Abstract
Among 131 rice endosperm proteins previously identified by MS-based proteomics, most of the proteins showed low or almost no sequence similarity to known allergens in databases, whereas nine proteins did it significantly. The sequence of two proteins showed high overall identity with Hsp70-like hazel tree pollen allergen (Cor a 10) and barley α-amylase (Hor v 16), respectively, whereas the others showed low identity (28–58%) with lemon germin-like protein (Cit l 1), corn zein (Zea m 50 K), wheat chitinase-like xylanase inhibitor (Tri a XI), and kinase-like pollen allergen of Russian thistle (Sal k 1). Immuno-dot blot analysis showed that recombinant proteins for these rice seed homologs were positive in the IgE-binding, but not necessarily similarity dependent, from some allergic patients. These results suggest that utilization of proteome and sequence databases in combination with IgE-binding analysis was effective to screen and evaluate allergenic potential of rice seed protein components.
Graphical abstract
Allergenic potential of rice seed protein components was evaluated using proteome and allergen databases in combination with IgE-binding analysis.
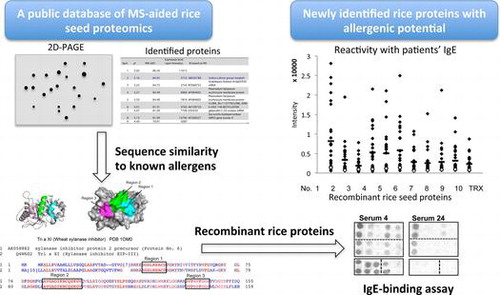
Allergenic potential of a given protein antigen is the ability to give allergic sensitization to naïve individuals and also to elicit allergic symptoms in individuals who have already been sensitized with the given antigen or some other cross-reactive antigens. Although the biochemical and physicochemical properties of a given protein itself is considered to determine the allergenic potential of the protein,Citation1,2) underlining basis of the determination is still not fully understood. Evaluating allergenic potential of protein antigens is also an important practical subject for clinical investigation, diagnosis, and therapy on a variety of allergic diseases. Moreover, as for genetically modified agricultural products, the allergen risk assessment of novel food proteins has attracted attention socially.Citation3)
Cereals including rice are important plant-derived food and feed as major source of calories and, therefore, have been major targets of plant breeding and gene manipulation for development of novel crop cultivars with improved traits.Citation4) At the same time, on the other hand, cereals are known as major causative food of some allergic disorders,Citation5) which are especially serious for people who feed chiefly on cereals. Therefore, assessment of allergenic potential for cereal seed proteins has attracted attention of researchers and stakeholders related to development of novel crop cultivars. Interest of allergenic potential assessment is taken in not only the direct products of introduced exogenous genes but also a variety of endogenous proteins which may increase in relative content as a consequence of breeding or gene manipulation.Citation6)
Sequence and structural similarities of a given protein to known allergens, which have already been characterized biochemically and immunologically, are useful to assess allergenic potential of the protein. Overall amino acid sequence similarity has often been adopted as an index of allergenic potential, according to the WHO/FAO scientific panel recommendation, that is, whether identity matches are above 35% over any 80 amino acid residue segment of the query protein compared to any known allergens.Citation7) Another sequence similarity is also taken into account, especially on immuno-cross reactivity, that short segments consisting of 6–8 amino acid residues of the protein exactly matches any known allergen sequences, simply because eight amino acid residues are approximate size/length for epitopes recognized by IgE antibody.Citation8) Identification and sequencing of a variety of food and respiratory allergens have greatly been increasing, and a large amount of biochemical and immunological information is stored in several public databases (www.ifr.ac.uk/protall/database.html, www.allergen.org/, www.allergenonline.org/, allergen.nihs.go.jp/ADFS/index.jsp?pagen=top, http://www.allergome.org/). In the Allergome database, for example, more than 2,500 allergens excluding isoforms have been registered and more than 7,500 sequences are available at this moment.
A clinical study on rice allergy, asthma, and eczema induced by rice flour exposure or cooked rice ingestion was reported first in Japan.Citation9) Later, in some broad-scale clinical studies with patients allergic to food or cereals, a substantial proportion of the patients was reported to be positive for skin reaction or serum IgE-binding to rice seed protein extracts.Citation10,11) Moreover, a recent clinical study showed that even ingestion of cooked rice could induce immediate-type hypersensitive reactions, including anaphylactic reaction.Citation12,13) Potentially allergenic proteins in rice seeds have been identified and characterized based on the reactivity with patients’ serum IgECitation14). The 14–16-kDa and 33-kDa allergenic proteins were identified to be α-amylase inhibitorsCitation15) and glyoxalase-1,Citation16) respectively. These proteins as well as 26-kDa globulin were suggested to be major allergenic components of rice seeds based on the result that IgE-binding to rice seed proteins was markedly reduced by suppressing the expression of these three proteins in transgenic rice plants.Citation17) On the other hand, allergenic potential of minor components of rice seed proteins remains to be investigated. Putative extensin and polygalacturonase proteins have recently been identified as potential allergens in rice pollen proteins and detected in a trace amount also in rice seed proteins.Citation18)
In this study, we aimed at searching components with allergenic potential by sequence similarity-based screening of rice seed whole proteins, which had previously been identified using MS-based proteomic analysis. Allergenic potential of several rice seed protein components with sequence similarity to known allergens was further estimated by analyzing the IgE-binding to their recombinant proteins.
