ABSTRACT
Plants flower under appropriate day-length conditions by integrating temporal information provided by the circadian clock with light and dark information from the environment. A sub-group of plant specific circadian clock-associated PSEUDO-RESPONSE REGULATOR (PRR) genes (PRR7/PRR3 sub-group) controls flowering time both in long-day and short-day plants; however, flowering control by the other two PRR gene sub-groups has been reported only in Arabidopsis thaliana (Arabidopsis), a model long-day plant. Here, we show that an Arabidopsis PRR9/PRR5 sub-group gene can control flowering time (heading date) in rice, a short-day plant. Although PRR5 promotes flowering in Arabidopsis, transgenic rice overexpressing Arabidopsis PRR5 caused late flowering. Such transgenic rice plants produced significantly higher biomass, but not grain yield, due to the late flowering. Concomitantly, expression of Hd3a, a rice florigen gene, was reduced in the transgenic rice.
Abbreviations
CCT: CONSTANS, CONSTANS-LIKE, and TOC1; HD: HEADING DATE; LHY: LATE ELONGATED HYPOCOTYL; Ppd: photoperiod; PR: pseudo-receiver; PRR: PSEUDO-RESPONSE REGULATOR; TOC1: TIMING OF CAB EXPRESSION 1; ZTL: ZEITLUPE
GRAPHICAL ABSTRACT
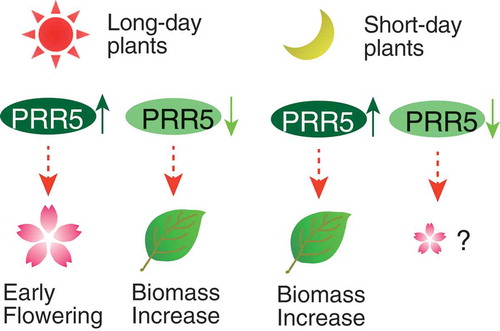
Model for the effect of the PRR9/PRR5 sub-group on flowering time and plant biomass.
Plant photosynthetic activity determines biomass. Elevated CO2 increases both photosynthetic activity and biomass in many plants, but there are also plants whose growth and yield are not enhanced by high CO2 [Citation1,Citation2]. Correlations between photosynthetic activity and yield in rice mutants support the idea that photosynthetic activity is a target for improving yield [Citation3]; however, achieving increased biomass by genetically modifying photosynthetic activity has often been challenging [Citation4]. Instead, genetic modifications to improve photosynthetic capacity, such as opening stomata under sufficient light conditions [Citation5] and creating a chloroplastic photorespiratory bypass [Citation6], result in increased biomass.
Increasing net photosynthesis by expanding the total area of leaves, a process that is developmentally controlled, is another way to improve biomass. Developmental perturbations such as late flowering in rice results in biomass gains [Citation7]. Among the high yielding cultivars of barley and sorghum, many of them show late flowering phenotypes [Citation8,Citation9]. Late flowering in Arabidopsis also enhances biomass [Citation10,Citation11]. Flowering time is controlled by a florigen that is encoded by FT in Arabidopsis [Citation12,Citation13]. FT expression in Arabidopsis is induced under a long-day photoperiod [Citation12,Citation13]. To flower at appropriate day-lengths, plants integrate the temporal information provided by the circadian clock with light and dark information provided by the environment [Citation14,Citation15]. Therefore, control of flowering and biomass can be achieved by modulating the circadian clock.
We previously reported that attenuation of redundant clock-associated Arabidopsis PSEUDO-RESPONSE REGULATOR (PRR) genes, including TIMING OF CAB EXPRESSION 1 (TOC1 also called PRR1), results in late flowering and increases biomass [Citation10]. The PRR genes were named since they encode proteins similar to “response regulator proteins” that play output roles in phosphorelay signal transduction in bacteria, yeast, and plants [Citation16]. Authentic response regulators in plants are crucial for cytokinin signal transduction in which cytokinin receptors (histidine kinases) transmit a phosphoryl group to response regulators via Hpt transmitter proteins in response to cytokinin [Citation17]. PRR proteins have an N-terminal pseudo-receiver (PR) domain. The PR domain lacks the key Asp residue that accepts a phosphoryl group from sensory kinases, suggesting that PRR proteins are not involved in cytokinin signal transduction. The PR domain functions in protein-protein interactions. PR domains of TOC1 and PRR5 are recognized by ZEITLUPE (ZTL), a blue-light sensory F-box protein in ubiquitin E3 ligase complexes that function in targeted protein degradation [Citation18,Citation19]. TOC1 and PRR5 are phosphorylated by Casein kinase 1 family prior targeted degradation by ZTL [Citation20–Citation22]. TOC1 forms heterodimers with PRR5 and PRR3 at PR domains that influence the stability and localization of TOC1 [Citation23]. PRR has C-terminal CONSTANS, CONSTANS-LIKE, and TOC1 (CCT) domains. CCT domains are crucial for protein-protein interaction and protein-DNA interaction [Citation24,Citation25]. Arabidopsis PRR9, PRR7, and PRR5 have a transcription repression motif between the PR and CCT domains [Citation26,Citation27]. Arabidopsis PRR5 is a transcriptional repressor, but if VP16, a strong transcriptional activator domain, is tandemly fused to PRR5 (PRR5-VP), then PRR5-VP acts as transcriptional activator. Transgenic Arabidopsis over-expressing PRR5-VP have late flowering and long hypocotyl phenotypes that are opposite to those of PRR5- overexpressing Arabidopsis [Citation10,Citation25]. Phylogenic analysis demonstrated that PRR proteins from flowering plants are classified into three sub-groups (the PRR9/PRR5 group, the PRR7/PRR3 group, and the TOC1 group) and suggested that these three groups had already separated before the divergence of monocots and eudicots [Citation28,Citation29].
