ABSTRACT
High-pressure processing as a unique technique of meat preservation is being widely used to preserve the meat and meat products by which a commercially feasible product’s production is possible to meet the consumer’s demand with no chemical preservatives and other harmful substances. Pressure treatment could have effects on colour, texture, protein denaturation, and meat microbial stability at ambient or low temperatures; it can help in minimizing the thermal exposure of the meat product to prevent the quality degradation, reduce microbial contamination and improve tenderness. This paper briefly reviews the attention of the high-pressure processing influence on meat product quality, namely texture, tenderness, colour, and microbial. The principles, HPP equipment, challenges, and future trends in high-pressure processing are also discussed.
1. Introduction
High-pressure processing (HPP) is known as cold pasteurization, which facilitates improvements in meat and meat products’ safety against microbial spoilage (Hugas et al., Citation2002). HPP is an important substitute with consumers’ higher acceptability (Nielsen et al., Citation2009) for hot pasteurization of meat products. The aim of industrial HPP is to stop pathogenic and perishable microorganisms’ growth and increase shelf life, while almost retaining the meat and its products with good characteristics and quality (Balasubramaniam et al., Citation2008; Jofré & Serra, Citation2016).
Heat treatment is the common method by which food processing and preservation are done. The continuous use of heat energy has transformed the food process industry and created a possibility for the long-term preservation of foods. Nevertheless, heat leads to objectionable variations in food nutritional and sensory quality. Therefore, today’s market favours preservation technologies that extend shelf life without harmful effects on the quality characteristics of foods. These types of technologies of food preservation are minimal processing methods. Minimal processing methods include processing procedures that cause a minimal change in the inherent fresh-like quality characteristics of a food item, but prolong the life stability of the food enough to be transported from the food industry to the customers. Among the many minimal processing methods, a non-thermal processing method e.g., high-pressure technology, is of great interest. HPP has gained great acceptance due to its effective commercial use on meat products (Patterson et al., Citation2007).
HHP is a process that is an emerging food processing technology without thermal impact wherein food is exposed to high hydrostatic pressures (200–700 MPa) by a non-compressible fluid (generally water) at reasonable temperatures (characteristically well lower than 100°C). This technology is important in the food manufacturing industry due to its deactivating microorganisms and biocatalysts at ambient or moderately low temperatures without a contrary impact on the flavour, colour and nutritive components of foods compared to thermal treatment (Hoover et al., Citation1989). HPT takes a short time with minimal effect on the sensory and physicochemical properties of food, and it increases safety, and shelf-life and reduces microorganisms to satisfactory levels. Thermal processing methods have been generally used in food processing technology. These methods generally decrease the properties of food to the most essential, but a non-thermal method reduces this damage. It is appropriate for foods containing moisture with vacuum in adaptable packaging. RTE products are good examples of HPP makes. HPP deactivates oxidative biocatalyst and pathogens in foods, and it increases storability to more than two times than other approaches (Abera, Citation2019). HPP extends the shelf-life of shared “lacón,” a cured cooked ready-to-eat product (Del Olmo et al., Citation2014).
Inevitably, sliced meat products suffer from microbiological recontamination throughout slicing and packaging. The evolution of microorganisms existing from this recontamination may limit the innocuous preservation and shelf-life of these products.
Usually, such preservation problems have only a reasonable effect on dry, cured, sliced products. However, it is a more serious concern for those products with high water activity, high pH, and almost no rival bacterial flora capable of delaying the spread of not good microorganisms. The presence and evolution of lactobacilli are among the main problems that cause advanced acidification of the product and limit product shelf-life to a comparatively short period (Gassiot & Masoliver, Citation2010). One of the important features of HPP is that it inactivates spoilage and harmful microbe vegetative cells at low and moderate temperatures without degrading the functional and nutritional food quality (Chen & Hoover, Citation2003; Hugas et al., Citation2002). HPP is mostly performed as a final hygienization step following production and/or packaging activities to promote food product safety and storage stability.
Keeping these issues in mind, HPP has been used to maximize the safety and freshness throughout the product's shelf-life.
2. HPP principles
According to Yordanov and Angelova (Citation2010), there are three basic principles to control food behaviour during high-pressure treatment.
First Le Chatelier’s principle deals with changes in equilibrium due to applying pressure. It states that pressure enhances any process (phase transition, chemical reaction, variation of molecular configuration) followed by a reduction in volume.
Any process resulting in a reduction in volume like a chemical reaction, phase transition, change in permanent geometry of molecules, increased by pressure. Thus, the HPP in reactions favour the outcome of a volume reduction. When the frequency pressure changes, the equilibrium moves to reduce the volume. Thus, the system is moved to the lowest volume (Srinivas et al., Citation2018).
Second, the Isostatic principle, which assumes that a uniform application of pressure operates similarly in all directions (), is the first concern when applying high pressure. When it decompresses food, the food gains its original shape. This special feature of HPP expands processes that are effectively commercialized (Abera, Citation2019; Kumar et al., Citation2018; Sehrawat et al., Citation2021). A good hydrostatic condition should be time- and space-independent. When a fluid is used to distribute pressure throughout the product, it can be determined. In high-pressure applications, irrespective of food shape or size, the pressure and, more crucially, their impacts are transmitted instantly and uniformly within the food product.
Figure 1. The isostatic processing principle (Yordanov & Angelova, Citation2010).
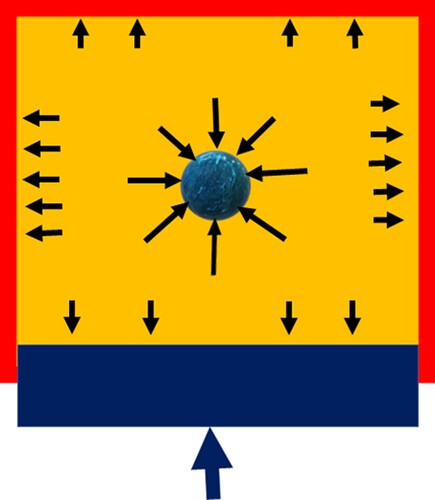
This distinguishing feature has facilitated the creation of commercially effective processes. This concept explains why pressure treatment does not cause macroscopical damage to non-porous products with high water content. Although air and water compress differently under pressure, foods with air pockets may have their structure and form altered by pressure treatment unless the food is elastic and composed of closed-cell foam out of which air could exit.
Third, in the microscopic ordering principle, an increase in pressure expands the degrees of the ordering of molecules of a particular substance at a constant temperature. The temperature and pressure exert opposing influences on molecule structure and chemical reactions (Yordanov & Angelova, Citation2010). It is necessary to consider the net combined pressure-temperature influence on the treated foods product to understand the impact of HPP on foods fully.
Food products undergo a decline due to pressure applied at the compression stage, as shown in . Before decompression, the product is subjected to pressure for just a while. The product will normally return to its original volume when decompression of the product takes place (Farr, Citation1990). when the product is under pressure, the compression and decompression, could cause a transient temperature variation in the product. As an outcome of physical compression, the temperature rises in food.
Figure 2. Pressure and temperature effect profile during HHP of food (Muntean et al., Citation2016).
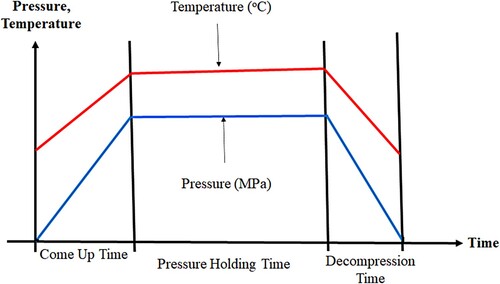
3. Pressure–temperature impact
shows the impact of pressure–temperature on the product. The temperature of the product at process pressure is not dependent on compression degree till heat exchange is reached as minimal or negligible between the product and the surroundings. When a properly insulated (adiabatic) system is decompressed, the product goes back to its previous temperature. However, due to heat losses during the compression phase, the product will revert to a temperature that is slightly lower than its initial temperature. The rapid heating and cooling caused by HPP processing provide a one-of-a-kind approach to enhance the temperature of the material mainly during the treatment and immediately cool it afterward. During room temperature, every 100 MPa of increased pressure raises the water temperature by around 3°C.
