ABSTRACT
Vancomycin (Van) is a common antibiotic that pollutes food, feed and ecological environment. In the present study, an anti-Van polyclonal antibodies (pAbs) and Eu3+-labeled goat anti-rabbit IgG monoclonal antibody based indirect competitive time-resolved fluoroimmunoassay (IC-TRFIA) was established for ultrasensitive determination of Van, and its half-maximum inhibition concentrations (IC50) was 13.74 ng/L and the limit of detection (LOD) was 1.07 ng/L. The IC-TRFIA showed strong cross-reactivity (CRs) of 36.2% for norvancomycin, and less than 0.1% for polymyxin B, kanamycin and ampicillin. It was found that the IC-TRFIA for Van spiked in different milk powder samples determination were with good accuracy, stability and repeatability, and its recoveries are 83.0–97.9%, also with its coefficient of variations (CVs) are 3.0–12.9% in intra-assay and inter-assay. The results showed that the established IC-TRFIA was promising for ultrasensitive determination of Van in food samples.
Highlights
Prepared high-quality Van-KLH/BSA conjugates.
Obtained high activity and purify anti-Van polyclonal antibodies.
Prepared high-quality Eu3+-labelled goat anti-rabbit IgG monoclonal antibody.
Firstly established an IC-TRFIA for Van based on the anti-Van polyclonal antibodies and Eu3+-labelled goat anti-rabbit IgG monoclonal antibody.
The IC-TRFIA can be used for ultrasensitive determination of Van in milk powder samples.
1. Introduction
Vancomycin (Van) is a kind of small molecule glycopeptide antibiotic that mainly applied for gram-positive bacteria prevention and control, especially for Staphylococcus aureus, Streptococcus pyogenes and Streptococcus pneumonia (Alvarez, Lopez Cortes, Molina, Cisneros, & Pachon, Citation2016). So far, Van has been widely used for the treatment of methicillin-resistant S. aureus in clinical applications, and it has played an important role in human and animal health safeguarding (Lee et al., Citation2018). However, owing to the long-term irrational use of antibiotics or even abuse, it has been a serious biosafety hazards for human and animal health and environmental microorganism (Grenni, Ancona, & Barra Caracciolo, Citation2018; Limbu, Zhou, Sun, Zhang, & Du, Citation2018). There are ample evidences to suggest that Van has a nephrotoxicity for human and animals, and it also has induced microbial resistance development (Aldawsari & Hosny, Citation2018; Omara, Citation2017). Therefore, it is important to effectively monitor the antibiotics including the Van residue in food and natural environment for human and animal health and even environmental microorganisms (Mehlhorn, Rahimi, & Joseph, Citation2018).
Currently, the reported methods that could be used for antibiotics determination contains instrument-based analysis [such as high-performance liquid chromatography (HPLC), gas chromatography-mass spectrometry (GC-MS) and liquid chromatography-tandem mass spectrometry (LC-MS)], antibody-based immunoassay [such as enzyme-linked immunosorbent assay (ELISA), time-resolved fluoroimmunoassay (TRFIA) and lateral-flow immunochromatographic assay (LF-IA)] and aptamer-based biosensors, etc. (Kong et al., Citation2017; Mehlhorn et al., Citation2018; Zhang et al., Citation2019). Among them, the antibody-based immunoassay is the hottest and the most potential application technology in the detection and analysis research fields, because its superiority for simple operation, rapid reaction, sensitive detection and low cost, etc. (Aga et al., Citation2016; Xu et al., Citation2015). However, it is hard to directly obtain the ultrasensitive antibodies to target-antigen by traditional animal immunization (polyclonal antibodies and monoclonal antibody preparation) and even antibody library screening (selection of genetically engineered antibody) (Liu et al., Citation2016; Lu et al., Citation2018). At present, it is an effective means by using special chemiluminescent group labeled-antibody to improve the detection limit of antibody for antigen, and it has been widely used in immunology detection field (Acharya et al., Citation2016; Li et al., Citation2017; Sheng et al., Citation2016; You, Li, Li, & Ma, Citation2016). Eu3+ is a kind of fluorescing rare earth metals ion with superiority of high luminous efficiency, long relaxation time and resist high background absorption, and it was widely labelled to antibody and then used to establish time-resolved fluoroimmunoassay for ultrasensitive determination of hazards in food and feed (Hu et al., Citation2018b; Xu et al., Citation2017; Zhang et al., Citation2015).
So far, there is no public report by using Eu3+-labelled time-resolved fluoroimmunoassay to detect the Van residue in food, feed or environmental samples. In this study, to establish Eu3+-labelled time-resolved fluoroimmunoassay for ultrasensitive determination of Van in food samples, firstly, we prepared the high-quality Van-KLH/BSA conjugates to stimulate rabbit produce high-affinity activity of anti-Van polyclonal antibodies, at the same time, we also prepared the high-quality Eu3+-labelled goat anti-rabbit IgG monoclonal antibody. Then an indirect competitive time-resolved fluoroimmunoassay was established based on the prepared purified anti-Van polyclonal antibodies and Eu3+-labelled goat anti-rabbit IgG monoclonal antibody for Van, and this detection method of practicability and applicability was evaluated by the determination of Van spiked in different milk powders samples.
2. Materials and methods
2.1. Materials and reagents
Vancomycin, norvancomycin, polymyxin B, kanamycin, ampicillin, N,N-dimethylformamide (DMF), N-hydroxysuccinimide (NHS), 1-ethyl-3-[3-dimethylaminopropyl]-carbodimide (EDC), keyhole limpet haemocyanin (KLH), bovine serum albumin (BSA), ovalbumin (OVA), tween 20, trypsin and 3,3,5,5-tetramethylbenzidine (TMB), Freund’s complete adjuvant and Freund’s incomplete adjuvant were all purchased from Sigma-Aldrich (Beijing, China). The New Zealand white rabbit used for Van-KLH immunization was supplied from the national research centre of veterinary biologicals engineering and technology in Jiangsu Academy of Agricultural Sciences (Nanjing, China), and all the experimental protocols were approved by the animal experiment ethics committee of authors’ institute. Eu3+, TRFIA assay buffer, fluorescence enhancement solution, TRFIA wash buffer were purchased from PerkinElmer Company (USA). Goat anti-rabbit IgG monoclonal antibody [HRP] and goat anti-rabbit IgG monoclonal antibody were purchased from GenScript Bio.Co.Ltd (Nanjing, China). Vancomycin monoclonal antibody [immunogen was Van-BTG (bovine thyroglobulin, BTG)] was purchased from United States Biological Company (USA). Whole milk powder, skim milk powder and instant milk powder were purchased from Suguo supermarket (Nestle, Switzerland). Other reagents were all purchased from GE Healthcare (Beijing, China).