Materials and methods
Databases and structural analysis
Information on proteins expressed in rice seeds was obtained from the Rice Proteome Database (National Institute of Agrobiological Sciences, http://cdna01.dna.affrc.go.jp/RPD/main.html), while cDNA sequences were from the rice full-length cDNA database, KOME (Knowledge-based Oryza Molecular biological Encyclopedia, http://cdna01.dna.affrc.go.jp/cDNA/). Similarity blast search was done with an allergen database, Allergome (http://www.allergome.org/). Known allergens with sequence similarity to rice seed proteins were searched using NCBI blastp (2.2.18) algorism. Sequence alignment was done using Cobalt, Constraint Based Protein Multiple Alignment Tool (NCBI). Molecular structure diagrams were drawn using the program PyMOL (W.L. DeLano The PyMOL Molecular Graphics System, DeLano Scientific, Palo Alto, CA, 2002).
Preparation of recombinant proteins
Rice full-length cDNA clones were provided by Rice Genome Resource Center (RGRC, AFFRC, Tsukuba, Japan). Using these cDNAs as a template and the primers with sequences corresponding to 5’- and 3’-ends listed in Table , the open reading frame cDNA excluding the signal sequence was amplified and introduced into pET32a expression vector (Novagen, Madison, WI) as described previously.Citation18) His-tagged thioredoxin (TRX) fusion proteins were expressed in E. coli BL21 (DE3) and affinity purified in accordance with manufacturer’s instruction. His-tagged bacterial TRX alone and His-tagged TRX-rice lipid transfer protein (LTP, AK071260) were also prepared as a negative control and a known seed allergen, respectively.
Table 1. Primer pairs for specific amplification of ORF for cDNAs encoding rice putative proteins. Recognition sites for restriction endonucleases are underlined.
SDS-PAGE
Proteins were separated by SDS-PAGE (12.5% acrylamide) according to Laemmli’s method,Citation19) followed by Coomassie Brilliant Blue R-250 (CBB) staining.
Patients’ sera
Human blood samples were obtained from patients with suspected food allergy under medical treatment for atopic dermatitis and/or bronchial asthma at the Fujita Health University Hospital, Department of Pediatrics. The study using the patients’ serum specimens was approved by the ethics committee of Fujita Health University School of Medicine. The informed consents were obtained from each patient or their guardians. Representative sera from 26 patients with higher IgE-ELISA values for rice seed salt-soluble proteins were selected among serum specimens from 60 patients in descending order as test samples (IgE-positive sera), while 2 sera with the lower IgE-ELISA values were selected in ascending order and used as control samples (IgE-negative sera).
The total-IgE concentration of the serum specimens was determined by two-site ELISA using anti-human IgE monoclonal antibody (MCA IgE E-01, Yamasa Corporation, Tokyo) as a capture antibody, HRP-labeled anti-human IgE (Biosource International, Inc., Camarillo, CA) as a detection antibody, and human serum specimens with known total-IgE concentration determined using a test kit (ImmunoCAP, Thermo Scientific Inc., Uppsala, Sweden). ELISA plates (Nunc-Ummuno™, Roskilde, Denmark) were coated with the capture antibody (1 μg/mL in PBS), blocked and incubated with 100-fold diluted human serum specimens. After the incubation with the HRP-labeled detection antibody, HRP activity was measured as described previously.Citation18)
Immuno-dot blotting assay for IgE-binding of recombinant proteins
Binding of human serum IgE to the recombinant proteins was analyzed using immune-dot blotting as described.Citation18) Briefly, sample proteins dissolved in 2 M urea/PBS was spotted on nitrocellulose membrane (1 μg/spot), incubated with human serum diluted by 100-fold, followed by HRP-labeled anti-human IgE (Biosource International, Inc.). HRP activity was detected using a chemiluminescent HRP substrate (Millipore, Billerica, MA). Using NIH Image-J program, the chemiluminescence dot images were standardized by the ‘rolling ball’ algorithm-based subtraction of background chemiluminescence, and the relative chemiluminescence intensity of each dot was quantified.