Fats and oils have a heat of compression value of 8–9°C per 100 MPa, while proteins and carbs have a heat of compression value of intermediate (Ganessingh et al., Citation2018; Juliano et al., Citation2012; Patazca et al., Citation2007; Rasanayagam et al., Citation2003).
4. HPP equipment
The HPP equipment consists of a high-pressure vessel and its closures, such as a pressure-generation system, a temperature monitoring device, and a material handling system () (Mertens, Citation1995; Van den Berg et al., Citation2001). The most significant component of high hydrostatic-pressure systems is the hydraulic vessel. When designing a vessel, several factors need to be considered. The vessel should be engineered to be dimensionally stable fail safely. If it fails, it should do so with a leak rather than a fracture. The pressure transmitting fluids are employed in the vessel to impart pressure to the products specimen equally and instantly. Water, inert gases, silicone oil, castor oil glycol solutions, sodium, ethanol solutions, and benzoate solutions have been the most often used fluids (Yaldagard et al., Citation2008).
Flexible packaging should be used to pack the food items. The items are stacked and placed into the high-pressure chamber. The vessel is charged with a pressure-transmitting fluid and closed. To facilitate operation and suitability with food products, high pressure is frequently carried out with water as a hydraulic fluid. Compressing the water surrounding the item is the basis of applying high pressure to it. At room temperature, as pressure builds up, the volume of water drops. Because fluid compression creates a minimal volume change, high-pressure vessels that use water do not pose the same operational risks as vessels that use pressurized gases (Balasubramaniam et al., Citation2016).
When the desired condition is achieved, the pump or piston is turned off, the valves are closed, and the pressure may be maintained without any additional energy. The vessel subsequently is decompressed by removing the pneumatically fluid after keeping the item for the required time at the desired pressure. Most items are kept at 600 MPa for 3–5 min in most applications. It is feasible to cycle at a rate of 5–6 cycles every hour, permitting compression, holding, depressurization, filling, and discharging. After pressure treatment, the processed product is taken from the vessel and kept as usual. High pressures can be generated by direct or indirect compressionand heating the pressure fluid (Elamin et al., Citation2015; Heinz et al., Citation2009). Direct compression is achieved by pressurizing a fluid with a piston pushed by a low-pressure pump at its bigger diameter end. The high-pressure dynamic shield between the piston and the internal surface of the vessel limits this procedure to small-diameter laboratory or pilot plant systems, but it permits very speedy pressurization (Farkas & Hoover, Citation2000). Pumping a pressure medium from a storage tank into a sealed, high-pressure vessel until the appropriate pressure is achieved using a high-pressure intensifier is indirect compression (). The pressure medium is heated by expanding the pressure fluid as the temperature increases to generate high pressure. When high pressure is combined with high temperature and very precise temperature control is required throughout the pressure vessel's internal volume, this approach is used (Palou et al., Citation2020).
5. Impact on quality of meat and meat products
5.1 Texture and tenderness
Many investigations have examined the effect of pressure on meat quality since the 1980s. Because of the interest in employing high pressure for beef tenderization, meat texture was the most researched quality feature in early studies (Simonin et al., Citation2012). Meat texture is strongly influenced by the connective tissue (principally collagen) and contractile (primarily actomyosin) systems. Collagen's triple helix is primarily balanced by hydrogen bonds, so, it is relatively inert to pressure under normal conditions. Indeed, pressure treatment at 150 MPa increased the thermostability of tendon collagen , from 58 to 64°C, as expected from the volume increase associated with the rupture of these bonds (Macfarlane, Citation1985). As a result, this baseline toughness is unlikely to be amenable to pressure treatment. The rupture of electrostatic and hydrophobic interactions within protein molecules following the re-development of intra- and intermolecular bonds within anf between protein molecules are the main causes of high-pressure impact on proteins (Galazka et al., Citation1995).
Tenderization of meat generally is caused by multiple transformations within the muscle during conditioning because of natural protease activity: Actin–myosin interactions are impaired, myofibrils are subdivided into smaller segments due to Zline disintegration, the connective tissue is weakened, and elastic filaments containing connectin are damaged (Koohmaraie, Citation1994). The study investigates the impact of high pressure on meat proteins and helps understand why raw meat changes texture and tenderness when subjected to pressure. The high pressure causes myofibril fragmentation to increase, as does ageing since myosin filaments dissociate and -actinin is released (Iwasaki et al., Citation2006). In addition, other changes occur in high pressure-treated meat proteins, which could explain the increase in hardness. When pressures of up to 300 MPa are applied to turkey meat, acto-myosin swells, increasing meat hardness (Scheibenzuber et al., Citation2002). Myofibrillar cross-sections are transformed under pressure of more than 300 MPa: myofibrils become unrecognizable forms, while myofilaments are fragmented (Jung, Ghoul, et al., 2000a; Scheibenzuber et al., Citation2002). To improve tenderness in the beef muscle, subject it for 20–30 min to 150–200 MPa at 60°C (Ma & Ledward, Citation2004; Macfarlane, Citation1973; Sikes et al., Citation2010). Jung, de Lamballerie-Anton, et al. (2000b) showed disorientation of myofibrils in post-rigor beef muscle exposed to high pressure (100–600 MPa, 10°C, 4.3 min); however, this did not lead to an increase in tenderness. It was determined that high pressurization of post-rigor beef was inadequate for getting better tenderness under the conditions tested.
Meat from several animal species changes texture resulting in a reduction in protein volume during HHP treatment. The solubilization of peptide bonds and amino acid-branched chains, volume of internal cavities, and the constitutive volume of atoms, all contribute to the volume of a protein in a solution. Because the interior cavities are compressed when high pressure is applied, the volume of a treated protein is reduced (Sun & Holley, Citation2010).
The structural and textural changes in HPP-treated meats are also associated with the denaturation of proteins (Khan et al., Citation2014; Yang et al., Citation2016). It has been often reported that the principal components of meat responsible for toughness are the myofibrillar and connective tissue proteins. The contribution of the myofibrillar component is greatly influenced by post-slaughter processing procedures, whereas that of the connective tissue proteins is largely determined during the life of the animal. The effects of HP on proteins, such as protein denaturation and aggregation, significant changes in actin–myosin interaction, -actinin release, and denaturation of myofibrillar proteins, could be directly associated with changes in meat texture (Chevalier et al., Citation2001; Guyon et al., Citation2016). HP causes tissue compression by creating new protein interactions (Jantakoson et al., Citation2012), resulting in fibre compression and connective tissue restructuring (Briones-Labarca et al., Citation2012; Hurtado et al., Citation2001). HP-induced tissue and fibre compaction may be attributable to sarcomere length reduction. A possible softening effect associated with the disintegration of myofibril structures has also been identified during HP processing (Ashie & Simpson, Citation1996).
Ma and Ledward (Citation2004) observed the effects on the texture of beef meat and found that when increasing pressure up to 400 MPa causes an increase in hardness, after which there is a light decrease as the pressure is increased further at 20°C. Similarly, when pressure was applied to the cod muscle, the hardness increased up to 400 MPa but decreased (Angsupanich & Ledward, Citation1998).
In meat, the changes in product structure and texture occur due to proteolysis and the solubilization of myofibrillar proteins (Sikes et al., Citation2009). High pressure improves the tenderness of fresh meat (Macfarlane, Citation1973), but increases hardness up to 400 MPa of post-rigor meat (Ma & Ledward, Citation2004) Sikes et al. (Citation2009) showed high pressure used to improve the texture of low-salt beef sausage batters. There was an increase in hardness and fracture strength with increasing pressure, significantly different from the non-pressure-treated control at 200 MPa (0.1 MPa). Hardness and fracture forces declined with increasing pressure between 200 and 400 MPa, and were not different from the control at 400 MPa. Pressure dramatically reduced cohesiveness, which differed from the control at 200 and 400 MPa. The increase in hardness of the duck meat samples treated with high pressure alone was attributed to muscle compaction, whereas the decrease in hardness of the samples treated with high pressure before or after thermal treatment than those of high-pressure-alone samples was attributed to the changes in muscle by modifications in meat protein conformation, protein denaturation, aggregation and gelation (Khan et al., Citation2014).
