ABSTRACT
Tricaine (TMS) is usually used as an anesthetic in the transportation of aquatic animals. However, when consumed, the numerous residual drug residues found in fish can cause a high risk to human health. Therefore, it is important to develop a rapid and sensitive method for the detection of this drug in food. Here we have developed a novel lateral-flow immunochromatographic strip (LF-ICS) method for the detection of TMS in fish samples, using a very effective anti-TMS monoclonal antibody with a half-maximal inhibitory concentration value of 31.79 ng/mL. After the K2CO3 and coating antigen concentrations were optimized in phosphate buffered saline (pH 7.4), the LF-ICS was used to determine TMS levels in fish samples spiked with varying concentrations of the drug. Following this, the values for the visual limit of detection and the cut-off for TMS were found to be less than 0.1 and 1 μg/mL, respectively. These results indicated that our developed LF-ICS is applicable for on-site screening of TMS in fish samples.
Introduction
Tricaine methanesulfonate (TMS), 3-Aminobenzoic acid ethyl ester methanesulfonate, also known as MS-222, finquel or metacaine, is a synthetic ester-type anesthetic with excellent water solubility (). It is commonly used in the fisheries industry and can keep the organism in a state of low energy consumption, low sensory perception and slowed metabolism, by acting on central and peripheral nervous systems (Carter et al., Citation2011; Su et al., Citation2015). TMS is an ideal anesthetic, as it has a rapid effect and allows the fish to recover quickly (Cakir & Strauch, Citation2005; Cho & Heath, Citation2000). The drug is widely used in the form of an aqueous solution to sedate fish for handling or transportation, which can effectively reduce casualties and ensure freshness of the fish. Many researchers also use TMS to immobilize fish during invasive surgical procedures (Küçük, Citation2010; Palmer & Mensinger, Citation2004; Rombough, Citation2007).
Despite the outstanding advantages of TMS as an aquatic anesthetic, the drug residues it produces in fish destined for human consumption can produce detrimental effects to human health, including endocrine disorders, allergic responses, and altered cardiovascular function (Carter et al., Citation2011; Vera et al., Citation2010). Guidelines and regulations for TMS use vary among countries, for example, TMS is not approved by France, Greece, Spain, or China for public health reason (Su et al., Citation2015; Topic Popovic et al., Citation2012), but is allowed as an anesthetic for use in certain types of fish in the United States and in several other countries (Carter et al., Citation2011). In addition, the fish anesthetized by TMS are usually required to undergo a withdrawal period before slaughtering, which is 5, 10, and 21 days in Canada, New Zealand, and the United States, respectively (Topic Popovic et al., Citation2012). In order to reduce the harm of TMS residues to humans, it is vital to develop an effective method for monitoring the drug in fish. Previous research looking at the detection of TMS has used various methodologies including liquid chromatography (Nochetto et al., Citation2009), high performance liquid chromatography-tandem mass spectrometry (Li et al., Citation2014; Scherpenisse & Bergwerff, Citation2007; Xie et al., Citation2019), and liquid chromatography-tandem mass spectrometry (Zhao et al., Citation2017), with their advantage of accuracy and high sensitivity. However, these methods are not suitable for the detection of TMS in multiple samples because they are time consuming are of low-throughput, require complicated sample pre-treatment processes and are expensive. Therefore, the development of a rapid and sensitive method for the analysis of TMS is of great importance.
In recent years, immunoassays based on specific binding of antigen and antibody have been widely used for the analysis of harmful substances in food due to their obvious advantages as they are rapid, inexpensive, and simple to use (Ahmed et al., Citation2020; Wang et al., Citation2017b). The lateral-flow immunochromatographic strip (LF-ICS), is a novel detection method combining nanoparticles and enzyme-linked immunosorbent assay (ELISA) and is simpler and faster than ELISAs. To date, it has been successfully applied to the detection of several anesthetics, such as procaine (Hao et al., Citation2018), morphine (Chen et al., Citation2019), and eugenol (Shen et al., Citation2019). To the best of our knowledge, however, there are no studies using immunoassay methodologies to detect TMS residues.
