Abstract
Objective
Nephrotoxicity is the main side effect of cyclosporine A and finding an effective combating method is urgent. The present study investigates the improving effect of erythropoietin (EPO) on cyclosporine A induce renal injury in rats and further explores its possible mechanism.
Methods
Recombinant adenovirus for expression of EPO was constructed and injected into kidney with multipoint. Levels of blood urea nitrogen (BUN) and serum creatinine (SCr) were detected by kits. HE staining and Masson’s trichrome staining were used to evaluate pathological changes. ELISA was performed to detect the levels of transforming growth factor (TGF)-β1, interleukin (IL)-1β, and IL-6 in serum. Levels of malondialdehyde (MDA) and superoxide dismutase (SOD) in kidney were detected according to manufacturer’s instruction. Western blotting was performed to observe the protein expression levels of peroxisome proliferator-activated receptor γ (PPAR γ), Toll-like receptor (TLR) 4, and TGF-β1.
Results
Results showed that EPO overexpression in rat kidney could significantly improve renal injury and fibrosis, suppress the release of inflammatory factors and reduce oxidative stress induced by cyclosporine A. Western blotting results showed that EPO overexpression could up-regulate the expression of PPARγ and down-regulate the expression of TLR4 and TGF-β1. Interestingly, when PPARγ activity was inhibited by T0070907, an effective and specific PPARγ inhibitor, the therapeutic effect of EPO was significantly attenuated.
Conclusion
Taken together, above results shown the protective effect of EPO on cyclosporine A-induced renal injury and confirmed that EPO’s anti-inflammation and antioxidative stress involving the PPAR γ/TLR4/TGFβ1 axis.
1. Introduction
Cyclosporin A (Cs A) is an immunosuppressive agent, which is mainly used for immunological rejection after organ transplantation, and greatly improves the success rate of organ transplantation. Cyclosporin A can inhibit the activation of T cells by inhibiting calcineurin, then preventing the phosphorylation of nuclear factor of activated T cells family members, which could activate the transcription of interleukin (IL) 2 and IL 4 genes [Citation1]. However, the use of cyclosporine A has acute and long-term toxic effects on the kidneys. In the short term, it can cause the reversible decrease of renal blood flow and glomerular filtration rate. In the long run, it can lead to irreversible renal failure characterized by extensive tubulointerstitial fibrosis [Citation2,Citation3]. Renal tubulointerstitial fibrosis is the end stage of almost all renal diseases. How to effectively prevent and improve cyclosporine A-induced nephrotoxicity is one of the urgent problems to be solved in clinical practice.
Erythropoietin (EPO) is a glycoprotein mainly derived from peritubular interstitial fibroblasts in the renal cortex and outer medulla. It can promote bone marrow erythrocyte maturation and erythropoiesis [Citation4]. Recombinant human EPO is mainly used to treat anemia caused by chronic renal failure, as well as anemia caused by radiotherapy and chemotherapy in cancer patients [Citation5,Citation6]. However, a series of studies have shown that indications benefiting from EPO treatment are clearly beyond the scope of anemia. In a variety of animal models, EPO exhibits multiple protective effects on tissues or organs, such as the pleiotropic effects on the cardiovascular system and kidney [Citation7–10]. In preliminary experiment, we have observed that EPO could effectively improve the renal injury induced by cyclosporine A in rats. The present study further explored its possible mechanism of action.
2. Materials and methods
2.1. Reagents
T0070907 was purchased from Selleck Chemicals (Houston, TX, USA). Anti-peroxisome proliferator-activated receptor γ (PPARγ), anti-Toll-like receptor (TLR) 4, anti-transforming growth factor (TGF)-β, and anti-β-actin were obtained from Abcam (Cambridge, Cambs, UK). ELISA kits of transforming growth factor (TGF)-β1, IL-1β, and IL-6 were purchased from Ray Biotech, Inc. (Atlanta, GA, USA). Malondialdehyde (MDA) and superoxide dismutase (SOD) detection kits were purchased from Jiancheng Bioengineering Institute of Nanjing (Nanjing, JS, CHN).
