Abstract
Alcoholic beverages are enjoyed together with meals worldwide, but their excessive intake is associated with an increased risk of various diseases. We investigated whether S-allyl-L-cysteine sulfoxide (ACSO), a sulfuric odor precursor of garlic, suppresses elevation in plasma ethanol concentration by accelerating ethanol metabolism and preventing ethanol absorption from the gut in rats. ACSO and garlic extract with a high ACSO content (Garlic-H) suppressed elevation in concentrations of ethanol and acetaldehyde in plasma and promoted the activities of alcohol dehydrogenase and aldehyde dehydrogenase. However, ACSO and Garlic-H did not affect plasma acetate so much. Furthermore, we examined the change in plasma ethanol concentration by injecting ACSO or Garlic-H into the ligated stomach or jejunum together with ethanol solution. ACSO and Garlic-H suppressed the absorption of ethanol from the stomach and jejunum, but suppression in the jejunum was less than in the stomach. In conclusion, ACSO inhibits ethanol absorption and accelerates ethanol metabolism.
A garlic odor precursor suppresses blood ethanol elevation.
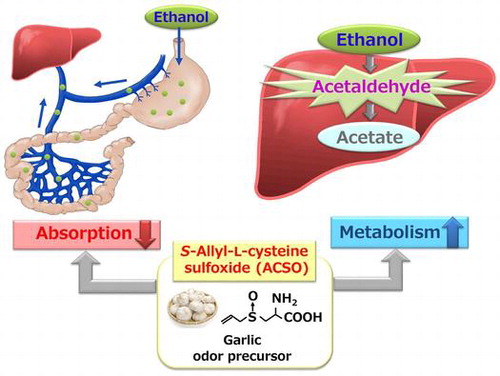
Abbreviations:
- ACSO: S-Allyl-L-cysteine sulfoxide
- ADH: Alcohol dehydrogenase
- ALD: Alcoholic liver diseases
- ALDH: Acetaldehyde dehydrogenase
- ARE: Antioxidant response element
- AUC: Areas under the time-concentration curves
- Bis-Tris: Bis(2-hydroxyethyl)amino-tris(hydroxymethyl)methane
- EDTA: Ethylenediaminetetraacetic acid
- Garlic-H: Garlic extract with a high ACSO content
- Garlic-N: Garlic extract not containing ACSO
- HPLC: High-performance liquid chromatography
- Keap1: Kelch-like ECH-associated protein
- NAD: Nicotinamide adenine dinucleotide
- NAD+: Oxidized form of NAD
- NADH: Reduced form of NAD
- NMR: Nuclear magnetic resonance
- Nrf2: Nuclear factor erythroid 2-related factor
- SD: Standard deviation
- SD rat: Sprague Dawley rat
Alcoholic beverages are commonly consumed by adults together with food worldwide, and are enjoyed because they stimulate our appetite and make meals more delicious. However, their excessive intake is associated with an increased risk of several diseases [Citation1]. Because alcohol is usually consumed with meals, understanding the effects of the diet on the pharmacokinetics of alcohol is important for reducing its harmful effect on human health. In the body, alcohol dehydrogenase (ADH) rapidly converts ethanol to its primary metabolite, acetaldehyde, which is largely responsible for mutagenic and carcinogenic. As acetaldehyde is largely responsible for alcohol-related symptoms, its rapid metabolism to acetate by acetaldehyde dehydrogenase (ALDH) is crucial to reducing the risk of health damage. In this regard, a large-scale population-based prospective cohort study demonstrated the risk of lung cancer due to alcohol intake [Citation2]. Also, although the risk of alcohol-induced gastric cancer was not certain due to the quality of the methods used, vegetable and fruit consumption was shown to be important for reducing such risk [Citation3]. In addition, Ushida et al. reported that sulforaphan derived from glucosinolate in cruciferous vegetables accelerated acetaldehyde metabolism by increasing ALDH activity, though no effect was observed on ethanol metabolism [Citation4].