2.2. Preparation and identification of Van-KLH/BSA conjugates
To obtain Van-KLH and Van-BSA conjugates used for immunizing antigen and coating antigen respectively, the Van was combined to KLH and BSA as described by our previous report (Xu et al., Citation2018) with its optimization. In brief, 2.5 mg Van standards dissolved in 0.4 mL DMF liquor, then slowly added into 1.2 mL DMF liquor contains 3.0 mg NHS and 3.0 mg EDC. The mixture system was shaking for 40 min at 25 rpm and room temperature, and then continue shaking for overnight at 25 rpm and 4°C. Next day, the mixture system was slowly added into the KLH/BSA solution contains 10 mg KLH or BSA dissolved in 12 mL 0.1 M sodium carbonate buffer (CBS, pH = 9.6), and then shaking 4 h at 25 rpm and room temperature in dark. Then the mixtures solution was added into Amicon Ultra-4-Ultracel-3 K (Millipore, USA) and centrifuged for 30 min at 10,000 rpm and 4°C. The ultrafiltration tube retentate was resuspended with 10 mL 0.1 M sodium phosphate buffer (PBS, pH = 7.4) and centrifuged for 30 min at 10,000 rpm and 4°C, and repeat this operating step five times. In final, to collect the ultrafiltration tube retentate and quantify its volume to 5 mL with 0.1 M PBS buffer, and the concentration of prepared Van-KLH or Van-BSA conjugates will be identified as 2 mg/mL. The coupling effect of prepared Van-KLH/BSA conjugates were analyzed by an ultraviolet full wavelength scanner (Agilent, USA) and ELISA based on an anti-Van monoclonal antibody (immunogen was Van-BTG), respectively.
The operating steps of anti-Van monoclonal antibody-based ELISA for Van-KLH/BSA conjugates as follow. In brief, the concentration of Van-KLH/BSA conjugates were diluted to 0.03, 0.06, 0.12, 0.25, 0.5, 1.0, 2.0 and 4.0 μg/mL with 0.1 M PBS buffer, and then coated 100 μL/well in 96-well plate for standing overnight at 4°C, respectively. The negative controls were coated the same concentration of KLH/BSA. Next day, the coated 96-well plate was washed with 300 μL/well of PBST solution (contains 0.1% tween 20 in PBS buffer) for three times, and then blocked with 300 μL/well of OPBS solution (5% OVA dissolved in PBS buffer) for incubating 2 h at 37°C in water bath kettle. The 96-well plate was washed as described above, and then added 100 μL/well of 1:2500 dilution of anti-Van monoclonal antibody in CBS buffer for incubating 2 h at 37°C in water bath kettle. The 96-well plate was washed again, and then added 100 μL/well of 1:2500 dilution of goat anti-mouse IgG monoclonal antibody [HRP] in CBS buffer for incubating 2 h at 37°C in water bath kettle. The 96-well plate was washed again, and then added 100 μL/well of TMB solution to wait the colour development for 20 min at room temperature. In final, the 96-well plate reaction system of OD values were measured by an automatic microplate reader (Berthold, Germany) after being added 50 μL/well of 2 M sulfuric acid to terminate the reaction. All the test experiments were repeated in triplicate and the presented data were the mean value.
2.3. Immunization of Van-KLH conjugates to rabbit
To stimulate rabbit produce the anti-Van pAbs effectively, total four rounds of intermittent injections with different gradient concentrations of Van-KLH conjugates were performed as follow. In brief, a healthy New Zealand white rabbit was selected as the experimental subject and extracted about 1 mL blood from its ear vein used for negative control. In the first round of immunization, the rabbit subcutaneous was injected 1 mL Van-KLH conjugates solution (0.8 mg/mL Van-KLH conjugate in PBS buffer) mixed with 1 mL Freund’s complete adjuvant of emulsified complexes. Two weeks later, 1 mL Van-KLH conjugates solution (1.0 mg/mL Van-KLH conjugate in PBS buffer) mixed with 1 mL Freund’s incomplete adjuvant of emulsified complexes were injected into the rabbit subcutaneous in the second round of immunization. Another two weeks later, 1 mL Van-KLH conjugates solution (1.2 mg/mL Van-KLH conjugates in PBS buffer) mixed with 1 mL Freund’s incomplete adjuvant of emulsified complexes were injected into the rabbit subcutaneous in the third round of immunization. After another week, 1 mL Van-KLH conjugates solution (1.6 mg/mL Van-KLH conjugates in PBS buffer) was directly injected into the rabbit in the fourth round of immunization (the booster immunization). Another five days later, the blood which contained anti-Van pAbs were collected from the immunized rabbit heart.
About 1 mL blood was collected from the immunized rabbit ear vein before each rounds of immunization and stood overnight at 4°C for separating out of serum. The titre of each rounds of collected serum to Van were analyzed from a serum-based ELISA (coated Van-BSA conjugates) as follow. In brief, 100 μL/well of Van-BSA conjugates solution (2.5 μg/mL Van-BSA conjugates in PBS buffer) were coated into 96-well plate for standing overnight at 4°C. Next day, the plate was washed with PBST solution and blocked with OPBS solution as described above. Each rounds of the collected serum were diluted nine times in a row according to 10-fold dilution method by CBS buffer, and then 100 μL/well of diluted serum were respectively added into the washed plate for incubating 2 h at 37°C in water bath kettle. The plate was washed again, and then added 100 μL/well of 1:2500 dilution of goat anti-rabbit IgG monoclonal antibody [HRP] in CBS buffer for incubating 2 h at 37°C in water bath kettle. In final, the plate was washed with PBST solution, added TMB solution for colour development, added 2 M sulfuric acid for termination reaction and measured by an automatic microplate reader for OD values, etc. steps were all performed as described above. All the test experiments were repeated in triplicate and the presented data were the mean value.