IgE-binding assay for heat-denatured recombinant proteins
Recombinant rice proteins dissolved in 2 M urea/PBS was further diluted with PBS to 5 μg/mL and heat denatured by boiling for 5 min and cooled immediately on ice. To evaluate possible contribution of disulfide bridges to protein heat stability, the recombinant proteins were diluted with 1% mercaptoethanol in 2 M urea/PBS and heat-treated similarly. IgE-binding to the native and heat-denatured proteins was assayed using ELISA as described previously.Citation18)
Results
Search of rice seed proteins with sequence similarity to known allergens
In the database of rice endosperm proteome, 131 proteins with molecular mass of 14–86 kDa and pI of 4.4~8.8 identified by MS analysis as each spot in 2D(IEF/SDS)-PAGE have been registered with their ID in sequence databases. Using these registered sequences, the blastp similarity search was done for each deduced amino acid sequence of the rice seed proteins against the Allergome database of known allergens. Most of the proteins’ sequence did not show significant similarity to that of any known allergens, but nine out of the 131 rice proteins were found to be homologous to one or more known allergens registered in the allergen database (Table ). Then, full-length cDNA clones corresponding to the nine proteins were retrieved from the rice full-length cDNA database, KOME. The molecular masses of several of the nine proteins shown in the 2D-PAGE were larger than those estimated from the deduced amino acid sequence, probably due to incomplete dissociation of the proteins during the treatment with SDS-containing buffer before the secondary dimension SDS-PAGE (http://cdna01.dna.affrc.go.jp/RPD/main.html).
Table 2. Some biochemical and structural properties of nine rice endosperm proteins with sequence similarity to known allergens and corresponding cDNA clones in the rice full-length cDNA database.
As summarized in Table , overall sequence identity of the rice proteins, except one (AK119653) described below, with known allergens was 70% or less, and four of them gave the identity of 50% or less. Three proteins (AK070271, AK101344, and AK068307), which had been identified as putative protein kinases, resembled a single allergen from pollen of Russian thistle (Sal k 2). Both of the two rice prolamin proteins (AK242325 and AK 242260) resembled prolamin allergens of corn (Zea m 50 kD Zein) and wheat (Tri a 36, LMW-glutenin). In particular, the putative rice luminal binding protein (Bip)/Hsp 70 (AK119653) showed high similarity (~90% identity) to a hazel homolog known as a hazel pollen allergen (Cor a 10), and moderate similarity also to fungal aero allergens, Cla h 4 and Pen c 9, which are heat shock 70 kDa proteins of plant-pathogenic fungi, Mycosphaerella tulasnei. The other three (AK062862, AK073487, and AK058882) were similar to a lemon peel (flavedo) germin-like allergen (Cit l 1), barley α-amylase (Hor v 16), and wheat xylanase inhibitor (Tri a XI), respectively. In summary, the seven known allergens with sequence similarity to the rice proteins included five food allergens of cereal seeds, two inhalant allergens of pollen from native grass and orchard, and one food/pollen allergen of citrus fruit.
Sequence comparison of rice proteins to known allergens
Sequences of three rice putative protein kinases, AK070271, AK10334, and AK068307 (protein Nos. 1, 7, and 9), were aligned with the corresponding homologous allergen, a pollen allergen from Russian thistle (Sal k 2) (Fig. (A)), showing only limited matches of these rice proteins with Sal k 2. There was only one match of 6 contiguous residues with Sal k 2 in AK068307 or AK10334, but not in AK070271.
Fig. 1. Amino acid sequence alignment of putative rice proteins with sequence similarity to known allergens. (A) The sequences of three rice putative protein kinases (the rice proteins Nos. 1, 7, and 9 in Table ) were aligned with that of a pollen allergen from Russian thistle (Sal k 2). Regions are boxed at which six contiguous residues match between the rice protein and Sal k 2. (B) The sequence of rice putative germin-like protein (the rice protein No. 3 in Table ) was aligned with that of a fruit allergen (Cit l 1, germin-like protein) from lemon peel (flavedo). Regions are boxed at which eight contiguous residues or more match between the two. (C) The sequence of rice putative xylanase inhibitor (the rice protein No. 6 in Table ) was aligned with that of a food allergen (Tri a XI, xylanase inhibitor) from wheat seed. Regions are boxed at which nine contiguous residues or more match between the two. The crystal structure of wheat xylanase inhibitor (PDB: 1OM0) is shown as ribbon and surface models (Bottom). The matched contiguous residues in the three regions as well as the disulfide bridge between Cys55 and Cys96 are colored, respectively.
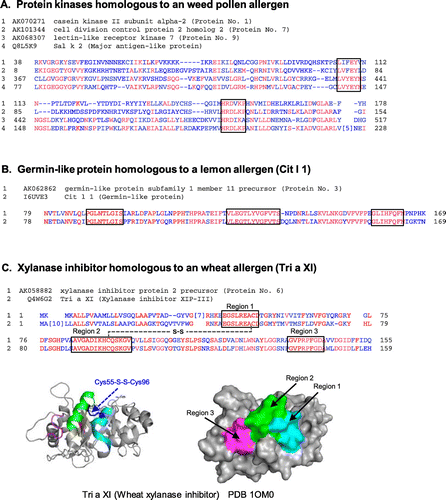
Putative rice prolamin 17, AK242325 (protein No. 5), which resembles another rice prolamin protein, AK242260 (protein No. 8), showed similarity in some parts of whole sequence to several plant food allergens, i.e., maize 50-kDa glutenin/zein (Zea m 50 kDa zein), a large number of wheat LMW-glutelin subunit iso-allergens (Tri a-series), and 2S globulins from sesame (Ses I 1) and Brazil nut (Ber e 1) (Table ). Sequence similarity between these rice prolamin proteins and the cereal seed allergens was found only in limited regions of whole sequences and many of the matched residues were Gln, an abundant amino acid residue of cereal prolamin proteins (data not shown). One segment with 12 contiguous amino acid residues, CQVMQQQCCQQL, of the rice p 17 (AK242325) was conserved in the sequence of wheat LMW-glutenin, Tri a 36, (10 matches, CHVMQRQCCQQL) and corn 50-kDa glutenin, Zea m 50 K, (9 matches, CQVLQQQCCHDL).
