ABSTRACT
Aminophylline (AMP), a bronchodilator drug, is a modified form of theophylline (the pharmacological active ingredient). AMP is often given to treat respiratory diseases, including bronchial asthma, blocked emphysema, panting bronchitis, and cardiogenic asthma. In this study, we developed a rapid and sensitive method to determine AMP concentration in mouse serum. A sensitive monoclonal antibody against AMP was produced by immunizing BALB/c mice with a well-characterized AMP2-keyhole limpet hemocyanin and coating antigen (hapten-AMP1-OVA). The coating antigen and goat anti-mouse IgG antibody were sprayed onto a nitrocellulose membrane representing the test and control lines, respectively. Under optimized conditions, the cut-off limits of the test strip were 50 ng/mL in 0.01 M phosphate buffered saline (pH 7.4) and 250 ng/mL in serum. The results were obtained within 10 min and revealed that this immunochromatographic strip test is a sensitive, rapid, and simple tool for the detection of AMP in serum.
Introduction
Aminophylline (AMP, C16H24N10O4, ) is a bronchodilator compound composed of the theophylline with ethylenediamine added (ratio of 2:1) to increase bioavailability, and is given to treat respiratory diseases including asthma, panting bronchitis, and cardiogenic asthma (Gelvez et al., Citation2004; Shiohira, Fujii, Koizumi, Kondoh, & Watanabe, Citation2009). AMP is also used as an ingredient of skin cream products but can allergic reactions due to the ethylenediamine component (Wang & Wang, Citation2006). AMP quickly releases theophylline in buffer solutions (pH 7.4) and blood samples, and when the dose is greater than 20 µg/mL, it can occasionally produce serious toxicity issues, including nausea, emesis, and central nervous system excitation (Kesavan & John, Citation2014; Peng, Wang, Xiao, Wang, & Ding, Citation2016). In order to avoid this situation, it is essential to develop an easy and sensitive analytical method capable of detecting this drug.
Numerous methods, such as high performance liquid chromatography (Tang, Wei, & Yin, Citation2003; Yin et al., Citation2016), spectroscopy (De Fabrizio, Citation1977; Mazurek & Szostak, Citation2006), spectrophotometry (Li & Zhang, Citation2008), electrochemical analysis kit for quality control (Guedes & Pio dos Santos, Citation2018), small-sized capillary electrophoresis with amperometry (Ge et al., Citation2013), CdTe quantum dots as fluorescence sensor (Wang, Wu, Wang, Wang, & Dou, Citation2013), chemiluminescence (Rezaei, Ensafi, & Zarei, Citation2012), and liquid chromatography tandem mass spectrometry (Babu et al., Citation2011) have been used to measure AMP or theophylline levels. All of the above methods require skilled operators. Because of the technical nature of instrumentation, they also have relatively high running costs, long clean up procedures, and are time consuming (Khaemba et al., Citation2016; Liu, Xu, Suryoprabowo, Song, & Kuang, Citation2018; Shen et al., Citation2019; Xu, Liu, Cui, Wu, & Kuang, Citation2019).
