Abstract
Certain dioxins, including 2,3,7,8,-tetrachloro-dibenzo-p-dioxin (TCDD), are exogenous ligands for an aryl hydrocarbon receptor (AhR) and induces various drug-metabolizing enzymes. In this study, we examined the effect of curcumin on expression of drug-metabolizing enzymes through the AhR and NF-E2 related factor 2 (Nrf2) pathways. Curcumin dose-dependently inhibited TCDD-induced expression of phase I enzyme cytochrome P450 1A1 (CYP1A1) and phase II enzymes NAD(P)H:quinone oxidoreductase-1 (NQO1) and heme oxygenase 1 (HO-1) but not tert-butyl hydroquinone-induced NQO1 and HO-1, suggesting that curcumin inhibited only AhR pathway, but not Nrf2 one directly. Furthermore, we used 14 curcumin derivatives and obtained the correlation between hydrophobicity of the compounds and suppressive effect against AhR transformation. Results from the quantitative structure active correlative analysis indicated that methoxy groups and β-diketone structure possessing keto-enol tautomerism in curcumin were necessary to inhibit AhR transformation, and the addition of methyl and methoxy group(s) to the curcumin increased the inhibition effect.
Curcumin inhibited TCDD-induced expression of phase I enzyme CYP1A1 and phase II enzymesNQO1 and HO-1 but not t-BHQ -induced NQO1 and HO-1.
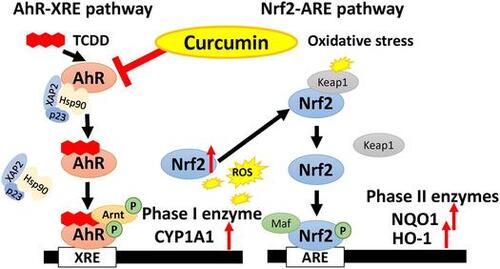
Xenobiotics such as medicine, certain food factors, and environmental pollutions are metabolized in the body, especially in the liver [Citation1]. The leading part of this metabolizing system is constituted by drug-metabolizing enzymes and the intracellular sensor molecules which regulates the expression of drug-metabolizing enzymes. These drug-metabolizing enzymes and their transcription factors are highly exist in the liver, and they are classified into phase I, II, and III by their reaction [Citation1,2]. Cytochrome P450 (CYP) family is one of the phase I enzymes acting at the first stage of the drug metabolism [Citation3]. CYP1, 2 or 3 and 4 families mainly participate in the metabolism of xenobiotics including environmental pollutions [Citation1]. Xenobiotics are oxidized by CYPs, and the major metabolites lose drug activity. However, CYPs sometimes induces toxicity of xenobiotics, which may causes various diseases including cancer [Citation3] For example, CYP1 family metabolizes polycyclic aromatic hydrocarbons (PAHs) such as benzo[a]pyrene (B[a]P) to possess cytotoxicity. B[a]P forms epoxide group during its metabolism, and the formed epoxide causes adduct to DNA and protein, leading to cell damage [Citation4]. Thus, inhibition of CYP expression or activity is involved in the prevention of PAHs-induced carcinogenesis. Phase II reaction is conjugation of glutathione, glucuronic acid, and sulfuric acid to the metabolites of phase I reaction to increase hydrophilicity of metabolites [Citation1,5,6]. Phase II reaction catalyzed by the enzymes such as glutathione transferase (GST), UDP-glucuronic acid transferase (UGT) [Citation5,6]. The metabolites formed by phase II enzymes are transported to the out of cells immediately through the phase III transporters such as ATP-binding cassette transporters [Citation7].
An aryl hydrocarbon receptor (AhR) is a ligand-activated transcription factor with a basic helix-loop-helix structure and is structurally belonging to the Per-Arnt-Sim family [Citation8]. It is known that certain PHAs and halogenated aromatic hydrocarbons (HAHs) including 2,3,7,8,-tetrachloro-dibenzo-p-dioxin (TCDD) are classical exogenous ligands for the AhR and induces CYP1A1 through an AhR-dependent pathway [Citation9]. In the absence of ligands, AhR forms an inactive complex with 90 kDa heat shock chaperon protein [Citation10], hepatitis B virus X-associated protein [Citation2,11] and p23 [Citation12] in cytoplasm. When the ligand binds to the AhR, this receptor dissociates from the complex and traslocates into nucleus [Citation8]. AhR forms heterodimer with AhR nuclear translocator (Arnt), phosphorylated, and the AhR/Arnt heterodimer binds to dioxin/xenobiotics responsive element (DRE/XRE) in nucleus. This activation system is called transformation of AhR [Citation8]. There is DRE/XRE sequences in the promoter domain of CYP1A1 gene, and the mechanism by which TCDD induces expression of CYP1A1 is already clarified [Citation1,2].
On the other hand, phase II drug metabolizing enzymes are regulated by NF-E2 related factor 2 (Nrf2), which is a transcription factor with basic leucine zipper structure to belong to the cap‘n’color family [Citation13]. Nrf2 induces antioxidant gene clusters such as glutathione synthetase and heme oxygenase 1 (HO-1), anti-inflammatory gene clusters such as inducible nitric oxide synthase and cyclooxygenase 2, and phase II drug-metabolizing enzyme clusters such as GST and NAD(P)H quinone oxidoreductase 1 (NQO1) to suppress cellular oxidative stress [Citation13]. Without oxidative stress condition, Nrf2 is suppressed by Kelch-like ECH-associated protein1 (Keap1) and disintegrated by proteasome. When oxidative stress occurs in the cells, Nrf2 dislocates from Keap1, and translocates into nucleus, phosphorylated, and forms a heterodimer with small Maf factor [Citation13]. Nrf2/Maf heterodimer binds to antioxidant/electrophile responsive element (ARE/EpRE) and induces the expression of downstream genes [Citation13]. AhR and Nrf2 regulates phase I enzymes and phase II enzymes independently, but it is reported that crosstalk exists between AhR and Nrf2 [Citation14]. Expression of Nrf2 are induced by TCDD through the transformation of AhR in Hepa-1c1c7 cell derived from a mouse liver tumor cells [Citation14]. In addition, it is reported that DRE/XRE sequences are exist in promoter domain of Nrf2 in mouse gene, and expression of Nrf2 is controlled by AhR [Citation14].