The PRR genes from diverse flowering plants have been reported to be involved in flowering time control through the control of FT expression; however, the direction of flowering time regulation by individual PRR differs [Citation30] (). In the TOC1 sub-group, only Arabidopsis TOC1 has been reported as a modulator of flowering time. Two toc1 mutants show early flowering phenotype [Citation14], whereas toc1-2 mutation enhances late flowering of prr5 [Citation31]. These findings suggest a complex molecular function of Arabidopsis TOC1 in flowering time regulation.
Figure 1. Phylogenic tree of PRR proteins and effect on flowering time in annual plants. Phylogenic tree was inferred by the neighbor-joining method. Protein sequences of pseudo-receiver (PR) domains of Arabidopsis PRR (AtPRR or AtTOC1), rice PRR (OsPRR), a barley PRR (Ppd-H1), a wheat PRR (Ppd-D1a), and a sorghum PRR (SbPRR37, Ma1) were used to construct the phylogenic tree. PF is a photoperiod condition for flowering. Sun and moon symbols indicate either a long-day plant or a short-day plant from which the corresponding PRR was isolated, respectively. E.PRR is the effect of the corresponding PRR on flowering time. Leaf and flower symbols indicate suppression and induction effects on flowering, respectively. For example, OsPRR37 (Hd2) is a flowering suppressor of a short-day plant. Note that AtPRR3 changes a conserved amino acid in the CCT domain, and the effect of AtTOC1 on flowering time is complicated. See details in the text.
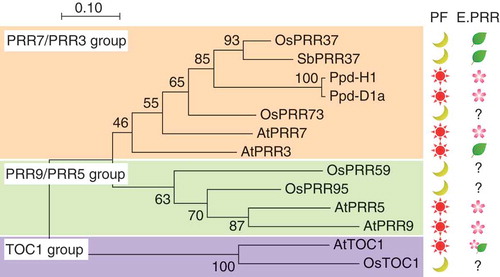
In long-day plants, proteins in the PRR7/PRR3 subgroup, except for Arabidopsis PRR3, promote flowering [Citation8,Citation30,Citation32,Citation33] (). Flowering time of Arabidopsis prr7 mutants and PRR7 overexpression lines indicate that PRR7 promotes flowering [Citation33,Citation34]. Polymorphisms were found in a PRR7/PRR3 sub-group gene, Photoperiod-H1 (Ppd-H1), that controls flowering time in spring-sown barley, a long-day plant [Citation8]. Barley carrying ppd-H1 alleles had lower expression levels of HvFT2, a homolog of FT, and flowered late [Citation8]. The causal gene for early flowering of wheat cultivars carrying a semi-dominant Photoperiod-D1a (Ppd-D1a) allele was identified as a PRR7/PRR3 sub-group gene [Citation32]. The early flowering Ppd-D1a allele has a deletion in the upstream region of the PRR gene that upregulates the PRR expression [Citation32]. Overexpression of Arabidopsis PRR3 resulted in late flowering [Citation35]. The highly conserved Arg residue in the CCT domain is changed to His in Arabidopsis PRR3, and this variant weakens the DNA-binding activity of PRR3 both in vitro and in vivo [Citation24,Citation25]. In short-day plants, the PRR7/PRR3 genes delay flowering time [Citation9,Citation36,Citation37]. To identify the genetic basis of flowering time in rice, a short-day plant, a quantitative trait loci (QTL) analysis for heading date (hd) was conducted using a Japonica rice “Nipponbare” and an Indica rice “Kasalath” as parents [Citation38,Citation39]. The Hd2 locus contains an OsPRR37 gene, and a nonsense mutation of OsPRR37 was found in a CCT domain in the Kasalath cultivar, which has a nonfunctional Hd2, suggesting that OsPRR37 is an Hd2 candidate [Citation29]. Furthermore, a QTL analysis using Milyang23 and H143 indicated that OsPRR37 is the locus for Hd2 [Citation36]. In sorghum (Sorghum bicolor (L.) Monench), another short-day plant, SbPRR37 is proposed to inhibit flowering during long days [Citation9]. Therefore, the available evidence suggests that the PRR7/PRR3 sub-group in short-day plants is involved in flowering time regulation. The evidence also tends to suggest that the PRR7/PRR3 group in long-day plants promotes flowering and delays flowering in short-day plants ().
In the PRR9/PRR5 sub-group, only Arabidopsis PRR proteins are known as promoters of flowering [Citation40] (). The PRR5 protein acts as a transcriptional repressor in the clock [Citation26] and directly represses the expression of clock-output genes including CYCLING DOF FACTOR (CDF) genes that encode key transcription factors that repress CONSTANS (CO) and FT to regulate flowering time [Citation25,Citation41,Citation42]. Flowering is inhibited by an artificial PRR5-VP fusion protein, which acts as transcriptional activator for PRR5-target genes [Citation25]. Arabidopsis PRRs also directly increase the stability of CO, a transcription factor that induces the expression of FT [Citation43]. In at least two pathways, the Arabidopsis PRR induces FT and promotes flowering. There is currently no evidence to support whether PRR9/PRR5 sub-group proteins in short-day plants are involved in flowering time ().