According to Fernandez et al. (Citation1998), chicken batters treated under 200 MPa resulted in higher hardness and chewiness in the product compared to non-pressure-treated samples, whereas chicken batters treated under 400 MPa having coarser, more irregular, less compact, and aggregated structures. The springiness, cohesiveness, and chewiness were all reduced in these samples. This phenomenon might be described partly because high pressure protects meat proteins against heat denaturation (Fernández-Martín et al., Citation1997).
5.2 Meat colour
Discoloration of poultry, lamb, beef, and pork, is common after high-pressure treatment. The degree of colour change is determined by the meat's chemical and physical state, particularly myoglobin and the atmospheric effects before and after pressurization. The oxidation of the bright red oxymyoglobin or the purple deoxymyoglobin into the brown metmyoglobin, and myoglobin denaturation, are responsible for the diminished redness. Compared to white chicken flesh, beef has a higher myoglobin level, making it more susceptible to discoloration. Furthermore, HP treatment produces denaturation and aggregation of myofibrillar proteins, changing surface reflectance and increased lightness (Bak et al., Citation2019). shows colour variations of different HP-treated meats and meat products at different pressures and temperatures. According to Raman spectroscopy of pork meat pressurized at 600–700 MPa, pressure caused oxymyoglobin to be converted into metmyoglobin, which can be further changed into a denatured ferrous. Because of its brownish appearance, such nonnative ferrous deoxy forms of myoglobin are highly undesirable and are likely to cause oxidative destruction of other such muscle constituents (e.g. lipids). In pork meat, deoxidation of myoglobin was also eventually beneficial since deoxymyoglobin seems to have a high-pressure resistance but can be transformed into a pressure-induced deoxymyoglobin (Buckow et al., Citation2013).
Table 1. Colour variations of different types of HP-treated meats and meat products.
The application of pressure of more than 200 MPa has dramatically altered the appearance of red meat after only a few minutes of treatment at low temperatures (Bak et al., Citation2019; Ledward, Citation2000; Tintchev et al., Citation2010). Major structural proteins in the muscle denatured as pressure were increased (Cheah & Ledward, Citation1996; Zhang et al. Citation2015), leading to an opaque colour of the flesh and further decreasing the visibility of other meat colours. Usually, after pressure treatments exceeding 250 MPa, the lightness (L values) of red meat increases dramatically, while the redness (values) decreases, resulting in grey-brown meat with a cooked appearance (Jung et al., Citation2003; Tintchev et al., Citation2010). Numerous colour changes in cured meat have been observed because of HP treatment. Generally, L* usually increases or stays stable after HP treatment, but a* and b* tend to decrease or remain unaffected. Some studies attribute the increase in lightness to increases in the myofibrilla component, namely protein denaturation resulting in higher light reflectance, comparable to uncured meat. Due to the initial heat denaturation of proteins, no additional increment in L* is detected after HP treatment in cooked, cured meat (Goutefongea et al., Citation1995).
Undoubtedly, the conditions of the HP treatment (most importantly, pressure and temperature) will impact the influence of ham colour. Compared to higher pressures treatment, low pressures of about 300 MPa have minimal impact on colour. Nevertheless, also at low pressures, myoglobin denaturation could be seen in porcine longissimus dorsi (LD) (Bak et al., Citation2012).
Korzeniowski et al. (Citation1999) observed that after 100 MPa HP treatment, 28% of myoglobin had been denatured, increasing to 66% denaturation of myoglobin after 400 MPa HP treatment. The observed colour variations were explained by a rise in the quantity of denatured myoglobin. Consequently, HP treatment at 100 and 200 MPa led to a slight increase in brightness, leading to a red-light colour, but then at 300 MPa, the colour turedn to pale pink, and at 400 MPa, the colour turned to greyish white. Massaux et al. (Citation1999) noticed a change in colour parameters in the case of an increase in L* and a lowering in a* when pressurized the pork LD at low pressures (50, 100, and 200 MPa) Chun et al. (Citation2014) was unable to detect any colour changes in LD successfully treated at pressures lower than 150 MPa. Many investigations have shown a lightness effect threshold pressure of approximately 300–400 MPa. As a result, again, no gain in lightness with increasing pressure could be evaluated beyond 400 MPa (Bak et al., Citation2012; Shigehisa et al., Citation1991; Tintchev et al., Citation2010). This is most probably due to myofibrillar and sarcoplasmic protein denaturation at pressures exceeding 300–400 MPa (Cheah & Ledward, Citation1996).
Minced beef meat is pressurized at 10°C for 10 min. L* colour values increased significantly in the range 200–350 MPa, the meat becomes pink, while a* values decrease at 400–500 MPa, the meat becomes grey-brown. The colour of minced beef meat was pink when pressurized at a pressure above 200 MPa and converted into grey-brown at above 400 MPa. L* values increased between 200and –350 MPa, and a* values decreased as pressure increased, particularly at above 400 MPa, whereas b* values persisted constantly. For minced beef meat pressurized at below 150–200 MPa and 20°C, there was no colour change, L* increased at 150–400 MPa, and a* decreased at above 350 MPa. Meat discoloration under pressure appears to be caused by “whitening” (200–350 MPa) and destruction of bright red (400–500 MPa). Critical pressure thresholds could be caused by various phenomena (Carlez et al., Citation1993, Citation1995).
shows that after treatment under pressures between 200 and 250 MPa and room temperature (20°C), beef, pork, turkey, and chicken meat only altered in brightness, whereas higher pressures led to a significant meat discoloration, ranging from intense red to pale pink (350 MPa) and white and grey colour (450 MPa) (Tintchev et al., Citation2010).
Figure 5. Colour variations of different type of meats under high pressure processing at different pressure (0–600 MPa) for 5 min at room temperature (20°C) (Bak et al., Citation2019).
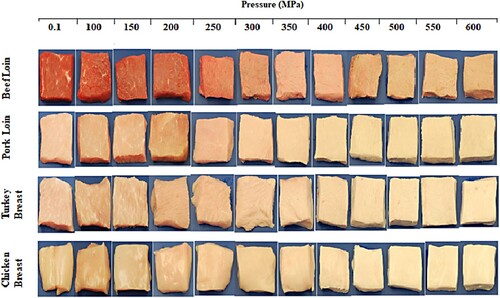
5.3 Microbial meat quality
Pressurized treatment is a food preservation technique that is mild for food while efficiently inactivating spoiling bacteria and foodborne microorganisms in many foods (Argyri et al., Citation2018). This practice was adopted to inhibit the microorganism’s growth and enzymes in muscle foods with minimum impact on flavour, colour, and nutritional quality (Hygreeva & Pandey, Citation2016). On low-sodium sliced vacuum-packaged turkey breast treated with a natural antibacterial agent, a study was conducted to examine the optimization of high hydrostatic pressure (HHP) processing for microbial inactivation (carvacrol). The HHP factors influenced the mortality rates and syneresis of the products investigated (p > 0.05), but not the pH values and lipid oxidation. Treatment at 600 MPa/180 s (at 25°C) is suitable for the tested low-sodium product, according to the required operating conditions for Listeria post-lethality treatment (Oliveira de et al., Citation2019).
Escriu and Mor-Mur (Citation2009) found that Salmonella Typhimurium and L. innocua levels in chicken breasts were reduced immediately after HPP treatment. Yuste et al. (Citation2000) evaluated the effects of pressurization under the treatment of 500 MPa at 65°C for 5 and 15 min, respectively, and heating pasteurization at 80–85°C for 40 min on cooked poultry sausages. They have found that against lactic acid bacteria and Listeria spp., the pressure was more effective than heat, and both treatments were quite efficient against enterobacteria. During the 18-week refrigerated vacuum storage of compressed sausages, Listeria spp. and enterobacteria grew minimally or not at all. HPP is a technology currently adopted by the meat industries to assure the safety of numerous hams or sausages items, as meat-derived goods are increasingly offered vacuum and sliced packaged. High-pressure pasteurization of poultry products and cooked meat is a viable alternative to heat pasteurization.
In general, the higher the pressure and the longer the time, the greater the microbicidal effect. For psychrotrophs and mesophiles, the highest reductions in counts were observed in poultry meat-treated samples at 450 MPa as 6.0 and 2.8 log CFU per gram, respectively (Yuste et al., Citation2002).