Various chemicals and food factors are known to activate or inactivate AhR. For example, TCDD, a prototypical exogenous ligands of AhR, promotes transformation of AhR and up-regulates the expression of phase I drug-metabolizing enzyme CYP1A [Citation15,19] and phase II enzymes such as NQO1 [Citation16]. In addition to the classic exogenous ligands, certain natural compounds existing in fruits and vegetables bind to the AhR [Citation9,15,17,18]. For example, luteolin in celery and perilla and catechin in tea are reported to act as the antagonist of AhR at low concentration close to physiological condition [Citation17]. On the other hand, these flavonoids are reported to act as the agonists for the AhR at higher concentration [Citation15]. As to Nrf2, tert-butyl hydroquinone (t-BHQ) is a known activator for Nrf2-ARE pathway, and up-regulates expression of HO-1 and other phase II enzymes [Citation19]. Many natural compounds are reported to induce the expression of phase II enzymes through Nrf2 pathway. For examples, quercetin in onion, carnosol in rosemary, and sulforaphane in broccoli are known to activate Nrf2-ARE pathway [Citation20–22]. Our previous report demonstrates that luteolin modulates expression of drug-metabolizing enzymes through the AhR and Nrf2 pathways in hepatic cells [Citation23]. From these reports, the natural compounds in the plant such as flavonoids may regulate expression of drug-metabolizing enzyme.
Curcumin is a yellow pigment in turmeric and has two polyphenolic aromatic rings connected to α, β-unsaturated carbonyl groups [Citation24,25]. In solution, curcumin forms a stable enol type or the enolate type deprotonated in α, β-unsaturation carbonyl groups [Citation24]. Curcumin possesses various bioactivity such as an antioxidant action, anti-inflammatory action, anticancer activity, improvement of immunity and disinfection [Citation24–27]. In addition, curcumin is reported to be both AhR antagonist [Citation28] and agonist [Citation29]. In our previous study, curcumin acts as an antagonist of AhR, and inhibits AhR phosphorylation in Hepa-1c1c7 cells through inhibiting PKCs [Citation28]. On the other hand, curcumin is reported to regulate activation of Nrf2: For example, curcumin induces expression of anti-oxidative enzymes which are regulated by Nrf [Citation2,25,30] and curcumin reduces heavy metal-induced oxidative stress [Citation31]. In this study, we investigated the suppression effects of curcumin on chemical-induced phase I and II drug-metabolizing enzymes through AhR and Nrf2 pathways. Furthermore, we also investigated that the structural characteristics of curcumin against the inhibition of TCDD caused AhR-dependent actions using 14 kinds of crucumin derivatives.
Material and methods
Materials
Curcumin and t-BHQ were purchased from Wako Pure Chemical Industries (Osaka, Japan). TCDD was purchased from AccuStandard (New Haven, CT, USA). Fourteen kinds of curcumin derivatives are kindly given from Dr. Mizushina (Kobe Gakuin University). These chemicals were dissolved in dimethylsulfoxide (DMSO). Chemical structures and calculated n-octanol/water distributed coefficient (CLogP) values of the derivatives were shown in Table . CLogP value was calculated using ChemDraw Ultra, Version 8 (Cambridge Soft Co. Cambridge, MA, USA). Anti-fluorescein isothiocyanate (FITC) antibody, peroxidase-conjugated streptavidin, and tetramethylbenzidine were purchased from DakoCytomation (Kyoto, Japan). Anti-CYP1A1 (Daiichi Pure Chemicals Co. LTD, Tokyo, Japan), anti-NQO1 (Santa Cruz Biotechnology Inc., Santa Cruz, CA, USA), anti-Nrf2 (Santa Cruz Biotechnology), anti-HO-1 (Abcam, Cambridge, England) anti-AhR (Santa Cruz Biotechnology), anti-Arnt (Santa Cruz Biotechnology), anti-LaminB (Santa Cruz Biotechnology), anti-phosphothreonine (Sigma-Aldrich Japan, Tokyo, Japan), anti-β-actin (Cell Signaling Technology, Danvers, MA, USA) and biotinyl secondary anti-Goat (Jackson Immuno Research Lab., West Grove PA, USA) antibodies were used in this study. A 26-bp double-stranded oligonucleotide dioxin response element (DRE) probe corresponded to the 26-bp AhR binding site [Citation32]; 5′-GAT CTG GCT CTT CTC ACG CAA CTC CG-3′ (coding) and 5′-GAT CCG GAG TTG CGT GAG AAG AGC CA-3′ (non-coding) was synthesized at Hokkaido System Science (Sapporo, Japan). All other reagents used were of the highest grade available from commercial source.
Table 1. Structures of curcumin and its derivatives used in this study.
Cells culture
Mouse hepatoma Hepa-1c1c7 cells were cultured in Eagle’s medium (Nissui Pharmaceutical, Tokyo, Japan) containing 10% (v/v) fetal bovine serum (FBS, Sigma-Aldrich), 2 mM l-glutamine, 100 U/mL penicillin and 100 μg/mL streptomycin. Human hepatoma HepG2 cells cultured in Dulbecco’s modified Eagle’s medium (Nissui Pharmaceutical) containing 10% (v/v) FBS, 2 mM l-glutamine, 100 U/mL penicillin and 100 μg/mL streptomycin. Cells were cultured under a humidified atmosphere of 95% (v/v) air and 5% (v/v) CO2 at 37 °C. After the cells were cultured (90% confluent) in a 60 mm dish and washed twice with phosphate buffered saline (PBS), they were treated with curcumin at 0.1, 1, and 10 μM. The cells were then exposed to TCDD at 0.1 nM or t-BHQ at 30 μM for another 24 h.
Preparation of cell lysate and nuclear fraction
HepG2 cells and Hepa-1c1c7 cells in 35 mm dishes were treated with curcumin at 0.1, 1 and 10 μM. The cells were then exposed to TCDD at 0.1 nM or t-BHQ at 30 μM for another 24 h. The cells were washed three times with PBS, then, harvested and homogenized with RIPA lysis buffer consisting of 50 mM Tris-HCl, pH 8.0, 150 mM NaCl, 1% (v/v) NP-40, 0.5% (w/v) deoxycholic acid, 0.1% (w/v) sodium dodecyl sulfate and 0.5 mM dithiothreitol (DTT) containing protease inhibitors [1 mM phenylmethylsulfonyl fluoride (PMSF), 5 μg/mL leupeptin and 5 μg/mL aprotinin] and phosphatase inhibitors [10 mM NaF and 1 mM Na3VO4] and placed on ice for 1 h with occasional mixing. The homogenate was centrifuged at 20,000 × g for 20 min at 4 °C, and the resulting supernatant was referred to as cell lysate.
Preparation of the post-nuclear and nuclear fraction was carried out according to previous method [Citation28]. Briefly, after washing the cells three times with PBS, the cells were harvested with lysis buffer consisting of 20 mM HEPES, pH 7.6, 20% (v/v) glycerol, 10 mM NaCl, 1.5 mM MgCl2, 0.2 mM EDTA and 0.5 mM DTT containing the same protease inhibitors and phosphatase inhibitors. The mixture was centrifuged at 1,000 × g for 10 min at 4 °C, and the supernatant obtained was used as a post-nuclear fraction. The precipitate obtained was suspended in hypertonic buffer consisting of 20 mM HEPES, pH 7.6, 20% (v/v) glycerol, 420 mM NaCl, 1.5 mM MgCl2, 0.2 mM EDTA and 0.5 mM DTT containing the protease and phosphatase inhibitors. This suspension was placed on ice for 1 h with occasional mixing and centrifuged at 15,000 × g for 20 min at 4 °C, and the supernatant was collected and used as a nuclear fraction.