Garlic (Allium sativum L.) and other alliums are distinctive because of the culinary value of their flavors and odors. In addition, garlic has anticancer, antithrombotic, antiatherosclerotic, antidiabetic and antioxidant functions, as well as immune modulation activity [Citation5–8]. It contains S-allyl-L-cysteine sulfoxide (ACSO), a garlic odor precursor, which is converted into allyl sulfenic acid, pyruvate, and ammonia by C-S lyase when its cells are damaged by cutting or crushing [Citation9]. Then, 2 molecules of allyl sulfenic acid produce diallyl thiosulfinate (allicin), which is further converted to diallyl disulfide and diallyl trisulfide. These lipophilic volatile sulfides are known to be bioactive [Citation8,10], but their strong odor limits their use in food. On the other hand, as ACSO is an odorless hydrophilic component, and has a taste-enhancing effect, it can be added to various kinds of food. If C-S lyase is inactivated by heating before cutting garlic bulbs, ACSO will be retained and odoriferous components not be produced. Although ACSO is not bioactive in vitro, it is metabolized to bioactive compounds in vivo after being absorbed from the small intestine [Citation11]. As ACSO is converted to allyl sulfenic acid, pyruvate, and ammonia by C-S lyase in vitro, pyruvate might be produced in vivo during metabolism to bioactive compounds. Pyruvate production may promote the metabolism of ethanol because supply of NAD+ is the rate-limiting factor for the metabolism of ethanol to acetaldehyde [Citation12], and NAD+ is produced from NADH when pyruvate is converted to lactate by lactate dehydrogenase.
Therefore, the present study was undertaken to investigate whether ACSO suppresses elevation in plasma ethanol concentration by accelerating ethanol metabolism. We also examined whether ACSO suppresses ethanol absorption from the gut by injecting ACSO into the ligated stomach and jejunum together with an ethanol solution and measuring plasma ethanol concentration.
Materials and methods
Materials
Ethanol (99.5%) was purchased from Kanto Chemical Co., Inc. (Tokyo, Japan). All chemicals in this study were analytical grade.
ACSO synthesis
ACSO synthesis was performed as previously described with some modifications [Citation13,14]. l-Cysteine methyl ester hydrochloride (3.0 g, 5.83 mmol) was dissolved in ethanol/H2O (2:1, v/v, 30 mL), before triethylamine (7.2 mL, 17.2 mmol) and allyl bromide (2.9 mL, 10.4 mmol) were added. After stirring for 20 min, the reaction mixture was then filtered and concentrated in vacuo to yield S-allyl-L-cysteine (white crystals). S-allyl-L-cysteine was dissolved in water and the 30% w/w hydrogen peroxide (weight of S-allyl-L-cysteine (g) × 1.26 mL) was added. After stirring overnight at room temperature, the solution was filtered in vacuo. Then, the filtrate was mixed with 1% acetate (5 mL) and 99.5% ethanol (75 mL) at 160 °C. The reaction mixture was cooled to 4 °C and stirred for 60 min and then all solvents and reagents were removed in vacuo. Without further procedures, the ACSO (white crystals) obtained should have been of sufficient purity for use in animal experiments and this was confirmed from NMR spectra recorded on an ECA500 spectrometer (JEOL, Tokyo, Japan).
Preparation of garlic extract
Garlic extract with a high ACSO content (Garlic-H) was prepared from fresh garlic incubated at 75 °C for 20 min to inactivate C-S lyase, and homogenized with 3 volumes of water. On the other hand, garlic extract not containing ACSO (Garlic-N) was prepared from fresh garlic homogenized with 3 volumes of water, and incubated at 40 °C for 60 min to allow the reaction by C-S lyase to take place. Each homogenate with and without being reacted by C-S lyase was filtered through a wire mesh strainer, and the residue was pressed on a filter cloth to obtain the remaining filtrate. Then, protease (Sumizyme MP, KYOWA CHEMICAL PRODUCTS Co., Ltd., Osaka, Japan) and cellulase (Acremo KM, KYOWA CHEMICAL PRODUCTS Co., Ltd.) were added to the gathered filtrate at an enzyme-to-filtrate weight ratio of 1:1000, and the enzymatic reaction was performed at 50 °C for 60 min. After being incubated at 75 °C for 20 min to inactivate enzymes and stored at 4 °C overnight, the supernatant was filtered through perlight (TOPCO, SHOWA CHEMICAL INDUSTRY Co., Ltd., Tokyo, Japan) and radiolite (RADIOLITE #300 and RADIOLITE FNF-A, SHOWA CHEMICAL INDUSTRY Co., Ltd.) under vacuum. The filtrate was mixed with 1/10 volume of 99.5% ethanol and the mixture was concentrated to Brix 60 under vacuum. Each concentrate (Garlic-H and Garlic-N) was stored at −80 °C until use.