2.4. Preparation and analysis of purify rabbit anti-Van pAbs
To obtain the purified rabbit anti-Van pAbs, the finally collected serum from the immunized rabbit heart was roughly purified by saturated ammonium sulfate precipitation and precisely purified by using a HiTrap protein A HP columns (GE, USA), successively. In brief, about 200 mL blood was collected from the Van-KLH conjugate immunized rabbit heart and stood for 1 h at room temperature immediately, and then transferred to 4°C for standing overnight. Next day, about 100 mL serum was collected from the blood and mixed with stroke-physiological saline solution [SPSS, contains 0.9% sodium chloride dissolved in double distilled water (ddH2O)] by ratio of 1:1 (v/v), and then drop by drop added saturated ammonium sulfate solution (SASS) until its concentration reached to 20%. The mixture system was stood for 30 min at room temperature, and then centrifuged for 20 min at 4°C and 10,000 g. The supernatant was collected and then drop by drop added SASS until its concentration reached to 50%. The mixture system stood and centrifuged as described above, and then the precipitated proteins were collected and dissolved in SPSS for standing 30 min at room temperature, with that drop by drop added SASS until its concentration reached to 33%. The roughly purified anti-Van pAbs (precipitated proteins) were obtained from the mixture system after being centrifuged for 20 min at 4°C and 10,000 g. In final, to obtain the high-purified anti-Van pAbs, the roughly purified antibody proteins were further precisely purified by employ HiTrap Protein A HP columns (GE, USA) as performed in the handbook described. (http://www.blossombio.com/pdf/products/UG_71700200AP.pdf). The purification efficacy of anti-Van pAbs proteins were analyzed by SDS-PAGE as described by our similar report (Xu et al., Citation2018).
2.5. Preparation and evaluation of Eu3+-labelled goat anti-rabbit IgG monoclonal antibody
Eu3+-labelled goat anti-rabbit IgG monoclonal antibody was prepared as follows. In brief, total 5 mg Eu3+ was adequately dissolved in 500 μL ddH2O for standing 1 h at room temperature, at the same time, total 1 mg goat anti-rabbit IgG monoclonal antibody was adequately dissolved in 100 μL ddH2O for standing 1 h at room temperature too. Taking 10 μL of 0.5 M CBS buffer with drop by drop added into the goat anti-rabbit IgG monoclonal antibody solution, and then adequately mixed with the Eu3+ solution for standing overnight at 4°C immediately. Next day, the mixture system was added into a dialysis bag (8 kDa) for continuing dialysis three days in 1 L ddH2O at 4°C, and the ddH2O was changed three times every day to completely removal the redundant Eu3+ and carbonate ions. In final, the retentate in dialysis bag was collected and its volume was quantified to 1 mL with ddH2O, and the concentration of prepared Eu3+-labelled goat anti-rabbit IgG monoclonal antibody will be identified as 1 mg/mL.
The optimal working concentration of Eu3+-labelled goat anti-rabbit IgG monoclonal antibody was determined by establishing an indirect non-competitive TRFIA for the purified anti-Van pAbs. In brief, a 96-wells plate was prewashed with 250 μL/well TRFIA wash buffer for three times, and then added 100 μL/well of anti-Van pAbs solution (contains 2.5 μg/mL purified anti-Van pAbs in TRFIA assay buffer) for shake-coating 2 h at 750 rpm and room temperature. The negative control added equal volume of TRFIA assay buffer. The plate was washed with TRFIA wash buffer, and then added 100 μL/well of series diluted of Eu3+-labelled goat anti-rabbit IgG monoclonal antibody solution (contains 1000, 2000, 3000, 4000, 5000, 6000, 7000, 8000, 9000 and 10000-fold dilution in TRFIA assay buffer) for shake-incubating 1 h at 750 rpm and room temperature. The plate was washed with TRFIA wash buffer again, and then added 100 μL/wells of fluorescence enhancement solution for shaking 15 min at 750 rpm and room temperature. In final, the 96-well plate reaction system of OD values were measured by an automatic microplate reader (Berthold, Germany), and the optimal working concentration of Eu3+-labelled goat anti-rabbit IgG monoclonal antibody was determined from the maximum ratio of positive/negative and it was used for the follow-up experiments. All the test experiments were repeated in triplicate and the presented data were the mean value.
2.6. Purified anti-Van pAbs based IC-TRFIA for Van and its analogues
The purified anti-Van pAbs and prepared Eu3+-labelled goat anti-rabbit IgG monoclonal antibody-based IC-TRFIA was established to detect Van and its analogues. In brief, a 96-wells plate was prewashed with TRFIA wash buffer as described above, and then added 100 μL/well of Van-BSA conjugates solution (2.5 μg/mL Van-BSA conjugates in TRFIA assay buffer) for coating overnight at 4°C. Next day, the plate was washed with TRFIA wash buffer, and then added 50 μL of 0.1, 0.5, 1.0, 2.0, 5.0, 10, 20, 40, 80 and 100 ng/L Van/analogues in TRFIA assay buffer respectively mixed with 50 μL of the purified anti-Van pAbs in TRFIA assay buffer for shake-incubating 2 h at 750 rpm and room temperature. The plate was washed with TRFIA wash buffer, and then added 100 μL/well of the optimal working concentration of Eu3+-labelled goat anti-rabbit IgG monoclonal antibody solution for shake-incubating 1 h at 750 rpm and room temperature. In final, the plate was washed with TRFIA wash buffer, added fluorescence enhancement solution and measured by an automatic microplate reader for OD values, etc. steps were all performed as described above. The key evaluation index of IC10, IC20, IC50 and IC80 of the purified anti-Van pAbs for Van and its analogues were calculated by the formula of [(P − S − N)]/(P − N) × 100%. “P” was the OD value of the positive control reaction (50 μL of purified anti-Van pAbs solution + 50 μL of TRFIA assay buffer). “S” was the OD value of the standard reaction (50 μL of purified anti-Van pAbs solution + 50 μL of serial concentration gradient of Van/analogues). “N” was the OD value of the negative control reaction (100 μL of TRFIA assay buffer). The CRs of anti-Van pAbs for Van analogues were calculated by the formula of [CR (%) = IC50 (Van)/IC50 (Van analogues)] × 100%. All the test experiments were repeated in triplicate and the presented data were the mean value.
2.7. Assessment of the established IC-TRFIA for Van by spiked milk powder samples
To assess the practicability and applicability of the established IC-TRFIA for detecting Van in food samples, which the whole milk powder, skim milk powder and instant milk powder were chosen and used for addition recovery experiment indoor. In brief, a 96-wells plate was prewashed with TRFIA wash buffer as described above, and then added 100 μL/well of Van-BSA conjugates solution (2.5 μg/mL Van-BSA conjugates in TRFIA assay buffer) for coating overnight at 4°C. Next day, the plate was washed with TRFIA wash buffer, and then each well was respectively added 50 μL of series levels of Van-milk solution mixed with 50 μL of the purified anti-Van pAbs in TRFIA assay buffer for shake-incubating 2 h at 750 rpm and room temperature. The series levels of Van-milk solution contains 0, 2, 10, and 50 ng/kg of Van spiked in the presented milk powders, and then dissolved (ratio of 1 mg milk powder: 1 mL TRFIA assay buffer) and further diluted 10-fold by TRFIA assay buffer, respectively. The plate was washed with TRFIA wash buffer, added the optimal working concentration of Eu3+-labelled goat anti-rabbit IgG monoclonal antibody solution, added fluorescence enhancement solution and measured by an automatic microplate reader for OD values, etc. steps were all performed as described above. All the test experiments were repeated in triplicate and the presented data were the mean value.