Immunoprecipitation
Nuclear fraction from Hepa-1c1c7 cells containing 500 μg proteins was pretreated with Protein A/G PLUS-Agarose beads (Santa Cruz Biotechnology) for 1 h. After the beads were removed by centrifugation, the fraction was incubated with 1.5 μL of anti-phosphothreonine antibody over night at 4 °C. The immune-complex was mixed with 15 μl of Protein A/G PLUS-Agarose. After the mixture was rotated for 1 h at 4 °C, the beads were washed twice with TBS containing 0.05% Tween 20 (TBS-T). Immunoprecipitation products were applied to western blotting analysis.
Western blotting analysis
The cell lysates were used for the detection of AhR, CYP1A1, NQO1, Nrf2, and β-actin by western blotting following the sodium dodecyl sulfate-polyacrylamide gel electrophoresis. Detection of CYP1A1, NQO1, Nrf2 and β-actin was performed using a 12% gel, whereas AhR used 8% gel. The proteins were transferred onto a polyvinylidene fluoride membrane (GE Healthcare Bio-Science Co., Piscataway, NJ, USA). The membrane was treated with commercial blocking solution (Blocking One, Nacalai Tesque, Kyoto, Japan) for 1 h at room temperature. The membrane was incubated with primary antibodies for AhR (1:10000), CYP1A1 (1:10000), NQO1 (1: 10000), and Nrf2 (1:10000) overnight at 4 °C, followed by the corresponding HRP-conjugated secondary antibody (1:100000) for 1 h at room temperature. The blots were developed using Immuno Star ® LD (Wako Pure Chemical, Osaka, Japan) Western Blotting Substrate and detected by Light-Capture II (ATTO, Tokyo, Japan). The density of the specific band was determined using ImageJ software (National Institutes of Health, Bethesda, MD, USA).
Southwestern chemistry-based ELISA (SW-ELISA)
The transformation of AhR was determined by SW-ELISA according to the previous report [Citation33]. Briefly, a 96-well microplate was courted with 100 μL of 4.6 μg/mL anti-FITC anti-body in 50 mM NaHCO3 buffer (pH 9.6) overnight at 4 °C. After washed three times with TBS-T, the plate was blocked with chemical blocking buffer (Nacalai Tesque, Kyoto, Japan) for 2 h at 20 °C, and washed three times with TBS-T. One hundred microliters of FITC-labeled DRE probe (250 fmol/mL) were added to the plate, followed by 2 h-incubation at 20 °C. The reaction mixture consisted of 10 μL of HEDGE buffer (25 mM HEPES, pH 7.4, 1.5 mM EDTA, 10% glycerol, 1 mM DTT) containing 750 mM KCl (final concentration at 150 mM) and 40 μL of nuclear fraction of cells. Aliquot of 50 μL of the reaction mixture containing the transformed AhR was plated onto DRE probe-bond 96-well microtiter, incubated for 2 h at 20 °C, and the plate was washed four times with TBS-T. One hundred microliters of the solution containing anti-Arnt goat IgG were added to each well, incubated for 1 h at 20 °C, and then the plate was washed three times with TBS-T. The detection of the bound specific antibody was carried out by incubating with biotinylated anti-goat IgG antibody, and the plate was washed three times with TBS-T and incubation with peroxidase-conjugates streptavidin for 30 min at 20 °C. Finally, the microtiter plate was washed three times with TBS-T and once with PBS. The bound peroxidase activity was visualized with tetramethylbenzidine. Adding 2 M sulfuric acid stopped color development with tetrametylbenzidine, and result was measures using Wallac ARVO sx multilabel counter (Perkin-Elmer Life Science, Boston MA, USA) at OD 450 nm.
Measurement of ethoxyresorufin-O-deethylase (EROD) activity
EROD activity as a catalytic measurement of cytochrome P450 1A induction in Hepa-1c1c7 cells was measured according to the previous report [Citation34]. The cells treated with 10 μM curcumin and its derivatives and/or 0.1 nM TCDD for 24 h, medium was changed to fresh one containing 1 μM 7-ethoxyresolfin as a substrate and 10 μM dicumarol and incubated for 1 h. Aliquots of 75 μL of the medium were withdrawn from each well and transferred to another 96-well plate. The dealkylated resorufin formed and released into culture medium was quantified, after the hydrolysis of the resorufin conjugates by treatment with 4.6 units/mL glucuronidase and 4.6 units/ml sulfatase. Cell monolayers on the 96-well plate were used for measurement of cell density by staining with crystal violet.
Quantitative structure active correlative (QSAR) analysis
QSAR analyses of suppressive effect on TCDD-induced EROD activity and AhR transformation were performed by Chemometrics software, Chemish (ChemInfoNavi, Yamaguchi, Japan). We used the back-propagation (BP) method that was one of the hierarchical neural networks [Citation35]. To carry out BP method, structures of curcumin and 14 curcumin derivatives in Table were converted to input data in Table . As main structure is common in all compounds, substituted groups were expressed as numerical values in each position. At R1 to R4 in Table , –OCH3, –OCOCH3, –OH and H were expressed as 1, 2, 3 and 4, respectively. At R5 and R7, –OH and = O were expressed as 1 and 2, respectively. At R6, –H2, double bond and (CH3)2 were expressed as 1, 2 and 3, respectively. At bonds B1 and B2, single and double bonds were expressed as 1 and 2, respectively. The case except above was expressed as 0. Since input data in Table was too large for applying into BP, the important variables were selected by genetic algorism.
Table 2. Input data of used curcumin derivatives for QSAR analysis.
Statistical analysis
The data are expressed as the mean ± SD of at least three independent determinations for each experiment. Dunnett’s test was used to determine the significance of differences between the treated and control groups. Statistical analysis was performed with JMP statistical software version 11.2.0 (SAS Institute. Cary, NC). The level of statistical significance was set to p < 0.05.
Results
The effect of curcumin on TCDD-induced phase I and II drug-metabolizing enzymes
It is known that TCDD induces various genes and proteins for drug-metabolizing enzymes [Citation9,14–16]. As shown in Figure (a), TCDD induced the protein expression of not only phase I drug-metabolizing enzyme CYP1A1, but also transcription factor for phase II drug-metabolizing enzymes Nrf2, and its downstream phase II drug-metabolizing enzymes NQO1 and HO-1 in human HepG2 cells. It was found that curcumin inhibited TCDD-induced protein expression of CYP1A1, Nrf2, NQO1, and HO-1 in a dose-dependent manner in HepG2 cells compared with the cells treated with TCDD alone. Curcumin itself at 10 μM did not affect expression of these enzymes. Since it is known that the AhR-dependent action of TCDD differs among species [Citation36], the same experiments were carried out in mouse Hepa-1c1c7 cells. We confirmed that curcumin showed similar effects; i.e. curcumin also inhibited TCDD-induced protein expression of CYP1A1, Nrf2, NQO1, and HO-1 dose-dependently in Hepa-1c1c7 cells (Figure (b)). Under our experimental conditions, curcumin did not show any cytotoxicity up to 10 μM against used hepatocytes estimated by crystal violet staining (Data not shown).