To examine the involvement of PRR9/PRR5 sub-group proteins in the flowering time of short-day plants as a model study, we analyzed a rice overexpressing the Arabidopsis PRR5 gene since PRR5 and PRR5-VP were characterized as flowering promoter and repressor, respectively [Citation25]. We found that both the vegetative growth period and the total biomass increased in the PRR5 overexpression lines. The study not only provides a technique for increasing biomass by artificially overexpressing a gene from the PRR9/PRR5 sub-group, but also suggests the reversibility of the same PRR9/PRR5 gene (Arabidopsis PRR5) in short-day and long-day plants for flowering time regulation.
Materials and methods
Analysis of PRR/TOC1 sequences
Protein sequences of PR domains of the PRR/TOC1 families in Arabidopsis and rice, and that of barley Ppd-H1 were used as previously reported [Citation28]. The PR domains of sorghum SbPRR37 (Ma1, AGN92436.1) and wheat Ppd-D1a (BAM31259.1) archived at NCBI (https://www.ncbi.nlm.nih.gov/search/) were searched as previously reported [Citation28]. The evolutionary history was inferred using the neighbor-joining method [Citation44]. The percentage of replicate trees in which the associated taxa clustered together in the bootstrap test (500 replicates) are shown next to the branches [Citation45]. The tree was drawn to scale with branch lengths in the same units as those of the evolutionary distances used to infer the phylogenetic tree. The evolutionary distances were computed using the Poisson correction method [Citation46] and are reported as the number of amino acid substitutions per site. Evolutionary analysis for PR domains were performed by MEGA7 [Citation47].
Plant materials and growth conditions
The cultivar “Nipponbare” was used in this study. DNA fragments of PRR5-VP and PRR5-FLAG were cloned into pActnos/Hmz [Citation48] between the Xba I and Sma I sites using an In-fusion Cloning Kit (Takara) and the appropriate primer sets (for PRR5-VP, 5′-AGAAGTCGACTCTAGATGACTAGTAGCGAGGAAGTAG-3′ and 5′-TCGAGCTCGGTACCCTTACTTGTCGTCATCGTCTTTG-3′, for PRR5-FLAG, 5′-AGAAGTCGACTCTAGATGACTAGTAGCGAGGAAGTAG-3′ and 5′-TCGAGCTCGGTACCCTTACGGGAATTCCCCACC-3′), generating pACT-PRR5-VP and pACT-PRR5-FLAG. DNA templates for PCR amplification were plasmids harboring PRR5-VP and PRR5-FLAG [Citation25]. These binary vectors were introduced into the rice genome via an Agrobacterium-mediated method [Citation49]. The empty vector containing pActnos/Hmz served as a control (VC). We generated five VC lines (#1 to #5), and 15 lines for pACT-PRR5-VP (#1 to #15) and pACT-PRR5-FLAG (#1 to #15), all of these were independent transgenic lines. Shoot lengths on or around the heading date for the T0 generation were measured ( and ).
Figure 2. Transgenic rice expressing PRR5-VP. (a) Accumulation of PRR5-VP protein in T0 transgenic rice. The numbers indicate independent transgenic rice lines. Two acrylamide gels were used (left and right of the red line), but immunoblotting was performed in a same experiment. Original gel images are shown in Supplemental Figure 1. (b) Photograph of PRR5-VP rice. Rice plants regenerated from callus on the same day are shown. (c) Relationship between plant height and relative PRR5-VP protein amount. Blue and green dots are vector and PRR5-VP plants, respectively.
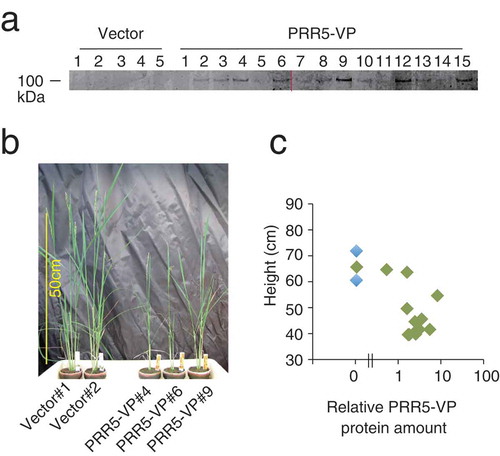
Figure 3. Transgenic rice expressing PRR5-FLAG. (a) Accumulation of PRR5-FLAG protein in T0 transgenic rice. The numbers indicate independent transgenic rice lines. Two acrylamide gels were used (left and right of the red line), but immunoblotting was performed on a same experiment. Original gel images are shown in Supplemental Figure 2. (b) Photograph of PRR5-FLAG rice. Rice plants regenerated from callus on the same day are shown. (c) Relationship between plant height and relative PRR5-FLAG protein amount. Blue and red dots are vector and PRR5-FLAG plants, respectively.
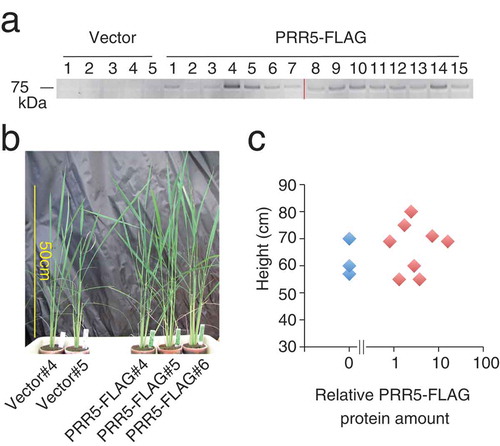
Immunoblotting
Two hundred mg of one leaf from each transgenic rice line from the T0 generation was sampled at 10 h after illumination and was frozen in a 2-mL test tube using liquid nitrogen. Frozen samples were crushed with zirconia beads (Tomy ZB-50) in a Tissue Lyser II (Qiagen). Similar amounts of proteins in samples were used. Immunoblotting analysis was performed using anti-FLAG antibody (F3165; Sigma-Aldrich) and anti-VP16 antibody (ab4808; Abcam) as described previously [Citation26]. PRR5-VP and PRR5-FLAG protein amounts were measured by Image J.