Recently, immunoassays have been widely used as screening methods for a variety of applications and a fluoroimmunosensor for theophylline using immobilized antibody (Garcinuno, Fernandez, Perez-Conde, Gutierrez, & Camara, Citation2000), microdevice for facile analysis of theophylline in whole blood by a cloned enzyme donor immunoassay (Nishiyama, Sugiura, Kaji, Tokeshi, & Baba, Citation2019), colorimetric theophylline aggregation assay using an RNA aptamer and non-crosslinking gold nanoparticles (Ma, Guo, Mao, Tang, & Miao, Citation2018), and a novel molecularly for the ultra-high sensitive detection of theophylline using mesoporous silica nanospheres as the signal (Gan et al., Citation2018) has also been developed. However, if a large number of samples are to be analyzed, enzyme-linked immunosorbent assays are useful method because of their high specificity, uncomplicated sample preparation, and low cost (Ge, Suryoprabowo, Xu, Zheng, & Kuang, Citation2018; Mukunzi, Isanga, Suryoprabowo, Liu, & Kuang, Citation2017; Mukunzi, Suryoprabowo, Song, Liu, & Kuang, Citation2018; Suryoprabowo, Liu, Peng, Kuang, & Xu, Citation2015). However, it has disadvantages such as a long incubation times for the enzyme–substrate reaction. Therefore, it is not suitable for on-site detection. Immunochromatographic or lateral flow immunoassays (LFIA) are increasingly popular as screening methods for the detection of analytes because they are simple, rapid, specific, and sensitive to use. Disposable liposome immunosensor for theophylline combining with immunochromatographic membrane and a thick-film electrode (Lee, Kim, Shin, Lee, & Park, Citation1999) have been developed The major advantage of using LFIA is that all the reagents are sprayed onto the strip and results can be obtained within 5-10 min (Jiang, Zeng, Liu, Song, & Kuang, Citation2018; Song et al., Citation2019; Li, Liu, Song, & Kuan, Citation2018). The aim of this study was to develop a LFIA for the detection of AMP in mice blood.
Materials and methods
Aminophylline was purchased from J&K Scientific (Shanghai, China). Complete Freund’s adjuvant (FCA), incomplete Freund’s adjuvant (FIA), and enzyme immunoassay-grade horseradish peroxidase-labeled goat anti-mouse immunoglobulin were obtained from Sigma-Aldrich (St. Louis, MO, USA). Gelatin was supplied by Beijing Biodee Biotechnology Co., Ltd. (Beijing, China). Tetramethylbenzidine and horseradish peroxidase were acquired from Aladdin Chemistry Co., Ltd. (Shanghai, China). All reagents used for cell fusion were purchased from Sunshine Biotechnology Co., Ltd. (Nanjing, China). Ovalbumin (OVA) and keyhole-limpet hemocyanin (KLH) were obtained from Solarbio Science & Technology, Co, Ltd. (Beijing, China). All other reagents and chemicals were supplied by the National Pharmaceutical Group Chemical Reagent Co, Ltd. (Shanghai, China).
Nitrocellulose (NC) high-flow plus membranes (Pura-bind RP) were obtained from Whatman-Xinhua Filter Paper Co., Ltd. (Hangzhou, China). Glass fiber membranes (CB-SB08) used for the sample pad, polyvinylchloride (PVC) backing materials and absorbance pads (SX18) were supplied by Goldbio Tech Co., Ltd. (Shanghai, China). The conjugated coating antigens (hapten-AMP (HAMP1-OVA)) and specific monoclonal antibodies (anti-AMP mAb, No. 1G5) were generated in our laboratory.
All buffer solutions were prepared using ultrapure water (Milli-Q purification system, Millipore Co., Bedford, MA, USA). The strip cutting instrument was a CM 4000 guillotine cutting system (Gene, Shanghai, China), and the dispensers were an Airjet Quanti 3000TM and a Biojet Quanti 3000TM (XinqidianGene Technology Co., Ltd., (Beijing, China)).
Preparation and characterization of anti-AMP monoclonal antibody
Hapten-AMP2 (AMP2, ) was conjugated to KLH () using the using the ester method. Briefly, HAMP2 (20 mg) was dissolved in N,N-dimethyl formamide (DMF, 500 µL), and was added to carboxyl-reactive carbodiimide cross linker (1-ethyl-3-[3-dimethylaminopropyl]carbodiimide hydrochloride, EDC, 24 mg) and N-hydroxysuccinimide (NHS, 16 mg), which were diluted with 2-morpholinoethanesulfonic acid (0.01 M of MES, 200 µL). This represented solution 1, which was stirred for 6 h at room temperature. KLH was dissolved in 0.05 M carbonate–bicarbonate buffer (pH 9.6; 5 mL). Solution 1 was added to the protein solution and stirred for 12 h in the dark. The conjugate was dialyzed against 0.01 M PBS for 3 days and then distilled water for a further 3 days. The conjugation of protein and AMP was assessed by UV absorption and the antigen was stored at −20 oC until use.