In the present study, an anti-TMS monoclonal antibody (mAb) with high sensitivity and specificity was prepared, and then a LF-ICS method was developed based on the mAb for the detection of TMS in fish.
Materials and methods
Chemicals
TMS and 3-Aminobenzoic acid (TMS-1) were obtained from J&K Scientific Ltd (Beijing, China). Enzyme immunoassay-grade horseradish peroxidase-labelled goat anti-mouse immunoglobulin was purchase from Kangcheng Bioengineering Co. (Shanghai, China). Incomplete Freund’s adjuvant, complete Freund’s adjuvant, Keyhole limpet hemocyanin (KLH; MW 4,500,000 Da), ovalbumin (OVA; MW 45,000 Da), bovine serum albumin (BSA; MW 67,000 Da), 3, 3′, 5, 5′-tetramethylbenzidine (TMB), Tween-20, gelatin, Tributylamine, and Isobutyl chloroformate were purchased from Sigm-Aldrich (St. Louis, MO, USA). Life Technologies Corporation (Shanghai, China) supplied all cell culture reagents (e.g. polyethylene glycol solution, 1640 cell culture medium, fetal bovine serum, hypoxanthine aminopterin thymidine supplement, and hypoxanthine thymidine supplement).
The LF-ICS materials, including the glass fibre membrane acting as the sample pad, the polyvinylchloride plate serving as backing support material, and H5076 filter paper used for the absorbent pad were all supplied by Goldbio Tech Co., Ltd. (Shanghai, China). Nitrocellulose (NC) membranes used to hold the antigen and anti-IgG antibody were provided by Whatman-Xinhua Filter Paper Co., Ltd. (Hangzhou, China). In addition, the coating antigens linked with ovalbumin (OVA; TMS-1-OVA) and specific nanogold-labelled antibody (anti-TMS) were prepared in our laboratory.
Solutions
For the preparation of phosphate buffered saline (PBS), 8 g of NaCl, 0.2 g of KCl, 1.44 g of Na2HPO4, and 0.24 g of NaH2PO4 were dissolved in purified water to make 1L (PBS, 0.01 M, pH 7.4). The washing buffer solution was made by adding 0.05% Tween-20 (v/v) to PBS (0.01 M, pH 7.4). Next 1.59 g of Na2CO3 and 2.93 g of NaHCO3 were dissolved into 1 L of purified water to make the coating buffer solution (CBS, 0.05 M, pH 9.6). The substrate solution consisted of 80% solution A (citric acid, Na2HPO4, and H2O2) and 20% solution B (0.06% v/v TMB in glycol). The stop solution was 2 M sulfuric acid and the blocking buffer was CBS (0.05 M, pH 9.6) containing 2% gelatin. Ultrapure water was generated from a Milli-Q Purification System (Millipore Co., Bedford, MA, USA).
Preparation of immunogen and coating antigen
Immunogen TMS-1-KLH
To prepare TMS-1-KLH, hapten of 3-aminobenzoic acid (TMS-1, ) was selected to bind to KLH using the dicyclohexylcarbodiimide (DCC) method (Kong et al., Citation2017; Wang et al., Citation2017a). Specifically, 6 g of TMS-1 was dissolved in 0.6 mL of N,N-dimethylformamide (DMF), and then 20 mg of N-Hydroxysuccinimide and 36 mg of DCC were added. After mixing at room temperature for 4 h, the solution was added dropwise into 10 mg of KLH solution diluted with an equal volume of CBS (0.05 M, pH 9.6), and the solution maintained at pH 9.0 with 0.5 M NaOH. The solution was continuously stirred at room temperature for 24 h, and then dialyzed against 0.01 M PBS for 3 days (The PBS was changed every 8 h). Finally, the conjugate was confirmed using a UV scanner.
Coating antigen TMS-1-OVA
The synthesis of the coating antigen TMS-1-OVA was performed using the mixed-anhydride method as described in our previous study (Lei et al., Citation2018b). Briefly, 4 mg of TMS-1 was dissolved in 0.4 mL of DMF, then 8.3 μL of tributylamine and 4.5 μL of isobutyl-chloroformate were added at intervals of 15 min. After stirring for 1 h, the solution was added dropwise to 7 mg OVA dissolved in 1.4 mL of 0.05 M CBS and the reaction was left for 6 h. All of the above reactions were performed at 4°C and the solution was dialyzed and confirmed as described earlier.