Figure 1. Effect of curcumin on TCDD-induced protein expression of phase I and phase II drug metabolizing enzymes in HepG2 cells and Hepa-1c1c7 cells. (a) HepG2 cells and (b) Hepa-1c1c7 cells were treated with the indicated concentrations of curcumin. The cells were then exposed to 0.1 nM TCDD or DMSO alone as a vehicle control for 24 h. Cell lysates were prepared and used for measurement of protein expression of CYP1A1, NQO1, Nrf2, HO-1 and β-actin by western blotting analysis with their respective antibodies. Typical representative data are shown from three independent experiments. Density of specific band was measured and shown after normalization by β-actin expression. The value of TCDD alone was set to 1. The results are represented as the mean ± SD (n = 3). Asterisks indicate significant difference from the value of TCDD alone by Dunnett’s test (*; p < 0.05, **; p < 0.01).
The effect of curcumin on t-BHQ-induced phase II drug-metabolizing enzymes
Figure shows that the effect of curcumin on t-BHQ-induced protein expression of Nrf2 and phase II drug-metabolizing enzymes. Curcumin did affect neither t-BHQ-induced the expression of NQO1 nor HO-1 at protein levels in HepG2 cells (Figure (a)). Curcumin itself at 10 μM did not alter the expression of these proteins. The same trend was also observed in Hepa-1c1c7 cells; i.e. curcumin did not affect t-BHQ-induced protein expression of NQO1 and HO-1 in Hepa-1c1c7 (Figure (b)). These results suggest that curcumin does not affect Nrf2 pathway, but it affects AhR pathway specifically and inhibits expression of its downstream drug-metabolizing enzymes.
Figure 2. Effect of curcumin on t-BHQ-induced protein expression of phase II drug-metabolizing enzymes in HepG2 cells. (a) HepG2 cells and (b) Hepa-1c1c7 cells were treated with the indicated concentrations of curcumin. The cells were then exposed to 30 μM t-BHQ or DMSO alone as a vehicle control for 24 h. Cell lysates were prepared and used for measurement of protein expression of Nrf2, NQO1, HO-1 and β-actin by western blotting analysis with their respective antibodies. Typical representative data are shown from three independent experiments. Density of specific band was measured and shown after normalization by β-actin expression. The value of TCDD alone was set to 1. The results are represented as the mean ± SD (n = 3). Asterisks indicate significant difference from the value of TCDD alone by Dunnett’s test (*; p < 0.05, **; p < 0.01).
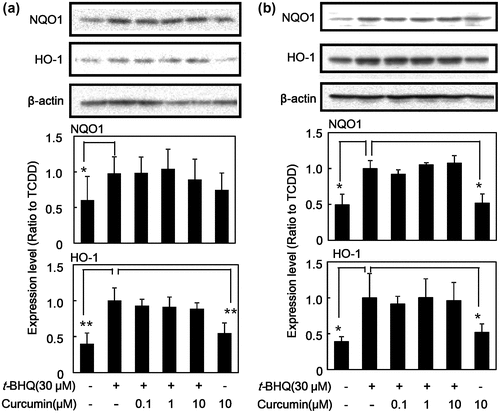
The effect of curcumin derivatives on TCDD-induced EROD activity and expression of CYP1A1
To obtain information on important moiety of curcumin structure for the suppressive effect on TCDD-caused AhR-dependent actions, we introduced synthesized 14 kinds of curcumin derivatives (Table ). As shown in Figure (a), curcumin suppressed TCDD-induced EROD activity as expected and compounds #2 and #3, which have another two methoxy groups at benzene rings and two methyl groups at β-carbon of diketone structure compared with curcumin, showed stronger suppressive activity than curcumin. On the other hand, compounds having acetyl group(s) at benzene rings lost the suppressive activity (#1, #8, and #10–13). Compounds neither having double bounds in carbon chain between two benzene rings (#5–7, #10–12 and #14) nor lost the β-diketone structure possessing keto-enol tautomerism (#4, #8 and #9) did not suppressed EROD activity. These results suggest that methoxy groups in the benzene rings, double bounds in carbon chains between two benzene rings, and β-diketone structure possessing keto-enol tautomerism are necessary to inhibition of the AhR-dependent actions in curcumin. To confirm the effect of curcumin derivatives on the EROD activity, protein expression of CYP1A1 was examined by western blotting using selected derivatives. As shown in Figure (b), compounds #2 and #3, in addition to parent compound curcumin strongly suppressed TCDD-induced CYP1A1 expression similar to the result of EROD activity.
Figure 3. Effect of curcumin derivatives on TCDD-induced EROD activity CYP 1A1 expression in Hepa-1c1c7 cells. Hepa-1c1c7 cells were treated with 10 μM of curcumin derivatives. The cells were then exposed to 0.1 nM TCDD or DMSO alone as a vehicle control for 24 h. (a) EROD activity was measured as described in Materials and Methods. (b) Cell lysates were prepared and used for measurement of protein expression of CYP1A1 and β-actin by western blotting analysis with their respective antibodies. Typical representative data are shown from three independent experiments. Density of specific band was measured and shown after normalization by β-actin expression. The value of TCDD alone was set to 1. The results are represented as the mean ± SD (n = 3). Asterisks indicate significant difference from the value of TCDD alone by Dunnett’s test (*; p < 0.05, **; p < 0.01).
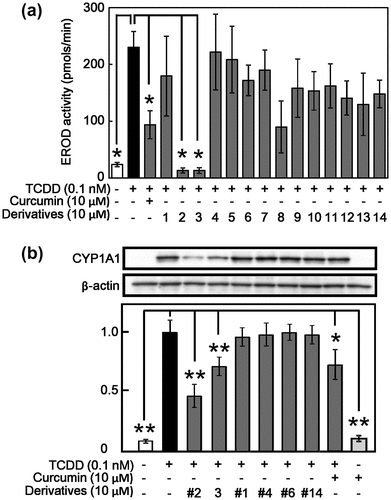
The effect of curcumin derivatives on TCDD-induced activation of AhR
AhR induces expression of CYP1A1 after binding DRE on DNA [Citation8,9,15]. Therefore, we next investigated the effect of curcumin and its derivatives on the binding ability of AhR to DRE by SW-ELISA. TCDD at 0.1 nM significantly increased the binding activity of AhR to DRE (OD 450 nm: 0.401 ± 0.018) approximately 1.4 times compared with DMSO treated control (0.287 ± 0.011) as expected. Curcumin significantly suppressed TCDD-increased DNA binding of AhR (0.345 ± 0.017). After the value of TCDD alone was set to 100%, suppression effect of curcumin derivatives are calculated (Figure ). Except for compounds #1, #4, and #6, all derivatives suppressed TCDD-increased AhR binding to DRE, especially compounds #2 and #3 showed strong suppressive effect.