Figure 2. (a) Chemical structure of theophylline-7-acetic acid (HAMP1); (b) The procedure for the synthesis of the hapten-AMP2 (HAMP2).
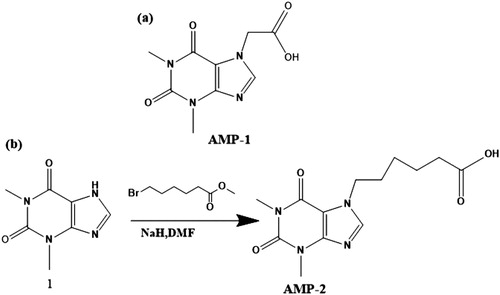
Hapten-AMP1 (theophylline-7-acetic acid, AMP1, ) was conjugated to OVA (). Briefly, HAMP1 (20 mg) was dissolved in DMF (500 µL), and stirred with the addition of N,N'-dicyclohexylcarbodiimide (DCC, 24 mg) and N-hydroxysuccinimide (NHS, 16 mg) and diluted with 2-morpholinoethanesulfonic acid (0.01 M of MES, 200 µL, solution 1). Following this, the solution was further stirred for 6 h at room temperature. OVA was dissolved in 0.05 M carbonate–bicarbonate buffer (pH 9.6; 5 mL). Solution 1 was added to the protein solution and stirred for 12 h in the dark. The conjugate was dialyzed against 0.01 M PBS for 3 days and then subsequently against distilled water for a further 3 days. The conjugation of protein and AMP was assessed by UV absorption. The antigen was stored at −20 oC until use.
Female BALB/c mice (6-8 weeks of age) were used to produce anti-AMP monoclonal antibody (mAb). The mice were immunized subcutaneously with HAMP2-KLH. FCA was used in the first immunization, and FIA was used in subsequent immunization. The mice were immunized every 3 weeks, using 100 µg for the first immunization and 50 µg for the remainder (2 - 5 times). Blood samples from the mice were analyzed by ELISA. The mouse with the highest titer was sacrificed and its splenocytes were fused with Sp 2/0 murine myeloma cells. The hybridomas were screened by indirect ELISA. The selected hybridoma cells were expanded and injected into BALB/c mice to produce mAbs (Deng et al., Citation2012; Suryoprabowo, Liu, Peng, Kuang, & Xu, Citation2014). The ascites were harvested and purified using the caprylic acid-ammonium sulfate precipitation method (Kong et al., Citation2017; Wang et al., Citation2017). The purified antibody solution was stored at −20 oC until further use.
Development of the lateral-flow immunochromatographic test strip device
Preparation of colloidal gold nanoparticles (GNP)
All solvents were prepared with ultrapure water and filtered through a transfer membrane (0.22 µm). Chloroauric acid (25 mL of a 0.1 g/L solution) was boiled with constant stirring, and then 1% (w/v) sodium citrate tribasic dihydrate solution (1.0 mL) was added. The mixture was stirred for 30 min until the color turned wine-red, then cooled to room temperature, and stored at 4 °C. Transmission electron microscopy (TEM) demonstrated that the gold nanoparticles were of uniform particle size (15 nm).
Preparation of colloidal gold-labeled mAb
The anti-AMP mAb (1G5) which had a half-maximal inhibitory concentration (IC50) of 5.80 ng/mL was used to prepare colloidal gold-labelled mAbs. Colloidal gold solution (10 mL) was adjusted to pH 7.0 with 0.1 M K2CO3 (40 µL). Briefly, 0.5 mL of 0.2 mg/mL mAb solution was added dropwise to the colloidal gold solution. After 35 min, 0.5 mL of 10% (w/v) bovine serum albumin (BSA) was added, and the mixture was stirred for two hours. The product was centrifuged for 45 min at 8000 rpm to remove residual gold aggregates. The solution was then separated into two layers, and the lower layer (red gold-labeled mAb) was collected. The conjugation product was reconstituted in 1 mL of gold-labeling resuspension buffer (0.02 M PBS, 5% sucrose, 2% sorbitol, 1% mannitol, 0.1% PEG, 0.1% Tween, and 0.04% NaN3) and stored at 4 oC until required.