Preparation of mAb against TMS
BALB/c mice (females, aged 6–8 weeks) were immunized with the immunogen TMS-1-KLH by subcutaneous multisite injections, and specific immunization methods are as described previously (Guo et al., Citation2015; Lei et al., Citation2018a). After the sixth immunization, the mice were evaluated using an indirect competitive ELISA (ic-ELISA). Relevant mice, as judged by the titre and half-maximal inhibitory concentration (IC50), were injected with 20 μg of the immunogen to the enterocoelia 3 days before sacrifice, and the spleen cells were extracted and fused with Sp2/0 myeloma cells isolated from respective mice. The hybridoma cells were injected into the mouse abdominal cavity and were inactivated with paroline; the ascites including anti-TMS mAb were collected a week later. Finally, the mAb against TMS was purified from ascites using the saturated ammonium sulfate method and tested by ic-ELISA as described by Ye et al. (Citation2018).
Development of the LF-ICS test device
Colloidal gold nanoparticle (GNP) synthesis
The synthesis of the GNPs was performed as described by Chen et al. (Citation2017a) Briefly, 50 mL of 0.1 g/L chloroauric acid solution in a clean conical flask was heated to boiling and continuously stirred. Then, 2 mL of fresh trisodium citrate solution (1% w/v) was rapidly added and stirring was continued. The solution was kept boiling until it appeared a wine-red colour, then the heat source was removed and allowed to cool to room temperature and the GNPs were stored at 4°C.
Preparation of colloidal gold-labelled mAb
The method of labelling anti-TMS mAb with GNPs was similar to previous studies (Chen et al., Citation2016; Wang et al., Citation2020). Firstly, anti-TMS mAb was diluted to 0.2 mg/mL with 0.01 M CBS, and the GNPs were adjusted to pH 7.0 with 0.1 M K2CO3. Then, 0.2 mL of the mAb was added slowly to the GNP solution (6 mL) and allowed to react for 50 min at room temperature. Next, 0.5 mL of 10% (m/v) BSA was added to block those GNP surfaces not bound by antibody, and 2 h after this the solution was centrifuged at 8000 rcf for 40 min. The sediment at the bottom was then washed two times using 0.02 M PBS solution (pH 7.4, containing 1% BSA, 5% sucrose, and 0.5% polyethylene glycol 6000) to remove excess reagent. Finally, the gold labelled anti-TMS mAb product was redissolved in 3 mL of basic resuspension buffer (0.02 M PBS, 0.1% polyethylene glycol, 2% sorbitol, 5% sucrose, 0.04% NaN3, 0.1% Tween, and 1% mannitol) and stored at 4°C.
Preparation and principle of the LF-ICS
The LF-ICS was prepared based on established methodology derived from our laboratory (Chen et al., Citation2017b; Guo et al., Citation2018). As (a) shows, the LF-ICS is comprised of a support plate, absorbent pad, NC membrane, and sample pad, which are assembled layer by layer in order. There are two capture lines immobilized on the NC membrane, a test line (T-line) sprayed with antigen TMS-1-OVA to capture the free gold-labelled mAb and a control line (C-line) sprayed with goat anti-mouse IgG antibody to capture both gold-labelled mAb and the conjugate of TMS and gold-labelled mAb. After this, the capture lines were dried for 4 h at 37°C, and then cut into strips of 2.8 mm diameter. Finally, the LF-ICS was stored at room temperature for future use.
Figure 2. Schematic illustrations of the LF-ICS. (a) The structure of the LF-ICS, (b) The principle of the LF-ICS test for TMS.