Figure 4. Effect of curcumin derivatives on TCDD-induced binding activity of AhR to DRE in Hepa-1c1c7 cells. Hepa-1c1c7 cells were treated with the indicated concentrations of curcumin and derivatives, then exposed to 0.1 nM TCDD or DMSO alone as a vehicle control for 2 h. Nuclear fraction of these cells were prepared. Binding activity of AhR to DRE sequence was measured by SW-ELISA as described in Materials and Methods. Typical representative data are shown from more than three independent experiments. The value of TCDD alone was set to 100%. The results are represented as the mean ± SD (n = 3). Asterisks indicate significant difference from the value of TCDD alone by Dunnett’s test (*; p < 0.05).
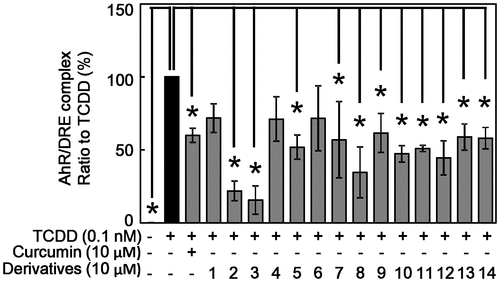
The effect of curcumin derivatives on AhR phosphorylation
Among these sequential processes of AhR transfomation, phosphorylation of AhR is important to the DNA binding activity of the AhR/Arnt heterodimer [Citation37,38]. Thus, we estimated phosphorylation of AhR in nucleus using compounds #2 and #3. As shown in Figure , both curcumin derivatives #2 and #3, in addition to curcumin, significantly inhibited TCDD-induced AhR phosphorylation. The derivatives #2 and #3 showed stronger effect than curcumin as the same manner as the inhibition of EROD activity and CYP1A1 expression (Figure ) and DNA binding activity (Figure ).
Figure 5. Effect of curcumin derivatives on TCDD-induced AhR phosphorylation. Hepa-1c1c7 cells were treated with the indicated concentrations of curcumin and derivatives (#2 and 3), then exposed to 0.1 nM TCDD or DMSO alone as a vehicle control for 2 h. Nuclear fraction of these cells were prepared. Phosphorylation of AhR was measured by immunoprecipitation with p-threonine antibody and immuno blotting analysis with AhR antibody. Typical representative data are shown from three independent experiments. Density of specific band was measured and shown after normalization by β-actin expression. The value of TCDD alone was set to 1. The results are represented as the mean ± SD (n = 3). Asterisks indicate significant difference from the value of TCDD alone by Dunnett’s test (*; p < 0.05, **; p < 0.01).
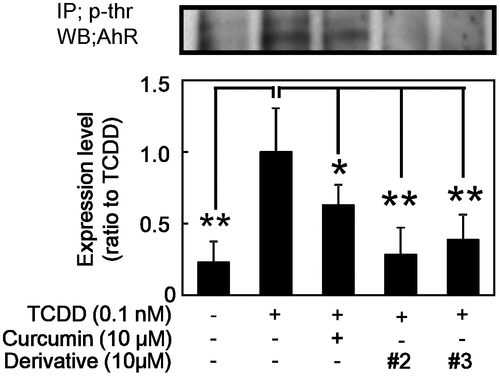
Correlation between CLogP and AhR suppressive effect of curcumin derivatives
Curcumin derivatives #2 and #3, which have additional methoxy groups at benzene rings and two methyl groups at β-carbon of carbonyl groups, strongly inhibited TCDD-induced AhR transformation (Figures and ). Addition of methoxy and methyl groups generally increases the hydrophobicity of the compound. Therefore, we examined the correlation between CLogP value and suppressive effect on AhR transformation of curcumin derivatives. Multiple correlation coefficient (value of R2) between CLogP value and suppressive effect on TCDD-induced EROD activity was 0.7228 (Figure (a)), while that between CLogP value and suppressive effect on AhR binding to DRE was 0.4336 (Figure (b)). These results indicated that correlation was observed between the hydrophobicity and suppression of AhR transformation of used derivatives.
Figure 6. Correlation between CLogP value and suppressive effect on AhR transformation of curcumin derivatives and QSAR analysis. (a and b) Correlation between CLogP and suppressive effect on EROD activity (a) or binding activity of AhR to DRE (b) of curcumin derivatives are shown. (c and d) Correlation between calculated value resulted from QSAR analysis and measured values of EROD activity (c) or binding activity of AhR to DRE (d) of curcumin derivatives are shown.
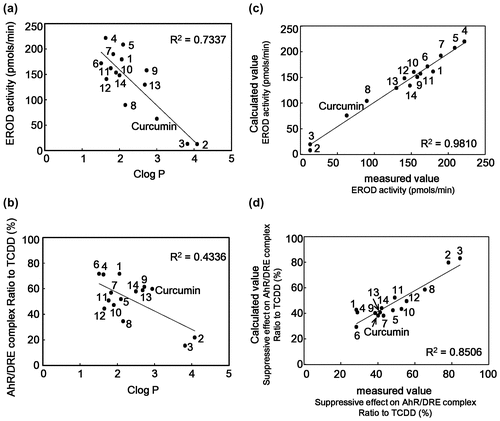
QSAR analysis for relationship between the structure of curcumin derivatives and their suppression effects on AhR transformation
To confirm the relationship between the structure of curcumin derivatives and their suppression effects on AhR transformation, we performed QSAR analysis. Results from suppressive effects on TCDD-induced EROD activity (Figure ) and on DNA binding activity (Figure ) were used as the events of AhR transformation. Theoretical values were calculated by Chemish (ChemInfoNavi) with the BP method [Citation35] and plotted against the measured values from Figures and . As shown in Figure (c) and (d), good correlation coefficients were observed between the measured values and calculated ones: R2 for the EROD and DNA binding activities were 0.9810 and 0.8506, respectively. When the important variables were selected by genetic algorism, variables in entry 2, 3, and 4 in Table were important for the suppressive effects on TCDD-induced EROD activity, while those in entry 2 and 6 were for the suppressive effect on AhR transformation. These QSAR analysis data also supported that the methoxy groups and β-diketone structure of curcumin were important to the inhibition of TCDD-caused AhR transformation.