Preparation of the NC capture membranes
The coating antigen (AMP1-OVA) and goat anti-mouse IgG antibody (0.2 mg/mL), were sprayed onto an NC membrane at a volume of 0.9 µL/cm, resulting in the formation of the test and control lines. Capture and control reagents were sprayed onto the glass fiber membrane to prepare the conjugate pad, which was dried for two hours at 37 oC. The NC membrane containing the capture reagents was pasted onto the center of the plastic backing plate. Polyvinylchloride (PVC) and the conjugate pad, sample pad, and absorbent pad were laminated and pasted onto the plate. Finally, the plate was cut into strips (2.9 mm wide) with a strip cutter.
Test procedure and principle
The gold labeled mAb (50 µL) was mixed with 150 µL of sample solution, allowed to react for 5 min, and then applied to the sample pad. The solution migrated along the absorbent pad, and the test results were obtained within 5 min. Procaine, if present in the sample, would compete with the AMP1-OVA conjugates embedded in the test line, for the finite amount of anti-AMP mAb in the sample. When a detectable level of AMP was present in the sample, the free AMP bound to the gold-labeled mAb, prevented the mAb binding to the AMP1-OVA in the test line. Therefore, the higher the level of AMP present in the sample, the weaker the colour of the test line. If no AMP is present, the limited amount of colloidal-gold-labeled mAb would be trapped by the immobilized HAMP1-OVA conjugate, and a visible red test line would appear.
When the sample flow reached the control line, containing the goat anti-mouse IgG antibody, it produced a visible reaction. Therefore, the control line will always appear in a successful test, whereas the test line will only appear when the sample is negative. The appearance of the control line alone is a positive result. If, however, no line appears, the test procedure was performed incorrectly, or the strip was invalid, indicating the test should be repeated with a new strip.
Determination of performance
Sensitivity of the test strip
The sensitivity of the test strip was determined by testing different AMP samples. A top concentration of AMP was diluted to produce a range of standard concentrations (0, 1, 2.5, 5, 10, 25, and 50 ng/mL) in 0.01 M PBS (pH 7.4), and the detection limit was determined. A sample solution (150 µL) was mixed with 50 µL of gold labelled mAb, allowed to react for 5 min, and added to the sample pad. After 5 min, the test strip reader recorded the colour intensities of the different strips. The lowest detection limit (LDL) detectable with the naked eye was defined as the concentration of AMP that resulted in a strip colour that was clearly different in intensity from the result obtained using 0 ng/mL AMP. Six replicate samples for each concentration were analyzed on the same day.
Detection of AMP in mouse serum
A simple pretreatment was used to determine AMP in mouse serum, which involved diluting blood 100 fold in extraction buffer (0.01M PBS, pH 7.4). The samples were then spiked with an AMP standard solution (10 µg/mL, prepared with 0.01 M PBS, pH 7.4). The AMP concentrations were 0.1, 0.25, 0.5, 1, and 2.5 ng/mL. A blank (unspiked) sample was used as the control and six replicates for each concentration were analyzed using the test strips.
Results and discussion
Optimization of the LFIA
The concentration of coating antigen and GNP-mAbs may affect the sensitivity of the LFAI. Therefore, strips with different concentrations of coating antigen (0.05 and 0.1 mg/mL) sprayed onto the test line were evaluated with 0.01 M PBS (pH 7.4) containing either 0 or 50 ng/mL AMP. As shown on , both control and test lines resulted in colour development. There are significant differences in colour intensity between 0.05 and 0.1 mg/mL of coating antigen concentration when viewed by the naked eyes. A coating concentration 0.1 mg/mL has greater colour intensity than 0.05 mg/mL, but significant different on colour intensity of the T line on positive results between two concentration, suggesting the a more sensitive detection could be achieved at 0.05 mg/mL. Therefore, 0.05 mg/mL coating antigen was selected for the preparation of the test line.