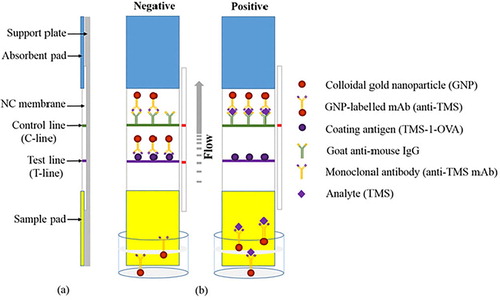
The principle of the LF-ICS is based on competitive binding between the target analyte and the coating antigen to gold-labelled mAb. As shown in (b), the sample solution is mixed with the gold-labelled mAb in microwell plates, and incubated for 5 min at 37°C. When the strip is inserted into the well, the solution flows upwards towards the absorption pad by capillary action, and the result can be observed 7 min later. If the analyte in the sample solution is negative, a red band will appear on the T-line as a result of the binding of the gold-labeled mAb and coating antigen. If the analyte in the sample solution is positive, the red bands on the T-line will be weak because the free colloidal gold-labeled mAb is reduced after reacting with the analyte, and therefore the colour becomes lighter as the analyte increases. The C-line is always red even if analyte in the sample solution is absent as the goat anti-mouse IgG antibody captures not only excess colloidal gold-labeled mAb, but also the compounds within the analyte and the antibody. This implies that the LF-ICS is invalid when the C-line is colourless.
Determination of LF-ICS performance
Sensitivity of the test strip
In this study, the sensitivity of the LF-ICS was measured by using several TMS standard dilutions (0.1, 0.25, 0.5, 1, 2.5, and 5 μg/mL). For each standard 150 μL was added to 50 μL of the gold-labelled mAb solution in the microwell plates and mixed for 5 min at 37°C. Then the strips were put into the solutions, and the results were observed 7 min later. For the LF-ICS, the visual limit of detection (vLOD) was defined as the minimum concentration of TMS producing a visible T-line visible when compared to the negative control group. The cut-off value was defined as the minimum concentration of TMS in which the T-line was colourless.
Sample preparation and analysis using the LF-ICS
TMS-negative fish samples, confirmed by HPLC-MS/MS, were used to test the LF-ICS in this study by adding TMS standards. Specifically, 0.5 mg/mL of TMS stock solution was prepared by dissolving TMS into DMF, and then gradually diluting to 0.2, 0.1, 0.05, and 0.02 mg/mL with DMF. Ten microliters of the above five TMS dilutions and a blank DMF were added to 2 g of negative fish samples respectively. After this, the spiked fish samples were extracted with 10 mL of ethyl acetate using a rotating shaker for 10 min in centrifuge tubes and then centrifuged for 10 min at 8000×g. The supernatants were collected and dried over flowing nitrogen at 50°C. Finally, the extracts were dissolved in 10 ml of PBS (0.5% Tween-20, v/v) and used for the LF-ICS analysis.
Results and discussion
Characterization of antigens and antibody
In view of the weak immunogenicity displayed by small molecules, TMS was coupled with KLH and OVA as the immunogen and coating antigen respectively. As shown in (a), the UV peak shape for TMS-1-KLH at 280 and 355 nm had changed significantly when compared to KLH, which can be influenced by TMS-1 attached to KLH. Similarly, TMS-1-OVA was also significantly different from OVA at 280 nm (b). Therefore, we had confirmed that TMS-1-KLH and TMS-1-OVA had been prepared successfully.
Figure 3. Ultraviolet−visible absorption spectra of immunogen TMS-1-KLH (a) and coating antigen TMS-1-OVA (b).
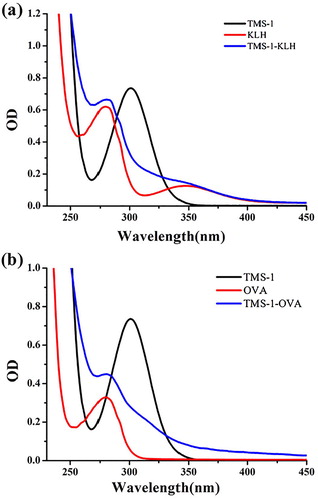
It is well known that a good monoclonal antibody is essential for the preparation of test strips, so our excellent anti-TMS mAb was screened and characterized by ic-ELISA. As shown in , a standard curve was created based on 0.1 μg/mL of TMS-1-OVA and 0.05 μg/mL of the mAb, and the corresponding regression equation used was y = 0.28 + 1.47/(1 + 100x/3179)0.95. The IC50 of TMS was 31.79 ng/mL and the linear range was 7.38−136.95 ng/mL (IC20 to IC80).
