitor of metalloproteinase‐1 through interleukin‐10 expression in human macrophages. Circulation 2004; 109: 2046–9
- Okamoto Y., Kihara S., Ouchi N., Nishida M., Arita Y., Kumada M., et al. Adiponectin reduces atherosclerosis in apolipoprotein E‐deficient mice. Circulation 2002; 106: 2767–70
- Matsuda M., Shimomura I., Sata M., Arita Y., Nishida M., Maeda N., et al. Role of adiponectin in preventing vascular stenosis—the missing link of adipo‐vascular axis. J Biol Chem 2002; 277: 37487–91
- Shibata R., Sato K., Pimentel D. R., Takemura Y., Kihara S., Ohashi K., et al. Adiponectin protects against myocardial ischemia‐reperfusion injury through AMPK‐ and COX‐2‐dependent mechanisms. Nat Med 2005; 11: 1096–103
- Ouchi N., Kihara S., Funahashi T., Nakamura T., Nishida M., Kumada M., et al. Reciprocal association of C‐reactive protein with adiponectin in blood stream and adipose tissue. Circulation 2003; 107: 671–4
- Kumada M., Kihara S., Sumitsuji S., Kawamoto T., Matsumoto S., Ouchi N., et al. Association of hypo‐adiponectinemia with coronary artery disease in men. Arterioscl Thromb Vasc Biol 2003; 23: 85–9
- Zoccali C., Mallamaci F., Tripepi G., Cutrupi S., Parlongo S., Malatino L., et al. Adiponectin, the most abundant adipocyte‐derived protein, is functionally related to metabolic risk factors and predicts cardiovascular outcomes in end stage renal disease. J Am Soc Nephrol 2002; 13: 134–41
- Pischon T., Girman C. J., Hotamisligil G. S., Rifai N., Hu F. B., Rimm E. B. Plasma adiponectin levels and risk of myocardial infarction in men. JAMA 2004; 291: 1730–7
- Efstathiou S. P., Tsioulos D. I., Tsiakou A. G., Gratsias Y. E., Pefanis A. V., Mountokalakis T. D. Plasma adiponectin levels and five‐year survival after first‐ever ischemic stroke. Stroke 2005; 36: 1915–19
- Weyer C., Funahashi T., Tanaka S., Hotta K., Matsuzawa Y., Pratley R. E., et al. Hypoadiponectinemia in obesity and type 2 diabetes: Evidence for a role of insulin resistance and/or hyperinsulinemia. J Clin Endocrinol Metab 2001; 86: 1930–5
- Hotta K., Funahashi T., Bodkin N. L., Ortmeyer H. K., Arita Y., Hansen B. C., et al. Circulating concentrations of the adipocyte protein, adiponectin, are decreased in parallel with reduced insulin sensitivity during the progression to type‐2 diabetes in rhesus monkeys. Diabetes 2001; 50: 1126–33
- Maeda N., Shimomura I., Kishida K., Nishizawa H., Matsuda M., Nagaretani H., et al. Diet‐induced insulin resistance in mice lacking adiponectin/ACRP30. Nat Med 2002; 8: 731–7
- Lindsay R. S., Funahashi T., Hanson R. L., Matsuzawa Y., Tanaka S., Tataranni P. A., et al. Adiponectin protects against development of type 2 diabetes in the Pima Indian population. Lancet 2002; 360: 57–8
- Kondo H., Shimomura I., Matsukawa Y., Kumada M., Takahashi M., Matsuda M., et al. Association of adiponectin/ACRP30/AdipoQ mutation with type 2 diabetes mellitus. A candidate gene for the insulin resistance syndrome. Diabetes 2002; 51: 2325–58
- Ohashi K., Ouchi N., Kihara S., Funahashi T., Nakamura T., Sumitsuji S., et al. Adiponectin I164T mutation is associated with the metabolic syndrome and coronary artery disease. J Am Coll Cardiol 2004; 43: 1195–200
- Ouchi N., Kobayashi H., Kihara S., Kumada M., Sato K., Inoue T., et al. Adiponectin stimulates angiogenesis by promoting cross‐talk between AMP‐activated protein kinase and Akt signaling in endothelial cells. J Biol Chem 2004; 279: 1304–09
- Chen H., Montagnani M., Funahashi T., Shimomura I., Quon M. J. Adiponectin stimulates production of nitric oxide in vascular endothelial cells. J Biol Chem 2003; 278: 45021–6