Figure 5. Optimization of the immunochromatographic strip. Concentration of coating antigen (A) 0.05 mg/mL; (B) 0.1 mg/mL. The dosage of the mAb that add in GNP: (1) 8 µg/L; (2) 10 µg/L. The standard concentration: (a) 0 ng/mL; (b) 50 ng/mL.
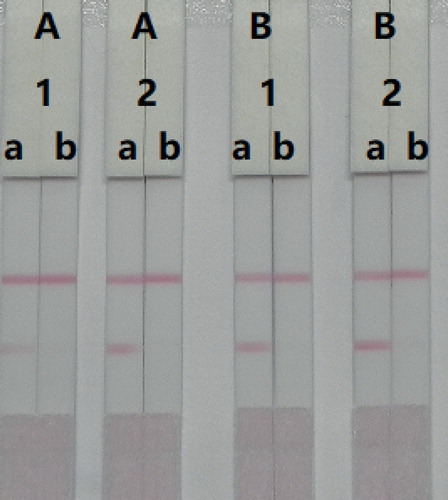
The amount of antibody that is added to the GNPs also affects the sensitivity of the LFIA. The reaction system which contained different concentrations of mAb (8 or 10 µg/L), was allowed to react with AMP-negative (0 ng/mL) and AMP-positive (50 ng/mL) samples. shows that there were significant differences in colour intensity between 8 and 10 µg/L mAb. We observed that the optimum concentration of mAb using GNPs was 10 µg/L of colloidal gold, as it contributed to strong colour intensities on both lines with the negative sample and was more sensitive at detecting the positive sample. Therefore, the optimal system for our developed immunochromatographic test strip consisted of 0.05 mg/mL coating antigen and 10 µg/L of mAb in GNP.
Figure 6. Colloidal gold immunochromatographic for AMP in 0.01 M PBS (pH 7.4). AMP concentration: 1 = 0 ng/mL; 2 = 1 ng/mL; 3 = 2.5 ng/mL; 4 = 5 ng/mL; 5 = 10 ng/mL; 6 = 25 ng/mL; and 7 = 50 ng/mL.
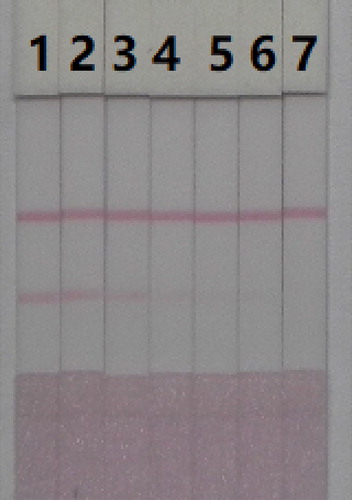
The sensitivity of the assay was investigated using a series of AMP standards diluted in 0.01 M PBS (pH 7.4). The LDL obtained by eye was defined as the amount of AMP that produced a colour reaction on the strip that was clearly different in intensity from the AMP-negative samples. shows that the colour intensity on the test line changed from strong (0 ng/mL) to weak and finally disappeared completely at 50 ng/mL.
Detection of AMP in mouse serum
In this study, a simple pre-treatment was used; mouse serum was dilute 100 fold in 0.01 M PBS (pH 7.4). We also optimize the concentration of mAbs within the GNPs and coating antigen to determine concentrations of AMP in mouse serum. Two different concentrations of mAbs (8 or 10 µg/mL) were allowed to react with AMP-negative (0 ng/mL) and AMP-positive (1 ng/mL) samples. As shown in , there were significant differences in colour intensity, between the 8 and 10 µg/mL concentrations. We observed the optimum concentration of mAb use with the GNPs was 10 µg/mL. There were also significant differences in colour intensity between the coating antigen concentrations at 0.05 and 0.1 mg/mL. We found that concentrations of coating antigen at 0.1 mg/mL had greater colour intensity than 0.05 mg/mL, suggesting that a more sensitive detection could be achieved at 0.1 mg/mL. Therefore, the optimum conditions for AMP determination in mouse serum were 0.1 mg/mL of coating antigen and 10 µg/mL of mAb for the GNPs.