Fernandez et al. (Citation2007) discovered that pressurizing beef muscle (M. longissimus dorsi) under 650 MPa at 20°C for ten minutes reduced TVCs. Due to the low initial numbers, no changes in LAB counts were seen in this study, whereas no changes in Enterobacteriaceae counts were found. Considine et al. (Citation2008) used a treatment of 400 MPa at 50°C for 15 min to inactivate a pressure-resistant E. coli. The rate of inactivation observed (5–6 log decline) could not be obtained with just 50°C or 400 MPa. When temperature and HPP are used alone, they can produce significant microbial inactivation, but when these two treatments are combined, inactivation rates are significantly increased, especially for bacterial spores. High-pressure effects on microorganisms are well known and acknowledged. Recent research suggests that cell membrane changes are the most common cause of cell death. At high pressure, ribosome fragmentation has decreased cell viability.
Molds and yeasts are more responsive to pressure than bacterial vegetative cells. Ascospores, on the other hand, could be highly tolerant to extreme pressure. The microorganism species and their growing environment have a great impact on cell sensitivity. It’s more difficult to destroy bacterial spores under pressure. To accomplish total spore inactivation, many combinations of temperature, time, pressure, and cycling treatments could be applied. Most food parasites, in other directions, may be killed by very low pressures (200–300 MPa). A high-pressure treatment under 400–600 MPa, 7–18°C, 5–10 min, is usually effective for falling the enterobacteria number below the detection level in products having high water activity, such as cooked ham, marinated beef loin, or blood sausages (Simonin et al., Citation2012).
The pressure treatment influence on microorganism inactivation and storage life extension of raw pork loin (smoked) and pork ham (cooked) was investigated. These samples were packed in bags (polyethylene) under vacuum and subjected to HPP at 300–600 MPa for 10–30 min. The findings demonstrate that applying 300–400 MPa pressure for 10 min had not prolonged the ham product shelf-life.
Microbiological variables, including total bacterial count, psychrophylic bacteria, acidophylic bacteria, and e. coli in samples tested kept in refrigerator environments for 4 weeks, were significantly reduced by applying 500 MPa for 10 min. The population of all pathogens tested was decreased by 105–106 fold when 600 MPa pressure was applied for 10 min (Karłowski et al., Citation2002).
Pseudomonas fluorescens, Citrobacter freundii, and L. innocua were effectively eradicated by compressing fresh beef products to 200, 280, and 400 MPa for 20 min, respectively. Low (4°C) and high (50°C) holding period temperatures resulted in the greatest inactivation. The same scientists discovered that microbial growth was slowed from 2 to 6 days at 200–300 MPa at 20°C while analyzing the development of bacteria during storage.
Pseudomonas sp., Lactobacillus sp., and coliforms had been totally inactivated during HPP treatment at 400–450 MPa, however, regained after storing at 3°C in the atmosphere. Only Pseudomonas survived storage under vacuum (after 9 days). Cooked beef products were treated under 408, 616, and 888 MPa (maximal temperatures during holding period ranging from 14.4°C to 28.4°C), respectively, with estimated microbiological spoiling durations of 27, 70, and >98 days. Salmonella and L. monocytogenes peaked at 2.5 log CFU/g were not eliminated completely by treating sliced ham at 400 MPa for 10 min at 17°C, although its concentrations were significantly reduced to >1 log CFU/g. Both pathogens were suppressed during the storage period at 1°C. But L. monocytogenes grew to 6 log CFU/g after 84 days at 6°C (Jofré & Serra, Citation2016). Linton et al. (Citation2004) Studied the influences on the microbiological quality of vacuum-packaged, minced treated chicken meat under hydrostatic pressure, and they found a fast rise in the aerobic count of meats after 8 days of storage at 3°C. This demonstrates the potential for HP to enhance the microbiological safety of processed meats. Bover-Cid et al. (Citation2017) reported that HPP affected the reduction of Salmonella enterica of dry-cured ham. When the applied pressure intensity was increased, the rate of elimination of S. enterica levels in dry-cured ham increased. S. enterica lethality was relatively minimal in research conducted at pressures up to 450 MPa, with log reductions ranging between 1.41 and 3.00 CFU per gram. At varying temperatures, 20°C and 40°C, meat samples were compressed under 200, 300, and 400 MPa. For monitoring, the safety of meat, microbiological quality of beef was tested HPP immediately and during 7 days storage period °C. Now after storage, all pressurized samples had bacteria counts that were well below the detection limit. HPP resulted in lower levels of TVCs, indicating a method for improving meat hygiene and increasing the storability of raw meat. At various pressure levels, HPP has been an excellent approach to minimize TVCs in meat. These results show that applying high pressure to meat at low or moderate temperatures enhances its microbial safety while minimizing the impact on the meat’s quality (McArdle et al., Citation2010).
Porto-Fett et al. (Citation2010) evaluated that applying combined a pressure of 483 MPa and a temperature of 19°C for 5 min for dry-fermented Genoa salami, resulted in a 100% decrease of the initial 5 logs (CFU per gram) of E. coli incubated into dry-fermented Genoa salami (characterized by pH values between 4.56 and 4.66 and aw values between 0.884 and 0.940). Numerous studies have already shown that exposing meat and meat products to a pressure of 600 MPa at 20°C for 180 s can kill the causative agents of listeriosis (Listeriamonocytogenes) and inhibit other life-threatening microorganisms such as E. coli, Salmonella, Vibrio, often these types of mould fungi, and pathogenic bacteria with little effect on taste, flavour, texture, appearance, and nutritional value of food (Diachkova et al., Citation2019).
With increasing pressure and pressurization duration, L. innocua survival in chicken meat samples was reduced. The effect of temperature on bacterial survival was not linear, with larger reductions at 0°C and 40°C compared to treatments at 20°C. Pressure, time, temperature, and the interaction of pressure and temperature were all significant parameters. After 10 min of treatment at 400 MPa and 0°C, no bacteria were found. Nevertheless, over 35 days of storage at 3°C, Aerobic Counts grew and surpassed the original level of microbial load (108 cfu/g) in all chicken meat samples (Bulut et al., Citation2014).
In high-pressured chicken patties samples, a total reduction of psychrotrophic microorganisms and lactic acid-forming bacteria growth was identified and a substantial decrease of mesophilic bacteria. HPP treatment under 500 MPa, at 10°C for 10 min, increased the storability of chicken patties by up to 3 weeks (Pietrzak et al., Citation2011). The use of high pressure under 600 MPa at 20°C for 10 min extended the storage life of vacuum-packed ham significantly (Pietrzak et al., Citation2007). The effect of high pressure on the inactivation of microorganisms in different types of meat products is shown in .
Table 2. HHP influences on microorganisms’ inactivation in different types of meat products.
6. Challenges and future trends in HPP
Consumers are increasingly concerned about healthful food, particularly meat products low in salt, nitrite, and polyphosphates. We must evaluate how to balance customer demand with microbial contamination affecting food safety. yet HPP can make it feasible to create meat products with few food additives. The HPP technology boosts the value proposition of products by extending storage life by lowering the microbial load, and maintaining texture, tenderness, and colour. Furthermore, it increases the shelf-life of the meat and meat products, lowers the defect rate, and aids in expanding the meat and meat product's target markets. HPP technique is a popular manufacturing alternative for retaining meat product quality due to its non-thermal processing characteristics. In addition, there are some advantages of this technique: Meat product size and shape do not affect high pressure. High pressure is time-/mass-independent, which means it operates instantly, minimizing processing time. It may be used at room temperature, lowering the amount of heat energy required for meat processing in traditional methods. Meat product is kept uniformly throughout without any particles escaping the treatment because HPP is an isostatic process, and this method is environmentally beneficial because it uses just electricity and produces no waste. But there are some drawbacks: Bacterial spores in meat and meat products are extremely pressure-resistant and require extremely high pressure to be inactivated. Enzymatic and oxidative breakdown of particular meat product components is caused by persistent enzyme activity and dissolved oxygen. To maintain their organoleptic and nutritional properties, numerous pressure-processed foods require low-temperature storage and distribution, and the meat products lack a cooked appearance and can lose flavour quickly. The non-covalent link, covalent bond, and protein structure of myofibrillar proteins were also modified. To be accepted, a full understanding of the HPP principle and its implementation is required. Although it was noted in certain places that there is a distinct kind of meat with its HPP range, the meat suitable/unsuitable for HPP and the packaging material of HPP treated meat should be clearly stated and understood. Consumer approval should also be evaluated. As a result, establishing applicable legislation and regulations is a significant problem for the future expansion of HPP technology in the meat market. Well-defined process conditions will assure microbiological safety, product quality, and compliance with food hygiene rules and regulations in the countries where the manufacturers are situated. HPP technology may potentially be coupled with existing trends in the meat business to help the meat industry grow. For example, high-quality fresh meat and functional, healthy meat are future development prospects in HPP. However, the influencing factors on the qualities of meat under HHP are complicated, and further research is needed.