3. Results and discussion
3.1. Preparation of Van-KLH and Van-BSA conjugates
The molecular weight (M.W.) of Van is 1485 Da, and it belongs to a micromolecule hapten, which has no immunogenicity in its natural state (Gefen et al., Citation2015). Therefore, to obtain the anti-Van pAbs or monoclonal antibody from animals, it is necessary to couple the Van with a macromolecular carrier protein and then used for immunization. At present, BSA, OVA and KLH are the most common macromolecular carrier proteins for haptens conjugation in small molecule hazards immunoassay filed (Li et al., Citation2018; Yao et al., Citation2019). In this study, we chose KLH and BSA for the macromolecular carrier proteins, and prepared Van-KLH and Van-BSA conjugates, respectively. The absorption spectra of Van-KLH and Van-BSA conjugates from an ultraviolet full wavelength scanner were showed in (A). It was found that they all had an obvious offset in characteristic maximum absorption wavelength (280 nm) which compared with Van, KLH [(A1)] or BSA [(A2)], and their specific spectra indicated that the Van was successfully conjugated to KLH and BSA, respectively. Furthermore, the Van-KLH and Van-BSA conjugates were identified by an anti-Van monoclonal antibody-based ELISA as showed in (B), their limits of detected concentration were 0.06 μg/mL [(B1)] and 0.12 μg/mL [(B2)] by ratio of P/N > 3.0, respectively. It was worth mentioning that the anti-Van monoclonal antibody was descended from Van-BTG immunogen to mice, which specifically recognized Van but not the KLH and BSA carrier proteins in the present ELISA. Therefore, according to the dual identification of ultraviolet full wavelength scanning and ELISA for the prepared Van-KLH and Van-BSA conjugates that confirmed the Van was successfully conjugated to KLH and BSA carrier proteins, and both of them were high-quality and could be used for the follow-up experiments.
Figure 1 (A): The absorption spectra curves of KLH and BSA carrier proteins, Van-KLH and Van-BSA conjugates and Van from an ultraviolet full wavelength scanner. The Van, KLH and Van-KLH conjugates were belongs to proteins and they had a characteristic maximum absorption wavelength at 280 nm, and the Van-KLH conjugates had an obvious offset in the characteristic maximum absorption wavelength that compared with Van and KLH at the same concentration (A1). The BSA and Van-BSA were also belongs to proteins and they had a characteristic maximum absorption wavelength at 280 nm, and the Van-BSA conjugates had an obvious offset in the characteristic maximum absorption wavelength that compared with Van and BSA at the same concentration too (A2). (B): The ELISA values of prepared Van-KLH (B1) and Van-BSA (B2) conjugates analyzed by anti-Van monoclonal antibody (Van-BTG immunogen), and the OD450 values were the means ± SDs from triplicate measurements.
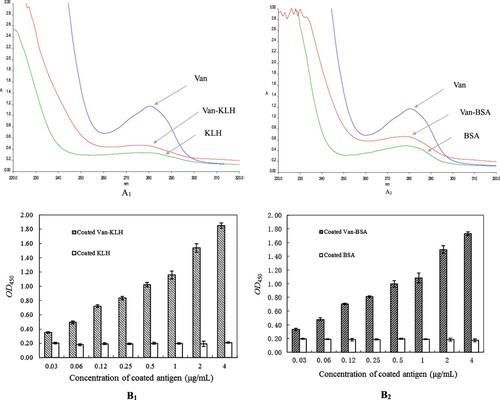
3.2. Immunization of Van-KLH conjugate to rabbit and preparation of purified anti-Van pAbs
It is a traditional and popular method to obtain high-affinity antibodies which are antigen-specific by using series gradients dose concentration of antigen injection, periodic interval and rounds of immunizations in the field of immune antibody preparation (Talib, Salam, & Sulaiman, Citation2018; Wang et al., Citation2018; Xu et al., Citation2019). According to the results in , we chose the Van-KLH conjugates as the immunogen to stimulate rabbit produce anti-Van pAbs. As showed in (A), after four rounds of series gradients dose concentration of Van-KLH conjugates antigen injection, the immune system of rabbit produced and accumulated lots of high-affinity activity of Van-specific pAbs for Van in serum. Total 100 mL serum with a titre of 1:1000000 was obtained from the final booster immunization of rabbit by heart blood collection, and the titre value indicated the prepared anti-Van pAbs had high-affinity activity to Van. In the present serum-based ELISA, we designedly chose the prepared Van-BSA conjugates as the coated antigen for the serum titre analyzation and the negative control was BSA, which could sufficiently eliminate distractions of KLH and BSA carrier proteins and the measured OD450 values were the only anti-Van pAbs specific binding to Van. Therefore, the presented titre of immunized serum for Van was credible, and the finally collected serum could be used for preparing purified anti-Van pAbs.
Figure 2 (A): The four rounds of immune efficacy analyzation of Van-KLH conjugate to rabbit by serum-based ELISA for Van, the coated antigen were Van-BSA and the negative control were coated equivalent BSA. The presented OD450 values were the means ± SDs from triplicate measurements. (B): The purify efficacy analyzation of anti-Van pAbs proteins from the booster immunized rabbit serum by SDS-PAGE, and the anti-Van pAbs proteins were successively purified by using saturated ammonium sulfate precipitation and HiTrap protein A HP columns. M: Protein marker; Lanes 1 and 2: The purified anti-Van pAbs proteins from HiTrap protein A HP columns were treated with β-mercaptoethanol denaturing buffer, and the two of heavy chain (approximately 50 kDa) and the two of light chain (approximately 25 kDa) were released, respectively. Lane 3: The purified anti-Van pAbs proteins (approximately 150 kDa) from HiTrap protein A HP columns were treated with non-denaturing buffer. Lanes 4 and 5: The primary purified anti-Van pAbs proteins from the booster immunized rabbit serum by using saturated ammonium sulfate precipitation; Lane 6: The primordial booster immunized rabbit serum.