Figure 7. Optimization of the immunochromatographic strip. Concentration of coating antigen (A) 0.05 mg/mL; (B) 0.1 mg/mL. The dosage of the mAb that add in GNP: (1) 8 µg/L; (2) 10 µg/L. The standard concentration: (a) 0 ng/mL; (b) 100 ng/mL.
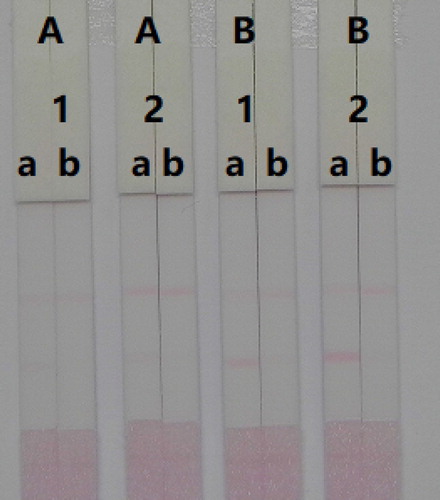
The advantages using immunochromatographic assay is its rapidity and ease of use. The mouse serum was spiked with AMP standards (10 µg/mL, prepared with 0.01 M PBS pH 7.4) at final concentrations of 10, 25, 50, 100, and 250 ng/mL. Under optimized conditions (), the colour intensity decreased as the AMP concentration increased. The colour signal changed from strong to weak with increasing concentration of AMP and disappeared completely at 250 ng/mL.
Conclusions
We have developed a sensitive anti-AMP mAb and immunogen (AMP1-OVA). An immunochromatographic strip test was developed as a sensitive, simple, and rapid analytical method for the detection of AMP in mouse serum. Under optimized conditions, the results demonstrated that the cut-off limits for the detection AMP were as low as 50 ng/mL in 0.01 M PBS (pH 7.4) and 250 ng/mL in mouse serum. The AMP strip test can be completed within 10 min in only one step, and therefore, represents a simple and rapid procedure.
Acknowledgements
This work is financially supported the grants from Natural Science Foundation of China and MOF (2016YFD0401101, CMB21S1614, CLE02N1515).
Disclosure statement
No potential conflict of interest was reported by the authors.
Additional information
Funding
References
- Babu, A. R. S., Thippeswamy, B., Vinod, A. B., Ramakishore, E. G., Anand, S., & Senthil, D. (2011). Determination of theophylline in rabbit plasma by triple quadrupole LC/MS. Pharmaceutical Methods, 2(4), 211–217.
- De Fabrizio, F. (1977). UV spectrophotometric determination of aminophylline, amobarbital, and ephedrine hydrochloride in an antiasthma capsule preparation. Journal of Pharmaceutical Sciences, 66(6), 811–813.
- Deng, X., Liu, L., Ma, W., Xu, C., Wang, L., & Kuang, H. (2012). Development and validation of a sandwich ELISA for quantification of peanut agglutinin (PNA) in foods. Food and Agricultural Immunology, 23(3), 265–272.
- Gan, T., Li, J., Zhao, A., Xu, J., Zheng, D., Wang, H., & Li, Y. (2018). Detection of theophylline using molecularly imprinted mesoporous silica spheres. Food Chemistry, 268, 1–8.
- Garcinuno, R. M., Fernandez, P., Perez-Conde, C., Gutierrez, A. M., & Camara, C. (2000). Development of a fluoroimmunosensor for theophylline using immobilised antibody. Talanta, 52(5), 825–832.
- Ge, W., Suryoprabowo, S., Xu, L., Zheng, Q., & Kuang, H. (2018). Rapid detection of penbutolol in pig urine using an immunochromatographic test strip. Food and Agricultural Immunology, 29(1), 1126–1136.