- Ouchi N., Ohishi M., Kihara S., Funahashi T., Nakamura T., Nagaretani H., et al. Association of hypoadiponectinemia with impaired vasoreactivity. Hypertension 2003; 42: 231–4
- Ohashi K., Kihara S., Ouchi N., Kumada M., Fujita K., Hiuge A., et al. Adiponectin replenishment ameliorates obesity‐related hypertension. Hypertension 2006; 47: 1108–16
- Shibata R., Ouchi N., Ito M., Kihara S., Shiojima I., Pimentel D. R., et al. Adiponectin‐mediated modulation of hypertrophic signals in the heart. Nat Med 2004; 10: 1384–9
- Miyoshi Y., Funahashi T., Kihara S., Taguchi T., Tamaki Y., Matsuzawa Y., et al. Association of serum adiponectin levels with breast cancer risk. Clin Cancer Res 2003; 9: 5699–704
- Mantzoros C., Petridou E., Dessypris N., Chavelas C., Dalamaga M., Alexe D. M., et al. Adiponectin and breast cancer risk. J Clin Endocrinol Metab 2004; 89: 1102–07
- Petridou E., Mantzoros C., Dessypris N., Koukoulomatis P., Addy C., Voulgaris Z., et al. Plasma adiponectin concentrations in relation to endometrial cancer. A case‐control study in Greece. J Clin Endocrinol Metab 2003; 88: 993–7
- Goktas S., Yilmaz M. I., Caglar K., Sonmez A., Kilic S., Bedir S. Prostate cancer and adiponectin. Urology 2005; 65: 1168–72
- Wei E. K., Giovannucci E., Fuchs C. S., Willett W. C., Mantzoros C. S. Low plasma adiponectin levels and risk of colorectal cancer in men. a prospective study. J Natl Cancer Inst 2005; 97: 1688–94
- Kamada Y., Matsumoto H., Tamura S., Fukushima J., Kiso S., Fukui K., et al. Hypoadiponectinemia accelerates hepatic tumor formation in a nonalcoholic steatohepatitis mouse model. J Hepatol 2007, Apr 9 [Epub ahead of print]
- Despres J. P., Golay A., Sjostrom L., Rimonabant in Obesity‐Lipids Study Group. Effects of rimonabant on metabolic risk factors in overweight patients with dyslipidemia. N Engl J Med 2005; 353: 2121–34
- Maeda N., Takahashi M., Funahashi T., Kihara S., Nishizawa H., Kishida K., et al. PPARγ ligands increase expression and plasma concentration of adiponectin, an adipose‐derived protein. Diabetes 2001; 50: 2094–9
- Iwaki M., Matsuda M., Maeda N., Funahashi T., Matsuzawa Y., Makishima M., et al. Induction of adiponectin, a fat‐derived antidiabetic and antiatherogenic factor, by nuclear receptors. Diabetes 2003; 52: 1655–63
- Furukawa S., Fujita T., Shimabukuro M., Iwaki M., Yamada Y., Nakajima Y., et al. Increased oxidative stress in obesity and its impact on metabolic syndrome. J Clin Invest 2004; 114: 1752–61
- Iwashima Y., Katsuya T., Ishikawa K., Kida I., Ohishi M., Horio T., et al. Association of hypoadiponectinemia with smoking habit in men. Hypertension 2005; 45: 1094–100
- Kurata A., Nishizawa H., Kihara S., Maeda N., Sonoda M., Okada T., et al. Blockade of Angiotensin II type‐1 receptor reduces oxidative stress in adipose tissue and ameliorates adipocytokine dysregulation. Kidney Int 2006; 70: 1717–24
- Funahashi T., Matsuzawa Y. Hypoadiponectinemia. A common basis for diseases associated with overnutrition. Curr Atheroscler Rev 2006; 8: 433–8
- Reducing Risks, Promoting Health Life. The World Health Report. World Health Organization, Geneva 2002
- Arai H., Yamamoto A., Matsuzawa Y., Saito Y., Yamada N., Oikawa S., et al. Prevalence of metabolic syndrome in the general Japanese population in 2000. J Atheroscler Thromb 2006; 13: 202–08
- Nakamura T., Tsubono Y., Kameda‐Takemura K., Funahashi T., Yamashita S., Hisamichi S., et al. Magnitude of sustained multiple risk factors for ischemic heart disease in Japanese employees. A case‐control study. Circ J 2001; 65: 11–17