2.2. Animals
Adult male Sprague Dawley (SD) rats, weighing 250–300 g, were obtained from the Nanchang University Laboratory Animal Center. The animals were allowed to access food and water ad libitum and maintained under a 12-h dark/light cycle at 22 °C–25 °C. Experiments were carried out according to the Guide for the Care and Use of Laboratory Animals published by the U.S. National Institutes of Health (NIH Publication No. 85-23, revised in 1996), and approved by the Ethics Committee of Nanchang University (No. 2017-0306).
2.3. Construction of recombinant adenovirus for expression of EPO
Using site-specific recombination cloning, EPO (accession no. NM_017001) was cloned into the GV287 vector (Shanghai Genechem Co., Ltd., Shanghai, China). Then lipofectamine 2000 (Invitrogen; Thermo Fisher Scientific, Inc.) was used to co-transfecting into 293 cells. Homologous recombination was used to producing recombinant adenovirus. In 293 cells, viruses were largely amplified. Centrifuged sample for 20 min at 3960 × g at 4 °C after 48 h and collected the supernatant. Ultra-speed centrifuge (Beckman, Fullerton, CA) was used to concentrate virus particles at 5000 × g for 2.5 h. Virus was packed and stored at −80 °C. Virus titer was detected by tissue culture infective dose 50. Overexpression efficiency was confirmed via western blotting.
2.4. Experimental groups and treatments
Rats were randomly divided into five groups (n = 6 per group), as follows: (1) control group (Ctrl), in which rats were received left nephrectomy and 100 μL 0.9% normal saline sc. (2) cyclosporin A-induced group (Cs A), in which rats were received left nephrectomy and Cs A 15 mg/kg/day sc [Citation11]. (3) EPO overexpression group (Cs A + EPO), in which rats were received left nephrectomy and Cs A 15 mg/kg/day sc with intrarenal delivery with 2 × 107 transduction units (TU) EPO recombinant lentivirus to the right intact kidney multipointly [Citation12]. (4) the negative control lentivirus group (Cs A + Ctrl-lv), in which rats were received left nephrectomy and Cs A 15 mg/kg/day sc with intrarenal delivery with 2 × 107 transduction units (TU) lentivirus containing empty plasmids to the right intact kidney multipointly. (5) EPO overexpression combined with T0070907 administration (Cs A + EPO + T0070907), in which rats were received T0070907 1 mg/kg/day ip based on treatments of Cs A + EPO group. The titer of lentivirus and the doses of T0070907 used in the current experiments were determined during preliminary experiments. After 28 days, rats were anesthetized with pentobarbital sodium 50 mg/kg ip, blood was taken from inferior vena cava and serum was harvested with storing at −80 °C. After rats were sacrificed, the right kidneys were removed out and divided into two parts, one part was fixed with 4% polyformaldehyde and the other part was frozen at −80 °C.
2.5. Serum analysis
Rats were anesthetized with pentobarbital sodium 50 mg/kg intraperitoneally, and venous blood was collected from inferior vena cava. Blood urea nitrogen (BUN) and serum creatinine (SCr) were detected by kits according to the manufacturer’s instructions (Jiancheng Bioengineering Institute of Nanjing, Nanjing, JS, CHN). ELISA was used to detect TGF-β1, IL1β, and IL-6 levels in serum according to the manufacturers’ protocols.
2.6. Histopathological examination
Rats kidneys were dissected and fixed in 4% paraformaldehyde at room temperature for ≥12 h. After paraffin embedding and slicing, hematoxylin–eosin (HE) staining and Masson’s trichrome staining were performed following the manufacturer’s guidelines (Solarbio, Beijing, CHN) to determine changes of renal architecture. Renal injury scores were determined by three pathologists in a blinded fashion according to the extent of kidney injury. The scoring criteria refer to Sereno et al. [Citation13]. Scoring was defined according to the extension of the lesion: 1 (normal: 0%); 2 (mild: <25%); 3 (moderate: 25–50%; severe: >50%). A total of six discontinuous sections per specimen and five fields per section were selected for analysis to determine the total collagen content and % of fibrotic area using Image Pro Plus 6.0 software (National Institutes of Health, Bethesda, MD).