- Ge, J., Tong, F., Li, Y., Zhang, Y., Chu, Q., & Ye, J. (2013). Fast determination of Ethylendiamine in aminophylline tablets by small-sized capillary electrophoresis with Amperometric detection. Chinese Journal of Chemistry, 31(4), 525–528.
- Gelvez, J., Fakioglu, H., Olarte, J. L., Soliz, A., Totapally, B. R., & Torbati, D. (2004). Effect of aerosolized milrinone during drug-induced pulmonary hypertension in lambs. Pharmacological Research, 50(1), 87–91.
- Guedes, T. d. J., & Pio dos Santos, W. T. (2018). Fast and simple electrochemical analysis kit for quality control of narrow therapeutic index drugs. Electroanalysis, 30(8), 1732–1741.
- Jiang, W., Zeng, L., Liu, L., Song, S., & Kuang, H. (2018). Immunochromatographic strip for rapid detection of phenylethanolamine A. Food and Agricultural Immunology, 29(1), 182–192.
- Kesavan, S., & John, S. A. (2014). Fabrication of aminotriazole grafted gold nanoparticles films on glassy carbon electrode and its application towards the simultaneous determination of theophylline and uric acid. Sensors and Actuators B-Chemical, 205, 352–362.
- Khaemba, G. W., Tochi, B. N., Mukunzi, D., Joel, I., Guo, L., Suryobrobowo, S., … Xu, C. (2016). Development of monoclonal antibody and lateral test strip for sensitive detection of clenbuterol and related beta(2)-agonists in urine samples. Food and Agricultural Immunology, 27(1), 111–127.
- Kong, D., Xie, Z., Liu, L., Song, S., Kuang, H., Cui, G., & Xu, C. (2017). Development of indirect competitive ELISA and lateral-flow immunochromatographic assay strip for the detection of sterigmatocystin in cereal products. Food and Agricultural Immunology, 28(2), 260–273.
- Lee, K. S., Kim, T. H., Shin, M. C., Lee, W. Y., & Park, J. K. (1999). Disposable liposome immunosensor for theophylline combining an immunochromatographic membrane and a thick-film electrode. Analytica Chimica Acta, 380(1), 17–26.
- Li, Y., Liu, L., Song, S., & Kuan, H. (2018). Development of a gold nanoparticle immunochromatographic assay for the on-site analysis of 6-benzylaminopurine residues in bean sprouts. Food and Agricultural Immunology, 29(1), 14–26.
- Li, Q., & Zhang, H. (2008). A novel spectrophotometric method for the determination of aminophylline in pharmaceutical samples in the presence of methanol. Spectrochimica Acta Part a-Molecular and Biomolecular Spectroscopy, 70(2), 284–289.
- Liu, L., Xu, L., Suryoprabowo, S., Song, S., & Kuang, H. (2018). Rapid detection of tulathromycin in pure milk and honey with an immunochromatographic test strip. Food and Agricultural Immunology, 29(1), 358–368.
- Ma, X., Guo, Z., Mao, Z., Tang, Y., & Miao, P. (2018). Colorimetric theophylline aggregation assay using an RNA aptamer and non-crosslinking gold nanoparticles. Microchimica Acta, 185(1), 33.
- Mazurek, S., & Szostak, R. (2006). Quantitative determination of diclofenac sodium and aminophylline in injection solutions by FT-Raman spectroscopy. Journal of Pharmaceutical and Biomedical Analysis, 40(5), 1235–1242.
- Mukunzi, D., Isanga, J., Suryoprabowo, S., Liu, L., & Kuang, H. (2017). Rapid and sensitive immunoassays for the detection of lomefloxacin and related drug residues in bovine milk samples. Food and Agricultural Immunology, 28(4), 599–611.
- Mukunzi, D., Suryoprabowo, S., Song, S., Liu, L., & Kuang, H. (2018). Development of an indirect enzyme-linked immunosorbent assay and lateral-flow test strips for pefloxacin and its analogues in chicken muscle samples. Food and Agricultural Immunology, 29(1), 484–497.