Quantitative analyses of ACSO, arginine, pyruvate, and ammonia
The ACSO, arginine, and ammonia contents of each concentrate were quantified by an amino acid autoanalyser (JLC-500/V; JEOL Ltd, Tokyo, Japan). The quantitative analysis of pyruvate was performed by HPLC (Shimadzu Organic Acid Analysis System) with a conductivity detector. The components of each concentrate were separated with a Shim-pack SCR-102H ion-exclusion column (300 mm × 8.0 mm id) (Shimadzu), using a buffered solution consisting of 5 mmol/L p-toluenesulfonic acid, 20 mmol/L Bis-Tris and 0.1 mmol/L EDTA at a flow rate of 0.8 mL/min.
Animals
The animal experiments were performed in accordance with the Guidelines for Animal Experiments of the College of Bioresource Sciences, Nihon University (Approval numbers: AP15B002, AP15B043, AP15B060, and AP16B100). Seven-week-old male SD rats were purchased from Japan SLC, Inc. (Shizuoka, Japan). All rats were housed in plastic cages and fed a solid feed (CE-2) for 5–8 days prior to commencement of experiments under controlled temperature (22 ± 1 °C) and humidity (55 ± 15%) conditions, and 12-h light/dark cycle (light period 7:00–19:00). All rats were given ad libitum access to water.
Animal experimental designs
First, to measure the concentrations of ethanol, acetaldehyde, and acetate in plasma, 36 male, 7-week-old SD rats were randomly assigned to four groups (n = 9 in each group). After fasting overnight, they were orally administered 10 mL of 20% ethanol/kg body weight (Control group), garlic extract with 500 μmol ACSO in 10 mL of 20% ethanol/kg body weight (Garlic-H group), garlic extract of the same amount as Garlic-H without ACSO in 10 mL of 20% ethanol/kg body weight (Garlic-N group) or 500 μmol ACSO in 10 mL of 20% ethanol/kg body weight (ACSO group). Before and 0.5, 1, 2, 4, 6 h after administration, 70 μL of blood was collected by nicking the tail vein, and used to determine plasma ethanol, acetaldehyde, and acetate concentrations.
Second, to investigate the suppressive effect of each sample on ethanol absorption from the gut, 18 male, 7-week-old SD rats were randomly assigned to 3 groups: Control group, Garlic-H group, and ACSO group (n = 6 in each group). Stomach and small intestine ligation was performed as previously described [Citation15]. After fasting overnight, the abdominal cavity was opened, and the upper and lower regions of stomach or jejunum were ligated twice with 5–0 surgical silk. Then, 10 mL of 20% ethanol/kg body weight (Control group), garlic extract with 500 μmol ACSO in 10 mL of 20% ethanol/kg body weight (Garlic-H group), or 500 μmol ACSO in 10 mL of 20% ethanol/kg body weight (ACSO group) was injected into the ligated loop of the stomach and small intestine. Before and 5, 10, 30, and 60 min after injection, 70 μL of blood was collected from the portal vein, and used to determine plasma ethanol concentration.
Third, to investigate the activities of ADH and ALDH in the liver, 30 male, 7-week-old SD rats were randomly assigned to 3 groups: Control group, Garlic-H group, and ACSO group (n = 10 in each group). After fasting overnight, they were administered orally by gavage 10 mL of 20% ethanol/kg body weight (Control group), garlic extract with 500 μmol ACSO in 10 mL of 20% ethanol/kg body weight (Garlic-H group) or 500 μmol ACSO in 10 mL of 20% ethanol/kg body weight (ACSO group). After 60 min, rats were euthanized and their livers were harvested. The collected livers were immediately homogenized for the measurement of ADH and ALDH activities.
Determinations of ethanol, acetaldehyde, and acetate concentrations in plasma
All blood samples were collected in heparinized capillary tubes. Whole blood samples were immediately mixed with 1 mol/L perchloric acid and kept on ice. After 10 min, the tubes were centrifuged at 10,000 × g for 10 min at 4 °C, and the pH of supernatant fractions was adjusted to 7.5–8.0 by adding 0.7 mol/L K3PO4. After incubation for 10 min on ice, the deproteinized plasma was isolated by centrifugation at 2,300 × g for 10 min at 4 °C. The supernatant fractions were stored at -80 °C for analysis of acetate. Concentrations of ethanol and acetaldehyde in plasma were determined immediately using F-kit Ethanol and F-kit Acetaldehyde (Roche Diagnostics K.K, Tokyo, Japan), respectively. The quantitative analysis of acetate was conducted by the same method as that of pyruvate.