Flowering time measurement
The date when heading started was recorded. Rice plants from the T1 generation were grown on a synthetic soil (Mitsui-Toatsu No 3) in a greenhouse with irrigation and supplemental artificial light at 26°C/23 °C as described previously [Citation49]. Only rice plants having a transgene were analyzed.
Measurement of biomass
Rice plants from the T3 generation were grown as described previously [Citation49], for biomass measurement. Briefly, the plants were transplanted with approximately 1 g of slow-release fertilizer per plant and grown in a greenhouse with irrigation and supplemental artificial light at 26°C/23°C. Plants were harvested at the late grain-filling stage, dried for two weeks in a greenhouse, and the dry-weight for total leaves and stems, roots, and total panicles were measured. Four plants from each line were analyzed.
Gene expression analysis
Rice plants were grown on a commercial soil (Mikawa-Baido No 1, AICHI MEDERU) in a growth chamber (Nippon Medical and Chemical Instruments) at 12 h light 26°C/12 h dark 23°C. Eight-day-old rice plants were frozen in liquid nitrogen at 3 h intervals. Three biological replicates were used for each time point. RNA extraction, reverse transcription, and quantitative PCR were performed as previously described [Citation26]. Expression data were normalized to the expression of OsGPC1 [Citation49]. Primers used for RT-qPCR were as previously described for Hd3a [Citation50] and OsGPC1 [Citation49]; primers 5′- CAGCAGATGTTCAGCGAGC-3′ and 5′- CTTTAACTCAGTGTGGAGCG-3′ were used for OsLHY.
Results
PRR5-VP decreases rice biomass
We aimed to control rice flowering time and biomass by transforming plants with a single Arabidopsis PRR construct from the PRR9/PRR5 sub-group. In our previous study, overexpressing PRR5-VP in Arabidopsis resulted in late flowering and increased biomass. To test whether the introduction of PRR5-VP to rice would alter flowering time (heading date), rice was transformed with an ACTpro:PRR5-VP construct. Presumably, PRR5-VP was overexpressed in rice (PRR5-VP) due to control by an ACTIN gene promoter [Citation48,Citation49]. Cells resistant to the antibiotic generated shoots from callus, and shoots subsequently formed rice plants. PRR5-VP protein was detected in 15 T0 generation transgenic lines by immunoblotting ()). The growth of T0 plants that contained an immunologically cross-reactive protein band corresponding to the estimated molecular weight of PRR5-VP was analyzed under 14 h light/10 h dark conditions. Photographs of transgenic rice at eight weeks after shoot regeneration from callus suggested that flowering times (heading date) of PRR5-VP transgenic lines (#4, #6, and #9) were similar to those of the vector controls (#1 and #2) ()). Despite having similar flowering times, PRR5-VP transgenic rice was shorter with fewer leaves (,c)), implying that PRR5-VP rice affects biomass in ways other than by flowering time regulation.
PRR5-FLAG increases rice biomass
To address whether overexpression of PRR5 affects flowering time and biomass in rice, we introduced an ACTpro:PPR5-FLAG construct into rice (PRR5-FLAG). PRR5-FLAG accumulation in T0 plants was analyzed ()). The photograph taken seven weeks after shoot formation from calli showed that PRR5-FLAG and control plants were similar in height, but PRR5-FLAG seemed to have gained more biomass than the control (,c)).
To analyze flowering time of PRR5-FLAG transgenic rice, T1 generation rice were grown under greenhouse conditions [Citation49]. Days-to-heading was used as a measure of flowering time and was determined in rice expressing the Arabidopsis transgene (). Heading of non-transgenic rice started at about 70 days after germination. Heading of both vector controls (#1 and #4) also occurred at about 70 days after germination. PRR5-FLAG #4 started to head at about 81 days after germination. We could analyze the flowering time of only one plant of the PRR5-FLAG #6 line and found that the line flowered late (76 days). PRR5-VP #4 line had a similar flowering time (about 69 days) to that of the non-transgenic lines. Collectively, the results suggested that overexpression of PRR5-FLAG in rice caused late flowering.
Table 1. Heading date of T1 transgenic rice. Days-to-heading after germination was measured (mean ± S.E.). Only plants having a transgene were analyzed for Vector, PRR5-FLAG, and PRR5-VP rice.
To analyze biomass more precisely, we obtained the T3 generation of two independent lines of PRR5-FLAG and analyzed these plants (). As expected, the total biomass of PRR5-FLAG lines was greater than that of the control plants (Student’s t-test p < 0.05, )). The dry weights of vegetative tissues (leaves and stems) of PRR5-FLAG plants were significantly higher compared to those of the control (p < 0.05, )). The dry weights of roots from a PRR5-FLAG line were greater than the control (p < 0.05, )). On the other hand, the weight of total panicles in the PRR5-FLAG lines and the control were similar (p > 0.05, )). We also found that the rate of grain filling for PRR5-FLAG was significantly higher than that of the control (p < 0.01, )). In summary, the introduction of PRR5-FLAG to rice caused late flowering that eventually increased biomass.