- Nishiyama, K., Sugiura, K., Kaji, N., Tokeshi, M., & Baba, Y. (2019). Development of a microdevice for facile analysis of theophylline in whole blood by a cloned enzyme donor immunoassay. Lab on a Chip, 19(2), 233–240.
- Peng, A., Wang, Y., Xiao, J., Wang, S., & Ding, H. (2016). Development of a novel and simple method for clinical therapeutic drug monitoring of aminophylline in humans based on a MWNTs-SiO2/Au composite modified screen-printed electrode. Analytical Methods, 8(5), 1069–1077.
- Rezaei, B., Ensafi, A. A., & Zarei, L. (2012). Fast and sensitive chemiluminescence assay of aminophylline in human serum using luminol-diperiodatoargentate(III) system catalyzed by coated iron nanoparticles. Spectrochimica Acta Part a-Molecular and Biomolecular Spectroscopy, 90, 223–229.
- Shen, X., Liu, L., Xu, L., Ma, W., Wu, X., Cui, G., & Kuang, H. (2019). Rapid detection of praziquantel using monoclonal antibody-based ic-ELISA and immunochromatographic strips. Food and Agricultural Immunology, 30(1), 913–923.
- Shiohira, H., Fujii, M., Koizumi, N., Kondoh, M., & Watanabe, Y. (2009). Novel chronotherapeutic rectal aminophylline delivery system for therapy of asthma. International Journal of Pharmaceutics, 379(1), 119–124.
- Song, S., Suryoprabowo, S., Liu, L., Zheng, Q., Wu, X., & Kuang, H. (2019). Development of an immunochromatographic strip test for rapid detection of sodium nifurstyrenate in fish. Food and Agricultural Immunology, 30(1), 236–247.
- Suryoprabowo, S., Liu, L., Peng, J., Kuang, H., & Xu, C. (2015). Antibody for the development of specific immunoassays to detect nadifloxacin in chicken muscles. Food and Agricultural Immunology, 26(3), 317–324.
- Suryoprabowo, S., Liu, L. Q., Peng, J., Kuang, H., & Xu, C. L. (2014). Development of a broad specific monoclonal antibody for fluoroquinolone analysis. Food Analytical Methods, 7(10), 2163–2168.
- Tang, C., Wei, X. L., & Yin, C. H. (2003). Analysis of ginkgolides and bilobalide in Ginkgo biloba L. Extract injections by high-performance liquid chromatography with evaporative light scattering detection. Journal of Pharmaceutical and Biomedical Analysis, 33(4), 811–817.
- Wang, W., Liu, L., Song, S., Xu, L., Zhu, J., & Kuang, H. (2017). Gold nanoparticle-based paper sensor for multiple detection of 12 Listeria spp. By P60-mediated monoclonal antibody. Food and Agricultural Immunology, 28(2), 274–287.
- Wang, L. H., & Wang, C. C. (2006). Electrochemical oxidation of aminophylline at film platinum electrodes, and its determination in cosmetic and pharmaceutical products. Microchimica Acta, 153(1-2), 95–100.
- Wang, R.-Y., Wu, J., Wang, L.-J., Wang, R., & Dou, H.-J. (2013). Cdte quantum dots as fluorescence sensor for the determination of aminophylline in aqueous solution. Optics and Spectroscopy, 115(4), 596–600.
- Xu, X., Liu, L., Cui, G., Wu, X., & Kuang, H. (2019). Development of an immunochromatography assay for salinomycin and methyl salinomycin in honey. Food and Agricultural Immunology, 30(1), 995–1006.
- Yin, B., Li, J., Wang, S.-L., Yan, S.-Y., Sui, C., Wan, S.-J., … Wan, R.-Z. (2016). Studies on pharmacokinetics and bioavailability of aminophylline in partridge chickens. European Journal of Drug Metabolism and Pharmacokinetics, 41(1), 19–25.