Activities of ADH and ALDH
To determine the activities of ADH and ALDH in cytosolic/microsomal and mitochondrial fractions individually, harvested livers were homogenized at 4 °C in 100 mmol/L K2HPO4-KH2PO4 buffer (pH 7.4) containing 0.25 mol/L sucrose and 0.1 mmol/L EDTA, and the homogenates were centrifuged at 600 × g for 10 min at 4 °C to remove nuclei and cell debris. The supernatant fractions were then centrifuged at 10,000 × g for 15 min, and the supernatant fractions were further centrifuged at 105,000 × g for 60 min to obtain the cytosolic/microsomal fractions as supernatants that were used for the measurement of ADH and ALDH activities. The pellets were homogenized at 4 °C in 100 mmol/L K2HPO4-KH2PO4 buffer (pH 7.4) containing 0.25 mol/L sucrose and 0.1 mmol/L EDTA and centrifuged at 10,000 × g for 15 min at 4 °C. Next, the precipitates were sonically disrupted in a sonicator at 4 °C for 2 min. The supernatant fractions were then centrifuged at 105,000 × g for 60 min to obtain the mitochondrial fractions as supernatants that were used for the measurement of ALDH activity.
ADH activity in the cytosolic/microsomal fractions and ALDH activity in the cytosolic/microsomal and mitochondrial fractions were measured fluorometrically according to the modified method of Eriksson et al. [Citation16]. For the measurement of ADH activity, the assay mixture (1.2 mL) containing NAD+ (1.6 mg) and ALDH (0.32 U) in potassium pyrophosphate buffer (pH 9.0) (F-kit Ethanol, Roche Diagnostics K.K, Tokyo, Japan) was added to a cuvette at 37 °C and incubated for 3 min. The reaction was started by the addition of 100 μL of ethanol as a substrate of ADH and the cytosolic/microsomal fractions. In this reaction, 2 mol of NADH were generated from 1 mol of ethanol because acetaldehyde produced from ethanol was further converted to acetate by ALDH added in the reaction mixture. For the measurement of ALDH activity, the assay mixture (1.2 mL) containing NAD+ (0.32 mg) in potassium pyrophosphate buffer (pH 9.0) (F-kit Acetaldehyde, Roche Diagnostics K.K, Tokyo, Japan) was added to a cuvette at 37 °C and incubated for 3 min. The reaction was started by the addition of 100 μL of acetaldehyde as a substrate of ALDH and the cytosolic/microsomal or mitochondrial fractions.
The initial velocity of NADH generation was measured (λex 340 nm) at 37 °C for 10 min in a microplate spectrometer (CARY 50 UV–vis spectrometer, VARIAN Inc., Tokyo, JAPAN). Blank reaction rates without acetaldehyde were also determined. ADH and ALDH activities were normalized to the protein concentration and expressed as change in nano moles of NADH formed per min per mg of protein.
Statistical analysis
Statistical analyses were performed using Mac Toukeikaiseki Version 2.0 software package (Esumi, Co., Ltd., Tokyo, Japan). All values are expressed as means ± SD. Differences among groups were analyzed by the Tukey-Kramer test. Values with significant differences at p < 0.05 are expressed with different lower case letters. Asterisks indicate a significant difference at p < 0.05 compared with 0 h by the Tukey-Kramer test.
The areas under the time-concentration curves (AUC) were calculated by the trapezoidal method [Citation17].
Results
Contents of ACSO, arginine, pyruvate, and ammonia in garlic extract
As shown in Table , the ACSO contents of Garlic-H and Garlic-N were 1572 mg (8.9 mmol)/100 g and N.D., respectively, indicating that ACSO in garlic extract was converted to allyl sulfenic acid by C-S lyase and further to sulfides during incubation at 40 °C for 60 min. The arginine contents of Garlic-H and Garlic-N were 1417 and 1292 mg/100 g, respectively, so there was not so much difference between them. The pyruvate contents of Garlic-H and Garlic-N were 158 mg (1.8 mmol)/100 g and 366 mg (4.2 mmol)/100 g, respectively. In addition, the ammonia contents of Garlic-H and Garlic-N were 51 mg (3.0 mmol)/100 g and 236 mg (13.9 mmol)/100 g, respectively, which were increased by incubation at 40 °C for 60 min. Although the increase in pyruvate level by incubation was much lower than ACSO level in Garlic-H, the increase in both pyruvate and ammonia indicates the production of allyl sulfenic acid by C-S lyase.