2.7. Assessment of oxidative stress
The levels of MDA and SOD in kidney were detected according to manufacturer’s instruction (Jiancheng Bioengineering Institute of Nanjing, CHN).
2.8. Western blotting
The protein was extracted from renal tissue, separated by sodium dodecyl sulfate – polyacrylamide gel electrophoresis and electrotransferred onto polyvinylidene difluoride membranes that were probed with primary antibodies (1:500) diluted in 5% nonfat milk and incubated at 4 °C overnight. Then membranes were incubated with the horseradish peroxidase-conjugated secondary antibodies for 2 h at room temperature after washed by tris-buffered saline and tween 20 (TBS-T). The immune complexes were visualized by enhanced chemiluminescence after washing again and the band intensity was measured quantitatively and analyzed with the Image J v2.1.4.7 software (National Institutes of Health, Bethesda, MD).
2.9. Statistical analysis
Data were presented as means ± standard error of the mean from six independent experiments and analyzed by SPSS version 20.0 (IBM Corp., Armonk, NY). The assumption of normality was tested with the Shapiro–Wilk test for normality prior to additional analysis. Normally distributed data were analyzed by Student’s unpaired, two-tailed t-test (2 groups) or ANOVA (> 2 groups). Data not normally distributed were analyzed using the Mann–Whitney U test (2 groups) or Kruskal–Wallis one-way ANOVA (> 2 groups). A probability of p < 0.05 was set as the level of significance for all comparisons.
3. Results
3.1. Effect of EPO overexpression on renal function induced by cyclosporin A
Renal function in rats was assessed by measuring serum levels of SCr, BUN, and their clearances. As shown in , cyclosporin A induced high levels of SCr and BUN, and low levels of clearances of SCr and BUN when compared with the control group (p < 0.05). Erythropoietin overexpression could significantly ameliorate serum levels of SCr, BUN, and their clearances (p < 0.05 versus Cs A group). What’s more, when the activity of PPARγ was inhibited by T0070907, the effects of EPO on improving renal function were attenuated significantly as well as in group of CsA + EPO + Ctrl-lv (p < 0.05 versus Cs A + EPO group).
Figure 1. Effects of erythropoietin on renal function after cyclosporin A induction. (A) The mean level of SCr in each group. (B) The mean level of Cr clearance in each group. (C) The mean level of BUN in each group. (D) The mean level of BUN clearance in each group. (E) The HE staining of renal section of each group (200×). (F) The Masson’s trichrome staining of renal section of each group (400×). (G) Proprotion of renal fibrosis area. Data are presented as the means ± standard error of the mean for six independent experiments. *p < 0.05 versus the control group; ▲p < 0.05 versus the Cs A group; #p < 0.05 versus the Cs A + EPO group.
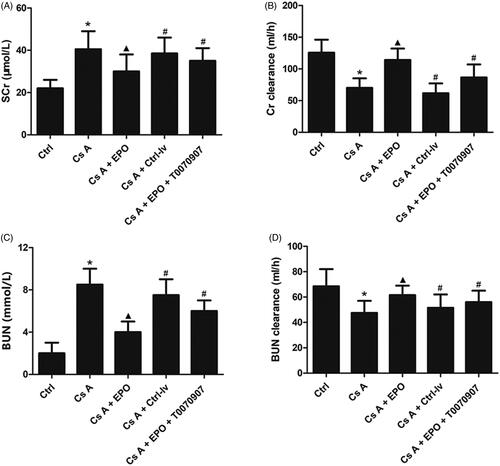
3.2. Effect of EPO overexpression on cyclosporin A-induced renal injury
HE staining was used to observe the pathological changes of renal architecture. As shown in , the renal architecture and nephrocyte of rats in control group were normal. In cyclosporin A-induced renal sections showed obvious vacuolar degeneration, atrophy, necrosis and exfoliation of kidney tubules, interstitial fibrosis, glomerular atrophy, and vascular congestion. In Cs A + EPO group, showed improvements in the histopathological changes compared with Cs A group. Interestingly, administration with T0070907 or lentivirus containing empty plasmids reversed the positive effect of EPO on architecture of kidney.