Discussion
Curcumin is a natural yellow pigment in curcuma longa, and has various health beneficial functions such as antioxidant, anti-inflammatory and anti-cancer activities [Citation24,25]. In our previous study, curcumin suppressed TCDD-induced AhR transformation and its related events in Hepa-1c1c7 cells [Citation28]. In this study, we investigated the effect of curcumin on TCDD- and t-BHQ-induced drug-metabolizing enzymes in cultured hepatocytes, and found that curcumin suppressed TCDD-induced protein expression of phase I enzyme CYP1A1 and phase II enzymes NQO1 and HO-1 in HepG2 and Hepa-1c1c7 cells in a dose-dependent manner (Figures and ), accompanied by the suppression of AhR transformation estimated by DNA binding activity (Figure ) through inhibiting phosphorylation of the AhR (Figure ). These results indicate that there is no difference among species on the inhibitory effect of curcumin against the expression of drug-metabolizing enzymes. On the other hand, curcumin did not affect t-BHQ-induced expression of phase II enzymes NQO1 and HO-1 (Figure ). Using curcumin derivatives and QSAR analysis, methoxy groups and β-diketone structure are important for suppressing the expression of drug-metabolizing enzymes (Figures ). Thus, curcumin specifically inhibited the AhR-dependent induction of phase I and phase II drug-metabolizing enzymes, but it failed to inhibit Nrf2-dependent induction of phase II drug-metabolizing enzymes.
Drug metabolism is classified into phase I and II enzymes and phase III transporters [Citation1,2,15]. The cellular detoxification system including drug metabolism plays an important role in protecting the cells from ultraviolet irradiation, heavy metals, and other environmental pollutants including PAHs and HAHs. It is known that certain PAHs and HAHs including TCDD induce CYP1A1 and 1A2 and these enzymes catalyze the metabolic activation of certain PAHs such as B[a]P to generate genotoxic metabolites that bind DNA to form adducts [Citation4]. These active metabolites mediate initiation of carcinogenesis. In this study, we confirmed that TCDD increased CYP1A1 expression, which is regulated by AhR transformation, in HepG2 and Hepa-1c1c7 cells (Figure ). Among the transformation processes, AhR translocates into the nucleus and forms a heterodimer with its partner molecule Arnt [Citation1,2,15]. This AhR/Arnt heterodimer functions as a transcription factor, and binds to the DRE and induces expression of various genes encoding drug-metabolizing enzymes including CYP1A1 [Citation9,15]. Curcumin decreased the TCDD-induced CYP1A1 expression (Figure ) as expected. In the previous study, we showed that curcumin suppressed TCDD-induced AhR transformation through inhibiting phosphorylation of both AhR and Arnt followed by inhibited their heterodimerization in Hepa1c1c7 cells, though this compound allowed TCDD-evoked translocation of AhR in the nucleus [Citation28]. In this study, we also confirm that curcumin inhibited phosphorylation of AhR in HepG2 cells (Figure ). These results suggest that curcumin is a modulator for the AhR, but not act as its antagonist. AhR regulates not only expression of phase I enzymes, but also phase II enzymes and phase III transports [Citation14]. In the present study, curcumin also inhibited TCDD-induced expression of Nrf2 and its downstream phase II drug-metabolizing enzymes (Figure ). From these results, curcumin inhibited phosphorylation of AhR followed by suppressed TCDD-induced drug-metabolizing enzymes in hepatocytes. Our previous reports demonstrated that protein kinase C [Citation28] and extracellular signal-regulated kineses (ERK1/2) [Citation39] were involved in phosphorylation of AhR. Further study is needed to clarify which kinase is a target of curcumin.
It was noteworthy that curcumin did not inhibit t-BHQ-induced phase II drug-metabolizing enzymes (Figure ). Moreover, curcumin itself did not induce both phase I and phase II drug-metabolizing enzymes (Figures and ). These results are identical with our previous in vivo ones that oral administration of curcumin at 10 mg/kg body weight for three successive days did not increase CYP 1A1 expression and EROD, quinone reductase, and GST activities [Citation40]. However, there is discrepancy between our results and others: It was reported that curcumin induced phase II enzymes such as NQO1, HO-1 and GST [Citation41–44]. It is known that Nrf2 plays a pivotal role in the induction of phase II enzymes [Citation13,45]. These are two mechanisms of Nrf2 activation: phosphorylation of Nrf2 and modification of Kelch-like ECH-associated protein 1 (keap1). Various protein kinases activate Nrf2 and affect the process for detachment of Nrf2 from keap1 that increases Nrf2 stability and its translocation into nucleus [Citation45]. Phosphorylation of Nrf2 at serine 40 is necessary for detachment of Nrf2 from keap1 [Citation45]. t-BHQ induces phase II drug-metabolizing enzymes through ERK2 activation [Citation46]. On the other hand, curcumin was reported to activate p38 MAPK but not ERK in human hepatoma HUH7 cells [Citation42]. However, we did not observe Nrf2 translocation into nucleus by curcumin, while t-BHQ didwwwww (data not shown). We assume that the discrepancy between our results and others are due to the difference in used cell lineage.
In the present study, we found that methoxy groups and β-diketone structure possessing keto-enol tautomerism in curcumin were necessary to inhibit AhR transformation using curcumin derivatives and QSAR analysis. Moreover, we also found that CLogP value of the derivatives correlate to the suppressive effect on EROD activity and DNA binding activity (Figure ). In the previous study, we demonstrated that curcumin is able to bind to the AhR in a cell free system, but suppresses AhR transformation by inhibiting phosphorylation of AhR [Citation28]. These results suggest that methoxy groups and β-diketone structure in curcumin are important to interact with certain kinases such as protein kinase C and ERK1/2 and AhR. It was reported that amide group in Gln381 in the ligand binding domain of AhR is important chemical side-chain to form hydrogen bond with exogenous ligands [Citation47]. β-Diketone structure in curcumin may form hydrogen bond amide group in Gln381 in the ligand binding domain of AhR. However, interaction of curcumin with AhR is not critical [Citation28]. We assume curcumin may form hydrogen bond with certain kinase(s) that is involved in phosphorylation of the AhR. Among curcumin derivatives used in this study, compounds #2 and #3 showed stronger activity than the parent compound curcumin (Figures and ). They also showed stronger inhibition against phosphorylation of the AhR than curcumin (Figure ), suggesting that they inhibited AhR transformation with the same molecular mechanism as curcumin. Compounds #2 and #3 have additional methoxy groups at benzene rings and two methyl groups at β-carbon of carbonyl groups. These functional groups will contribute to increase hydrophobicity of the molecules, and may lead to promote cellular intake and increase affinity to the AhR, though it needs further study such as docking simulation in future.
One important question is arisen: whether curcumin aglycone is present in the body at effective concentrations. Most of aglycone of polyphenols is conjugated in the intestines and liver before being excreted into urine, but small amounts of unchanged aglycone may exist in the body [Citation15]. Although curcumin is reported to its low-bioavailability [Citation48], it is reported that when curcumin was administrated at 25 mg/kg body weight to male Wistar rats, the concentration of curcumin in the liver reached about 0.5 nmol/mg protein 15 min after administration [Citation49]. It is also reported that micelle formation or emulsion formation improves absorption of curcumin [Citation50]. As to the dioxins, their contamination levels in food is usually single pg WHO-TEQ/g fat [Citation51]. This suggests that exposure levels of dioxins are much lower than used TCDD concentration in this study, and therefore, physiological concentration range of curcumin may inhibit TCDD-induced drug-metabolizing enzymes in hepatocytes.