The rice putative germin-like protein 16, AK062862 (protein No. 3), was similar to several plant food allergens, including a lemon peel (flavedo) germin-like allergen (Cit l 1), a hazelnut allergen (Cor a 9), and a peanut allergen (Ara h 3), though the overall sequence identity was low (Table ). The sequence of a putative rice germin-like protein was aligned with that of the lemon allergen (Cit l 1) with the highest overall similarity (Fig. (B)). Three complete matches consisting of 8, 9, and 13 contiguous amino acid residues were present between AK062862 and Cit l 1, though overall similarity was 58%.
The sequence of a putative xylanase inhibitor, AK058882 (protein No. 6), was similar to wheat chitinase-like xylanase inhibitor (Tri a XI), a tropical fruit (Indian plum) cross-reacting allergen (Ziz m 1) and a rubber contact allergen, hevamine-A (a chitin-binding protein, Hev b 14) (Table ). The sequence of AK058882 was compared to that of Tri a XI (Fig. (C) top). Overall identity of the rice protein (AK058882) was 50% to the wheat allergen (Tri a XI). There were three complete matches consisting of 9, 9, and 14 contiguous amino acid residues apart each other. These three matched regions were mapped on the 3D diagrams (ribbon and surface models) of Tri a XI (Fig. (C) bottom). The regions 1 and 2 consisting of 9 and 14 matched residues formed two parallel-arranged α-helix structures connected with a disulfide bridge between Cys55 and Cys96 on the molecular surface. The region 3 with nine matched residues was located adjacent to the region 2 with α-helix. Thus, the three regions conserved between the rice putative xylanase inhibitor and wheat allergen, Tri a XI, were mapped adjacently on the surface of the Tri a XI molecule.
The putative rice α-amylase (AK073487) showed high similarity (~70%) to barley seed food allergen, α-amylase (Hor v 16), and low similarity (below 30%) to a fungal occupational aeroallergen (Asp o 21), α-amylase from Aspergillus oryzae (Table ).
IgE-binding to the recombinant rice seed proteins with sequence similarity to known allergens
The nine rice proteins Nos. 1 to 9 (see Table ) were prepared as His-tagged TRX-fusion recombinant proteins and affinity-purified. The SDS-PAGE of these protein preparation showed that the recombinant rice proteins were expressed as the TRX-fusion with molecular mass expected from the size of each full-length cDNA (Fig. (A)). Although expression levels of the rice proteins No. 3 (germin-like protein), No. 5 (prolamin 17), and No. 9 (kinase-like protein) were relatively low as seen in the lanes of total cell lysate, the recombinant proteins for all of the nice rice proteins were prepared in amounts large enough to be used for the following IgE-binding immunoassay. The 28 serum specimens were characterized by measuring the total-IgE concentration using two-site ELISA (Fig. (B)). The total-IgE level differed remarkably from one serum to another, and the average of total-IgE with SD of the 26 rice-positive ones was 2861 ± 2034. The residual 2 serum specimens selected as rice-negative ones showed very low total-IgE concentration.
Fig. 2. Expression and affinity purification of recombinant rice proteins and total-IgE concentration of patients’ serum specimens. (A) The putative rice seed proteins encoded by the nine full-length cDNA clones (GeneBank ID: AK070271, AK119653, AK062862, AK073487, AK242325, AK058882, AK101344, AK242260, and AK068307) were expressed as His-tagged TRX-fusion proteins by an E. coli expression system and purified using Ni-affinity chromatography. Total proteins of transformed E. coli lysate (T) and affinity-purified proteins (P) were analyzed by SDS-PAGE (12.5% acryl amide gel stained with CBB R-250). The protein number (1–9) in this study is shown above each strip of the PAGE gel. The bands of His-tagged TRX-fusion proteins are indicated with black arrowhead. (B Total-IgE concentration of the patients’ serum specimens (rice-positive, Nos. 1 to 26; rice-negative, Nos. 27 and 28) was determined by two-site ELISA using two kinds of anti-human IgE as described in Materials and Methods. The total-IgE concentration is expressed as international units (IU) per mL.