7. Conclusion
HHP treatment can be used as a promising technique for meat tenderizing and texturizing benefits and to preserve the meat products. The impacts are most typically found in more extreme. HP treatments are textural features changes and discoloration, with mechanisms exhibiting interdependence of processes. High-pressure treatment is a reliable method for inactivating microorganisms and controlling their activity in meat and meat products during storage. Based on microbial load and product nature, pressures from 400 to 600 MPa are usually required to ensure effective microbial inactivation. Much research is now being conducted on the use of high pressure to texturize meat products. It is now popular to adopt high pressure to specific products to achieve combined texturization and pasteurization. High pressure is no longer considered a simple substitute for normal pasteurization, but rather as a method to develop unique meat products. Finally, microbial inactivation with no significant meat colour changes and good texture using HP treatment could offer the meat industry an effective tool to prolong meat product storage life; therefore, market boundaries internationally.
Acknowledgements
We thank HOD, Chemical Engineering, Adigrat University for the assistance.
Disclosure statement
No potential conflict of interest was reported by the author(s).
References
- Abera, G. (2019). Review on high-pressure processing of foods. Cogent Food & Agriculture, 5(1), Article 1568725. https://doi.org/10.1080/23311932.2019.1568725
- Ananth, V., Dickson, J. S., Olson, D. G., & Murano, E. A. (1998). Shelf life extension, safety, and quality of fresh pork loin treated with high hydrostatic pressure. Journal of Food Protection, 61(12), 1649–1656. https://doi.org/10.4315/0362-028X-61.12.1649
- Angsupanich, K., & Ledward, D. A. (1998). High pressure treatment effects on cod (Gadus morhua) muscle. Food Chemistry, 63(1), 39–50. https://doi.org/10.1016/s0308-8146(97)00234-3
- Argyri, A. A., Papadopoulou, O. S., Nisiotou, A., Tassou, C. C., & Chorianopoulos, N. (2018). Effect of high pressure processing on the survival of Salmonella Enteritidis and shelflife of chicken fillets. Food Microbiology, 70, 55–64. https://doi.org/10.1016/j.fm.2017.08.019
- Ashie, I. N. A., & Simpson, B. K. (1996). Application of high hydrostatic pressure to control enzyme related fresh seafood texture deterioration. Food Research International, 29(5-6), 569–575. https://doi.org/10.1016/S0963-9969(96)00012-9
- Aymerich, T., Jofre, A., Garriga, M., & Hugas, M. (2005). Inhibition of Listeria monocytogenes and Salmonella by natural antimicrobials and high hydrostatic pressure in sliced cooked ham. Journal of Food Protection, 68(1), 173–177. https://doi.org/10.4315/0362-028X-68.1.173
- Bak, K. H., Bolumar, T., Karlsson, A. H., Lindahl, G., & Orlien, V. (2019). Effect of high pressure treatment on the color of fresh and processed meats: A review. Critical Reviews in Food Science and Nutrition, 59(2), 228–252. https://doi.org/10.1080/10408398.2017.1363712
- Bak, K. H., Lindahl, G., Karlsson, A. H., & Orlien, V. (2012). Effect of high pressure, temperature, and storage on the color of porcine longissimus dorsi. Meat Science, 92(4), 374–381. https://doi.org/10.1016/j.meatsci.2012.02.002
- Balasubramaniam, V. M., Barbosa-Cánovas, G. V., & Lelieveld, H. L. (2016). High-pressure processing equipment for the food industry. In V. Balasubramaniam, G. Barbosa-Cánovas, & H. Lelieveld (Eds.), High pressure processing of food (pp. 39–65). Springer. https://doi.org/10.1007/978-1-4939-3234-4_3
- Balasubramaniam, V. M., Farkas, D., & Turek, E. (2008). Preserving foods through high-pressure processing. Food Technology, 62(11), 32–38.
- Bover-Cid, S., Belletti, N., Aymerich, T., & Garriga, M. (2017). Modelling the impact of water activity and fat content of dry-cured ham on the reduction of Salmonella enterica by high pressure processing. Meat Science, 123, 120–125. https://doi.org/10.1016/j.meatsci.2016.09.014
- Bover-Cid, S., Belletti, N., Garriga, M., & Aymerich, T. (2011). Model for Listeria monocytogenes inactivation on dry-cured ham by high hydrostatic pressure processing. Food Microbiology, 28(4), 804–809. https://doi.org/10.1016/j.fm.2010.05.005
- Briones-Labarca, V., Perez-Won, M., Zamarca, M., Aguilera-Radic, J. M., & Tabilo- Munizaga, G. (2012). Effects of high hydrostatic pressure on microstructure, texture, colour and biochemical changes of red abalone (Haliotis rufecens) during cold storage time. Innovative Food Science & Emerging Technologies, 13, 42–50. https://doi.org/10.1016/j.ifset.2011.09.002
- Buckow, R., Sikes, A., & Tume, R. (2013). Effect of high pressure on physicochemical properties of meat. Critical Reviews in Food Science and Nutrition, 53(7), 770–786. https://doi.org/10.1080/10408398.2011.560296
- Bulut, S., Chapleau, N., de Lamballerie, M., & Le-Bail, A. (2014). High pressure processing of chicken meat: Change in total aerobic counts after pressure treatment and during chilled storage. Microbiology Research Journal International, 4(5), 540–549. https://doi.org/10.9734/BMRJ/2014/8214
- Campus, M., Flores, M., Martinez, A., & Toldrá, F. (2008). Effect of high pressure treatment on colour, microbial and chemical characteristics of dry cured loin. Meat Science, 80(4), 1174–1181. https://doi.org/10.1016/j.meatsci.2008.05.011
- Carlez, A., Rosec, J. P., Richard, N., & Cheftel, J. C. (1993). High pressure inactivation of Citrobacter freundii. Pseudomonas fluorescens and Listeria innocua in inoculated minced beef muscle. LWT-Food Science and Technology, 26(4), 357–363. https://doi.org/10.1006/fstl.1993.1071
- Carlez, A., Veciana-Nogues, T., & Cheftel, J. C. (1995). Changes in colour and myoglobin of minced beef meat due to high pressure processing. LWT-Food Science and Technology, 28(5), 528–538. https://doi.org/10.1006/fstl.1995.0088
- Cheah, P. B., & Ledward, D. A. (1996). High pressure effects on lipid oxidation in minced pork. Meat Science, 43(2), 123–134. https://doi.org/10.1016/0309-1740(96)84584-0
- Chen, H., & Hoover, D. G. (2003). Modeling the combined effect of high hydrostatic pressure and mild heat on the inactivation kinetics of Listeria monocytogenes Scott A in whole milk. Innovative Food Science & Emerging Technologies, 4(1), 25–34. https://doi.org/10.1016/S1466-8564(02)00083-8
- Chevalier, D., Le Bail, A., & Ghoul, M. (2001). Effects of high pressure treatment (100–200 MPa) at low temperature on turbot (Scophthalmus maximus) muscle. Food Research International, 34(5), 425–429. https://doi.org/10.1016/S0963-9969(00)00187-3
- Chun, J. Y., Min, S. G., & Hong, G. P. (2014). Effects of high-pressure treatments on the redox state of porcine myoglobin and color stability of pork during cold storage. Food and Bioprocess Technology, 7(2), 588–597. https://doi.org/10.1007/s11947-013-1118-4
- Considine, K. M., Kelly, A. L., Fitzgerald, G. F., Hill, C., & Sleator, R. D. (2008). High-pressure processing–effects on microbial food safety and food quality. FEMS Microbiology Letters, 281(1), 1–9. https://doi.org/10.1111/j.1574-6968.2008.01084.x
- De Alba, M., Montiel, R., Bravo, D., Gaya, P., & Medina, M. (2012). High pressure treatments on the inactivation of Salmonella Enteritidis and the physicochemical, rheological and color characteristics of sliced vacuum-packaged dry-cured ham. Meat Science, 91(2), 173–178. https://doi.org/10.1016/j.meatsci.2012.01.015
- Del Olmo, A., Calzada, J., & Nuñez, M. (2014). Effect of high pressure processing and modified atmosphere packaging on the safety and quality of sliced ready-to-eat “lacón”, a cured–cooked pork meat product. Innovative Food Science & Emerging Technologies: IFSET: The Official Scientific Journal of the European Federation of Food Science and Technology, 23, 25–32. https://doi.org/10.1016/j.ifset.2014.03.003
- Del Olmo, A., Morales, P., Ávila, M., Calzada, J., & Nuñez, M. (2010). Effect of single cycle and multiple-cycle high-pressure treatments on the colour and texture of chicken breast fillets. Innovative Food Science & Emerging Technologies, 11(3), 441–444. https://doi.org/10.1016/j.ifset.2010.01.012
- Diachkova, A., Tikhonov, S., & Tikhonova, N. (2019). The effect of high pressure processing on the shelf life of chilled meat and fish. International Journal of Pharmaceutical Research & Allied Sciences, 8(3), 98–108.