In conclusion, curcumin inhibited AhR transformation and modulated its downstream expression of phase I and II drug-metabolizing enzymes. Curcumin attenuated AhR transformation, but not Nrf2 directly. Therefore, curcumin may beneficial to inhibit chemical carcinogen-induced carcinogenesis. Methoxy groups in the benzene rings and β-diketone structure in curcumin are necessary to inhibit AhR transformation, and the addition of methoxy group(s) to the benzene rings and methyl groups at β-carbon of carbonyl groups increases the inhibition effect. Curcumin may acts as an AhR modulator in human hepatocytes, but further experiments are needed to investigate its effect on TCDD-caused toxicity such as affection of cell differentiation and immunotoxicity.
Author contributions
HA designed the study and supervised experiments. RN and SF performed biochemical experiments and analyzed data. MK carried out QSAR analysis. RN, YY and HA wrote the manuscript. All authors reviewed and approved the final manuscript.
Disclosure statement
No potential conflict of interest was reported by the authors.
Funding
This work was supported in part by Japan Society for the Promotion of Science (JSPS) KAKENHI [grant number 17H00818] to HA and YY.
References
- Xu C, Li C, Kong AN. Induction of phase I, II and III drug metabolism/transport by xenobiotics. Arch Pharm Res. 2005;28:249–268.10.1007/BF02977789
- Nakata K, Tanaka Y, Nakano T, et al. Nuclear receptor-mediated transcriptional regulation in phase I, II, and III xenobiotic metabolizing systems. Drug Metab Pharmacokinet. 2006;21:437–457.10.2133/dmpk.21.437
- Nebert DW. Dalton TP the role of cytochrome P450 enzymes in endogenous signalling pathways and environmental carcinogenesis. Nat Rev Cancer. 2006;6:947–961.10.1038/nrc2015
- Badal S, Delgoda R. Role of the modulation of CYP1A1 expression and activity in chemoprevention. J Appl Toxicol. 2014;34:743–753.10.1002/jat.v34.7
- Sheehan D, Meade G, Foley V, et al. Structure, function and evolution of glutathione transferases: implications for classification of non-mammalian members of an ancient enzyme superfamily. Biochem J. 2001;360:1–16.10.1042/bj3600001
- Guillemette C. Pharmacogenomics of human UDP-glucuronosyltransferase enzymes. Pharmacogenomics J. 2003;3:136–158.10.1038/sj.tpj.6500171
- Dermauw W, Van Leeuwen T. The ABC gene family in arthropods: comparative genomics and role in insecticide transport and resistance. Insect Biochem Mol Biol. 2014;45:89–110.10.1016/j.ibmb.2013.11.001
- Landers J, Bunce N. The Ah receptor and the mechanism of dioxin toxicity. Biochem J. 1991;276:273–287.10.1042/bj2760273
- Denison MS, Pandini A, Nagy SR, et al. Ligand binding and activation of the Ah receptor. Chem Biol Interact. 2002;141:3–24.10.1016/S0009-2797(02)00063-7
- Pongratz I, Mason G, Poellinge L. Dual roles of the 90-kDa heat shock protein hsp90 in modulating functional activities of the dioxin receptor. Evidence that the dioxin receptor functionally belongs to a subclass of nuclear receptors which require hsp90 both for ligand binding activity and repression of intrinsic DNA binding activity. J Biol Chem. 1992;267:13728–13734.
- Hollingshead B, Petrulis J, Perdew G. The aryl hydrocarbon (Ah) receptor transcriptional regulator hepatitis B virus X-associated protein 2 antagonizes p23 binding to Ah receptor-Hsp90 complexes and is dispensable for receptor function. J Biol Chem. 2004;279:45652–45661.10.1074/jbc.M407840200
- Shetty P, Bhagwat B, Chan W. P23 enhances the formation of aryl hydrocarbon receptor-DNA complex. Biochem Pharmacol. 2003;65:941–948.10.1016/S0006-2952(02)01650-7
- Petri S, Korner S, Kiaei M. Nrf2/ARE signaling pathway: key mediator in oxidative stress and potential therapeutic target in ALS. Neurol Res Int. 2012;2012:878030.
- Köhle C, Bock KW. Coordinate regulation of Phase I and II xenobiotic metabolisms by the Ah receptor and Nrf2. Biochem Pharmacol. 2007;73:1853–1862.10.1016/j.bcp.2007.01.009
- Ashida H, Nishiumi S, Fukuda I. An update on the dietary ligands of the AhR. Expert Opin Drug Metab Toxicol. 2008;4:1429–1447.10.1517/17425255.4.11.1429
- Marchand A, Barouki R, Garlatti M. Regulation of NAD(P)H: quinone oxidoreductase 1 gene expression by CYP1A1 activity. Mol Pharmacol. 2004;65:1029–1037.10.1124/mol.65.4.1029
- Zhang S, Qin C, Safe S. Flavonoids as aryl hydrocarbon receptor agonists/antagonists: effects of structure and cell context. Environ Health Perspect. 2003;111:1877–1882.10.1289/ehp.6322
- Xue Z, Li D, Yu W, et al. Mechanisms and therapeutic prospects of polyphenols as modulators of the aryl hydrocarbon receptor. Food Funct. 2017;8:1414–1437.10.1039/C6FO01810F
- Martin D, Rojo AI, Salinas M, et al. Regulation of heme oxygenase-1 expression through the phosphatidylinositol 3-kinase/akt pathway and the Nrf2 transcription factor in response to the antioxidant phytochemical carnosol. J Biol Chem. 2004;279:8919–8929.10.1074/jbc.M309660200
- Costa LG, Garrick JM, Roquè PJ, et al. Mechanisms of neuroprotection by quercetin: counteracting oxidative stress and more. Oxid Med Cell Longev. 2016;2016:2986796.