Figure 4. Biomass of PRR5-FLAG-expressing rice. (a) Photograph of T3 plants at about 100 days after germination. Red bars indicate 20 cm (left scale). Dry weight of (b) total plant tissues, (c) vegetative tissues (leaves and stems), (d) roots, and (e) grains. (f) Grain filling rate. Error bars indicate the SE of four biological replicates, and asterisks indicate statistical differences compared with the control line (Student t test; P < 0.05) for (b) to (f).
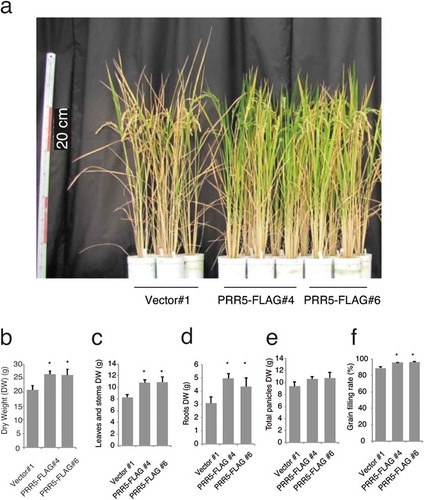
Expression of Hd3a in PRR5-FLAG rice
To understand the possible molecular mechanism responsible for the late flowering of PRR5-FLAG, we analyzed the expression of Hd3a, a florigen gene in rice [Citation51]. Rice plants were grown in 12 h light/12 h dark conditions for 8 days, and shoots were harvested at 3 h intervals. Three biological replicates were used to determine expression. Hd3a expression in PRR5-FLAG was significantly lower than the control during at time 3 and 15 (student-t test p < 0.05) ()). Hd3a expression at the other time points seemed to be lowered in PRR5-FLAG but not significantly (P > 0.05). Downregulation of Hd3a at time 3 was also observed in the other PRR5-FLAG overexpression line (), right). The data suggests that PRR5-FLAG represses flowering though the down-regulation of Hd3a expression ()).
Figure 5. Expression of Hd3a and OsLHY in PRR5-FLAG rice. (a) Expression of Hd3a and (b) OsLHY normalized with glyceraldehyde-3-phosphate dehydrogenase (OsGPC1) in rice. Note that OsGPC1 expression was not cyclic. Blue and red lines indicate Vector#1 and PRR5-FLAG#4, respectively. Blue and red dots indicate biological replicates of Vector#1 and PRR5-FALG#4, respectively (left panels). Maximal expression in Vector#1 was defined as 1.0. Gray areas indicate the dark period. Asterisks are significant changes between Vector #1 and PRR5-FLAG #4 (Student’s t-test p < 0.05) (left panels). Expression of Hd3a and OsLHY in PRR5-FLAG#6 at 3 h after light on (n = 3, right panels). Bar and dots indicate mean and individual values, respectively. (c) Model for the effect of the PRR9/PRR5 sub-group on flowering time and plant biomass. Enhancement of PRR5 function in long-day plants results in early flowering, but attenuation of PRR5 causes a biomass increase due to late flowering. Enhanced PRR function in short-day plants increases biomass likely through flowering-dependent pathways.
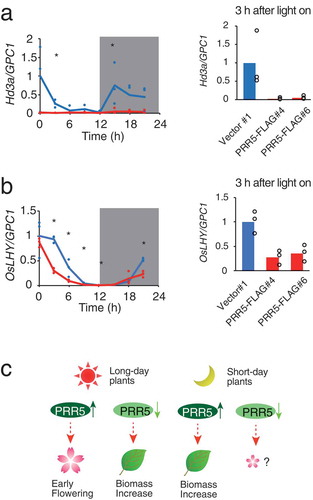
We also examined whether the presence of PRR5-FLAG in rice regulates the expression of a possible OsPRR-direct target gene, OsLHY [Citation28] ()). Expression of OsLHY peaked at time 0 in the control plants and PRR5-FLAG plants. The expression of OsLHY was lower in PRR5-FLAG at time 3, 9, and 21. Downregulation of OsLHY at time 3 was also observed in the other PRR5-FLAG overexpression line (), right). These data also suggested that PRR5 action on OsLHY is not as strong in rice as in Arabidopsis LHY.
To recapitulate, biomass in a short-day plant rice was greater after the introduction of a single gene, PRR5-FLAG, that caused early flowering in Arabidopsis, a long-day plant ()).
Discussion
Tuning flowering time has been a crucial trait allowing for the spread of crops globally. In rice, genetic studies demonstrated that there are some genetic loci (heading date) that control flowering time. OsPRR37 is known to be the responsible gene for the Hd2 locus, which is involved in photoperiodic flowering regulation [Citation36]. Functional Hd2 is proposed to inhibit the transition of vegetative growth to the reproductive phase under long-day conditions [Citation39]. One sorghum PRR7/PRR3 subgroup gene (SbPRR37) inhibits the transition of flowering time [Citation9]; however, it has not been clear whether the PRR9/PRR5 sub-group is involved in flowering time regulation in short-day plants. In this study, we found that the introduction of Arabidopsis PRR5-FLAG resulted in late flowering. This study is the first demonstration that a PRR9/PRR5 sub-group gene delays flowering time in a short-day plant ()). Although we did not use rice PRR9/PRR5 to compare the same constructs (PRR5-FLAG and PRR5-VP) in both a long-day plant (Arabidopsis)10 and a short-day plant (rice) as a proof-of-concept study, we suggest that rice PRR9/PRR5 genes are also involved in flowering time since proteins in the PRR9/PRR5 sub-group are very similar to each other24,25. Opposite functions of flowering time regulators (e.g., PRR7/PRR3 subgroup, ELF3, CO) between long-day plants and short-day have been reported previously [Citation9,Citation36,Citation52,Citation53].