- Elamin, W. M., Endan, J. B., Yosuf, Y. A., Shamsudin, R., & Ahmedov, A. (2015). High pressure processing technology and equipment evolution: A review. Journal of Engineering Science & Technology Review, 8(5), 75–83. https://doi.org/10.25103/jestr.085.11
- Escriu, R., & Mor-Mur, M. (2009). Role of quantity and quality of fat in meat models inoculated with Listeria innocua or Salmonella Typhimurium treated by high pressure and refrigerated stored. Food Microbiology, 26(8), 834–840. https://doi.org/10.1016/j.fm.2009.05.011
- Farkas, D. F., & Hoover, D. G. (2000). High pressure processing. Journal of Food Science, 65, 47–64. https://doi.org/10.1111/j.1750-3841.2000.tb00618.x
- Farr, D. (1990). High pressure technology in the food industry. Trends in Food Science & Technology, 1, 14–16. https://doi.org/10.1016/0924-2244(90)90004-I
- Fernandez, P., Cofrades, S., Solas, M. T., Carballo, J., & Colmenero, F. J. (1998). High pressure cooking of chicken meat batters with starch, egg white, and iota carrageenan. Journal of Food Science, 63(2), 267–271. https://doi.org/10.1111/j.1365-2621.1998.tb15723.x
- Fernandez, P. P., Sanz, P. D., Molina-Garcia, A. D., Otero, L., Guignon, B., & Vaudagna, S. R. (2007). Conventional freezing plus high pressure–low temperature treatment: Physical properties, microbial quality and storage stability of beef meat. Meat Science, 77(4), 616−625. https://doi.org/10.1016/j.meatsci.2007.05.014
- Fernández-Martín, F., Fernández, P., Carballo, J., & Jiménez Colmenero, F. (1997). Pressure/heat combinations on pork meat batters: Protein thermal behavior and product rheological properties. Journal of Agricultural and Food Chemistry, 45(11), 4440–4445. https://doi.org/10.1021/jf9702297
- Galazka, V. B., Ledward, D. A., Dickinson, E., & Langley, K. R. (1995). High pressure effects on emulsifying behavior of whey protein concentrate. Journal of Food Science, 60(6), 1341–1343. https://doi.org/10.1111/j.1365-2621.1995.tb04587.x
- Ganessingh, V., Sahibdeen, R., & Maharaj, R. (2018). An evaluation of the impact of novel processing technologies on the phytochemical composition of fruits and vegetables. In T. Asao & M. Asaduzzaman (Eds.), Phytochemicals-source of antioxidants and role in disease prevention. IntechOpen. https://doi.org/10.5772/intechopen.77730
- Garriga, M., Grebol, N., Aymerich, M. T., Monfort, J. M., & Hugas, M. (2004). Microbial inactivation after high-pressure processing at 600 MPa in commercial meat products over its shelf life. Innovative Food Science & Emerging Technologies, 5(4), 451–457. https://doi.org/10.1016/j.ifset.2004.07.001
- Gassiot, M., & Masoliver, P. (2010). Commercial high pressure processing of ham and other sliced meat products at Esteban Espuña, S.A. In Case studies in novel food processing technologies (pp. 21–33). Woodhead Publishing. https://doi.org/10.1533/9780857090713.1.21.
- Goutefongea, R., Rampon, V., Nicolas, N., & Dumont, J. P. (1995, August). Meat color changes under high pressure treatment. 41 International Congress of Meat Science and Technology.
- Guyon, C., Meynier, A., & de Lamballerie, M. (2016). Protein and lipid oxidation in meat: A review with emphasis on high-pressure treatments. Trends in Food Science & Technology, 50, 131–143. https://doi.org/10.1016/j.tifs.2016.01.026
- Hassan, Y., Mészáros, L., Simon, A., Tuboly, E., Mohácsi-Farkas, C., & Farkas, J. (2002). Comparative studies on gamma radiation and high pressure induced effects on minced beef. Acta Alimentaria, 31(3), 253–264. https://doi.org/10.1556/aalim.31.2002.3.6
- Heinz, V., Knoch, A., & Lickert, T. (2009). Product innovation by high pressure processing. New Food, 2, 43–44.
- Hoover, D. G., Metrick, C., Papineau, A. M., Farkas, D. F., & Knorr, D. (1989). Biological effects of high hydrostatic pressure on food microorganisms. Food Technology (Chicago), 43(3), 99–107.
- Huang, M., Moreira, R. G., & Murano, E. (1999). Use of hydrostatic pressijre to produce high quality and safe fresh pork sausage. Journal of Food Processing and Preservation, 23(4), 265–284. https://doi.org/10.1111/j.1745-4549.1999.tb00385.x
- Hugas, M., Garriga, M., & Monfort, J. M. (2002). New mild technologies in meat processing: High pressure as a model technology. Meat Science, 62(3), 359–371. https://doi.org/10.1016/S0309-1740(02)00122-5
- Hurtado, J. L., Montero, P., Borderías, J., & Solas, M. (2001). High-pressure/temperature treatment effect on the characteristics of octopus (Octopus vulgaris) arm muscle. European Food Research and Technology, 213(1), 22–29. https://doi.org/10.1007/s002170100321
- Hygreeva, D., & Pandey, M. C. (2016). Novel approaches in improving the quality and safety aspects of processed meat products through high pressure processing technology – A review. Trends in Food Science & Technology, 54, 175–185. https://doi.org/10.1016/j.tifs.2016.06.002
- Iwasaki, T., Noshiroya, K., Saitoh, N., Okano, K., & Yamamoto, K. (2006). Studies of the effect of hydrostatic pressure pretreatment on thermal gelation of chicken myofibrils and pork meat patty. Food Chemistry, 95(3), 474–483. https://doi.org/10.1016/j.foodchem.2005.01.024
- Jantakoson, T., Kijroongrojana, K., & Benjakul, S. (2012). Effect of high pressure and heat treatments on black tiger shrimp (Penaeus monodon Fabricius) muscle protein. International Aquatic Research, 4(1), 1–12. https://doi.org/10.1186/2008-6970-4-19
- Jofré, A., Aymerich, T., & Garriga, M. (2009). Improvement of the food safety of low acid fermented sausages by enterocins A and B and high pressure. Food Control, 20(2), 179–184. https://doi.org/10.1016/j.foodcont.2008.04.001
- Jofré, A., Garriga, M., & Aymerich, T. (2008). Inhibition of Salmonella sp. Listeria monocytogenes and Staphylococcus aureus in cooked ham by combining antimicrobials, high hydrostatic pressure and refrigeration. Meat Science, 78(1-2), 53–59. https://doi.org/10.1016/j.meatsci.2007.06.015
- Jofré, A., & Serra, X. (2016). Processing of meat products utilizing high pressure. In V. M. Balasubramaniam, V. Barbosa-Cánovas Gustavo, & L. M. Lelieveld Huub (Eds.), High pressure processing of food (pp. 591–623). Springer.