Figure 2. Effects of erythropoietin on renal injury after cyclosporin A induction. (A) The HE staining of renal section of each group (200×). (B) The Masson’s trichrome staining of renal section of each group (400×). (C) Renal injury scores. (G) Proprotion of renal fibrosis area. Data are presented as the means ± standard error of the mean for six independent experiments. *p < 0.05 versus the control group; ▲p < 0.05 versus the Cs A group; #p < 0.05 versus the Cs A + EPO group.
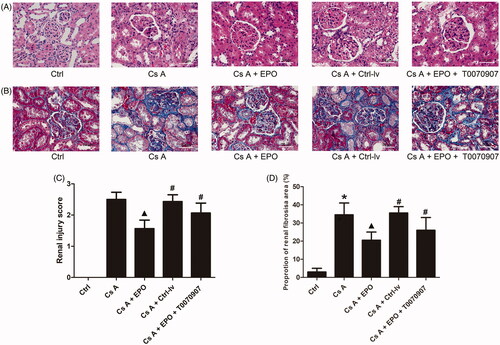
Masson’s trichrome staining was performed to evaluate renal fibrosis. As shown in , chronic intoxication with cyclosporin A induced an increase of collagen fibers in rat kidney when compared to control (p < 0.05), which was significantly improved by overexpressed EPO in the group of Cs A + EPO (p < 0.05 versus the Cs A group). However, administration with T0070907 or lentivirus containing empty plasmids significantly increased accumulation of collagen fibers (p < 0.05 versus Cs A + EPO group).
3.3. Effects of EPO overexpression on releases of inflammatory factors
ELISA was used to detect the release levels of IL-6, IL-1β, and TGF-β1 in serum. As shown in , The levels of IL-6, IL-1β, and TGF-β1 in peripheral blood of rat induced by cyclosporin A were significantly higher than those in control (p < 0.05). EPO could suppress the increase of IL-6, IL-1β, and TGF-β1 induced by cyclosporin A in rats serum (p < 0.05 vs. Cs A group). However, the levels of IL-6, IL-1β, and TGF-β1 in peripheral blood of rats increased significantly when the activity of PPARγ was inhibited or lentivirus containing empty plasmids were administrated, reversing the effects of EPO (p < 0.05 versus Cs A + EPO group).
Figure 3. Effects of erythropoietin on releases of IL-6, IL-1β, and TGF-β1. (A) The concentration of IL 6 in serum of each group. (B) The concentration of IL-1β in serum of each group. (C) The concentration of TGF-β1 in serum of each group. Data are presented as the means ± standard error of the mean for six independent experiments. *p < 0.05 versus the control group; ▲p < 0.05 versus the Cs A group; #p < 0.05 versus the Cs A + EPO group.
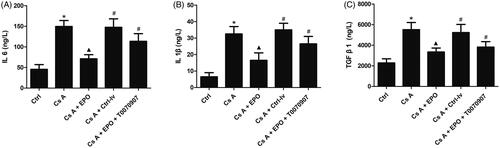
3.4. Effect of EPO overexpression on oxidative stress
The levels of SOD and MDA in kidneys of rats in each group were detected. As shown in , SOD level was significantly decreased (p < 0.05) and MDA level was significantly increased (p < 0.05) in the Cs A group when compared to the control group. Erythropoietin overexpression could significantly increase SOD level and decrease MDA level (p < 0.05 vs. Cs A group). However, above effects of EPO were significantly reversed in the group of CsA + EPO + Ctrl-lv or CsA + EPO + T0070907 (p < 0.05 vs. Cs A + EPO group).
Figure 4. Effect of erythropoietin on oxidative stress reaction in concanavalin A-induced renal injury. (A) The concentration of MDA in kidney of each group. (B) The concentration of SOD in kidney of each group. Data are presented as the means ± standard error of the mean for six independent experiments. *p < 0.05 versus the control group; ▲p < 0.05 versus the Cs A group; #p < 0.05 versus the Cs A + EPO group.