A PRR gene in the short-day plant sorghum (SbPRR37) inhibits the transition of flowering time by modulating the expression of genes likely involved in flowering time [Citation9]. SbPRR37 expression is observed in the morning and in the evening during long-days (16 h light 8 h dark), and CO expression around dawn was attenuated in SbPRR37 mutants. On the other hand, possible flowering activators Ehd1, FT, and ZCN8 are upregulated in nonfunctional alleles of SbPRR37, showing that the PRR controls a transcriptional cascade for flowering time in a short-day plant. The expression of Hd3a, a rice florigen gene, was lower than the control in PRR5-FLAG-expressing rice ()), suggesting that PRR5-FLAG can control Hd3a expression even though many genes are considered to regulate Hd3a directly or indirectly. We hypothesize that PRR5 can indirectly regulate Hd3a transcription through modulating clock output genes such as homologues of Arabidopsis CDF, direct targets of Arabidopsis PRR5. It is also possible that PRR5-FLAG directly controls transcription of Hd3a, since the expression timing of rice PRR and that of Hd3a are inversely correlated [Citation28] ()), and Arabidopsis CO and PRR form complexes to control FT transcription [Citation43]. The molecular mechanism for flowering time regulation in which PRR9/PRR5 controls Hd3a expression in rice will be examined using the rice PRR9/PRR5 sub-group in the future.
A rice mutant for OsGI is known to have an early flowering phenotype only when grown in a greenhouse or a growth chamber, but not in the field [Citation54]. Therefore, it is important to state that our results were obtained in from greenhouse-grown and growth chamber-grown plants. Thus, PRR5-FLAG-expressing rice should be carefully analyzed from field-grown material to judge whether our strategy is really useful for gaining biomass. Another rice study demonstrated a correlation between flowering time and biomass; however, this study also showed that flowering time and grain yield were not correlated [Citation7]. Our results also demonstrated that the total panicle dry weight of PRR5-FLAG-expression lines was not greater than that of the control line ()). The increase in the grain filling rate by PRR5-FLAG was an unexpected result ()). We also found a very low grain filling rate of PRR5-VP-expressing rice, suggesting that the PRR9/PRR5 sub-group may control grain filling. Many circadian clock investigations have used plants in the vegetative phase. Understanding the relationship between the clock and grain filling is an agriculturally beneficial issue.
PRR5-VP-expressing rice did not flower early (), although PRR5-VP rice had shorter leaves and a lower biomass. These results may suggest that the threshold for PRR5-VP activity to regulate flowering time is greater than that for leaf length; however, it is possible to create early flowering rice by attenuating the PRR9/PRR5 function by other methods such as genome-editing that targets OsPRR95 and OsPRR59. Early flowering rice may meet some recent demands by farmers who want to escape rice grain damage from unexpectedly hot temperatures in the summer in the temperate zone. Global climate change will force us to alter the current areas for maize and soya production in the near future [Citation55]. Tuning crop flowering time has been used to spread and adapt crops to other climate areas [Citation30,Citation37]. Further understanding of flowering time will be a significant boon for generating cultivars with altered flowering times and will create new agricultural tools for precisely controlling flowering time against the emerging patterns of global climate change.
Author contributions
N.N. conceived the idea, performed analyses, and co-wrote and revised the manuscript. T.Ku performed analyses and co-wrote the manuscript. N.M. performed analyses. T.Kib. conceived the idea and co-wrote the manuscript. T.Kin. co-wrote the manuscript. H.S. conceived the idea and co-wrote the manuscript.
Supfig.pdf
Download PDF (2.7 MB)Acknowledgments
We thank Prof. Makoto Matsuoka for providing the binary vector.
Disclosure statement
No potential conflict of interest was reported by the authors.
Additional information
Funding
References
- Ainsworth EA, Long SP. What have we learned from 15 years of free-air CO2 enrichment (FACE)? A meta-analytic review of the responses of photosynthesis, canopy. New Phytol. 2005 Feb;165(2):351–371.
- Terashima I, Yanagisawa S, Sakakibara H. Plant responses to CO2: background and perspectives. Plant Cell Physiol. 2014 Feb;55(2):237–240.
- Yamori W, Kondo E, Sugiura D, et al. Enhanced leaf photosynthesis as a target to increase grain yield: insights from transgenic rice lines with variable Rieske FeS protein content in the cytochrome b(6)/f complex. Plant Cell Environ. 2016 Jan;39(1):80–87.
- Ishikawa C, Hatanaka T, Misoo S, et al. Functional incorporation of sorghum small subunit increases the catalytic turnover rate of rubisco in transgenic rice. Plant Physiol. 2011 Jul;156(3):1603–1611.
- Wang Y, Noguchi K, Ono N, et al. Overexpression of plasma membrane H+-ATPase in guard cells promotes light-induced stomatal opening and enhances plant growth. Proc Natl Acad Sci U S A. 2014 Jan 7;111(1):533–538.
- Kebeish R, Niessen M, Thiruveedhi K, et al. Chloroplastic photorespiratory bypass increases photosynthesis and biomass production in Arabidopsis thaliana. Nat Biotechnol. 2007 May;25(5):593–599.