- Juliano, P., Bilbao-Sainz, C., Koutchma, T., Balasubramaniam, V. M., Clark, S., Stewart, C. M., Dunne, C. P., & Barbosa-Cánovas, G. V. (2012). Shelf-stable egg-based products processed by high pressure thermal sterilization. Food Engineering Reviews, 4(1), 55–67. https://doi.org/10.1007/s12393-011-9046-4
- Jung, S., de Lamballerie-Anton, M., & Ghoul, M. (2000). Modifications of ultrastructure and myofibrillar proteins of post-rigor beef treated by high pressure. Food Science and Technology, 33(4), 313–319. https://doi.org/10.1006/fstl.2000.0654
- Jung, S., Ghoul, M., & de Lamballerie-Anton, M. (2000). Changes in lysosomal enzyme activities and shear values of high pressure treated meat during ageing. Meat Science, 56(3), 239–246. https://doi.org/10.1016/s0309-1740(00)00048-6
- Jung, S., Ghoul, M., & de Lamballerie-Anton, M. (2003). Influence of high pressure on the color and microbial quality of beef meat. LWT-food Science and Technology, 36(6), 625–631. https://doi.org/10.1016/S0023-6438(03)00082-3
- Karłowski, K., Windyga, B., Fonberg-Broczek, M., Ścieżyńska, H., Grochowska, A., Górecka, K., & Porowski, S. (2002). Effects of high pressure treatment on the microbiological quality, texture and colour of vacuum packed pork meat products. International Journal of High Pressure Research, 22(3-4), 725–732. https://doi.org/10.1080/08957950212424
- Khan, M. A., Ali, S., Abid, M., Cao, J., Jabbar, S., Tume, R. K., & Zhou, G. (2014). Improved duck meat quality by application of high pressure and heat: A study of water mobility and compartmentalization, protein denaturation and textural properties. Food Research International, 62, 926–933. https://doi.org/10.1016/j.foodres.2014.04.006
- Koohmaraie, M. (1994). Muscle proteinases and meat aging. Meat Science, 36(1–2), 93–104. https://doi.org/10.1016/0309-1740(94)90036-1
- Korzeniowski, W., Jankowska, B., & Kwiatkowska, A. (1999). The effect of high pressure on some technological properties of pork. Electronic Journal of Polish Agricultural Universities, 2(2), 1–8. http://www.ejpau.media.pl/volume2/issue2/food/art-04.html
- Kruk, Z. A., Yun, H., Rutley, D. L., Lee, E. J., Kim, Y. J., & Jo, C. (2011). The effect of high pressure on microbial population, meat quality and sensory characteristics of chicken breast fillet. Food Control, 22(1), 6–12. https://doi.org/10.1016/j.foodcont.2010.06.003
- Kumar, A., Sehrawat, R., Swer, T. L., & Upadhyay, A. (2018). High pressure processing of fruits and vegetables processing. In R. Sehrawat, A. Khan Khursheed, R. Goyal Megh, & K. Paul Prodyut (Eds.), Technological interventions in the processing of fruits and vegetables (pp. 165–184). Apple Academic Press.
- Ledward, D. A. (2000). Effects of pressure on protein structure. International Journal of High Pressure Research, 19(1-6), 1–10. https://doi.org/10.1080/08957950008202528
- Linton, M., McClements, J. M. J., & Patterson, M. F. (2004). Changes in the microbiological quality of vacuum-packaged, minced chicken treated with hydrostatic pressure. Innovative Food Science & Emerging Technologies, 5(2), 151–159. https://doi.org/10.1016/j.ifset.2003.12.004
- López-Caballero, M. E., Carballo, J., & Jiménez-Colmenero, F. (1999). Microbiological changes in pressurized, prepackaged sliced cooked ham. Journal of Food Protection, 62(12), 1411–1415. https://doi.org/10.4315/0362-028X-62.12.1411
- López-Caballero, M. E., Carballo, J., & Jiménez-Colmenero, R. (2002). Microbial inactivation in meat products by pressure/temperature processing. Journal of Food Science, 67(2), 797–801. https://doi.org/10.1111/j.1365-2621.2002.tb10679.x
- Ma, H. J., & Ledward, D. A. (2004). High pressure/thermal treatment effects on the texture of beef muscle. Meat Science, 68(3), 347–355. https://doi.org/10.1016/j.meatsci.2004.04.001
- Macfarlane, J. J. (1973). Prerigor pressurization of muscle. Effect on pH, shear value, and taste panel assessment. Journal of Food Science, 38(2), 294–298. https://doi.org/10.1111/j.1365-2621.1973.tb01409.x
- Macfarlane, J. J. (1985). High pressure technology and meat quality. In R. A. Lawrie (Ed.), Developments in meat science (pp. 155–184). Elsevier Applied Science Publishers.
- Massaux, C., Bera, F., Steyer, B., Sindic, M., & Deroanne, C. (1999). High hydrostatic pressure effects on freezing and thawing processes of pork meat. In H. Ludwig (Ed.), Advances in high pressure bioscience and biotechnology (pp. 497–500). Springer. https://doi.org/10.1007/978-3-642-60196-5_112
- McArdle, R., Marcos, B., Kerry, J. P., & Mullen, A. (2010). Monitoring the effects of high pressure processing and temperature on selected beef quality attributes. Meat Science, 86(3), 629–634. https://doi.org/10.1016/j.meatsci.2010.05.001
- Mertens, B. (1995). Hydrostatic pressure treatment of food: Equipment and processing. In G. W. Gould (Ed.), New methods of food preservation (pp. 135–158). Springer. https://doi.org/10.1007/978-1-4615-2105-1_7
- Moerman, F., Mertens, B., Demey, L., & Huyghebaert, A. (2001). Reduction of Bacillus subtilis, Bacillus stearothermophilus and Streptococcus faecalis in meat batters by temperature high hydrostatic pressure pasteurization. Meat Science, 59(2), 115–125. https://doi.org/10.1016/S0309-1740(00)00145-5
- Muntean, M. V., Marian, O., Barbieru, V., Cătunescu, G. M., Ranta, O., Drocas, I., & Terhes, S. (2016). High pressure processing in food industry–characteristics and applications. Agriculture and Agricultural Science Procedia, 10, 377–383. https://doi.org/10.1016/j.aaspro.2016.09.077
- Nielsen, H., Sonne, A. M., Grunert, K. G., Banati, D., Pollák-Tóth, A., Lakner, Z., Olsen, N. V., Žontar, T. P., & Peterman, M. (2009). Consumer perception of the use of high-pressure processing and pulsed electric field technologies in food production. Appetite, 52(1), 115–126. https://doi.org/10.1016/j.appet.2008.09.010
- Oliveira de, T. L. C., Haddad, G. D. B. S., Ramos, A. D. L. S., Ramos, E. M., Piccoli, R. H., & Cristianini, M. (2019). Optimization of high pressure processing to reduce the safety risk of low-salt ready-to-eat sliced Turkey breast supplemented with carvacrol. British Food Journal, 121(11), 2592–2606. https://doi.org/10.1108/BFJ-10-2018-0646
- Palou, E., López-Malo, A., Barbosa-Canovas, G. V., Swanson, B. G., & Rahman, M. S. (2020). High-pressure preservation of foods. In M. S. Rahman (Ed.), Handbook of food preservation (pp. 843–872). CRC Press.
- Patazca, E., Koutchma, T., & Balasubramaniam, V. M. (2007). Quasi-adiabatic temperature increase during high pressure processing of selected foods. Journal of Food Engineering, 80(1), 199–205. https://doi.org/10.1016/j.jfoodeng.2006.05.014
- Patterson, M. F., Linton, M., & Doona, C. J. (2007). Introduction to high pressure processing of foods. In C. J. Doona & F. E. Freeherry (Eds.), High Pressure Processing of Foods (pp. 1–14). Blackwell Publishing Professional.