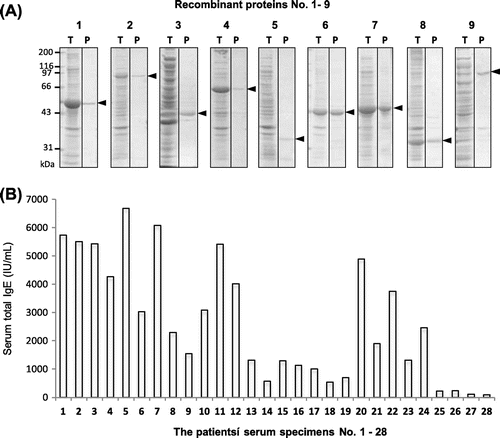
Reactivity with patients’ IgE of the rice proteins was analyzed using immuno-dot blotting with TRX as a negative control as well as rice seed soluble proteins, LTP, and egg ovalbumin (OVA) for comparison. Representative blots are shown in Fig. . In many cases of the 26 serum specimens, markedly positive IgE-binding signals were detected for at least one of the nine recombinant proteins or more, though there was considerable individual variability among the serum specimens tested. Some serum specimens (e.g., Serum 7 and 20) showed IgE-binding most of the nine proteins, while the others only one (e.g., Serum 11 and 21) or a few (e.g., Serum 18 and 24). As a whole, serum specimens with strong IgE-binding to rice seed soluble proteins (positive controls) tended to show marked IgE-binding to the recombinant rice proteins with sequence similarity to known allergens. Exceptionally, a few specimens (e.g., serum 10 and 24) with weak IgE-binding to the rice seed showed strong IgE-binding to some recombinant proteins.
Fig. 3. IgE-binding to recombinant rice proteins with sequence similarity to known allergens.
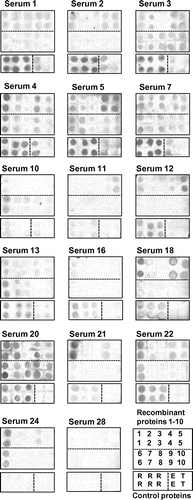
The staining intensity for the IgE-binding of each dot was semi-quantitated and plotted against each protein tested (Fig. (A)). IgE-binding signals of the serum specimens higher than those of the control TRX were detected frequently for the four rice proteins 1, 4, 5, and 6, that is, putative protein kinase (AK070271), α-amylase (AK073487), prolamin 17 (AK242325), and xylanase inhibitor (AK058882), respectively. Moreover, the mean value of the IgE-binding intensity of the four proteins tended to be higher than that of egg albumin but lower than that of rice seed proteins, though a large variation was observed from one serum to another. To analyze the IgE-binding to each rice protein on individual serum specimen in detail, the signal intensity of each spot was divided by that of TRX on each blot, and the values relative to TRX over 2.0 (regarded as positive) were compared among the nine rice proteins and the 26 serum specimens tested (Fig. (B)). The four rice proteins 1, 4, 5, and 6 (AK070271, AK073487, AK242325, and AK058882) gave the positive IgE-binding to 12 (43%) serum specimens or more out of 28. Twelve serum specimens gave strong positive signals (over 5.0) to at least one rice protein. Among them, a half of the serum specimens (serum 4, 7, 11, 12, 20, and 22) showed relatively high total-IgE levels, whereas some others (serum 13, 18, and 26) showed low levels of total IgE (see Fig. (B)).
Fig. 4. Semi-quantitative analysis of IgE-binding to recombinant rice proteins as estimated by immuno-dot blotting. (A) Signal intensity of IgE-binding to each protein dot for the 28 serum specimens was semi-quantified using Image-J and plotted on the nine recombinant rice proteins and the control proteins, LTP, TRX, rice seed proteins, and egg albumin (EA). The mean value of the 26 positive sera is also shown as a horizontal bar. (B) TRX-rice protein fusion/TRX ratio in the IgE-binding intensity is compiled as a matrix of the nine rice recombinant proteins and the 28 serum specimens including 2 negative controls. The relative values above 2.0 and 5.0, which were regarded, respectively, as positive and strong positive in the IgE-binding, were highlighted with gray and black backgrounds, respectively.
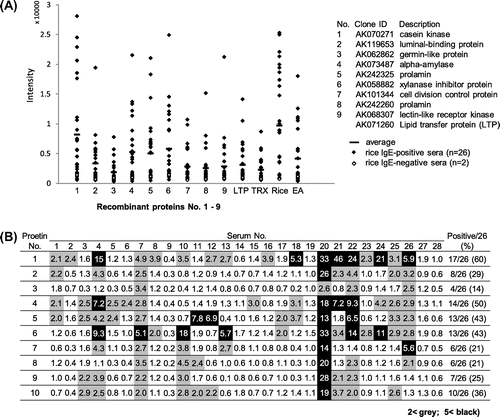
Effect of heat-induce protein denaturation on IgE-binding was examined for rice α-amylase (AK073487) and xylanase inhibitor (AK058882). The IgE-binding as determined using ELISA for three serum specimens available relatively in a large quantity is shown in Fig. , in which net IgE-binding to each rice protein was obtained by subtracting the IgE-ELISA value to TRX as the fusion partner. When the proteins were heat-treated in PBS, in all of the three representative serum specimens, the heat treatment decreased the IgE-binding by 25–50% to α-amylase, but almost not to xylanase inhibitor. By contrast, when they were heat-treated in the presence of 2-mercaptoethanol, IgE-binding was decreased by 25–35% also as to xylanase inhibitor.