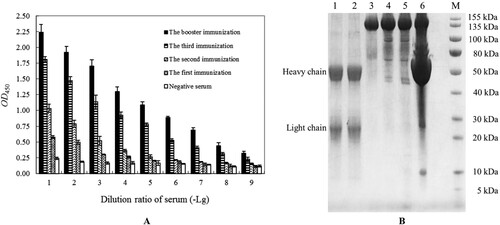
Usually, it is necessary to obtain purified antibody proteins, containing pAbs, monoclonal antibody and even genetically engineered antibody for the establishment of immunological detection methods (Liu et al., Citation2015; Ruano-Gallego, Fraile, Gutierrez, & Fernandez, Citation2019; Xu et al., Citation2019). At present, it is a common and effective way to obtain purified antigen-specific pAbs proteins from immunized serum by using saturated ammonium sulfate precipitation to roughly purify and then further using HiTrap protein A HP columns to precisely purify (Eivazi et al., Citation2015; Fan et al., Citation2015). As showed in (B), the anti-Van pAbs proteins were effectively captured and other proteins or weak-binding activity of proteins to Van were washed out gradually by saturated ammonium sulfate precipitation and HiTrap protein A HP columns. The obtained purified anti-Van pAbs proteins existed a sharp and clear pAbs size of protein band (approximately 150 kDa) in non-denaturing buffer, and it released two sharp and clear characteristic of heavy chain (approximately 50 kDa) and light chain (approximately 25 kDa) protein bands in denaturing buffer on SDS-PAGE, respectively. The finally collected purified pAbs proteins with a concentration of 4.5 mg/mL and its purity was 93.9%, indicating that we obtained a high-quality and concentration of anti-Van pAbs and it completely satisfied the application of the follow-up experiments.
3.3. Preparation of Eu3+-labelled goat anti-rabbit IgG monoclonal antibody
It is necessary to determine the optimal working concentration of Eu3+-labelled antibody or antigen in TRFIA (Hu et al., Citation2018a; Hu et al., Citation2018b), which not only minimize background interference but also minimize cost investment in the test. As showed in , the P/N values of prepared Eu3+-labelled goat anti-rabbit IgG monoclonal antibody for anti-Van pAbs in TRFIA were not positively or negatively correlated with its dilution. Finally, the determined optimal working concentration of Eu3+-labelled goat anti-rabbit IgG monoclonal antibody was 1:4000 dilution with P/N value of 20.82, and it was obviously better than the maximum dilution (1:10000) with P/N value of 3.76 and the minimum dilution (1:1000) with P/N value of 16.96 in the present TRFIA. In addition, the 1:4000 dilution of Eu3+-labelled goat anti-rabbit IgG monoclonal antibody below the recommended working concentration range of 1:5000 dilution for the employed goat anti-rabbit IgG monoclonal antibody, which could be used for IC-TRFIA establishment of Van in the follow-up experiments.
Figure 3. The optimal working concentration analyzation of the prepared Eu3+-labelled goat anti-rabbit IgG monoclonal antibody for the purified anti-Van rabbit pAbs by using an indirect non-competitive TRFIA. The presented P/N values were the means ± SDs from triplicate measurements. “P”: coated anti-Van rabbit pAbs in TRFIA assay buffer. “N”: added equal volume of TRFIA assay buffer.
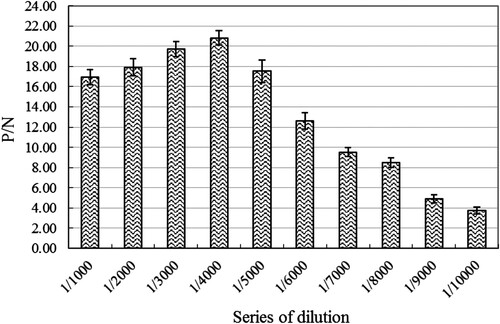
3.4. IC-TRFIA establishment of Van and its analogues
Indirect competitive immunoassay, containing IC-ELISA and IC-TRFIA which were the most common method for the small molecule hazards (such as small molecule pesticides, biotoxins and antibiotics, etc.) determination (Kavanagh, Elliott, & Campbell, Citation2015; Ni et al., Citation2019; Watanabe, Miyake, & Yogo, Citation2013). Because of its superiority, the key evaluation index of antibody to antigen, containing IC10 (LOD), IC50 and IC20–IC80 (linear working range), which could be calculated out directly. The standard curve of established IC-TRFIA based on the purified anti-Van pAbs and Eu3+-labelled goat anti-rabbit IgG monoclonal antibody-based for Van was showed in . It was found that the IC50 value was 13.74 ng/L, the IC20–IC80 were 3.43–43.62 ng/L, and the IC10 reached up to 1.07 ng/L according to the established IC-TRFIA for Van. The LOD value was 1.07 ng/L in the presented IC-TRFIA for Van, which is more sensitive (approximately 60-fold) compared with Kong et al. (Citation2017) established IC-ELISA for Van with LOD value of 0.06 ng/mL, and approximately 460-fold, 2.0 × 105-fold and 2.5 × 105-fold compare with Liang et al. (Citation2015), Sheng and Zhou (Citation2017) and Usman and Hempel (Citation2016) which were established fluorescence “turn-off” biosensor, two-dimensional liquid chromatography and HPLC for Van with LOD value of 0.46 ng/mL, 0.2 and 0.25 μg/mL respectively. In addition, it was slightly better than Liu, Yan, Hua, and Wang (Citation2013), Hu et al. (2018), Zhou et al. (Citation2015) and Sheng et al. (Citation2016) established IC-TRFIAs for thiacloprid, aflatoxin B1, chloramphenicol and diniconazole with LOD value of 1.9, 3.55, 8.0 and 21 ng/L, respectively. At present, the Van belongs to a banned antibiotic drug in animal food in China, although there is no maximum residue limit standard of Van in it, the LOD value of 1.07 ng/L in the presented IC-TRFIA for Van is far below the maximum residue limit standard of other antibiotics (Liu, Steele, & Meng, Citation2017).
Figure 4. The standard curve of established IC-TRFIA for Van based on the purified anti-Van pAbs and Eu3+-labelled goat anti-rabbit IgG monoclonal antibody. The presented inhibition ratio values were the means ± SDs from triplicate measurements.
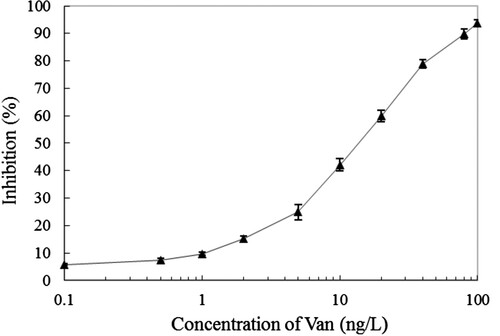
The cross-reactivity (CRs) of antibody to antigen analogue (contains structural and functional analogues) is an important indicator for specificity evaluation in the field of immunoassay (He et al., Citation2019; Luo et al., Citation2017; Shi et al., Citation2018). As showed in , the presented IC-TRFIA showed strong CRs of 36.2% for norvancomycin, and hardly binding to polymyxin B (less than 0.1%), kanamycin (not detected) and ampicillin (not detected). Containing similar chemical structural domain or common chemical group is the basic need for preparing the broad-specificity antibody to antigen and its analogues, in other words, it is a must that more or less CRs between them in immunoassay based on antibody (Dong et al., Citation2016; Zhao et al., Citation2016). The chemical structural and antibacterial function of norvancomycin is extremely similar to Van, and the presented CRs of 36.2% indicated the established IC-TRFIA could be used for simultaneous ultrasensitive determination.