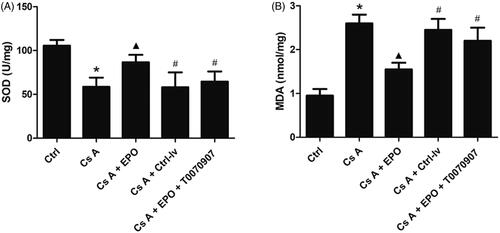
3.5. Effects of EPO overexpression on protein expression levels of PPARγ, TLR4, and TGF-β1 in cyclosporin A-induced renal injury
Western blotting was performed to detect the protein expression level of PPARγ, TLR4, and TGF-β1. As shown in , the expression levels of TLR4 and TGF-β1 were significantly upregulated while PPARγ was downregulated in kidney tissue affected by the toxicity of cyclosporin A in the Cs A group when compared with the control (p < 0.05). The overexpression of EPO could markedly inhibit the expression levels of TLR4 and TGF-β1, and increase the expression level of PPARγ (p < 0.05 versus LF group). Interestingly, when the activity of PPARγ was inhibited by T0070907, the effects of EPO on inhibition of TLR4 and TGF-β1 expressions were reversed (p < 0.05 versus Cs A + EPO group). However, the expression of PPARγ did not change significantly, which may be related to the fact that T0070907 could effectively inhibit the activity of PPAR γ without interfering with its protein expression. T0070907 covalently modifies PPAR γ on cysteine 313 in helix 3, and inhibits its protein activity as a result. There is no sufficient evidence that T0070907 can affect the transcription and translation of PPARγ gene [Citation14].
Figure 5. Effects of erythropoietin on protein expression levels of PPAR γ, TLR4, and TGF-β1 in concanavalin A-induced renal injury. (A) The bands of PPAR γ, TLR4, and TGF-β1 in each group. (B) The relative band intensity of PPARγ in each group. (C) The relative band intensity of TLR4 in each group. (D) The relative band intensity of TGF-β1 in each group. Data are presented as the means ± standard error of the mean for six independent experiments. *p < 0.05 versus the control group; ▲p < 0.05 versus the Cs A group; #p < 0.05 versus the Cs A + EPO group.
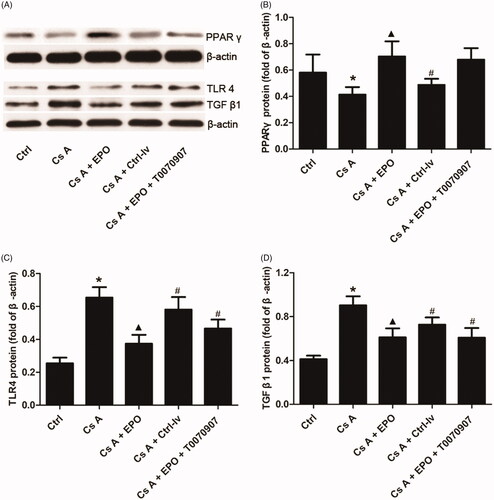
4. Discussion
Cyclosporin A is widely used for immunosuppression, but nephrotoxicity caused by long-term use has been a problem that has plagued the medical community. Cyclosporin A can cause zonal tubulointerstitial fibrosis, tubular atrophy, and afferent artery lesions [Citation15]. There are currently no effective methods and drugs for the prevention and treatment of cyclosporine A nephrotoxicity. In this study, we observed that EPO overexpression could significantly improve chronic renal injury and fibrosis induced by cyclosporine A in rats. The renal protective effect of EPO has been reported in many literatures. Zhao et al. [Citation16] found that EPO can improve renal dysfunction and interstitial fibrosis in rats with unilateral ureteral obstruction. Acikgoz’ study showed that the antifibrotic effect of EPO on rats with obstructive renal fibrosis may be regulated by nuclear factor kappa B (NF-кB) signaling pathway [Citation17]. Shi et al. [Citation18] showed that EPO can protect acute renal ischemia-reperfusion injury in mice and is closely related to the activity of EPO receptor. However, the other study suggested that although EPO has protective effects on renal function and structure in renal ischemia–reperfusion injury, the extraphysiological dose required for renal protection contributes to fibrosis and chronic kidney disease [Citation9], which inconsistent with our results. This may be due to the different renal injury models used.