Fig. 5. Effect of heat-induced denaturation on the IgE-binding to putative rice α-amylase and xylanase inhibitor. The recombinant proteins of putative rice α-amylase (AK073487) and xylanase inhibitor (AK058882) were heat denatured by boiling in the absence (A) and presence (B) of 2-mercaptoethanol and then subjected to the quantitative IgE-binding analysis using ELISA on the three serum specimens, which were IgE-binding positive and available relatively in large amount. The ELISA values for native (white bars) and heat-denatured (gray bars) proteins are shown as mean ± SE of three determinations.
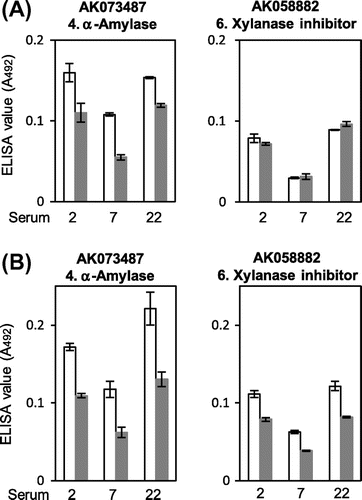
Discussion
Rice proteins expressing in seed endosperm and resembling known allergens in the amino acid sequence were selected and the binding of recombinant proteins with IgE from patients’ serum was analyzed to assess allergenic potential of these rice proteins. Although the IgE-binding was analyzed using a limited number of serum specimens in this study, all of the nine recombinant rice proteins showed positive IgE-binding with 4–17 of the 26 serum specimens (15–65%) as analyzed by immuno-dot blotting, suggesting that the similarity-based search of unknown potential allergens using the NCBI blastp (2.2.18) algorism was reasonable. However, IgE-binding was not necessarily dependent of or correlate with the sequence similarity. For example, the putative rice luminal binding protein (Bip)/Hsp 70 (AK119653) showed high similarity (~90% identity) to a hazel homolog known as a hazel pollen allergen (Cor a 10), but gave relatively low IgE-binding. It seems likely that high IgE-binding of the putative rice Bip/Hsp 70 is detected if the test samples are serum specimens from patients with pollen allergy. It is noteworthy that four (rice proteins 1, 4, 5, and 6) of the nine proteins were positive in the IgE-binding to 13 (50%) serum specimens or more and strongly positive to 3–7 of the 26 serum specimens. These results may indicate that proteins with sequence similarity to known allergens show IgE-binding with high frequency to serum specimens from patients with some sort of allergy. The rice seed protein components reactive with patients’ IgE found in this study had not ever been found in previous studies on rice seed potential allergens, in which traditional procedures had been adopted, i.e., protein fractionation and isolation followed by IgE-binding assay for isolated proteins or IgE-immunoblotting after SDS-PAGE separation of rice proteins. Such unidentification of the potential allergens in these studies may be ascribed to the fact that the rice proteins with allergenic potential found in this study is too small in relative content in rice seed proteins to be purified and/or identified. Therefore, the potential allergens identified in this study are minor components and may not be relevant clinically. However, these potential allergens could be included in the list of rice proteins to be monitored in the safety assessment.
In the safety assessment of IgE immuno-cross reactivity of unknown proteins, short segments consisting of 6–8 amino acid residues have been considered from a viewpoint of sequence-dependent epitopes. Moreover, previous bioinformatic structural studies on known allergens suggested that conformation-dependent cross-reactive epitopes can be predicted and are worth considering for the allergenic potential estimation of novel proteins, when 3D structures of any allergens or related proteins are available.Citation20,21) In this study, the contiguous residues matched in the sequence alignments between rice and wheat xylanase inhibitors were mapped on the 3D structure of the wheat one, showing that several separated segments of the matched residues sterically neighbor and correctively form a surface region in a folded protein molecule. This 3D mapping result suggests that the three matched contiguous residues form a surface area sterically similar to that of the wheat allergen, Tri a XI. Thus, allergenic potential of rice xylanase inhibitor may be higher than expected from the sequence similarity alone. Possible conformation-dependent epitope(s) of the putative rice putative xylanase inhibitor were further examined using heat-treated proteins. In contrast to rice α-amylase, rice putative xylanase inhibitor was heat stable in its IgE-binding ability. It remained to be analyzed further whether the heat stability in IgE-binding was due to its conformational stability to heat or its sequence-dependent IgE-binding ability. Nevertheless, the 25–35% decrease in IgE-binding to putative xylanase inhibitor by the heat treatment in the presence of 2-mercaptoethanol suggested the presence of disulfide-dependent conformational epitopes in putative xylanase inhibitor and would be consistent with the above described assumption that allergenic potential of rice xylanase inhibitor is higher than expected from the sequence similarity alone.