Optimization of the test strip
In this study, the performance of the LF-ICS was optimized by testing different volume of 0.1 M K2CO3 added to the GNP solution and the concentration of TMS-1-OVA sprayed onto the T-line. The coating antigen concentration is closely related to the T-line colour and LF-ICS sensitivity, and we found that the red band was invisible when too few coating antigens were immobilized on T-line, whereas too much coating antigen lead to low sensitivity of the LF-ICS seeing that some antibodies were bond to the excess coating antigens fixed weakly on T-line and remove easily by capillarity. Therefore, different TMS-1-OVA concentrations (0.3 and 0.8 mg/mL) were used to test the strips using the TMS standard solutions (0 and 2.5 μg/mL). As shown in , 0.8 mg/mL of TMS-1-OVA resulted in obvious red bands in the TMS-negative samples and a striking contrast was seen between 0 and 2.5 μg/mL of TMS standard solution on the T-line.
Figure 5. Optimization of the LF-ICS in 0.01 M PBS solution. The concentration of TMS-1-OVA (A) 0.3 mg/mL, (B) 0.8 mg/mL. The volume of K2CO3 (a) 8 μL, (b) 10 μL. The concentration of TMS standards: (1) 0 μg/mL, (2) 2.5 μg/mL.
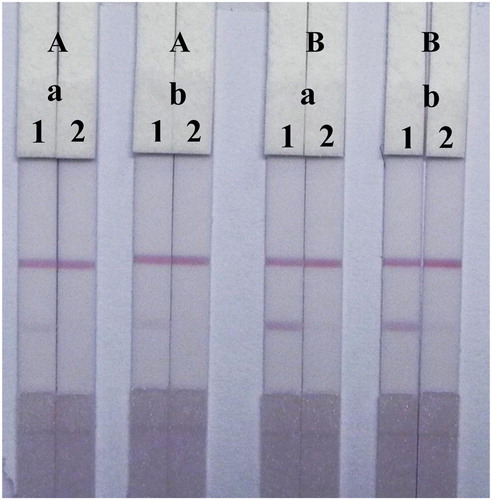
Regardless of the amount of antibody, the main factor affecting assay performance was the amount of 0.1 M K2CO3 which influenced the characteristics of the GNP mAb by adjusting the pH of the solution. Specifically, the positively charged mAb (IgG) can tightly bind to the negatively charged GNPs by electrostatic forces in adaptive alkaline conditions. The result () showed that the colour intensity and sensitivity of the strips were well defined at 0.8 mg/ml of TMS-1-OVA, but the amount of 0.1 M K2CO3 (8 and 10 μL) had no significant effect on the performance of the LF-ICS. This indicated that the GNP mAb was well synthesized and stable in the solutions used both for 8 and 10 μL of 0.1 M K2CO3.
The sensitivity of the LF-ICS assay was optimal when 0.8 mg/ml of TMS-1-OVA was sprayed onto the T-line and 8 μL of 0.1 M K2CO3 was added to the GNP solution. The results are shown in , the colour intensity of the red bands on the T-line gradually faded away with increasing TMS from 0 to 1 μg/mL and vanished completely at 2.5 μg/mL. Therefore, the values for the vLOD and the cut-off value of the LF-ICS for TMS were less than 0.1 and 2.5 μg/mL respectively in 0.01 M PBS (pH 7.4).
Detection of TMS in fish samples
Unlike PBS solutions, residues in fish samples and organic solvents left in the extraction solution affect capillarity action and the binding of antigens and antibodies in the LF-ICS. To reduce this matrix interference, the LF-ICS was optimized by adding six different surfactants (1% of polyethylene glycol, polyvinyl pyrrolidone, BSA, Tween-20, Brij-30, and On-870) to the basic resuspension buffers, and tested using TMS-negative fish extract (0 μg/mL) and TMS-positive fish extract (2.5 μg/mL). As shown in , surfactants enhanced all colour intensities on the C-line of the LF-ICS tested with fish samples. The LF-ICS used with polyvinyl pyrrolidone performed best when compared to the other surfactants, based on colour intensity on the T-line and sensitivity.