Renal interstitial fibrosis is the main toxic effect of Cs A treatment, and renal fibrosis is the end stage of almost all kidney diseases [Citation2]. A large number of studies have shown that TGF-β plays a key role in the fibrosis process [Citation19]. TGF-β1 is the most abundant isoform of the TGF-β family and can be secreted by various types of renal cells or infiltrating inflammatory cells [Citation20]. TGF1-β1 stimulates fibroblast proliferation, extracellular matrix synthesis, and epithelial–mesenchymal transition to promote fibrosis [Citation21]. TGF-β1 also has a proinflammatory effect, and the relationship between renal inflammation and fibrosis has been fully confirmed. Inflammation is a protective response of the kidney after injury, but sustained inflammatory stimulation can lead to the production of profibroblasts and growth factors, which in turn recruit and activate myofibroblasts, leading to fibrosis of glomerular and interstitial [Citation22]. In addition, oxidative stress also plays a crucial role in the occurrence and development of renal fibrosis. Renal fibrosis is associated with ATP loss due to mitochondrial dysfunction, while mitochondrial dysfunction is associated with increased free radical production and oxidative stress [Citation23]. TGF-β stimulates the production of reactive oxygen species (ROS) in various types of cells through nicotinamide adenine dinucleotide phosphate (NADPH), mitochondria, and/or microsomes, while ROS activate TGF-β and mediate many of TGF-β’s fibrogenic effects [Citation24]. In the kidney, TGF-β1 can induce oxidative stress through nicotinamide adenine dinucleotide phosphate (NADPH) oxidase [Citation25]. The present results showed that Cs A could induce high expression of TGF-β1 and large release of IL-1 and IL-6 in rat kidneys, and aggravate oxidative stress. After overexpression of EPO in kidney, the expression of TGF-β1 was significantly inhibited, the levels of IL-1 and IL-6 were markedly decreased, and the oxidative stress responses were obviously reduced. It suggested that the protective effect of EPO on renal injury induced by Cs A in rats was related to its ability to inhibit the expression of TGF-β1, antiinflammation, and antioxidative stress.
TLR4 is a key member of TLRs family. Activated TLR4 can induce inflammatory response by activating NF-кB [Citation26]. TLR4 can also regulate the expression of TGF-β1 and promote the recruitment of fibroblasts in the kidney, leading to the occurrence of renal fibrosis [Citation27]. Study has confirmed that abnormal activation of TLR4 is involved in the proinflammatory response of cyclosporine A nephrotoxicity, and inhibiting TLR4 activity can effectively improve renal injury and fibrosis induced by cyclosporine A [Citation28]. In addition, TLR4 is also involved in the regulation of oxidative stress. Activation of TLR4 can cause oxidative stress and endothelial dysfunction, which participating in the development of cardiovascular disease [Citation29]. Injection of angiotensin into the body will activate TLR4, which can trigger oxidative stress and vascular damage [Citation30]. After TLR4 was knocked out, oxidative stress response and activation of macrophages were reduced, and extracellular matrix deposition of kidney was inhibited in mice [Citation27]. In this study, we observed that cyclosporine A could upregulate the expression of TLR4 in kidney of rat, while overexpressed EPO could significantly inhibit the expression of TLR4, suggesting that TLR4 participated in the renal protection of EPO.