Table 1. ACSO, arginine, pyruvate and ammonia concentrations of garlic extract.
Changes in plasma concentrations of ethanol, acetaldehyde, and acetate after oral loading of ethanol
Table shows the concentration of ethanol in plasma. The plasma concentration of ethanol in all groups showed a peak at 60 min after administration and then decreased after that. The plasma ethanol concentration in the Garlic-H group from 30 to 360 min, the Garlic-N group at 30 and 270 min and the ACSO group from 180 to 360 min after oral administration was significantly lower than that in the Control group for the same times. The AUC for plasma ethanol of the Garlic-H and ACSO groups was significantly lower than that of the Control group, indicating the suppressive effect of ACSO on the increase in plasma ethanol concentration. On the other hand, the AUC for plasma ethanol of the Garlic-N group showed lower tendency than that of the Control group though there was no significant difference between them.
Table 2. Effect of garlic extract and ACSO on plasma-ethanol concentration after oral administration of ethanol.
Next, we determined the concentration of acetaldehyde in plasma (Table ). The plasma concentration of acetaldehyde in the Control and Garlic-N groups showed peaks at 120 min after ethanol administration, while that in the Garlic-H and ACSO groups showed peaks at 60 min after ethanol administration, and then decreased after that. Furthermore, the plasma acetaldehyde concentration in the Garlic-H group at 120 and 360 min, in the ACSO group at 120, 180, and 360 min, and in the Garlic-N group at 120 min was significantly lower than that in the Control group at the same times after ethanol administration. Although the AUC for plasma acetaldehyde of the Garlic-H, Garlic-N and ACSO groups was significantly lower than that of the Control group, there were certain differences among them and the value was greater in descending order for the Garlic-H, ACSO, and Garlic-N groups.
Table 3. Effect of garlic extract and ACSO on plasma-acetaldehyde concentration after oral administration of ethanol.
As acetaldehyde is further metabolized to nontoxic acetate by ALDH, we next measured the concentration of acetate in plasma (Table ). The plasma concentration of acetate in all groups was significantly increased at 30 min, maintained at an almost constant level up to 180 min and then was decreased at 360 min. The rate of decrease after 180 min was greater in the Garlic-H and ACSO groups than in the Garlic-N and Control groups. However, for all groups, there was no significant difference at each time point or in the AUC of plasma acetate.
Table 4. Effect of garlic extract and ACSO on plasma-acetate concentration after oral administration of ethanol.
Changes in plasma ethanol concentration after injection of ethanol into gut
To examine the reason for the suppression of elevation in ethanol concentration by Garlic-H and ACSO, their effect on ethanol absorption from the gut was measured.
In the stomach, the plasma concentration of ethanol in all groups was significantly increased at 5 min and was maintained until 60 min (Table ). The elevation in plasma ethanol concentration was significantly suppressed in the Garlic-H and ACSO groups, Garlic-H having a greater effect than ACSO.
Table 5. Effects of garlic extract and ACSO on plasma ethanol concentration after injection of ethanol into stomach.
In the jejunum, the plasma concentration of ethanol showed a peak 5 min after its injection into the ligated loop, and then decreased (Table ). ACSO significantly suppressed the increase in the plasma ethanol concentration from 10 to 60 min, while Garlic-H suppressed it at 5, 30 and 60 min after injection.
Table 6. Effects of garlic extract and ACSO on plasma ethanol concentration after injection of ethanol into the small intestine.
Activities of ADH and ALDH in liver
To examine the reason for suppression of ethanol elevation with Garlic-H and ACSO, the activities of ADH and ALDH, enzymes that primarily metabolize ethanol, were measured.
In the hepatic cytosolic fractions, ADH activity was significantly higher in the Garlic-H and ACSO groups than in the Control group (Figure ). Regarding ALDH activity, while no significant difference was observed for cytosolic fractions, activity in mitochondrial fractions was almost double in the Garlic-H and ACSO groups as compared with the Control group (Figure ).