- Hasegawa T, Sakai H, Tokida T, et al. Rice cultivar responses to elevated CO2 at two free-air CO2 enrichment (FACE) sites in Japan. Funct Plant Biol. 2013;40(2):148–159.
- Turner A, Beales J, Faure S, et al. The pseudo-response regulator Ppd-H1 provides adaptation to photoperiod in barley. Science. 2005 Nov 11;310(5750):1031–1034.
- Murphy RL, Klein RR, Morishige DT, et al. Coincident light and clock regulation of pseudoresponse regulator protein 37 (PRR37) controls photoperiodic flowering in sorghum. Proc Natl Acad Sci U S A. 2011 Sep 27;108(39):16469–16474.
- Nakamichi N, Takao S, Kudo T, et al. Improvement of Arabidopsis biomass and cold, drought and salinity stress tolerance by modified circadian clock-associated PSEUDO-RESPONSE REGULATORs. Plant Cell Physiol. 2016 MAY;57(5):1085–1097.
- Redei G. Supervital mutants of Arabidopsis. Genetics. 1962 Apr;47(4):443–460.
- Kobayashi Y, Kaya H, Goto K, et al. A pair of related genes with antagonistic roles in mediating flowering signals. Science. 1999 Dec 3;286(5446):1960–1962.
- Kardailsky I, Shukla VK, Ahn JH, et al. Activation tagging of the floral inducer FT. Science. 1999 Dec 3;286(5446):1962–1965.
- Yanovsky MJ, Kay SA. Molecular basis of seasonal time measurement in Arabidopsis. Nature. 2002 Sep 19;419(6904):308–312.
- Imaizumi T, Kay SA. Photoperiodic control of flowering: not only by coincidence. Trends Plant Sci. 2006;Nov;11(11):550–558.
- Makino S, Kiba T, Imamura A, et al. Genes encoding pseudo-response regulators: insight into His-to-Asp phosphorelay and circadian rhythm in Arabidopsis thaliana. Plant Cell Physiol. 2000 Jun;41(6):791–803.
- Mizuno T, Nakamichi N. Pseudo-Response Regulators (PRRs) or True Oscillator Components (TOCs). Plant Cell Physiol. 2005 May;46(5):677–685.
- Kiba T, Henriques R, Sakakibara H, et al. Targeted degradation of PSEUDO-RESPONSE REGULATOR5 by an SCFZTL complex regulates clock function and photomorphogenesis in Arabidopsis thaliana. Plant Cell. 2007 Aug;19(8):2516–2530.
- Kim WY, Fujiwara S, Suh SS, et al. ZEITLUPE is a circadian photoreceptor stabilized by GIGANTEA in blue light. Nature. 2007 Sep 20;449(7160):356–360.
- Saito AN, Matsuo H, Kuwata K, et al. Structure-function study of a novel inhibitor of the Casein kinase 1 family in Arabidopsis thaliana. Plant Direct. 2019 Sep;3(9):e00172.
- Ono A, Sato A, Fujimoto KJ, et al. 3,4-dibromo-7-azaindole modulates Arabidopsis circadian clock by inhibiting Casein kinase 1 activity. Plant Cell Physiol. 2019 Nov 1;60(11):2360–2368.
- Uehara TN, Mizutani Y, Kuwata K, et al. Casein kinase 1 family regulates PRR5 and TOC1 in the Arabidopsis circadian clock. Proc Natl Acad Sci U S A. 2019 Jun 4;116(23):11528–11536.
- Fujiwara S, Wang L, Han L, et al. Post-translational regulation of the Arabidopsis circadian clock through selective proteolysis and phosphorylation of pseudo-response regulator proteins. J Biol Chem. 2008 Aug 22;283(34):23073–23083.
- Gendron JM, Pruneda-Paz JL, Doherty CJ, et al. Arabidopsis circadian clock protein, TOC1, is a DNA-binding transcription factor. Proc Natl Acad Sci U S A. 2012 Feb 6;109(8):3167–3172.
- Nakamichi N, Kiba T, Kamioka M, et al. Transcriptional repressor PRR5 directly regulates clock-output pathways. Proc Natl Acad Sci U S A. 2012 Oct 16;109(42):17123–17128.
- Nakamichi N, Kiba T, Henriques R, et al. PSEUDO-RESPONSE REGULATORS 9, 7, and 5 are transcriptional repressors in the Arabidopsis circadian clock. Plant Cell. 2010 Mar;22(3):594–605.
- Wang L, Kim J, Somers DE. Transcriptional corepressor TOPLESS complexes with pseudoresponse regulator proteins and histone deacetylases to regulate circadian transcription. Proc Natl Acad Sci U S A. 2013 Jan 8;110(2):761–766.
- Toda Y, Kudo T, Kinoshita T, et al. Evolutionary insight into the clock-associated PRR5 transcriptional network of flowering plants. Sci Rep-Uk. 2019;27:9.
- Murakami M, Matsushika A, Ashikari M, et al. Circadian-associated rice pseudo response regulators (OsPRRs): insight into the control of flowering time. Biosci Biotechnol Biochem. 2005 Feb;69(2):410–414.
- Nakamichi N. Adaptation to the local environment by modifications of the photoperiod response in crops. Plant Cell Physiol. 2015 Apr;56(4):594–604.
- Ito S, Niwa Y, Nakamichi N, et al. Insight into missing genetic links between two evening-expressed pseudo-response regulator genes TOC1 and PRR5 in the circadian clock-controlled circuitry in Arabidopsis thaliana. Plant Cell Physiol. 2008 Feb;49(2):201–213.