PPARγ is a member of the ligand-activated nuclear transcription superfamily, which expresses in the kidney and participates in the regulation of many physiological activities, including cell cycle, inflammation, cytokine production, and organ fibrosis [Citation23]. Yang et al. found that PPARγ agonist pioglitazone can improve renal injury, glomerulosclerosis, and interstitial fibrosis in alloxan-induced elderly diabetic rats by inhibiting the expression of TGF-β, reducing oxidative stress and improving mitochondrial function [Citation31]. Zambrano et al. [Citation32] used GW9662 to antagonize PPAR γ and found that it could aggravate renal fibrosis induced by hypertension in rats. In this study, we observed that the expression of PPARγ was significantly downregulated in cyclosporine A induced injured kidney of rats. Overexpression of EPO could significantly upregulate the expression of PPARγ, suggesting that the renal protective effects of EPO may be related to PPARγ pathway. Inhibiting the activity of the PPARγ pathway by T0070907, we observed that the effects of EPO on antiinflammation, anti-oxidative stress, inhibition of renal fibrosis, and improvement of renal function were all attenuated. More importantly, the expression levels of TLR-4 and TGF-β1 were upregulated after PPARγ pathway was inhibited, which indicated that PPARγ, as the upstream regulatory pathway of TLR-4 and TGF-β1, participated in the protective effects of EPO on cyclosporine A-induced renal injury.
In conclusion, our study found that overexpression of EPO in rat kidney can significantly improve cyclosporine A-induced renal injury, ameliorate renal fibrosis, suppress the release of inflammatory factors and reduce oxidative stress. PPAR γ/TLR 4/TGF-β1 axis may be the common signal pathway for EPO to achieve the above positive effects.
Data availability
The scientific and statistical data used to support the findings of this study are included within the article.
Disclosure statement
No potential conflict of interest was reported by the author(s).
Additional information
Funding
References
- Clipstone NA, Crabtree GR. Identification of calcineurin as a key signalling enzyme in T-lymphocyte activation. Nature. 1992;357(6380):695–697.
- Newton L. Cyclosporine-associated chronic nephropathy. N Engl J Med. 1984;311(11):699–705.
- Remuzzi G. Cyclosporine-induced renal dysfunction in experimental animals and humans. Kidney Int. 1995;48(2):63–80.
- Kalantar-Zadeh K. History of erythropoiesis-stimulating agents, the development of biosimilars, and the future of anemia treatment in nephrology. Am J Nephrol. 2017;45(3):235–247.
- Ohashi Y, Uemura Y, Fujisaka Y, et al. Meta-analysis of epoetin beta and darbepoetin alfa treatment for chemotherapy-induced anemia and mortality: individual patient data from Japanese randomized, placebo-controlled trials. Cancer Sci. 2013;104(4):481–485.
- Ramanath V, Gupta D, Jain J, et al. Anemia and chronic kidney disease: making sense of the recent trials. Rev Recent Clin Trials. 2012;7(3):187–196.
- Ahmet I, Tae HJ, Brines M, et al. Chronic administration of small nonerythropoietic peptide sequence of erythropoietin effectively ameliorates the progression of postmyocardial infarction-dilated cardiomyopathy. J Pharmacol Exp Ther. 2013;345(3):446.
- Fatemeh Y, Mohsen N, Behrang AA, et al. Effect of erythropoietin on kidney allograft survival: early use after transplantation. Iran J Kidney Dis. 2012;6(1):44–48.
- Gobe GC, Bennett NC, Malcolm W, et al. Increased progression to kidney fibrosis after erythropoietin is used as a treatment for acute kidney injury. Am J Physiol Renal Physiol. 2014;306(6):F681–F692.
- Liu F, Wen Y, Kang J, et al. Regulation of TLR4 expression mediates the attenuating effect of erythropoietin on inflammation and myocardial fibrosis in rat heart. Int J Mol Med. 2018;42(3):1436–1444.
- Shi SH, Zheng SS, Jia CK, et al. Inhibitory effect of tea polyphenols on transforming growth factor-beta1 expression in rat with cyclosporine A-induced chronic nephrotoxicity. Acta Pharmacol Sin. 2004;25(1):98–103.
- Yang H, Xie T, Li D, et al. Tim-3 aggravates podocyte injury in diabetic nephropathy by promoting macrophage activation via the NF-kappaB/TNF-alpha pathway. Mol Metab. 2019;23:24–36.
- Sereno J, Nunes S, Rodrigues-Santos P, et al. Conversion to sirolimus ameliorates cyclosporine-induced nephropathy in the rat: focus on serum, urine, gene, and protein renal expression biomarkers. Biomed Res Int. 2014;2014:1–17.