Figure 7. Optimization of 1% surfactants in fish sample. Surfactants: (a) polyethylene glycol, (b) polyvinyl pyrrolidone, (c) BSA, (d) Tween-20, (e) Brij-30, (f) On-870. The concentration of TMS standards: (1) 0 μg/mL, (2) 2.5 μg/mL.
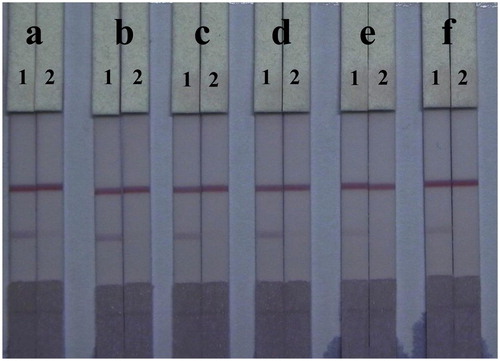
Sensitivity is an important feature for the evaluation of samples using LF-ICS. In this study, we tested our LF-ICS using spiked fish samples. As shown in , TMS in the spiked samples weakened the colour intensity on T-line by competition when compared to the negative sample (0 ng/mL). The value of the vLOD of the LF-ICS for TMS were less than 0.1 μg/mL in fish samples, and the cut-off was 1 μg/mL.
Conclusion
In general, an excellent mAb against TMS was produced based on the hapten TMS-1, and a novel LF-ICS method was developed to detect TMS in fish samples. The amount of K2CO3 added to the GNP solution and the concentration of TMS-1-OVA sprayed onto the T-line were optimized with the aim of strengthening the performance of the LF-ICS in 0.01 M PBS. Surfactants were also optimized to minimize matrix interference in assay samples. The fish samples only need a simple preparation procedure before the LF-ICS test, and results can be obtained within 15 min using the naked eye. As a simple, fast, high-throughput and reliable detection method for TMS, the LF-ICS is a promising assay and could be widely applied to the on-site screening of large numbers of fish samples in the future.
Disclosure statement
No potential conflict of interest was reported by the author(s).
Additional information
Funding
References
- Ahmed, S., Ning, J., Peng, D., Chen, T., Ahmad, I., Ali, A., Lei, Z., Abu bakr Shabbir, M., Cheng, G., & Yuan, Z. (2020). Current advances in immunoassays for the detection of antibiotics residues: A review. Food and Agricultural Immunology, 31(1), 268–290. https://doi.org/10.1080/09540105.2019.1707171
- Cakir, Y., & Strauch, S. M. (2005). Tricaine (MS-222) is a safe anesthetic compound compared to benzocaine and pentobarbital to induce anesthesia in leopard frogs (Rana pipiens). Pharmacological Reports, 57(4), 467–474.