Discussion
Moderate alcohol intake may have a favorable effect on our appetite, but excessive intake can cause adverse sensations such as nausea and dizziness. Asian people are prone to acute alcohol intoxication because about 50% of them do not have ALDH2 [Citation18], one of the key enzymes for metabolizing acetaldehyde to acetate. About 10–30% of ethanol is absorbed from the stomach and the rest is mainly from the jejunum. Although the amount of ethanol absorbed from the stomach is higher when it is administered with food than with water, the increase in blood ethanol concentration is much suppressed with food [Citation19]. As alcohol is usually consumed with meals, their components may affect its absorption and metabolism. Therefore, it would be significant to determine food components that have novel therapeutic effects regarding the prevention and treatment of alcohol-related disease. In the present study, we showed, for the first time, that a sulfuric odor precursor in garlic had the property of suppressing elevation in plasma ethanol concentration by accelerating ethanol metabolism and preventing ethanol absorption from the gut in rats.
As supply of NAD+ is the rate-limiting factor for the metabolism of ethanol to acetaldehyde by ADH [Citation12], and NAD+ is produced from NADH during the conversion of pyruvate to lactate by lactate dehydrogenase, ethanol metabolism may be accelerated by the administration of pyruvate. However, there has been no evidence on the promotion of ethanol metabolism by supplementation of pyruvate though the increase in NADH by ethanol metabolism promoted the conversion of pyruvate to lactate [Citation20]. As ACSO was converted to some active components after absorption from the small intestine [Citation11], pyruvate would have been produced during the conversion of ACSO to active components. Therefore, we hypothesized that oral administration of ACSO may enhance ethanol metabolism. However, contrary to this assumption, Kishimoto et al. reported that, after intragastrical administration of ethanol, fresh garlic juice suppressed the ethanol-oxidizing system as well as elevation of acetaldehyde and acetate concentrations in serum in spite of having no effect on ethanol concentration [Citation21]. As the juice was prepared by grating garlic bulbs, ACSO would have been converted to sulfides, but a certain amount of pyruvate might have remained. However, no information was provided on the components of the garlic juice, which made it difficult to specify the factors behind the suppression of the ethanol-oxidizing system. On the other hand, our study showed that garlic extracts and synthesized ACSO suppressed elevation of ethanol concentration in plasma.
Since we measured the ACSO, arginine, pyruvate, and ammonia contents of the extracts, this can be discussed in quantitative terms. In our study, 500 μmol ACSO in 10 mL of 20% ethanol/kg body weight was administered to the rats. Therefore, if all of the ACSO had been absorbed from the small intestine and converted to pyruvate, up to 500 μmol pyruvate/kg body weight would have been produced in the blood. As shown in Supplemental Table 1, oral administration of 500 μmol pyruvate in 10 mL of 20% ethanol/kg body weight was not sufficient to suppress elevation in ethanol concentration. Therefore, pyruvate production could not be the factor responsible for suppressing elevation of ethanol concentration in plasma.
Two mechanisms can be considered for the suppressive effect of ACSO on elevation of ethanol concentration in plasma: (1) suppression of ethanol absorption from the gut, (2) promotion of ethanol metabolism in the liver. We carried out experiments with the aim of determining which of these mechanisms is responsible for this effect of ACSO.
When garlic extract containing ACSO or synthesized ACSO was injected into the ligated loop of the stomach or small intestine together with ethanol solution, the suppressive effect of ACSO was observed from 5 min after injection, being greater in the Garlic-H group than in the ACSO group and more distinct in the stomach than in the small intestine. As our previous findings have shown that ACSO is stable in the gut, and converted into some active compounds after the absorption from the small intestine [Citation11], ACSO itself would have a function to suppress ethanol absorption from the stomach. However, as garlic extract was even more effective than ACSO, it could contain some other active components besides ACSO. As no transporters are used in the absorption of ethanol in the gut, the suppressive effect of ACSO on ethanol absorption might be attributable to retardation of peristaltic movement. Further study is necessary to confirm this assumption.
If suppression of ethanol absorption from the gut is the only factor behind the effect of ACSO, the shape of the curve of acetaldehyde and acetate concentrations would be similar to that of the ethanol concentration, showing a peak at 60 min after ethanol administration. However, there was no clear peak in the acetaldehyde and acetate concentrations. The acetaldehyde concentration in the Garlic-H, Garlic-N, and ACSO groups started to decrease significantly as compared with the Control group from 120 min after ethanol administration, while the acetate concentration in these groups was almost the same as that of the Control group up to 180 min, and that in the Garlic-H and ACSO groups was decreased at 360 min. These results indicate acceleration of ethanol metabolism in the liver and therefore, ADH and ALDH activities were measured.