Table 1. The CRs of the purified anti-Van pAbs and Eu3+-labelled goat anti-rabbit IgG monoclonal antibody-based IC-TRFIA for Van analogues (the presented values were the means from triplicate measurements).
3.5. IC-TRFIA for Van spiked in the milk powder samples
It is a common and effective way to evaluate the practicability and applicability of a newly established immunoassay based on antibody by the determination of antigen standard substance spiked in different samples indoor (Deng et al., Citation2016; Wang et al., Citation2018; Zhang, He, Zhao, Wang, & Jin, Citation2016). Van is a commonly used antibiotic drug to treat bovine mastitis, and it becomes one of the key monitoring object in milk and its products (Bhattacharyya et al., Citation2016; Kong et al., Citation2017; Yang et al., Citation2016). In the study, to evaluate the practicability and applicability of the established IC-TRFIA for the determination of Van in samples, we specially chose three different milk powders, containing whole milk powder, skim milk powder and instant milk powder as its spiked samples. According to the linear working range (IC20–IC80) of 3.43–43.62 ng/L from the established IC-TRFIA for Van (), we designed three different concentration levels (contains 2, 10 and 50 ng/kg) of Van spiked in the presented milk powders and then used for determination. The results showed in , the recoveries of three different concentration levels of Van spiked in whole milk powder, skim milk powder and instant milk powder samples were 83.0–96.5% with CVs of 5.4–12.9%, 83.4–93.5% with CVs of 4.5–9.0% and 83.5–97.9% with CVs of 3.0–9.0% in intra-assay and inter-assay, respectively. In summary, the established IC-TRFIA for the determination of Van spiked in the presented milk powder samples were all with recoveries of 83.0–97.9% and CVs of 3.0–12.9% in intra-assay and inter-assay, which indicated that the detection method had excellent accuracy, stability and reproducibility.
Table 2. The purified anti-Van pAbs and Eu3+-labelled goat anti-rabbit IgG monoclonal antibody-based IC-TRFIA for Van in spiked milk powder samples (the given values were the means ± SDs from triplicate measurements).
4. Conclusion
High-quality antibody material is the most prerequisite for the establishment of immunoassay. In the study, we successfully obtained the high binding activity of anti-Van pAbs by the prepared high-quality of Van-KLH conjugate to immunize rabbit. At the same time, a high-quality Eu3+-labelled goat anti-rabbit IgG monoclonal antibody was prepared and its optimal working concentration was determined successfully. In final, the purified anti-Van pAbs and optimized Eu3+-labelled goat anti-rabbit IgG monoclonal antibody-based IC-TRFIA was established with LOD value of 1.07 ng/L, indicating that the IC-TRFIA was ultrasensitive for Van. Furthermore, the IC-TRFIA had a good practicability and applicability for the determination of Van spiked in milk powder samples which indicated it was promising for ultrasensitive determination of Van residue in food, feed and environment samples.
Ethical statement
All involved animal experiments in the present study were compliance with ethical standards.
Disclosure statement
No potential conflict of interest was reported by the authors.
Additional information
Funding
References
- Acharya, D., Bastola, P., Le, L., Paul, A. M., Fernandez, E., Diamond, M. S., … Bai, F. (2016). An ultrasensitive electrogenerated chemiluminescence-based immunoassay for specific detection of Zika virus. Scientific Reports, 6, 1. doi: 10.1038/s41598-016-0001-8
- Aga, D. S., Lenczewski, M., Snow, D., Muurinen, J., Sallach, J. B., & Wallace, J. S. (2016). Challenges in the measurement of antibiotics and in evaluating their impacts in agroecosystems: A critical review. Journal of Environment Quality, 45(2), 407–419. doi: 10.2134/jeq2015.07.0393
- Aldawsari, H. M., & Hosny, K. M. (2018). Solid lipid nanoparticles of vancomycin loaded with ellagic acid as a tool for overcoming nephrotoxic side effects: Preparation, characterization, and nephrotoxicity evaluation. Journal of Drug Delivery Science and Technology, 45, 76–80. doi: 10.1016/j.jddst.2018.02.016
- Alvarez, R., Lopez Cortes, L. E., Molina, J., Cisneros, J. M., & Pachon, J. (2016). Optimizing the clinical use of vancomycin. Antimicrobial Agents and Chemotherapy, 60(5), 2601–2609. doi: 10.1128/AAC.03147-14
- Bhattacharyya, D., Banerjee, J., Bandyopadhyay, S., Mondal, B., Nanda, P. K., Samanta, I., … Bandyopadhyay, S. (2016). First report on vancomycin-resistant staphylococcus aureus in bovine and caprine milk. Microbial Drug Resistance, 22(8), 675–681. doi: 10.1089/mdr.2015.0330
- Deng, L.-H., Dai, J.-B., Xu, Z.-L., Yang, J.-Y., Wang, H., Xiao, Z.-L., … Shen, Y.-D. (2016). Application of time-resolved fluroimmunoassay for determination of furaltadone metabolite 3-amino-5-morpholinomethyl-2-oxazolidinone. Chinese Journal of Analytical Chemistry, 44(8), 1286–1290. doi: 10.1016/S1872-2040(16)60951-9
- Dong, S., Zhang, C., Zhang, X., Liu, Y., Zhong, J., Xie, Y., … Liu, X. (2016). Production and characterization of monoclonal antibody broadly recognizing Cry1 toxins by use of designed polypeptide as hapten. Analytical Chemistry, 88(14), 7023–7032. doi: 10.1021/acs.analchem.6b00429
- Eivazi, S., Majidi, J., Aghebati Maleki, L., Abdolalizadeh, J., Yousefi, M., Ahmadi, M., … Zolali, E. (2015). Production and purification of a polyclonal antibody against purified mouse IgG2b in rabbits towards designing mouse monoclonal isotyping kits. Advanced Pharmaceutical Bulletin, 5(1), 109–113.