A putative cAMP-dependent protein (Ser/Thr) kinase (AK070271) was one of the three rice protein kinases with sequence similarity to Sal k 2, which had been reported as a potential pollen allergen from Salsola kali (Russian thistle) based on in vitro IgE-binding.Citation22) The sequence of Sal k 2 (Q8L5K9) had been registered in protein databases, but molecular properties of this pollen protein remained uncharacterized. Russian thistle is an annual weed in the genus of Salsola, growing often in arid region of the world. In several Middle East countries, pollen of this weed is known to be one of the main causes of respiratory allergic diseases,Citation23) whereas pollen allergy to the species of Salsola has not been reported so far in East-Asian countries including Japan. The expression level of the protein kinase (AK070271) as estimated by 2D-PAGE is not high in the rice endosperm (http://gene64.dna.affrc.go.jp/RPD/database_en.html), whereas the mRNA of AK070271 is expressed considerably in various tissues, including endosperm and anther, of rice plant (http://ricexpro.dna.affrc.go.jp/). Moreover, possible orthologs (over 95% sequence identity) are present widely in the Poaceae family plant, including cereals, millet, sorghum, and a model grass plant (Brachypodium distachyon). Therefore, it is likely that IgE reacting with the recombinant protein (AK070271) was produced originally against the possible ortholog antigens of seed and pollen from not only rice but also some other plant in the Poaceae family.
Rice α-amylase (AK073487) structurally resembled Hor v 16, presumed to be a barley homolog. The barley α-amylase, Hor v 16, was identified as a potential allergen recognized by patients’ serum IgE with rather low frequency only in two large-scale survey researches using allergen microarray.Citation24,25) Relative abundance of the α-amylase (AK073487) in rice endosperm (see Table ), presence of several isozymes in rice, and presence of possible orthologs (over 70% sequence identity) in some other cereal and millet species suggest that the IgE in the serum specimens used in this study was produced against α-amylase by ingestion of rice and/or some other cereals and millet. Sensitization by inhalation of cereal flour could not be ruled out, as is the case with occupational allergy (asthma) to fungal α-amylase, Asp o 21.Citation26–28)
In this study, allergenic potential of rice seed proteins was assessed and, consequently, several potential allergens in rice seeds were newly identified using a combination of a proteome database, sequence similarity search in known allergen databases, and IgE-binding assay for recombinant proteins of the selected candidates. This approach we took in this study will become more useful with enrichment of the databases, in both identification of potential allergens and assessment of allergenic potential of plant and animal tissues for food and feed. The rice potential allergens newly identified in this study may be additional targets to be monitored in the safety assessment of novel rice varieties developed by molecular breeding or gene manipulation.
Author Contribution
K. Hirano carried out recombinant protein preparation, immunological assay, and data analysis and made manuscript draft. S. Hino generated expression plasmid constructs. K. Oshima and D. Nadano advised on recombinant protein preparation and plasmid construction, respectively. A. Urisu took blood samples and prepared serum. F. Takaiwa advised on research planning and rice databases. T. Matsuda directed the research and completed the manuscript with the other authors’ contributions.
Funding
This work was supported by the Global COE program ‘Advanced Systems-Biology’ from the Ministry of Education, Culture, Sports, Science and Technology of Japan, JSPS KAKENHI [grant number 23658110]; Genomics and Agricultural Innovation from the Ministry of Agriculture, Forestry and Fisheries of Japan to FT in part [grant number GMC0006].
Acknowledgments
The authors thank Drs. K. Kitajima, C. Sato, and T. Okajima for critical comments and suggestion.
Disclosure statement
No potential conflict of interest was reported by the authors.
References
- Astwood JD, Leach JN, Fuchs RL. Stability of food allergens to digestion in vitro. Nat. Biotechnol. 1996;14:1269–1273.10.1038/nbt1096-1269
- Untersmayr E, Jensen-Jarolim E. The role of protein digestibility and antacids on food allergy outcomes. J. Allergy Clin. Immunol. 2008;121:1301–1308.10.1016/j.jaci.2008.04.025
- Goodman RE, Tetteh AO. Suggested improvements for the allergenicity assessment of genetically modified plants used in foods. Curr. Allergy Asthma Rep. 2011;11:317–324.10.1007/s11882-011-0195-6
- Tatham AS, Shewry PR. Allergens to wheat and related cereals. Clin. Exp. Allergy. 2008;38:1712–1726.