- Beales J, Turner A, Griffiths S, et al. A pseudo-response regulator is misexpressed in the photoperiod insensitive Ppd-D1a mutant of wheat (Triticum aestivum L.). Theor Appl Genet. 2007 Sep;115(5):721–733.
- Yamamoto Y, Sato E, Shimizu T, et al. Comparative genetic studies on the APRR5 and APRR7 genes belonging to the APRR1/TOC1 quintet implicated in circadian rhythm, control of flowering time, and early photomorphogenesis. Plant Cell Physiol. 2003 Nov;44(11):1119–1130.
- Matsushika A, Murakami M, Ito S, et al. Characterization of Circadian-associated pseudo-response regulators: I. Comparative studies on a series of transgenic lines misexpressing five distinctive PRR genes in Arabidopsis thaliana. Biosci Biotechnol Biochem. 2007 Feb;71(2):527–534.
- Murakami M, Yamashino T, Mizuno T. Characterization of circadian-associated APRR3 pseudo-response regulator belonging to the APRR1/TOC1 quintet in Arabidopsis thaliana. Plant Cell Physiol. 2004 May;45(5):645–650.
- Koo BH, Yoo SC, Park JW, et al. Natural variation in OsPRR37 regulates heading date and contributes to rice cultivation at a wide range of latitudes. Mol Plant. 2013 May 27;6(6):1877–1888.
- Li MW, Liu W, Lam HM, et al. Characterization of two growth period QTLs reveals modification of PRR3 genes during soybean domestication. Plant Cell Physiol. 2019 Feb;60(2):407–420.
- Yano M, Sasaki T. Genetic and molecular dissection of quantitative traits in rice. Plant Mol Biol. 1997 Sep;35(1–2):145–153.
- Yamamoto T, Kuboki Y, Lin SY, et al. Fine mapping of quantitative trait loci Hd-1, Hd-2 and Hd-3,controlling heading date of rice, as single Mendelian factors. Theor Appl Genet. 1998;97:37–44.
- Nakamichi N, Kita M, Niinuma K, et al. Arabidopsis clock-associated pseudo-response regulators PRR9, PRR7 and PRR5 coordinately and positively regulate flowering time through the canonical CONSTANS-dependent photoperiodic pathway. Plant Cell Physiol. 2007 Jun;48(6):822–832.
- Imaizumi T, Schultz TF, Harmon FG, et al. FKF1 F-box protein mediates cyclic degradation of a repressor of CONSTANS in Arabidopsis. Science. 2005 Jul 8;309(5732):293–297.
- Song YH, Smith RW, To BJ, et al. FKF1 conveys timing information for CONSTANS stabilization in photoperiodic flowering. Science. 2012 May 25;336(6084):1045–1049.
- Hayama R, Sarid-Krebs L, Richter R, et al. PSEUDO RESPONSE REGULATORs stabilize CONSTANS protein to promote flowering in response to day length. EMBO J. 2017 Apr 3;36(7):904–918.
- Saitou N, Nei M. The neighbor-joining method: a new method for reconstructing phylogenetic trees. Mol Biol Evol. 1987 Jul;4(4):406–425.
- Felsenstein J. Confidence limits on phylogenies: an approach using the bootstrap. Evolution. 1985 Jul;39(4):783–791.
- Zuckerkandl E, Pauling L. Evolutionary divergence and convergence in proteins. In: Bryson V, Vogel HJ, editors. Evolving genes and proteins. New York: Academic Press; 1965. p. 97–166.
- Kumar S, Stecher G, Tamura K. MEGA7: molecular evolutionary genetics analysis version 7.0 for bigger datasets. Mol Biol Evol. 2016 Jul;33(7):1870–1874.
- Sentoku N, Sato Y, Matsuoka M. Overexpression of rice OSH genes induces ectopic shoots on leaf sheaths of transgenic rice plants. Dev Biol. 2000 Apr 15;220(2):358–364.
- Kudo T, Makita N, Kojima M, et al. Cytokinin activity of cis-zeatin and phenotypic alterations induced by overexpression of putative cis-Zeatin-O-glucosyltransferase in rice. Plant Physiol. 2012 Sep;160(1):319–331.
- Komiya R, Ikegami A, Tamaki S, et al. Hd3a and RFT1 are essential for flowering in rice. Development. 2008 Feb;135(4):767–774.
- Kojima S, Takahashi Y, Kobayashi Y, et al. Hd3a, a rice ortholog of the Arabidopsis FT gene, promotes transition to flowering downstream of Hd1 under short-day conditions. Plant Cell Physiol. 2002 Oct;43(10):1096–1105.
- Hayama R, Yokoi S, Tamaki S, et al. Adaptation of photoperiodic control pathways produces short-day flowering in rice. Nature. 2003 Apr 17;422(6933):719–722.
- Saito H, Ogiso-Tanaka E, Okumoto Y, et al. Ef7 encodes an ELF3-like protein and promotes rice flowering by negatively regulating the floral repressor gene Ghd7 under both short- and long-day conditions. Plant Cell Physiol. 2012 Apr;53(4):717–728.
- Izawa T, Mihara M, Suzuki Y, et al. Os-GIGANTEA confers robust diurnal rhythms on the global transcriptome of rice in the field. Plant Cell. 2011 May;13(23):1741–1755.
- Fodor N, Challinor A, Droutsas I, et al. Integrating plant science and crop modeling: assessment of the impact of climate change on soybean and maize production. Plant Cell Physiol. 2017 Nov;58(11):1833–1847.