- Chen CC, Chen HL, Hsieh CW, et al. Upregulation of NF-E2-related factor-2-dependent glutathione by carnosol provokes a cytoprotective response and enhances cell survival. Acta Pharmacol Sin. 2011;32:62–69.10.1038/aps.2010.181
- He C, Li B, Song W, et al. Sulforaphane attenuates homocysteine-induced endoplasmic reticulum stress through Nrf-2-driven enzymes in immortalized human hepatocytes. J Agric Food Chem. 2014;62:7477–7485.10.1021/jf501944u
- Zhang T, Kimura Y, Jiang S, et al. Luteolin modulates expression of drug-metabolizing enzymes through the AhR and Nrf2 pathways in hepatic cells. Arch Biochem Biophys. 2014;557:36–46.10.1016/j.abb.2014.05.023
- Aggarwal BB, Harikumar KB. Potential therapeutic effects of curcumin, the anti-inflammatory agent, against neurodegenerative, cardiovascular, pulmonary, metabolic, autoimmune and neoplastic diseases. Int J Biochem Cell Biol. 2009;41:40–59.10.1016/j.biocel.2008.06.010
- Kunnumakkara AB, Bordoloi D, Padmavathi G, et al. Curcumin, the golden nutraceutical: multitargeting for multiple chronic diseases. Br J Pharmacol. 2017;174:1325–1348.10.1111/bph.v174.11
- Mahajanakatti AB, Murthy G, Sharma N, et al. Exploring inhibitory potential of curcumin against various cancer targets by in silico virtual screening. Interdiscip Sci. 2014;6:13–24.
- Camacho-Barquero L, Villegas I, Sánchez-Calvo JM, et al. Curcumin, a Curcuma longa constituent, acts on MAPK p38 pathway modulating COX-2 and iNOS expression in chronic experimental colitis. Int Immunopharmacol. 2007;7:333–342.10.1016/j.intimp.2006.11.006
- Nishiumi S, Yoshida K, Ashida H. Curcumin suppresses the transformation of an aryl hydrocarbon receptor through its phosphorylation. Arch Biochem Biophys. 2007;466:267–273.10.1016/j.abb.2007.08.007
- Ciolino H, Daschner P, Wang T, et al. Effect of curcumin on the aryl hydrocarbon receptor and cytochrome P450 1A1 in MCF-7 human breast carcinoma cells. Biochem Pharmacol. 1998;56:197–206.10.1016/S0006-2952(98)00143-9
- Balogun E, Hoque M, Gong P, et al. Curcumin activates the heme oxygenase-1 gene via regulation of Nrf2 and the antioxidant-responsive element. Biochem J. 2003;371:887–895.10.1042/bj20021619
- Xie Y, Zhao QY, Li HY, et al. Curcumin ameliorates cognitive deficits heavy ion irradiation-induced learning and memory deficits through enhancing of Nrf2 antioxidant signaling pathways. Pharmacol Biochem Behav. 2014;126:181–186.10.1016/j.pbb.2014.08.005
- Denison MS, Fisher JM, Whitlock JP Jr. Protein-DNA interactions at recognition sites for the dioxin-Ah receptor complex. J Biol Chem. 1989;264:16478–16482.
- Fukuda I, Nishiumi S, Yabushita Y, et al. A new southwestern chemistry-based ELISA for detection of aryl hydrocarbon receptor transformation: application to the screening of its receptor agonists and antagonists. J Immunol Methods. 2004;287:187–201.10.1016/j.jim.2004.02.003
- Donato MT, Gomezlechon MJ, Castell JV. A microassay for measuring cytochrome P450IA1 and P450IIB1 activities in intact human and rat hepatocytes cultured on 96-well plates. Anal Biochem. 1993;213:29–33.10.1006/abio.1993.1381
- Rumelhart DE, Hinton GE, Williams RJ. Learning representations by back-propagating errors. Nature 1986;323:533–536.10.1038/323533a0
- Kociba RJ, Schwetz BA. Toxicity of 2,3,7 8-tetrachlorodibenzo-p-dioxin (TCDD). Drug Metab Rev. 1982;13:387–406.10.3109/03602538209029986
- Mahon MJ, Gasiewicz TA. Ah receptor phosphorylation: localization of phosphorylation sites to the C-terminal half of the protein. Arch Biochem Biophys. 1995;318:166–174.10.1006/abbi.1995.1217
- Swanson HI. DNA binding and protein interactions of the AHR/ARNT heterodimer that facilitate gene activation. Chem Biol Interact. 2002;141:63–76.10.1016/S0009-2797(02)00066-2
- Mukai R, Shirai Y, Saito N, et al. Suppression mechanisms of flavonoids on aryl hydrocarbon receptor-mediated signal transduction. Arch Biochem Biophys. 2010;501:134–141.10.1016/j.abb.2010.05.002
- Nishiumi S, Yamamoto N, Kodoi R, et al. Antagonistic and agonistic effects of indigoids on the transformation of an aryl hydrocarbon receptor. Arch Biochem Biophys. 2008;470:187–199.
- Balogun E, Hoque M, Gong P, et al. Curcumin activates the haem oxygenase-1 gene via regulation of Nrf2 and the antioxidant-responsive element. Biochem J. 2003;371:887–895.10.1042/bj20021619
- McNally SJ, Harrison EM, Ross JA, et al. Curcumin induces heme oxygenase 1 through generation of reactive oxygen species, p38 activation and phosphatase inhibition. Int J Mol Med. 2007;19:165–172.
- Bao W, Li K, Rong S, Yao P, et al. Curcumin alleviates ethanol-induced hepatocytes oxidative damage involving heme oxygenase-1 induction. J Ethnopharmacol. 2010;128:540–553.
- Trujillo J, Chirino YI, Molina-Jijón E, et al. Renoprotective effect of the antioxidant curcumin: Recent findings. Redox Biol. 2013;1:448–456.10.1016/j.redox.2013.09.003
- Qin S. Hou DX (2016) multiple regulations of keap1/Nrf2 system by dietary phytochemicals. Mol Nutr Food Res. 2016;60:1731–1755.10.1002/mnfr.v60.8
- Yu R, Lei W, Mandlekar S, et al. Role of a mitogen-activated protein kinase pathway in the induction of phase II detoxifying enzymes by chemicals. J Biol Chem. 1999;274:27545–27552.10.1074/jbc.274.39.27545
- Yoshikawa E, Miyagi S, Dedachi K, et al. Specific interactions between aryl hydrocarbon receptor and dioxin congeners: Ab initio fragment molecular orbital calculations. J Mol Graph Model. 2010;29:197–205.10.1016/j.jmgm.2010.06.008
- Garcea G, Jones DJ, Singh R, et al. Detection of curcumin and its metabolites in hepatic tissue and portal blood of patients following oral administration. Br J Cancer. 2004;90:1011–1015.10.1038/sj.bjc.6601623
- Suresh D. Srinivasan K (2010) Tissue distribution & elimination of capsaicin, pipeline & curcumin following oral intake in rats. Indian J Med Res. 2010;131:682–691.
- Gong C, Deng S, Wu Q, et al. Improving antiangiogenesis and anti-tumor activity of curcumin by biodegradable polymeric micelles. Biomaterials 2013;34:1413–1432.10.1016/j.biomaterials.2012.10.068
- Piskorska-Pliszczynska J, Struciński P, Mikołajczyk S, et al. Dioxins and PCBs in ostrich meat and eggs – levels and implications. Food Addit Contam Part A Chem Anal Control Expo Risk Assess. 2017;34:2190–2200.