After ethanol is metabolized to acetaldehyde by ADH, acetaldehyde is metabolized to acetate by two major isoforms of ALDH, designated as cytosolic ALDH1 and mitochondrial ALDH2. A catalytic deficiency of the ALDH2 isozyme often causes flushing and other vasomotor symptoms after alcohol intake due to high acetaldehyde concentration. As about half of Orientals do not possess ALDH2 [Citation17], they are more susceptible to such effects of ethanol than Caucasians. In our study, the activities of ADH and mitochondrial ALDH were significantly increased in the Garlic-H and ACSO groups as compared with the Control group, though no significant difference was observed for cytosolic ALDH (Figures and ). These findings strongly suggest that increase in the activity of ALDH2 in mitochondria would be principally responsible for the fast metabolism of acetaldehyde in the ACSO and Garlic-H groups.
Nuclear factor erythroid 2-related factor (Nrf2) is a transcription factor that up-regulates a diverse array of antioxidant genes. In this regard, Ushida et al. reported that ALDH expression was under the major control of Nrf2, and ALDH activity was increased by the administration of sulforaphan and the blood acetaldehyde concentration was decreased [Citation4]. It is therefore very likely that ACSO affects the Keap1/Nrf2/ARE pathway and accelerates acetaldehyde metabolism by inducing ALDH2 activity. However, the reason for the increase in ADH and mitochondrial ALDH activities without affecting cytosolic ALDH activity by ACSO will be examined in further studies.
There are several limitations in the present study. First, although ACSO is metabolized to bioactive compounds in vivo after the absorption from the small intestine [Citation11], we did not directly examine whether ACSO itself or its metabolites affected ethanol metabolism and Nrf2 pathway in the liver. Further studies are necessary to identify the active compounds and specify their mechanism of action. Second, the single oral administration of ACSO and ethanol would represent an acute effect which may differ from a chronic effect. Chronic alcohol consumption is a leading cause of alcoholic liver diseases (ALD), including hepatic steatosis, steatohepatitis, fibrosis, and cirrhosis [Citation22]. Typically, ALD pathogenesis is correlated with increases in oxidative stress, activity of the innate immune system, and concentrations of pro-inflammatory cytokines and gut-derived lipopolysaccharides [Citation23–25]. Therefore, further studies should be carried out to examine the preventive effects against ALD of ACSO and Garlic-H.
In conclusion, S-allyl-L-cysteine sulfoxide, a garlic odor precursor, suppressed elevation in blood ethanol concentration by preventing ethanol absorption from the gut and accelerating ethanol metabolism in the liver.
Author contributions
All the authors designed the research plan after conducting an appropriate discussion; H. U-K. and A. H. mainly performed the ACSO synthesis and animal experiments. T. S. prepared the garlic extract and determined its component contents and plasma acetate concentration. A. H. and A. S. mainly performed the quantitative analyses. H. U-K. and H.K wrote the manuscript with the assistance of Y. Y., M. A., and T. S. H. K. supervised the research.
Disclosure statement
No potential conflict of interest was reported by the authors.
Supplemental data
Supplemental data for this article can be accessed https://doi.org/10.1080/09168451.2018.1447357
BBB-170646_Final-Supplemental_Table.pdf
Download PDF (34.1 KB)References
- Roerecke M, Rehm J. Alcohol intake revisited: risks and benefits. Curr Atheroscler Rep. 2012;14(6):556–562.10.1007/s11883-012-0277-5
- Shimazu T, Inoue M, Sasazuki S, et al. Alcohol and risk of lung cancer among Japanese men: data from a large-scale population-based cohort study, the JPHC study. Cancer Causes Control. 2008;19(10):1095–1102.10.1007/s10552-008-9173-2
- Shimazu T, Tsuji I, Inoue M, et al. Alcohol drinking and gastric cancer risk: an evaluation based on a systematic review of epidemiologic evidence among the Japanese population. Jpn J Clin Oncol. 2008;38(1):8–25.10.1093/jjco/hym152
- Ushida Y, Talalay P. Sulforaphane accelerates acetaldehyde metabolism by inducing aldehyde dehydrogenases: relevance to ethaenol intolerance. Alcohol Alcohol. 2013;48(5):526–534.10.1093/alcalc/agt063
- Peleg A, Hershcovici T, Lipa R, et al. Effect of garlic on lipid profile and psychopathologic parameters in people with mild to moderate hypercholesterolemia. Isr Med Assoc J. 2003;5(9):637–640.