- Zaytseva YY, Wallis NK, Southard RC, et al. The PPAR gamma antagonist T0070907 suppresses breast cancer cell proliferation and motility via both PPAR gamma-dependent and -independent mechanisms. Anticancer Res. 2011;31(3):813–823.
- Bennett WM, Demattos A, Meyer MM, et al. Chronic cyclosporine nephropathy: the Achilles’ heel of immunosuppressive therapy. Kidney Int. 1996;50(4):1089–1100.
- Zhao XF, Liu YH, Han ZM, et al. Effect of erythropoietin on the expression of dynamin-related protein-1 in rat renal interstitial fibrosis. Exp Ther Med. 2015;9(6):2065–2071.
- Acikgoz Y, Can B, Bek K, et al. The effect of simvastatin and erythropoietin on renal fibrosis in rats with unilateral ureteral obstruction. Ren Fail. 2014;36(2):252–257.
- Shi M, Flores B, Li P, et al. Effects of erythropoietin receptor activity on angiogenesis, tubular injury and fibrosis in acute kidney injury: a “U-Shaped” relationship. Am J Physiol Renal Physiol. 2017;314(4):ajprenal.00306.02017.
- Yao LH. Diverse roles of TGF-β/Smads in renal fibrosis and inflammation. Int J Biol Sci. 2011;7(7):1056–1067.
- Agnieszka L, Mateusz S, Alicja J, et al. TGF-β1/Smads and miR-21 in renal fibrosis and inflammation. Mediators Inflamm. 2016;2016(1):1–12.
- Böttinger EP. TGF-β in renal injury and disease. Semin Nephrol. 2007;27(3):309–320.
- Xiao-Ming M, Nikolic-Paterson DJ, Yao LH. Inflammatory processes in renal fibrosis. Nat Rev Nephrol. 2014;10(9):493.
- Lv W, Booz GW, Fan F, et al. Oxidative stress and renal fibrosis: recent insights for the development of novel therapeutic strategies. Front Physiol. 2018;9(1):105.
- Liu RM, Gaston Pravia KA. Oxidative stress and glutathione in TGF-beta-mediated fibrogenesis. Free Radic Biol Med. 2010;48(1):1–15.
- Lee SY, Kim SI, Choi ME. Therapeutic targets for treating fibrotic kidney diseases. Transl Res. 2015;165(4):512–530.
- Zhang L, Sun D, Bao Y, et al. Nerolidol protects against LPS-induced acute kidney injury via inhibiting TLR4/NF-κB signaling. Phytother Res. 2017;31(3):459–465.
- Pushpakumar S, Ren L, Kundu S, et al. Toll-like receptor 4 deficiency reduces oxidative stress and macrophage mediated inflammation in hypertensive kidney. Sci Rep. 2017;7(1):6349.
- González-Guerrero C, Cannata-Ortiz P, Guerri C, et al. TLR4-mediated inflammation is a key pathogenic event leading to kidney damage and fibrosis in cyclosporine nephrotoxicity. Arch Toxicol. 2017;91(4):1925–1939.
- Dange RB, Agarwal D, Masson GS, et al. Central blockade of TLR4 improves cardiac function and attenuates myocardial inflammation in angiotensin II-induced hypertension. Cardiovasc Res. 2014;103(1):17–27.
- De PB, Palacios R, Martín A, et al. Toll-like receptor 4 upregulation by angiotensin II contributes to hypertension and vascular dysfunction through reactive oxygen species production. Plos One. 2014;9(8):e104020.
- Hai-Chun Y, Sebastien D, Yiqin Z, et al. The PPARgamma agonist pioglitazone ameliorates aging-related progressive renal injury. J Am Soc Nephrol. 2009;20(11):2380–2388.
- Sonia Z, Blanca AJ, Ruiz-Armenta MV, et al. L-carnitine attenuates the development of kidney fibrosis in hypertensive rats by upregulating PPAR-γ. Am J Hypertens. 2014;27(3):460–470.