- Zuidmeer L, Goldhahn K, Rona RJ, et al. The prevalence of plant food allergies: a systematic review. J. Allergy Clin. Immunol. 2008;121:1210–1218.10.1016/j.jaci.2008.02.019
- Panda R, Ariyarathna H, Amnuaycheewa P, et al. Challenges in testing genetically modified crops for potential increases in endogenous allergen expression for safety. Allergy. 2013;68:142–151.10.1111/all.12076
- Ladics GS, Selgrade MK. Identifying food proteins with allergenic potential: evolution of approaches to safety assessment and research to provide additional tools. Regul. Toxicol. Pharmacol. 2009;54:S2–S6.10.1016/j.yrtph.2008.10.010
- Bannon GA, Ogawa T. Evaluation of available IgE-binding epitope data and its utility in bioinformatics. Mol. Nutr. Food Res. 2006;50:638–644.10.1002/(ISSN)1613-4133
- Shibasaki M, Suzuki S, Nemoto H, et al. Allergenicity and lymphocyte-stimulating property of rice protein. J. Allergy Clin. Immunol. 1979;64:259–265.10.1016/0091-6749(79)90141-6
- Hill DJ, Hosking CS, Zhie CY, et al. The frequency of food allergy in Australia and Asia. Environ. Toxicol. Pharmacol. 1997;4:101–110.10.1016/S1382-6689(97)10049-7
- André F, André C, Colin L, et al. Role of new allergens and of allergens consumption in the increased incidence of food sensitizations in France. Toxicology. 1994;93:77–83.10.1016/0300-483X(94)90198-8
- Kumar R, Srivastava P, Kumari D, et al. Rice (Oryza sativa) allergy in rhinitis and asthma patients: a clinico-immunological study. Immunobiology. 2007;212:141–147.10.1016/j.imbio.2006.11.006
- Trcka J, Schäd SG, Scheurer S, et al. Rice-induced anaphylaxis: IgE-mediated allergy against a 56-kDa glycoprotein. Int. Arch. Allergy Immunol. 2012;158:9–17.10.1159/000330641
- Urisu A, Yamada K, Masuda S, et al. 16-Kilodalton rice protein is one of the major allergens in rice grain extract and responsible for cross-allergenicity between cereal-grains in the Poaceae family. Int. Arch. Allergy Immunol. 1991;96:244–252.10.1159/000235502
- Izumi H, Adachi T, Fujii N, et al. Nucleotide sequence of a cDNA clone encoding a major allergenic protein in rice seeds Homology of the deduced amino acid sequence with members of α-amylase/trypsin inhibitor family. FEBS Lett. 1992;302:213–216.10.1016/0014-5793(92)80443-K
- Usui Y, Nakase M, Hotta H, et al. A 33-kDa allergen from rice (Oryza sativa L. Japonica). cDNA cloning, expression, and identification as a novel glyoxalase I. J. Biol. Chem. 2001;276:11376–11381.10.1074/jbc.M010337200
- Wakasa Y, Hirano K, Urisu A, et al. Generation of transgenic rice lines with reduced contents of multiple potential allergens using a null mutant in combination with an RNA silencing method. Plant Cell Physiol. 2011;52:2190–2199.10.1093/pcp/pcr151
- Hirano K, Hino S, Oshima K, et al. Allergenic potential of rice-pollen proteins: expression, immuno-cross reactivity and IgE-binding. J. Biochem. 2013;154:195–205.10.1093/jb/mvt044
- Laemmli UK. Cleavage of structural proteins during the assembly of the head of bacteriophage T4. Nature. 1970;227:680–685.10.1038/227680a0
- Jenkins JA, Griffiths-Jones S, Shewry PR, et al. Structural relatedness of plant food allergens with specific reference to cross-reactive allergens: an in silico analysis. J. Allergy Clin. Immunol. 2005;115:163–170.10.1016/j.jaci.2004.10.026
- Dall’Antonia F, Gieras A, Devanaboyina SC, et al. Prediction of IgE-binding epitopes by means of allergen surface comparison and correlation to cross-reactivity. J. Allergy Clin. Immunol. 2011;128:872–879.10.1016/j.jaci.2011.07.007
- Assarehzadegan MA, Sankian M, Jabbari F, et al. Allergy to Salsola Kali in a Salsola Incanescens-rich area: role of extensive cross allergenicity. Allergol. Int. 2009;58:261–266.10.2332/allergolint.08-OA-0041
- Ezeamuzie CI, Thomson MS, Al-Ali S, et al. Asthma in the desert: spectrum of the sensitizing aeroallergens. Allergy. 2000;55:157–162.10.1034/j.1398-9995.2000.00375.x
- Scala E, Alessandri C, Bernardi M, et al. Cross-sectional survey on immunoglobulin E reactivity in 23077 subjects using an allergenic molecule-based microarray detection system. Clin. Exp. Allergy. 2010;40:911–921.10.1111/cea.2010.40.issue-6
- Ott H, Weißmantel S, Kennes L, et al. Molecular microarray analysis reveals allergen-and exotoxin-specific IgE repertoires in children with atopic dermatitis. J. Eur. Acad. Dermatol. Venereol. 2014;28:100–107.10.1111/jdv.2013.28.issue-1
- BlancoCarmona J, Picón SJ, Sotillos MG. Occupational asthma in bakeries caused by sensitivity to α-amylase. Allergy. 1991;46:274–276.10.1111/all.1991.46.issue-4
- Losada E, Hinojosa M, Quirce S, et al. Occupational asthma caused by α-amylase inhalation: Clinical and immunologic findings and bronchial response patterns. Journal of Allergy and Clinical Immunology. 1992;89:118–125.10.1016/S0091-6749(05)80048-X
- Smith TA, Lumley KP, Hui EH. Allergy to flour and fungal amylase in bakery workers. Occup. Med. (Lond). 1997;47:21–24.10.1093/occmed/47.1.21