- Patterson, M. F., Mackle, A., & Linton, M. (2011). Effect of high pressure, in combination with antilisterial agents, on the growth of Listeria monocytogenes during extended storage of cooked chicken. Food Microbiology, 28(8), 1505–1508. https://doi.org/10.1016/j.fm.2011.08.006
- Pietrzak, D., Cegiełka, A., Fonberg-Broczek, M., & Ziarno, M. (2011). Effects of high pressure treatment on the quality of chicken patties. High Pressure Research, 31(2), 350–357. https://doi.org/10.1080/08957959.2011.552503
- Pietrzak, D., Fonberg-Broczek, M., Mucka, A., & Windyga, B. (2007). Effects of high pressure treatment on the quality of cooked pork ham prepared with different levels of curing ingredients. High Pressure Research, 27(1), 27–31. https://doi.org/10.1080/08957950601091087
- Pingen, S., Sudhaus, N., Becker, A., Krischek, C., & Klein, G. (2016). High pressure as an alternative processing step for ham production. Meat Science, 118, 22–27. https://doi.org/10.1016/j.meatsci.2016.03.014
- Porto-Fett, A. C. S., Call, J. E., Shoyer, B. E., Hill, D. E., Pshebniski, C., Cocoma, G. J., & Luchansky, J. B. (2010). Evaluation of fermentation, drying, and/or high-pressure processing on viability of Listeria monocytogenes. Escherichia coli O, 157, H7, Salmonella spp., and Trichinella spiralis in raw pork and Genoa salami. International Journal of Food Microbiology, 140(1), 61–75. https://doi.org/10.1016/j.ijfoodmicro.2010.02.008
- Rasanayagam, V., Balasubramaniam, V. M., Ting, E., Sizer, C. E., Bush, C., & Anderson, C. (2003). Compression heating of selected fatty food materials during high- pressure processing. Journal of Food Science, 68(1), 254–259. https://doi.org/10.1111/j.1365-2621.2003.tb14148.x
- Rubio, B., Martinez, B., García-Cachán, M. D., Rovira, J., & Jaime, I. (2007). Effect of high pressure preservation on the quality of dry cured beef “Cecina de Leon”. Innovative Food Science & Emerging Technologies, 8(1), 102–110. https://doi.org/10.1016/j.ifset.2006.08.004
- Rubio, R., Bover-Cid, S., Martin, B., Garriga, M., & Aymerich, T. (2013). Assessment of safe enterococci as bioprotective cultures in low-acid fermented sausages combined with high hydrostatic pressure. Food Microbiology, 33(2), 158–165. https://doi.org/10.1016/j.fm.2012.09.012
- Ruiz-Capillas, C., Colmenero, F. J., Carrascosa, A. V., & Muñoz, R. (2007). Biogenic amine production in Spanish dry-cured “chorizo” sausage treated with high-pressure and kept in chilled storage. Meat Science, 77(3), 365–371. https://doi.org/10.1016/j.meatsci.2007.03.027
- Scheibenzuber, M., Ruß, W., Görg, A., & Meyer-Pittroff, R. (2002). Scanning electron microscopic study of high pressure induced microstructural changes of proteins in Turkey and pork meat. In Progress in Biotechnology (Vol. 19, pp. 545–550). Elsevier. https://doi.org/10.1016/S0921-0423(02)80151-4
- Sehrawat, R., Kaur, B. P., Nema, P. K., Tewari, S., & Kumar, L. (2021). Microbial inactivation by high pressure processing: Principle, mechanism and factors responsible. Food Science and Biotechnology, 30(1), 19–35. https://doi.org/10.1007/s10068-020-00831-6
- Shigehisa, T., Ohmori, T., Saito, A., Taji, S., & Hayashi, R. (1991). Effects of high hydrostatic pressure on characteristics of pork slurries and inactivation of microorganisms associated with meat and meat products. International Journal of Food Microbiology, 12(2-3), 207–215. https://doi.org/10.1016/0168-1605(91)90071-V
- Sikes, A., Tornberg, E., & Tume, R. (2010). A proposed mechanism of tenderising post-rigor beef using high pressure–heat treatment. Meat Science, 84(3), 390–399. https://doi.org/10.1016/j.meatsci.2009.09.007
- Sikes, A. L., Tobin, A. B., & Tume, R. K. (2009). Use of high pressure to reduce cook loss and improve texture of low-salt beef sausage batters. Innovative Food Science & Emerging Technologies, 10(4), 405–412. https://doi.org/10.1016/j.ifset.2009.02.007
- Simonin, H., Duranton, F., & De Lamballerie, M. (2012). New insights into the highpressure processing of meat and meat products. Comprehensive Reviews in Food Science and Food Safety, 11(3), 285–306. https://doi.org/10.1111/j.1541-4337.2012.00184.x
- Simpson, R. K., & Gilmour, A. (1997). The resistance of Listeria monocytogenesto high hydrostatic pressure in foods. Food Microbiology, 14(6), 567–573. https://doi.org/10.1006/fmic.1997.0117
- Srinivas, M. S., Madhu, B., Srinivas, G., & Jain, S. K. (2018). High pressure processing of foods. A review. The Andhra Agricultural Journal, 65, 467–476.
- Sun, X. D., & Holley, R. A. (2010). High hydrostatic pressure effects on the texture of meat and meat products. Journal of Food Science, 75(1), R17–R23. https://doi.org/10.1111/j.1750-3841.2009.01449.x
- Tintchev, F., Wackerbarth, H., Kuhlmann, U., Toepfl, S., Knorr, D., Hildebrandt, P., & Heinz, V. (2010). Molecular effects of high- pressure processing on food studied by resonance Raman. Annals of the New York Academy of Sciences, 1189(1), 34–42. https://doi.org/10.1111/j.1749-6632.2009.05204.x
- Van den Berg, R. W., Hoogland, H., Lelieveld, H. L. M., & Van Schepdael, L. (2001). High pressure equipment designs for food processing applications. In Ultra high pressure treatments of foods (pp. 297–313). Springer. https://doi.org/10.1007/978-1-4615-0723-9_11
- Yaldagard, M., Mortazavi, S. A., & Tabatabaie, F. (2008). The principles of ultra high pressure technology and its application in food processing/preservation: A review of microbiological and quality aspects. African Journal of Biotechnology, 7(16), 2739–2767. https://doi.org/10.5897/AJB07.923
- Yang, H., Han, M., Wang, X., Han, Y., Wu, J., Xu, X., & Zhou, G. (2015). Effect of high pressure on cooking losses and functional properties of reduced-fat and reduced-salt pork sausage emulsions. Innovative Food Science & Emerging Technologies, 29, 125–133. https://doi.org/10.1016/j.ifset.2015.02.013
- Yang, H., Khan, M. A., Yu, X., Zheng, H., Han, M., Xu, X., & Zhou, G. (2016). Changes in protein structures to improve the rheology and texture of reduced-fat sausages using high pressure processing. Meat Science, 121, 79–87. https://doi.org/10.1016/j.meatsci.2016.06.004
- Yordanov, D. G., & Angelova, G. V. (2010). High pressure processing for foods preserving. Biotechnology & Biotechnological Equipment, 24(3), 1940–1945. https://doi.org/10.2478/V10133-010-0057-8
- Yuste, J., Pla, R., Capellas, M., & Mor-Mur, M. (2002). Application of high-pressure processing and nisin to mechanically recovered poultry meat for microbial decontamination. Food Control, 13(6-7), 451–455. https://doi.org/10.1016/S0956-7135(01)00071-8
- Yuste, J., Pla, R., Capellas, M., Ponce, E., & Mor-Mur, M. (2000). High-pressure processing applied to cooked sausages: Bacterial populations during chilled storage. Journal of Food Protection, 63(8), 1093–1099. https://doi.org/10.4315/0362-028X-63.8.1093
- Yuste, J., Pla, R., Capellas, M., Sendra, E., Beltran, E., & Mor- Mur, M. (2001). Oscillatory high pressure processing applied to mechanically recovered poultry meat for bacterial inactivation. Journal of Food Science, 66(3), 482–484. https://doi.org/10.1111/j.1365-2621.2001.tb16135.x
- Zhang, Z., Yang, Y., Tang, X., Chen, Y., & You, Y. (2015). Chemical forces and water holding capacity study of heat-induced myofibrillar protein gel as affected by high pressure. Food Chemistry, 188, 111–118. https://doi.org/10.1016/j.foodchem.2015.04.129