- Fan, W., Tang, Q., Shen, C., Qin, D., Lu, C., & Yan, Q. (2015). Preparation and characterization of polyclonal antibody against Kaposi’s sarcoma-associated herpesvirus lytic gene encoding RTA. Folia Microbiologica, 60(6), 473–481. doi: 10.1007/s12223-015-0387-x
- Gefen, T., Vaya, J., Khatib, S., Rapoport, I., Lupo, M., Barnea, E., … Pitcovski, J. (2015). The effect of haptens on protein-carrier immunogenicity. Immunology, 144(1), 116–126. doi: 10.1111/imm.12356
- Grenni, P., Ancona, V., & Barra Caracciolo, A. (2018). Ecological effects of antibiotics on natural ecosystems: A review. Microchemical Journal, 136, 25–39. doi: 10.1016/j.microc.2017.02.006
- He, J., Tao, X., Wang, K., Ding, G., Li, J., Li, Q. X., … Xu, T. (2019). One-step immunoassay for the insecticide carbaryl using a chicken single-chain variable fragment (scFv) fused to alkaline phosphatase. Analytical Biochemistry, 572, 9–15. doi: 10.1016/j.ab.2019.02.022
- Hu, S., Li, D., Huang, Z., Xing, K., Chen, Y., Peng, J., & Lai, W. (2018a). Ultra-sensitive method based on time-resolved fluorescence immunoassay for detection of sulfamethazine in raw milk. Food and Agricultural Immunology, 29(1), 1137–1149. doi: 10.1080/09540105.2018.1520816
- Hu, X., Yao, J., Wang, F., Yin, M., Sun, Y., Hu, M., … Zhang, G. (2018b). Eu(3+) -labeled IgG-based time-resolved fluoroimmunoassay for highly sensitive detection of aflatoxin B1 in feed. Journal of the Science of Food and Agriculture, 98(2), 674–680. doi: 10.1002/jsfa.8514
- Kavanagh, O., Elliott, C. T., & Campbell, K. (2015). Progress in the development of immunoanalytical methods incorporating recombinant antibodies to small molecular weight biotoxins. Analytical and Bioanalytical Chemistry, 407(10), 2749–2770. doi: 10.1007/s00216-015-8502-z
- Kong, D., Xie, Z., Liu, L., Song, S., Kuang, H., & Xu, C. (2017). Development of ic-ELISA and lateral-flow immunochromatographic assay strip for the detection of vancomycin in raw milk and animal feed. Food and Agricultural Immunology, 28(3), 414–426. doi: 10.1080/09540105.2017.1293014
- Lee, B.-L., Asakura, K., Azechi, T., Sasano, H., Matsui, H., Hanaki, H., … Yahara, K. (2018). Rapid and easy detection of low-level resistance to vancomycin in methicillin-resistant Staphylococcus aureus by matrix-assisted laser desorption ionization time-of-flight mass spectrometry. Plos One, 13(3), e0194212. doi: 10.1371/journal.pone.0194212
- Li, B., Yuan, Z., Hung, H.-C., Ma, J., Jain, P., Tsao, C., … Jiang, S. (2018). Revealing the immunogenic risk of polymers. Angewandte Chemie International Edition, 57(42), 13873–13876. doi: 10.1002/anie.201808615
- Li, M., Zhang, Y., Xue, Y., Hong, X., Cui, Y., Liu, Z., & Du, D. (2017). Simultaneous determination of β2-agonists clenbuterol and salbutamol in water and swine feed samples by dual-labeled time-resolved fluoroimmunoassay. Food Control, 73, 1039–1044. doi: 10.1016/j.foodcont.2016.10.019
- Liang, W., Liu, S., Liu, Z., Li, D., Wang, L., Hao, C., & He, Y. (2015). Electron transfer and fluorescence “turn-off” based CdTe quantum dots for vancomycin detection at nanogram level in aqueous serum media. New Journal of Chemistry, 39(6), 4774–4782. doi: 10.1039/C4NJ01764A
- Limbu, S. M., Zhou, L., Sun, S.-X., Zhang, M.-L., & Du, Z.-Y. (2018). Chronic exposure to low environmental concentrations and legal aquaculture doses of antibiotics cause systemic adverse effects in Nile tilapia and provoke differential human health risk. Environment International, 115, 205–219. doi: 10.1016/j.envint.2018.03.034
- Liu, Y., Lin, M., Zhang, X., Xu, C., Jiao, L., Zhong, J., … Liu, X. (2016). Applications of mutagenesis methods on affinity maturation of antibodies in vitro. Journal of Zhejiang University, 42(1), 1–7. in chinese.
- Liu, X., Steele, J. C., & Meng, X. Z. (2017). Usage, residue, and human health risk of antibiotics in Chinese aquaculture: A review. Environmental Pollution, 223, 161–169. doi: 10.1016/j.envpol.2017.01.003
- Liu, Y., Wu, A., Hu, J., Lin, M., Wen, M., Zhang, X., … Liu, X. (2015). Detection of 3-phenoxybenzoic acid in river water with a colloidal gold-based lateral flow immunoassay. Analytical Biochemistry, 483, 7–11. doi: 10.1016/j.ab.2015.04.022
- Liu, Z., Yan, X., Hua, X., & Wang, M. (2013). Time-resolved fluoroimmunoassay for quantitative determination of thiacloprid in agricultural samples. Analytical Methods, 5(14), 3572–3576. doi: 10.1039/c3ay00033h
- Lu, X., Jiang, D. J., Yan, J. X., Ma, Z. E., Luo, X. E., Wei, T. L., … He, Q. H. (2018). An ultrasensitive electrochemical immunosensor for Cry1Ab based on phage displayed peptides. Talanta, 179, 646–651. doi: 10.1016/j.talanta.2017.11.032
- Luo, L., Lei, H. T., Yang, J. Y., Liu, G. L., Sun, Y. M., Bai, W. D., … Xu, Z. L. (2017). Development of an indirect ELISA for the determination of ethyl carbamate in Chinese rice wine. Analytica Chimica Acta, 950, 162–169. doi: 10.1016/j.aca.2016.11.008
- Mehlhorn, A., Rahimi, P., & Joseph, Y. (2018). Aptamer-based biosensors for antibiotic detection: A review. Biosensors (Basel), 8(2).