- Lamm DL, Riggs DR. enhanced immunocompetence by garlic: role in bladder cancer and other malignancies. J Nutr. 2001;131(3):1067S–1070S.10.1093/jn/131.3.1067S
- Fleischauer AT, Arab L. Garlic and cancer: a critical review of the epidemiologic literature. J Nutr. 2001;131(3):1032S–1040S.10.1093/jn/131.3.1032S
- Thomson M, Ali M. Garlic [Allium sativum]: a review of its potential use as an anti-cancer agent. Curr Cancer Drug Targets. 2003;3(1):67–81.10.2174/1568009033333736
- Block E. The chemistry of garlic and onions. Sci Am. 1985;252(3):114–121.10.1038/scientificamerican0385-114
- Tsuchiya H, Nagayama M. Garlic allyl derivatives interact with membrane lipids to modify the membrane fluidity. J Biomed Sci. 2008;15(5):653–660.10.1007/s11373-008-9257-8
- Akao M, Shibuya T, Shimada S, et al. In vivo production of bioactive compounds from S-allyl-L-cysteine sulfoxide, garlic odor precursor, that inhibit platelet aggregation. J Clin Biochem Nutr. 2008;43(Suppl. 1):502–504.
- Alexandrovich YG, Kosenko EA, Sinauridze EI, et al. Rapid elimination of blood alcohol using erythrocytes: mathematical modeling in in vitro study. BioMed Res Int. 2017;2017:14. DOI:10.1155/2017/5849593.
- Iberl B, Winkler G, Muller B, et al. Quantitative determination of allicin and alliin from garlic by HPLC*. Planta Med. 1990;56(3):320–326.10.1055/s-2006-960969
- Hakamata W, Koyama R, Tanida M, et al. A simple synthesis of alliin and allo-alliin: x-ray diffraction analysis and determination of their absolute configurations. J Agric Food Chem. 2015;63(50):10778–10784.10.1021/acs.jafc.5b05501
- Kumagai H, Koizumi A, Suda A, et al. Enhanced calcium absorption in the small intestine by a phytate-removed deamidated soybean globulin preparation. Biosci Biotechnol Biochem. 2004;68(7):1598–1600.10.1271/bbb.68.1598
- Eriksson CJ, Marselos M, Koivula T. Role of cytosolic rat liver aldehyde dehydrogenase in the oxidation of acetaldehyde during ethanol metabolism in vivo. Biochem J. 1975;152(3):709–712.10.1042/bj1520709
- Matthews JN, Altman DG, Campbell MJ, et al. Analysis of serial measurements in medical research. BMJ. 1990;300:230–235.10.1136/bmj.300.6719.230
- Impraim C, Wang G, Yoshida A. Structural mutation in a major human aldehyde dehydrogenase gene results in loss of enzyme activity. Am J Hum Genet. 1982;34(6):837–841.
- Levitt MD, Li R, DeMaster EG, et al. Use of measurements of ethanol absorption from stomach and intestine to assess human ethanol metabolism. Am J Physiol. 1997;273:G951–957.
- Zakhari S, Li TK. Determinants of alcohol use and abuse: Impact of quantity and frequency patterns on liver disease. Hepatology. 2007;46(6):2032–2039.10.1002/hep.v46:6
- Kishimoto R, Ueda M, Yoshinaga H, et al. Combined effects of ethanol and garlic on hepatic ethanol metabolism in mice. J Nutr Sci Vitaminol. 1999;45:275–286.10.3177/jnsv.45.275
- Altamirano J, Bataller R. Alcoholic liver disease: pathogenesis and new targets for therapy. Nat Rev Gastroenterol Hepatol. 2011;8(9):491–501.10.1038/nrgastro.2011.134
- Purohit V, Bode JC, Bode C, et al. Alcohol, intestinal bacterial growth, intestinal permeability to endotoxin, and medical consequences: summary of a symposium. Alcohol. 2008;42(5):349–361.10.1016/j.alcohol.2008.03.131
- Stewart S, Jones D, Day CP. Alcoholic liver disease: new insights into mechanisms and preventative strategies. Trends Mol Med. 2001;7(9):408–413.10.1016/S1471-4914(01)02096-2
- Miller AM, Horiguchi N, Jeong WI, et al. Molecular mechanisms of alcoholic liver disease: innate immunity and cytokines. Alcohol Clin Exp Res. 2011;35(5):787–793.10.1111/acer.2011.35.issue-5