- Carter, K. M., Woodley, C. M., & Brown, R. S. (2011). A review of tricaine methanesulfonate for anesthesia of fish. Reviews in Fish Biology and Fisheries, 21(1), 51–59. https://doi.org/10.1007/s11160-010-9188-0
- Chen, Y., Guo, L., Liu, L., Song, S., Kuang, H., & Xu, C. (2017a). Ultrasensitive immunochromatographic strip for fast screening of 27 sulfonamides in honey and pork liver samples based on a monoclonal antibody. Journal of Agricultural and Food Chemistry, 65(37), 8248–8255. https://doi.org/10.1021/acs.jafc.7b03190
- Chen, Y., Kong, D., Liu, L., Song, S., Kuang, H., & Xu, C. (2016). Development of an ELISA and immunochromatographic assay for tetracycline, oxytetracycline, and chlortetracycline residues in milk and honey based on the class-specific monoclonal antibody. Food Analytical Methods, 9(4), 905–914. https://doi.org/10.1007/s12161-015-0262-z
- Chen, W., Li, X.-n., Wu, Q., Yao, L., & Xu, J. (2019). Rapid and easy determination of morphine in chafing dish condiments with colloidal gold labeling based lateral flow strips. Food Science and Human Wellness, 8(1), 40–45. https://doi.org/10.1016/j.fshw.2018.11.002
- Chen, Y., Liu, L., Xu, L., Song, S., Kuang, H., Cui, G., & Xu, C. (2017b). Gold immunochromatographic sensor for the rapid detection of twenty-six sulfonamides in foods. Nano Research, 10(8), 2833–2844. https://doi.org/10.1007/s12274-017-1490-x
- Cho, G., & Heath, D. (2000). Comparison of tricaine methanesulphonate (MS222) and clove oil anaesthesia effects on the physiology of juvenile chinook salmon oncorhynchus tshawytscha (Walbaum). Aquaculture Research, 31(6), 537–546. https://doi.org/10.1046/j.1365-2109.2000.00478.x
- Guo, L., Song, S., Liu, L., Peng, J., Kuang, H., & Xu, C. (2015). Comparsion of an immunochromatographic strip with ELISA for simultaneous detection of thiamphenicol, florfenicol and chloramphenicol in food samples. Biomedical Chromatography: BMC, 29(9), 1432–1439. https://doi.org/10.1002/bmc.3442
- Guo, L., Wu, X., Liu, L., Kuang, H., & Xu, C. (2018). Gold nanoparticle-based paper sensor for simultaneous detection of 11 benzimidazoles by one monoclonal antibody. Small, 14(6), 1701782. https://doi.org/10.1002/smll.201701782
- Hao, K., Suryoprabowo, S., Song, S., Liu, L., Zheng, Q., & Kuang, H. (2018). Development of an immunochromatographic test strip for the detection of procaine in milk. Food and Agricultural Immunology, 29(1), 1150–1161. https://doi.org/10.1080/09540105.2018.1523371
- Kong, D., Xie, Z., Liu, L., Song, S., Kuang, H., Cui, G., & Xu, C. (2017). Development of indirect competitive ELISA and lateral-flow immunochromatographic assay strip for the detection of sterigmatocystin in cereal products. Food and Agricultural Immunology, 28(2), 260–273. https://doi.org/10.1080/09540105.2016.1263985
- Küçük, S. (2010). Efficacy of tricaine on peocilia latipinna at different temperatures and concentrations. African Journal of Biotechnology, 9(5), 755–759. https://doi.org/10.5897/AJB09.1353
- Lei, X., Song, S., Tao, H., Liu, L., Zheng, Q., Xu, C., & Kuang, H. (2018a). Development of indirect competitive enzyme-linked immunosorbent and immunochromatographic strip assays for tiamulin detection in chicken. ACS Omega, 3(3), 3581–3586. https://doi.org/10.1021/acsomega.8b00289
- Lei, X., Xu, L., Song, S., Liu, L., & Kuang, H. (2018b). Development of an ultrasensitive ic-ELISA and immunochromatographic strip assay for the simultaneous detection of florfenicol and thiamphenicol in eggs. Food and Agricultural Immunology, 29(1), 254–266. https://doi.org/10.1080/09540105.2017.1371114
- Li, J., Liu, H., Yu, M., Wu, L., Wang, Q., Lv, H., Ma, B., & Song, Y. (2014). Rapid determination of tricaine mesylate residues in fish samples using modified QuEChERS and high-performance liquid chromatography-tandem mass spectrometry. Analytical Methods, 6(22), 9124–9128. https://doi.org/10.1039/C4AY00784K