- Ni, T., Peng, D., Wang, Y., Pan, Y., Xie, S., Chen, D., … Yuan, Z. (2019). Development of a broad-spectrum monoclonal antibody-based indirect competitive enzyme-linked immunosorbent assay for the multi-residue detection of avermectins in edible animal tissues and milk. Food Chemistry, 286, 234–240. doi: 10.1016/j.foodchem.2019.02.011
- Omara, S. T. (2017). MIC and MBC of honey and gold nanoparticles against methicillin-resistant (MRSA) and vancomycin-resistant (VRSA) coagulase-positive S. aureus isolated from contagious bovine clinical mastitis. Journal of Genetic Engineering and Biotechnology, 15(1), 219–230. doi: 10.1016/j.jgeb.2017.02.010
- Ruano-Gallego, D., Fraile, S., Gutierrez, C., & Fernandez, L. A. (2019). Screening and purification of nanobodies from E. coli culture supernatants using the hemolysin secretion system. Microbial Cell Factories, 18(1), 47. doi: 10.1186/s12934-019-1094-0
- Sheng, E., Shi, H., Zhou, L., Hua, X., Feng, L., Yu, T., & Wang, M. (2016). Dual-labeled time-resolved fluoroimmunoassay for simultaneous detection of clothianidin and diniconazole in agricultural samples. Food Chemistry, 192, 525–530. doi: 10.1016/j.foodchem.2015.07.023
- Sheng, Y., & Zhou, B. (2017). High-throughput determination of vancomycin in human plasma by a cost-effective system of two-dimensional liquid chromatography. Journal of Chromatography A, 1499, 48–56. doi: 10.1016/j.chroma.2017.02.061
- Shi, Q., Huang, J., Sun, Y., Yin, M., Hu, M., Hu, X., … Zhang, G. (2018). Utilization of a lateral flow colloidal gold immunoassay strip based on surface-enhanced Raman spectroscopy for ultrasensitive detection of antibiotics in milk. Spectrochimica Acta Part A: Molecular and Biomolecular Spectroscopy, 197, 107–113. doi: 10.1016/j.saa.2017.11.045
- Talib, N. A. A., Salam, F., & Sulaiman, Y. (2018). Development of polyclonal antibody against clenbuterol for immunoassay application. Molecules, 23, 4.
- Usman, M., & Hempel, G. (2016). Development and validation of an HPLC method for the determination of vancomycin in human plasma and its comparison with an immunoassay (PETINIA). Springerplus, 5, 124. doi: 10.1186/s40064-016-1778-4
- Wang, J., Peng, T., Zhang, X., Yao, K., Ke, Y., Shao, B., … Jiang, H. (2018). A novel hapten and monoclonal antibody-based indirect competitive ELISA for simultaneous analysis of alternariol and alternariol monomethyl ether in wheat. Food Control, 94, 65–70. doi: 10.1016/j.foodcont.2018.06.027
- Watanabe, E., Miyake, S., & Yogo, Y. (2013). Review of enzyme-linked immunosorbent assays (ELISAs) for analyses of neonicotinoid insecticides in agro-environments. Journal of Agricultural and Food Chemistry, 61(51), 12459–12472. doi: 10.1021/jf403801h
- Xu, C., Liu, X., Zhang, C., Zhang, X., Zhong, J., Liu, Y., … Liu, X. (2017). Establishment of a sensitive time-resolved fluoroimmunoassay for detection of Bacillus thuringiensis Cry1Ie toxin based nanobody from a phage display library. Analytical Biochemistry, 518, 53–59. doi: 10.1016/j.ab.2016.11.006
- Xu, C., Miao, W., He, Y., Zu, Y., Liu, X., Li, J., & Liu, X. (2019). Construction of an immunized rabbit phage display antibody library for screening microcystin-LR high sensitive single-chain antibody. International Journal of Biological Macromolecules, 123, 369–378. doi: 10.1016/j.ijbiomac.2018.11.122
- Xu, F., Ren, K., Yang, Y.-z., Guo, J.-p., Ma, G.-p., Liu, Y.-m., … Li, X.-b. (2015). Immunoassay of chemical contaminants in milk: A review. Journal of Integrative Agriculture, 14(11), 2282–2295. doi: 10.1016/S2095-3119(15)61121-2
- Xu, C., Yang, Y., Liu, L., Li, J., Liu, X., Zhang, X., … Liu, X. (2018). Microcystin-LR nanobody screening from an alpaca phage display nanobody library and its expression and application. Ecotoxicology and Environmental Safety, 151, 220–227. doi: 10.1016/j.ecoenv.2018.01.003
- Yang, S., Ouyang, H., Su, X., Gao, H., Kong, W., Wang, M., … Fu, Z. (2016). Dual-recognition detection of staphylococcus aureus using vancomycin-functionalized magnetic beads as concentration carriers. Biosensors and Bioelectronics, 78, 174–180. doi: 10.1016/j.bios.2015.11.041
- Yao, C.-Y., Xu, Z.-L., Wang, H., Zhu, F., Luo, L., Yang, J.-Y., … Shen, Y.-D. (2019). High affinity antibody based on a rationally designed hapten and development of a chemiluminescence enzyme immunoassay for quantification of alternariol in fruit juice, maize and flour. Food Chemistry, 283, 359–366. doi: 10.1016/j.foodchem.2018.12.127
- You, X., Li, Y., Li, B., & Ma, J. (2016). Gold nanoclusters-based chemiluminescence resonance energy transfer method for sensitive and label-free detection of trypsin. Talanta, 147, 63–68. doi: 10.1016/j.talanta.2015.09.033
- Zhang, X., He, K., Zhao, R., Wang, L., & Jin, Y. (2016). Cloning of scFv from hybridomas using a rational strategy: Application as a receptor to sensitive detection microcystin-LR in water. Chemosphere, 160, 230–236. doi: 10.1016/j.chemosphere.2016.06.084
- Zhang, Y., Li, X. Q., Li, H. M., Zhang, Q. H., Gao, Y., & Li, X. J. (2019). Antibiotic residues in honey: A review on analytical methods by liquid chromatography tandem mass spectrometry. TrAC Trends in Analytical Chemistry, 110, 344–356. doi: 10.1016/j.trac.2018.11.015
- Zhang, Z., Tang, X., Wang, D., Zhang, Q., Li, P., & Ding, X. (2015). Rapid on-site sensing aflatoxin B1 in food and feed via a chromatographic time-resolved fluoroimmunoassay. PLoS One, 10(4), e0123266. doi: 10.1371/journal.pone.0123266
- Zhao, Y., Liang, Y., Liu, Y., Zhang, X., Hu, X., Tu, S., … Tu, K. (2016). Isolation of broad-specificity domain antibody from phage library for development of pyrethroid immunoassay. Analytical Biochemistry, 502, 1–7. doi: 10.1016/j.ab.2016.02.020
- Zhou, B., Zhang, J., Fan, J., Zhu, L., Zhang, Y., Jin, J., & Huang, B. (2015). A new sensitive method for the detection of chloramphenicol in food using time-resolved fluoroimmunoassay. European Food Research and Technology, 240(3), 619–625. doi: 10.1007/s00217-014-2363-0