- Nochetto, C. B., Reimschuessel, R., Gieseker, C., Cheely, C.-S., & Carson, M. C. (2009). Determination of tricaine residues in fish by liquid chromatography. Journal of AOAC International, 92(4), 1241–1248. https://doi.org/10.1093/jaoac/92.4.1241
- Palmer, L. M., & Mensinger, A. F. (2004). Effect of the anesthetic tricaine (MS-222) on nerve activity in the anterior lateral line of the oyster toadfish, Opsanus tau. Journal of Neurophysiology, 92(2), 1034–1041. https://doi.org/10.1152/jn.01151.2003
- Rombough, P. J. (2007). Ontogenetic changes in the toxicity and efficacy of the anaesthetic MS222 (tricaine methanesulfonate) in zebrafish (Danio rerio) larvae. Comparative Biochemistry and Physiology Part A: Molecular & Integrative Physiology, 148(2), 463–469. https://doi.org/10.1016/j.cbpa.2007.06.415
- Scherpenisse, P., & Bergwerff, A. A. (2007). Determination of residues of tricaine in fish using liquid chromatography tandem mass spectrometry. Analytica Chimica Acta, 586(1-2), 407–410. https://doi.org/10.1016/j.aca.2006.11.008
- Shen, X., Wu, X., Liu, L., & Kuang, H. (2019). Development of a colloidal gold immunoassay for the detection of four eugenol compounds in water. Food and Agricultural Immunology, 30(1), 1318–1331. https://doi.org/10.1080/09540105.2019.1687658
- Su, M., Sun, X., Yang, C., Peng, X., Liu, H., & Cao, J. (2015). Progress of the research and application of fish anesthetics MS-222 and eugenolon in fresh seafoods transportation. Journal of Food Safety and Quality, 6(1), 25–29.
- Topic Popovic, N., Strunjak-Perovic, I., Coz-Rakovac, R., Barisic, J., Jadan, M., Persin Berakovic, A., & Sauerborn Klobucar, R. (2012). Tricaine methane-sulfonate (MS-222) application in fish anaesthesia. Journal of Applied Ichthyology, 28(4), 553–564. https://doi.org/10.1111/j.1439-0426.2012.01950.x
- Vera, L., Ros-Sanchez, G., Garcia-Mateos, G., & Sanchez-Vazquez, F. J. (2010). MS-222 toxicity in juvenile seabream correlates with diurnal activity, as measured by a novel video-tracking method. Aquaculture, 307(1-2), 29–34. https://doi.org/10.1016/j.aquaculture.2010.06.028
- Wang, Z., Beier, R. C., & Shen, J. (2017b). Immunoassays for the detection of macrocyclic lactones in food matrices–a review. TrAC Trends in Analytical Chemistry, 92, 42–61. https://doi.org/10.1016/j.trac.2017.04.008
- Wang, J., Wang, Y., Pan, Y., Chen, D., Liu, Z., Feng, L., Peng, D., & Yuan, Z. (2017a). Preparation of a generic monoclonal antibody and development of a highly sensitive indirect competitive ELISA for the detection of phenothiazines in animal feed. Food Chemistry, 221, 1004–1013. https://doi.org/10.1016/j.foodchem.2016.11.062
- Wang, Z., Wu, X., Liu, L., Xu, L., Kuang, H., & Xu, C. (2020). Rapid and sensitive detection of diclazuril in chicken samples using a gold nanoparticle-based lateral-flow strip. Food Chemistry, 312, 126116. https://doi.org/10.1016/j.foodchem.2019.126116
- Xie, C., Li, Q., Han, G., Liu, H., Yang, J., & Li, J. (2019). Stable isotope dilution assay for the accurate determination of tricaine in fish samples by HPLC–MS–MS. Biomedical Chromatography, 33(5), e4512. https://doi.org/10.1002/bmc.4512
- Ye, L., Wu, X., Xu, L., Zheng, Q., & Kuang, H. (2018). Preparation of an anti-thiamethoxam monoclonal antibody for development of an indirect competitive enzyme-linked immunosorbent assay and a colloidal gold immunoassay. Food and Agricultural Immunology, 29(1), 1173–1183. https://doi.org/10.1080/09540105.2018.1523373
- Zhao, D.-H., Wang, Q., Wang, X.-F., Li, Z.-G., Li, Y.-X., Huang, K., & Li, L.-D. (2017). Determination of MS-222 in water samples by solid-phase extraction coupled with liquid chromatography/tandem mass spectrometry. Journal of Chromatographic Science, 55(8), 813–817. https://doi.org/10.1093/chromsci/bmx041